Tsunami Warning System: Preparing for the unpredictable
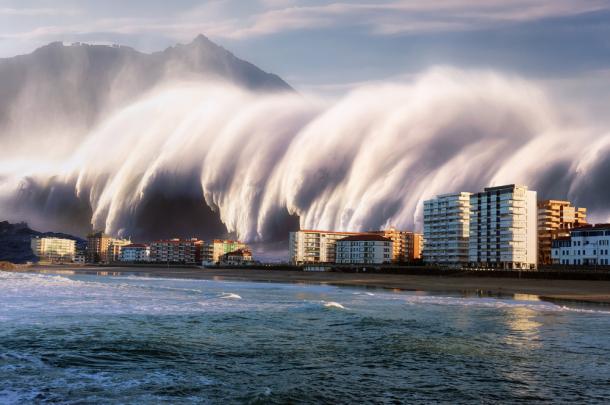
UNESCO is the UN Agency in charge of ocean science. With its Intergovernmental Oceanographic Commission joined by 150 Member States, and its expertise in the culture and education fields, UNESCO coordinates actions by governments, scientists, the private sector, civil society and other UN organizations. Together, we created the tsunami warning system. We map the ocean depths, identify species, work to ensure that ocean literacy is included in school curricula and protect ocean sites, which are home to critical biodiversity and incomparable beauty. In this story, we tell you more about UNESCO’s work on the tsunami early warning system, how it works and how it saves lives.
Tsunamis are rare events. But when nature’s fury is unleashed, their deadly effects are devastating. The initial impact may make the front page of news media, but the aftermath on communities, livelihoods and the environment will linger for many years after the natural disaster strikes.
In the last century, 58 of them have claimed more than 260,000 lives, surpassing any other natural hazard. More are expected in the future as the sea-level rises due to climate change.
Therefore, preparing for the unpredictable can mean the difference between surviving and not.
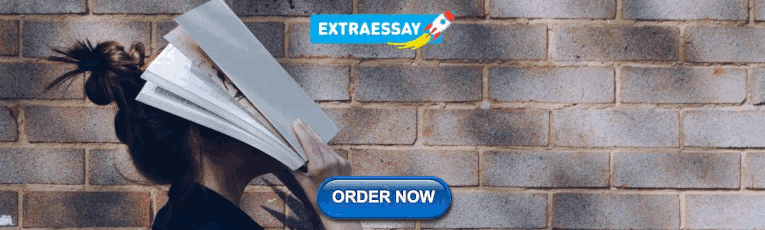
11 March, 14:00 UTC: the Earth shakes
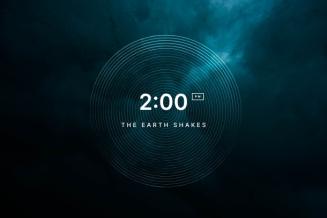
An earthquake occurs in the Atlantic, 100 kilometres east of the Lesser Antilles – the long, delicate arc of small Caribbean islands fanning out between the Caribbean Sea and the open ocean. The volcanic archipelago, home to 3.2 million people, is perched on one of the tectonic plates that sit on the Earth’s crust.
Just as the plate slips along the Caribbean fault line, a massive burst of energy akin to a nuclear warhead explosion sets off a giant shockwave 25 kilometres beneath the planet’s surface.
Strong earthquakes are highly destructive in their own right. But they can also trigger other cataclysmic natural hazards.
As the seabed suddenly rises, it displaces colossal volumes of water, producing powerful waves that spread outward in all directions, just like the ripples from a stone thrown into a pond.
The smaller, ocean-facing Antilles are on the frontline of the looming wave. The larger islands – Cuba, Haiti, Jamaica and Puerto Rico – as well as the communities in the Gulf of Mexico and coastal Venezuela, are also under threat. Nearly 160 million people are in imminent danger.
Tsunami Waves : digitally-scanned photos from the ITIC collection
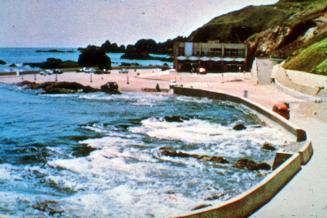
11 March, 14:02 UTC: the earthquake is detected, tsunami sensors activate
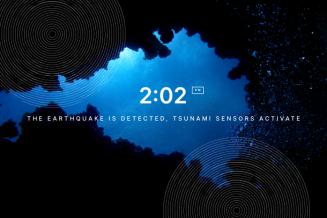
The monitoring station in Martinique picks up the tremor and estimates it at 8.5 on the Richter scale. The large magnitude is cause for concern. Major earthquakes that occur beneath the sea lead to deadly tsunamis. Still, visual detection in the vast open ocean remains challenging because the powerful tsunami waves are low in height while they travel across deep water.
That is why shore-based tide gauges and deep-ocean buoys continuously monitor the oceans to detect any threatening changes. These silent sentinels can spy and track any minuscule change in the temperature of the seafloor and its pressure.
An ocean buoy anchored in the depths of Barbuda – a flat coral island hugged by white-and-pink sand beaches and crystal-clear waters – senses the force of the hidden submarine wave and alerts the monitoring centre on the sister island of Antigua.
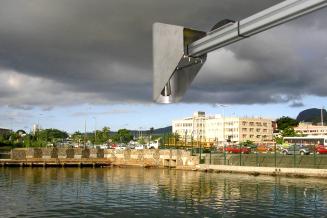
11 March, 14:05 UTC: the Tsunami Warning System raises the alarm
Antigua feeds the data on the force of the approaching wave into the Tsunami Warning System, which alerts all observatories in the region. Authorities in all neighbouring countries are immediately alerted: the rapid transmission of information to dedicated centres is vital to lessen the damage caused by tsunamis.
Disaster prediction and prevention Preventing large-scale disasters calls for a high degree of international and multilateral cooperation. After the 1960 Chilean tsunami, which left a trail of death and damage as far away as Japan, UNESCO’s Intergovernmental Oceanographic Commission (IOC-UNESCO) stepped in to set up the Pacific Ocean Tsunami Warning System, the first of its kind.
The 9.5 magnitude earthquake in Chile, the largest recorded in the 20th century, set off a tsunami that battered the South American coastline for over 4,000 kilometres with waves up to 25 metres high.
Fifteen hours later, the tsunami, which by then had travelled 10,000 kilometres, struck Hawaii, then Japan and the Philippines. The final death toll was over 2,000.
The scale of the disaster highlighted the need for a warning alert system in the Pacific, where most of the world’s deadliest tsunamis occur. Over the years, the alert system has evolved beyond issuing warnings. UNESCO’s role now includes prevention, preparing communities to respond to tsunami threats and fostering the latest tracking and detection technologies.
Other exposed regions, the Indian Ocean, the Caribbean, the Northeast Atlantic and the Mediterranean, have also adopted Early Tsunami Warning Systems based on the Pacific model.
11 March, 14:15 UTC: public alerts go out
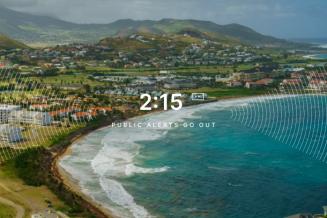
The tidal wave is already in sight of the Lesser Antilles. There is little time left before it hits the coast. Tsunami waves in the deep ocean can travel thousands of kilometres, up to 800 kilometres per hour, the speed of a jet aircraft.
In Martinique, the local government alerts the municipalities and the media, which immediately publish alerts. Police sirens and loudspeakers give evacuation orders. Guided by their teachers, schoolchildren rush out of their classrooms, heading for higher ground. Office workers and tourists seek safety on the rooftops of high-rise buildings.
Empowering communities to react An early warning system can be effective only when the population is well aware of the tsunami phenomenon and knows what to do in case of an emergency.
This is vital when tsunamis are generated close to the coast and there may not be time for official evacuation orders.
As part of IOC-UNESCO coordination plans, communities are empowered to play an active role through self-evacuation if a strong earthquake is felt or a strong roaring sound – similar to a train or a jet aircraft – is heard. Highly visible and labelled evacuation routes help show the best access to nearby higher grounds or the higher floors of tsunami-proof buildings.
11 March, 14:16 UTC: the sea level drops
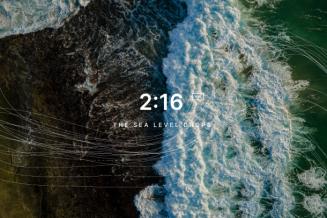
As the tsunami approaches the coast, the sea is drawn back due to the vacuum effect caused by the wave. The water along the shoreline of Anguilla, the most northerly of the Leeward Islands in the Lesser Antilles, is dragged back dramatically, exposing the shallow coral reef and stranding many marine creatures.
The expanding shoreline is nature’s warning that a tsunami is approaching. It is a sign that there are only seconds or, at best few minutes, before the full impact of the first wave.
When the sea disappears Survivors of the 2004 Indian Ocean tsunami said the water had receded for up to 2.5 kilometres along the coastlines of Indonesia and Thailand. Bystanders, many of them children, lingered on the exposed beach to observe the phenomenon and collect stranded fish.
The lack of tsunami awareness, combined with the absence of a coordinated alert system, contributed to the high death toll. There were an estimated 227,000 fatalities in 14 countries, with India, Indonesia, Sri Lanka and Thailand being the hardest-hit.
Still, some coastal communities in Indonesia were able to evacuate despite the lack of alarms, thanks to local traditions and folklore developed during previous tsunami disasters.
The tragedy highlighted the importance of understanding the early signals of an approaching tsunami.
For example, the "disappearing sea" may not happen at all. Sometimes the sea suddenly swells without any warning signs, surprising people and giving them little time to flee.
Tsunamis can be detected using the human senses
Tsunami evacuation signs.
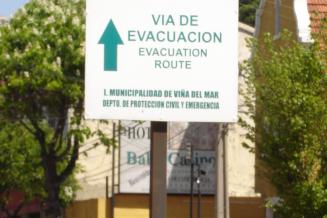
11 March, 14:20 UTC: the first wave hits
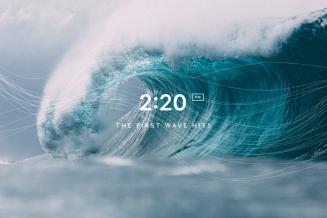
When the swell approaches the shore, the leading edge of the wave begins to slow down in shallow waters. As the tsunami loses its speed nearing the coast, the first wave suddenly swells as much as 20 metres in height. The massive wall of water rushes towards the coastline, demolishing everything in its way. The force of the wave is powerful enough to overturn boats, crumble palm trees and sweep away beach shacks.
Why is it called tsunami ? The flatter the coast, the stronger is the impact from the waves. This is the reason why the effects of the tsunamis are more devastating in ports, beaches and in the mouths of the rivers. It also explains the origin of the word. In Japanese, tsunami means bay or harbour wave.
11 March, 14:40 UTC: the second wave hits
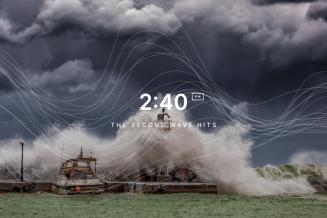
Tsunamis always surge in multiple waves. The first wave may not be the largest, and often it is the second or later waves that are the biggest.
The second wave, towering at 30 metres, hits after as little as five minutes. The coastal areas are completely devastated and under water.
We heard a second wave, and another. There were no houses anymore.
A tsunami survivor
In 1950, Markus Kailhulu was a 12-year-old living in the village of Hutumuri in Indonesia, when a tsunami hit the Moluccas. The villagers had evacuated to higher ground and witnessed the destruction brought by the waves.
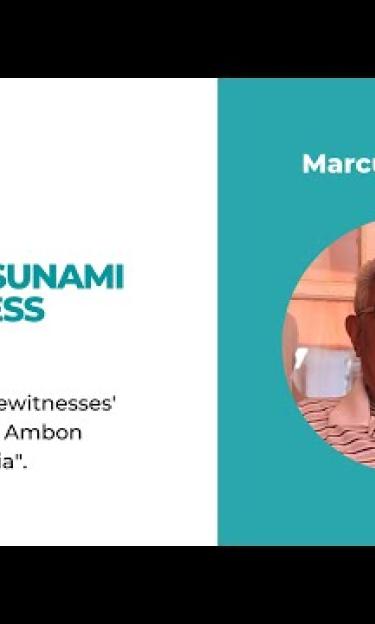
We went to see and it looked like a flood from up there. We heard a second wave, and another. There were no houses anymore, all gone,’ he says. ‘The waves swept it all. It hit the edge of the mountain, it went back while taking the houses. The church was the only building left.
11 March, 15:00 UTC: other waves hit
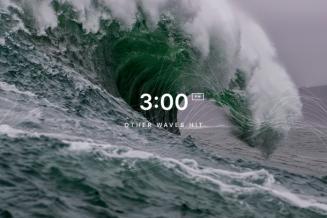
The first two waves were massive walls of water. The others now resemble a surging tide that inundates coastal areas, carrying debris from the destruction caused by previous waves.
Entire neighbourhoods have been washed away. Up on the hills, people stare at the devastation below with fear and incomprehension.
World Tsunami Awareness Day People experiencing a tsunami should be aware that the danger may not have passed and should await official confirmation that it is safe to return. Raising awareness and education among the coastal communities is essential to prepare citizens on how to respond to the risk of tsunamis and cope with their aftermath. The UN-supported World Tsunami Awareness Day is the brainchild of Japan, which due to its repeated experience with tsunamis, has built up over the years major expertise in early warnings and public awareness to reduce future impacts. The event, held every year on 5 November, calls on countries, international bodies and civil society to raise tsunami awareness and share innovative approaches to reduce the death toll and devastation. Posters, flyers, e-learning courses and guidelines as well as games teach children, who are among the most vulnerable groups, how to identify and cope with a tsunami.
World Tsunami Awareness Day
"The game is fun and worth a try." Tsunami Ready board game, World Tsunami Awareness Day 2021, Indian Ocean.
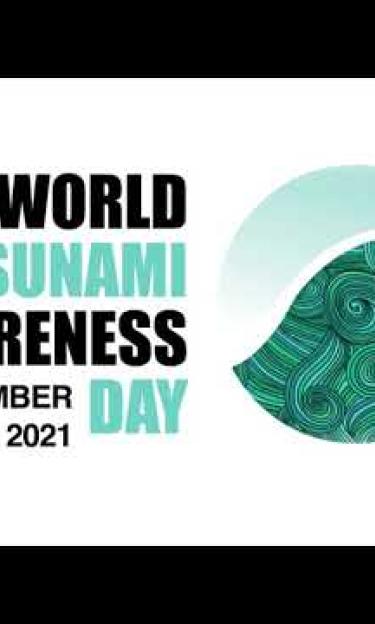
Playing the Tsunami Ready game
The Indian Ocean Tsunami Information Centre has developed a Tsunami Ready board game for children living in the coastal communities. "Playing the game, I’m able to learn a lot, such as what are the mitigation efforts that we can do at community, family as well as individual levels," says Sasa Tsairoo, a young game player who took part in the World Tsunami Awareness Day in 2021. "The game is fun and worth a try."
11 March, 19:43: rescue and recovery begins
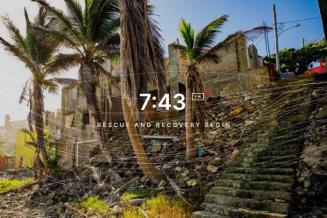
Local authorities issue an "all clear" that it’s safe to return to the coastal areas. People rush out into the streets, stunned. In the midst of flooding and devastation, search and rescue teams are busy across the archipelago in a desperate attempt to find survivors. Essential utilities like water, telecommunications, gas lines and electricity are inoperable. The coastline is devastated with flooding, damaged buildings, debris, fires and hazardous spills. Many are missing. Many more have lost their homes and may have to stay in shelters or public buildings until the reconstruction begins.
Reality or fiction? This report is, in fact, the fictional scenario of a tsunami in the Caribbean, based on a drill exercise.
Caribe Wave is an annual tsunami preparedness exercise set up by the United Nations and overseen by IOC-UNESCO. The date and time of the simulation are not a random choice: it is the anniversary of the Japan earthquake and tsunami that killed nearly 16,000 people on 11 March 2011.
Under IOC-UNESCO’s oversight, the drills allow different countries and territories, emergency management agencies and communities at risk to test, validate and update their tsunami response plans.
The exercise, which in 2019 involved up to 800,000 people to simulate a catastrophic scenario, focuses on the coordination among countries, improving response procedures and training the local population to become prepared.
It also plays a crucial role in fostering resilient communities. Tsunamis are a real threat in the Caribbean. At least 75 have hit the region over the past 500 years. Some countries facing the Gulf of Mexico are also exposed to the double threat of tsunamis along their Pacific coastlines.
Thanks to Caribe Wave, over 50 coastal communities are now considered Tsunami Ready. This means that these communities now have the tools to face not just tsunamis, but also other coastal hazards.
Tsunami Ready recognition As of 2021, six countries have piloted UNESCO’s Tsunami Ready Programme, while seven more are in progress. The programme aims to build resilient communities through awareness and preparedness strategies that will protect life, livelihoods and property from future tsunamis.
One of the achievements in becoming Tsunami Ready for us in Saint Kitts and Nevis was the ability to enhance our disaster preparedness. That was very vital and critical in also encompassing coastal hazards
"The ability to enhance our disaster preparedness" – World Tsunami Awareness Day 2021, Saint Kitts and Nevis
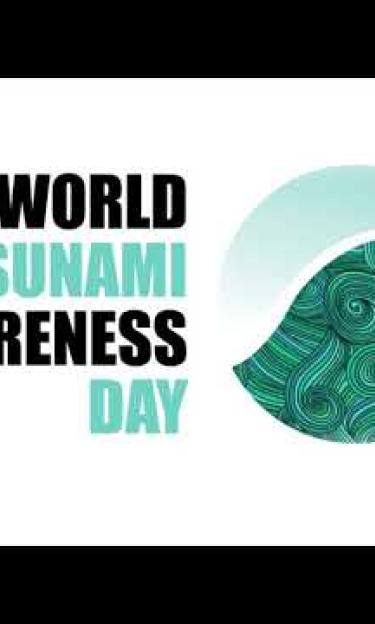
Preparing for future tsunamis
Millions of people live in coastal areas across the world where the rising sea level is increasing the risk of tsunamis.
In 2021, the United Nations set the goal of making all at-risk communities Tsunami Ready by 2030. IOC-UNESCO Tsunami Ready recognition has shown how different countries and communities can work together to reduce the risk of catastrophic coastal hazards that can cause death and destruction, hitting the livelihoods of vulnerable populations.
By improving warnings, enhancing preparedness and practicing response drills, these communities can prepare and become resilient, together.
"The Tsunami Ready programme reduces the risk for our communities." World Tsunami Awareness Day 2021
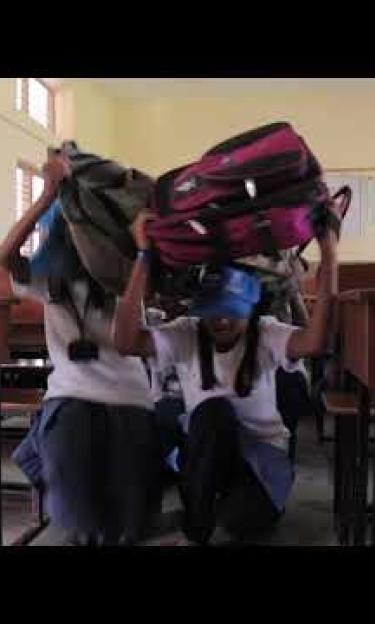
Related items
- Warning systems

Tsunami Warning and Preparedness: An Assessment of the U.S. Tsunami Program and the Nation's Preparedness Efforts (2011)
Chapter: 1 introduction, chapter one introduction, the tsunami threat in the united states.
The 2004 Indian Ocean tsunami resulted in catastrophic losses of life and property and demonstrated how destructive tsunamis can be. More than 200,000 people died, with most occurring in Indonesia, which was near the tsunami source, but deaths were also reported in countries as far away as Somalia. Recently, the Samoan (September 2009) and Chilean (February 2010) tsunamis reminded the world of how quickly a tsunami can move onshore and destroy lives. In comparison to extreme weather—such as floods, hurricanes, or tornadoes—tsunamis have caused comparatively few fatalities in the United States over the past 200 years. Modern records kept since 1800 tally less than 800 lives lost due to tsunamis in the United States and territories. 1 In 1960, a magnitude 9.5 Chilean earthquake generated tsunami waves that killed 61 people and caused $24 million in property damage in Hilo, Hawaii (Eaton et al., 1961). The 1964 Good Friday earthquake in Alaska generated a tsunami that devastated local Alaskan communities and inundated distant communities as far south as Crescent City, California.
Earlier tsunamis—yet to be repeated in modern times—include tsunami waves of North American origin in the year 1700 that caused flooding and damage as far away as Japan. Paleo-records indicate that the Cascadia subduction zone off the Washington, Oregon, and northern California coasts has repeatedly generated potentially catastrophic tsunamis (Atwater et al., 2005). Because of the relative infrequency of catastrophic tsunamis in recent U.S. history, mobilizing the required resources to maintain the nation’s warning and preparedness capabilities is challenging.
Tsunamis are caused by a variety of geological processes, such as earthquakes, subaerial and submarine landslides, volcanic eruptions, or very rarely from meteorite impacts ( Box 1.1 ). However, it takes a large event (e.g., typically an earthquake of magnitude greater than 7.0) to generate a damaging tsunami. Therefore, determining the likelihood of future tsunamis for U.S. coastal communities requires an understanding of the likelihood of reoccurrence of such geological processes, the likely magnitude of such events, and the location of the sources (see Chapter 3 for additional details). Because most tsunamis result from earthquakes, the tsunami hazard is high along U.S. shores that adjoin boundaries between tectonic plates, particularly along the subduction zones of Alaska, the Pacific Northwest, the Caribbean, and the Marianas ( Figure 1.1 ). However, U.S. shores are also exposed to tsunamis generated far from them. For example, Hawaii has been struck by tsunamis that have been generated by earthquakes off the coasts of South America, Russia, and Alaska (Cox and Mink, 1963). Submarine landslides,
probably triggered by earthquakes, account for much of the known tsunami hazard along the U.S. Atlantic and Gulf coasts, and in southern California (Dunbar and Weaver, 2008). Seismically active faults and the potential for landslides in the Caribbean pose a significant tsunami risk for that region (Dunbar and Weaver, 2008).
Tsunami hazard zones of U.S. coastal communities contain thousands of residents, employees, and tourists, and represent significant economic components of these coastal communities (Wood, 2007; Wood et al., 2007; Wood and Soulard, 2008). The economic and social risks from tsunamis grow with increasing population density along the coasts. To reduce societal risks posed by tsunamis, the nation needs a clear understanding of the nature of the tsunami hazard (e.g., source, inundation area, speed of onset) and the societal characteristics of coastal communities (e.g., the number of people, buildings, infrastructure, and economic activities)
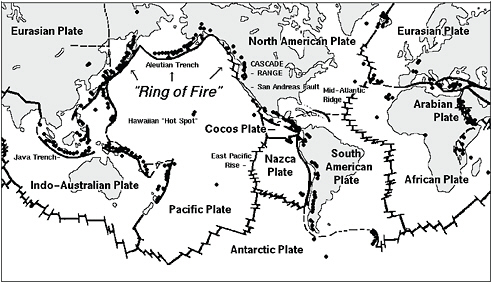
FIGURE 1.1 Global map of active volcanoes and plate tectonics illustrating the “Ring of Fire” and depicting subduction zones; both areas associated with frequent seismic activity. SOURCE: http://vulcan.wr.usgs.gov/Imgs/Gif/PlateTectonics/Maps/map_plate_tectonics_world_bw.gif; USGS .
that make them vulnerable to future tsunamis. With a clear understanding of the tsunami hazards and social vulnerability that comprise tsunami risk, officials and the general public can then prepare for future events and hopefully reduce this risk. 2
When assessing tsunami hazard and developing risk reduction measures, it is important to consider the distance between a coastal community and potential tsunami sources as well as the probability of occurrence. Near-field tsunamis (see Box 1.1 ) pose a greater threat to human life than far-field tsunamis because of the short time between generation and flooding; because the extent of flooding is likely greater; and because the flooded area may be reeling from an earthquake (National Science and Technology Council, 2005). Near-field tsunamis account for most U.S. tsunami deaths outside of Hawaii, but even Hawaii has suffered losses from near-field tsunamis. Because it takes a very large earthquake to impact the far-field, more triggering events have the potential to impact communities that are within an hour or less from the source. For example, an earthquake generated within the Cascadia fault zone along the northern California, Oregon, and Washington coasts will allow only minutes for evacuation of
the coastal communities after the earthquake is felt. In addition, tsunami observations demonstrate an increase in wave height with proximity to the source, resulting in extensive coastal flooding by a near-field tsunami. Consequences of a near-field tsunami are far greater for any given location.
Far-field tsunamis afford hours of advance notice for evacuation and are likely to have smaller wave heights than those in the tsunami’s near field. However, the farther a coastal community from the earthquake source the less likely it is to have felt the earthquake and the more dependent it is on an instrumental detection system to provide warnings. Timely and accurate warnings are required to implement orderly evacuations and to avoid frequent unnecessary evacuations, which can be costly. The National Science and Technology Council (NSTC) report (2005) concludes that “the challenge is to design a tsunami hazard mitigation program to protect life and property from two very different types of tsunami events.”
GOALS AND SCOPE OF THIS REPORT
The 2004 Indian Ocean tsunami, spurred two congressional acts intended to reduce losses of life and property from future tsunamis. The Emergency Supplemental Appropriations Act for Defense, the Global War on Terror, and Tsunami Relief, 2005 (P.L. 109-13), included $24 million to improve tsunami warnings by expanding tsunami detection and earthquake monitoring capabilities. This Act was followed in 2006 by the Tsunami Warning and Education Act (P.L. 109-424), which directs the National Oceanic and Atmospheric Administration (NOAA) to strengthen the nation’s tsunami warning system (TWS), work with federal and state partners toward the mitigation of tsunami hazards, establish and maintain a tsunami research program, and assist with efforts to provide tsunami warnings and tsunami education overseas.
Section 4(j) of the Tsunami Warning and Education Act calls upon the National Academy of Sciences (NAS) “to review the tsunami detection, forecast, and warning program established under this Act to assess further modernization and coverage needs, as well as long-term operational reliability issues.” In response, NOAA asked the NAS to assess options to improve all aspects of the tsunami program. This request is reflected in the first part of the committee’s charge (see Appendix B ) and accordingly focuses on efforts on tsunami detection, forecasting, and warning dissemination.
The NAS, in accepting this charge and in consultation with NOAA, broadened the review’s scope to include an assessment of progress toward additional preparedness efforts to reduce loss of life and property from tsunamis in the United States as part of the National Tsunami Hazard Mitigation Program (NTHMP). The main rationale for this broadened scope was to address Section 5(a) in P.L. 109-424, which called for “a community-based tsunami hazard mitigation program to improve tsunami preparedness of at-risk areas in the United States and its territories.” Such a tsunami hazard mitigation program requires partnership among federal, state, tribal, and local governments. Its strategies include identifying and defining tsunami hazards, making inventories of the people and property in tsunami hazard zones, and providing the public with knowledge and infrastructure for evacuation, particularly for near-field
tsunamis that come ashore in a few minutes. The broadened scope aims at encompassing the range of national tsunami warning and preparedness efforts.
The Range of Options Available for Tsunami Hazard Mitigation
As the scope of the study was broadened to include aspects of tsunami hazard mitigation, the committee recognized the need to define the term “mitigation” and set some boundaries for the study, because the full suite of mitigation options exceeds the purview and capacity of this particular study. The definition of hazard mitigation and the actions it includes differ among various hazard communities. Some members of the academic community consider the full range of hazard mitigation options to include three classes of actions (White and Haas, 1975): (1) modifying the natural causes of hazards, (2) modifying society’s vulnerability (e.g., levees, wind- and seismic-resistant houses), and (3) redistributing the losses that occur (e.g., insurance, emergency response). In contrast, natural hazard practitioners consider the range of human adjustment to natural hazards to fall into two major classes of actions: (1) mitigation of potential losses through interventions in the constructed world in ways that lessen potential losses from nature’s extremes (e.g., land-use management, control and protection works, building codes), and (2) preparedness for, response to, and recovery from specific events and their associated losses (Mileti, 1999).
Focus on Warning and Preparedness
Although land-use planning and adjusting building codes is important in mitigating the impacts of tsunamis, the charge to the committee is focused primarily on the detection, forecast, and warning for near- and far-field tsunamis and issues directly related to the effective implementation of those warnings. To be responsive to its charge, the report focuses on the second class of mitigation actions, which generally includes pre-event planning to develop preparedness plans, appropriate organizational arrangements, training and exercises for issuing event-specific public warnings, an adequate emergency response, and plans for recovery and reconstruction. These types of adjustment are based on the notion that the adequacy of pre-event planning determines the effectiveness of event-specific response. This view also places insurance in the preparedness class.
THE NATION’S TSUNAMI WARNING AND PREPAREDNESS EFFORTS
Only very recently has there been a national interest in tsunami warning and preparedness. Before 2004, most efforts were spearheaded by local, state, or regional initiative operating on very limited budgets. Integrating these existing individual efforts into a national tsunami program has led to a very different type of program than that of a national tsunami warning program designed from the outset. The history of tsunami warning and preparedness efforts can be traced back to two of the six destructive tsunamis that caused causalities on U.S. soil.
These efforts were originally part of the National Geodetic Survey, which developed the two tsunami warning centers (TWCs) in Hawaii and Alaska after the 1946 Aleutian tsunami (Unimak Island, AK) and the 1964 Alaskan tsunami (Prince William Sound, AK) ( Figure 1.2 ). These centers eventually became part of NOAA’s National Weather Service (NWS), but each is located in different NWS regions and is managed independently.
Concern about tsunamis in Washington, Oregon, and California increased in the late 1980s and early 1990s when several new scientific studies revealed their near-field tsunami threat from the Cascadia subduction zone (Atwater, 1987; Heaton and Hartzell, 1987). California was reminded of its potential tsunami threat by an earthquake near Cape Mendocino in 1992, which generated a small tsunami that arrived in Eureka only minutes after the earthquake occurred. These and other developments prompted a more urgent call to produce comprehensive assessments of tsunami risk and preparedness at the state and federal level.
Congress responded to this call in a 1995 Senate Appropriations Committee request to NOAA to develop a plan for reducing tsunami risk to coastal communities. NOAA suggested the formation of a national committee to address tsunami threat, leading to the establishment of the NTHMP that same year. The NTHMP is tasked with coordinating the various federal, state, territorial, and commonwealth tsunami efforts. NOAA’s Tsunami Program was established in 2005 to incorporate all the current tsunami efforts at NOAA (see below). To respond to the committee’s charge (see Appendix B ) and assess progress made toward improved tsunami warning and preparedness, the committee begins its evaluation with an inventory of the elements of the NTHMP and NOAA’s Tsunami Program.
National Tsunami Hazard Mitigation Program
The NTHMP has a Coordinating Committee (steering committee) that works to collaborate on the tsunami mitigation efforts of the NTHMP and three subcommittees: a Mapping and Modeling Subcommittee, a Warning Coordination Subcommittee, and a Mitigation and Education Subcommittee. 3 In addition to coordinating individual efforts, the NTHMP provides guidance to NOAA’s TWSs. Federal partners include NOAA, the U.S. Geological Survey (USGS), and the Federal Emergency Management Agency (FEMA). State partners originally included Hawaii, Alaska, Washington, Oregon, and California, and now include all 29 U.S. coastal states and territories.
The USGS contributes to the seismic network that the TWCs use through operating and maintaining their respective seismic networks and to the tsunami research and risk assessments and conducts an independent seismic analysis of potential tsunamigenic earthquakes at its National Earthquake Information Center (NEIC). The USGS and NOAA both support the Global Seismographic Network (GSN), which provides high-quality seismic data to assist earthquake detection (including tsunamigenic earthquakes). Both agencies also support earthquake and seismic studies to improve tsunami warning efforts and tsunami disaster response and hazards assessments. FEMA is responsible for hazard mitigation and emergency response; as

FIGURE 1.2 Timelines for U.S. tsunami warning centers, programs, tsunami budget, deaths from tsunamis in the United States and its territories, and earthquakes of magnitude 8.0 or larger worldwide since the year 1900. Sources of data for this figure include: NOAA (federal spending); http://www.ngdc.noaa.gov/hazard/tsu_db.shtml (tsunami fatalities); http://earthquake.usgs.gov/earthquakes/eqarchives/ (great earthquake history). SOURCE: Committee member.
part of its mitigation efforts it has issued Guidelines for Design of Structures for Vertical Evacuation from Tsunamis (Federal Emergency Management Agency, 2008). FEMA becomes the lead federal agency in managing the emergency response once a tsunami has caused damage to U.S. coastlines.
The National Science Foundation (NSF) used to be a partner of the NTHMP, but as its involvement decreased the decision was made in 2009 to remove it from the NTHMP. Its primary function is to provide research funding and to partner with other federal agencies in research and development. NSF provides funding for the GSN. NSF has also been actively involved with investments regarding tsunami research infrastructure, such as the Network for Earthquake and Engineering Simulation (NEES), Earthquake Engineering and Research Centers (EERCs), and the Southern California Earthquake Center (SCEC) (Bement, 2005). Because it is not part of the NTHMP and its funding decisions are primarily driven by the demand in the research community, this report does not include an explicit discussion of NSF’s role but rather discusses the role of the broader research community in the nation’s tsunami efforts.
NOAA has been carrying most of the responsibility and obtains most of the funding to provide tsunami warnings, maintain observing networks (including seismic networks not funded by the USGS in Alaska and Hawaii), manage and archive data, and conduct research (further discussed in the next section).
The coastal states, U.S. territories, and commonwealths contribute their own initiatives and resources to the nation’s preparedness and education efforts; these vary in extent and approach from state to state. In particular, states are responsible for providing communities with inundation maps that allow municipalities to produce evacuation maps and guidance, and to educate the public about the hazard and appropriate responses. Local officials in turn are responsible for transmitting tsunami alerts throughout their respective jurisdictions, issuing evacuation orders, managing evacuations, and declaring all-clears.
NOAA’s Tsunami Program
In 2006, the Tsunami Warning and Education Act (P.L. 109-424) charged NOAA with addressing the nation’s priorities in tsunami detection, warning, and mitigation. NOAA’s Tsunami Program assumed the responsibilities to plan and execute NOAA’s tsunami efforts, primarily the program’s budget, strategic plan, and the coordination of activities among its NOAA organizational components and external partners, including the NTHMP. NOAA’s Tsunami Program advocates an end-to-end TWS, which includes detection, warnings and forecasts, message dissemination, outreach and education, and research.
NOAA’s Tsunami Program is supported by five line offices ( Table 1.1 ): NWS; the Office of Marine and Aviation Offices (OMAO); the National Ocean Service (NOS); Oceanic and Atmospheric Research (OAR); and the National Environmental Satellite, Data, and Information Service (NESDIS). The NWS, as the administrator for NOAA’s Tsunami Program, is primarily responsible for helping community leaders and emergency managers in strengthening their local tsunami
TABLE 1.1 Tsunami Program Matrix
warning and preparedness programs through its TsunamiReady program as well as operating the TWCs.
The Pacific Region’s Pacific Tsunami Warning Center (PTWC) and the Alaska Region’s West Coast/Alaska Tsunami Warning Center (WC/ATWC) are administered within the NWS, although the two TWCs report to their respective regional NWS offices. The two TWCs have distinct areas of responsibility as described in Chapter 5 . The NWS also houses the National Data Buoy Center (NDBC), which operates and maintains the Deep-ocean Assessment and Reporting of Tsunamis (DART) buoys. These buoys monitor and alert the TWCs of sea level changes associated with a tsunami. OMAO collaborates by providing detection system maintenance support and conducting coastal surveys. NOS provides state and local coastal emergency managers with hazard-related information such as training and assessment tools, and also operates coastal tide stations and sea level gauges that monitor changes in sea level. OAR comprises a research network involving internal research laboratories, grant programs, and collaborative efforts between NOAA and academic institutions. Pacific Marine Environmentla Laboratory (PMEL), within OAR, focuses on designing optimal tsunami monitoring networks, improving forecast modeling, and improving impact assessment on coastal communities. NESDIS provides access to global environmental data; such as climate, geophysical, and oceanographic data. The National Geophysical Data Center (NGDC), housed within NESDIS, manages a database for historic tsunami events, maps, and DART and tide gauge records. Some negative consequences arising from this distribution of tsunami detection, forecast, warning, and planning functions across different parts of NOAA and across different NTHMP partners is discussed in greater detail in Chapters 3 and 5 .
ASSESSING THE NATION’S EFFORTS
Because tsunami warning and preparedness efforts are distributed across federal and state agencies and were historically conducted without a federal coordination mechanism, the committee faced a number of challenges in assessing progress in the nation’s ability to warn and prepare for the threat of tsunamis. The first challenge results from the need to assess many individual activities. Secondly, it is difficult to extrapolate from these individual activities to assess whether all the distributed efforts can function coherently during a tsunami to warn and evacuate people in a timely fashion. To help address these challenges, the committee began its analysis by sketching the required functions and elements of an idealized integrated warning and preparedness effort based on available research findings in the hazards and high-reliability organizations (HRO) literature (see section below). The committee then sought to compare its vision of an idealized system with the evolving status quo.
An ideal integrated TWS comprises multiple technologies, systems, individuals, and organizations. A comprehensive view of the elements therefore includes technical, organizational, social, and human components. The ideal system incorporates risk assessment, public education, tsunami detection, warning management, and public response ( Figure 1.3 ).
Protecting and warning the public begins with an understanding of the tsunami risk envi-
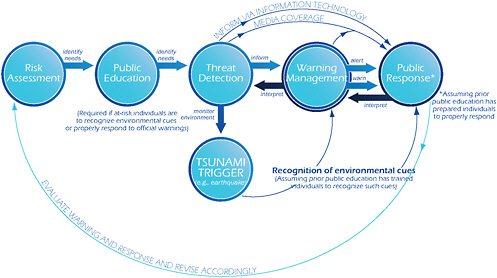
FIGURE 1.3 Components of an integrated warning system: Risk assessment includes all assessments required to effectively plan evacuations (including tsunami source determination, inundation modeling, and evacuation mapping) and prepare the communities to evacuate in the event a warning is issued or received. Risk assessments identify needs for public education. Public education aims to ensure maximum preparedness and a public that knows what to do when it receives a warning or feels the ground shaking in the case of near-field tsunamis. Threat detection comprises the continuous monitoring of the natural and technological environments that could create an emergency; it informs the warning management and public response component using threshold criteria and communication technology. Warning management interfaces the threat detection component with the public response component and is responsible for tsunami alerts, warnings, and evacuations; in consultation with the threat detection component it will alert and warn the public. Public response is the ultimate outcome of the integrated warning system, and it integrates public education, threat detection, natural cues from tsunami triggers, and warning management. SOURCE: Committee member; design by Jennifer Matthews, University of California, San Diego.
ronment. This must be done before a tsunami is generated in order to design the threat detection system, the education and awareness campaigns, and the evacuation and response plans. To understand the risk environment, both hazards (the physical characteristics of tsunamis and the inundation area) and vulnerabilities (the people and properties in harm’s way) need to be characterized (National Research Council, 2006). Pre-event public education is required to enable at-risk populations to correctly interpret: (1) natural cues from the environment (e.g., ground shaking from the earthquake) or (2) warnings from a technical detection system as a signal to evacuate to higher ground in a timely fashion. The threat detection component monitors the environment for threshold events using cues from natural and technical systems (Mileti, 1999; Mileti and Sorenson, 1990).
Once a significant tsunami is detected, the warning process needs to be managed. Tsunami information needs to be analyzed and decisions have to be made about the extent of the warning. Managers and decision makers issue warnings directly to the public. Ideally, officials managing the response also maintain situational awareness and information flow between the technical detection system and the public to update warnings and messages with the required protective actions to be taken. Because of the dominance of real-time communications, the Internet, and social networking, both the general public and media will increasingly access tsunami information directly from real-time information sources (e.g., the TWCs, seismometers, and water-level gauges) before local officials are able to respond. The public’s real-time access to different information sources, such as social media and networking systems, underscores the importance of public education to prepare both the public and the press for proper interpretation of information and response to detected hazards. An effective warning system monitors the public’s response and reactions in order to improve its processes for effective, understandable, actionable, reliable, and accurate warnings of impending danger. In the following chapters, the report covers the system components and compares the idealized system with current and/or planned efforts.
An integrated TWS has an impact on large populations and on a wide range of resources and, in the event of failure, has the potential to cause enormous economic, social, organizational, technological, and political losses. Although often seen as mainly comprising technical and technological elements, a warning system must, out of necessity, include the human dimension, such as people’s behavior, policies, procedures, and organizations. However, it is the human dimension that poses a significant challenge:
This involves the setting and running of national services (people), and the implementation of complex emergency-preparedness and awareness plans at the national and local levels to immediately inform every person of the threat. In the building of any early warning system, this is the difficult part. (Intergovernmental Oceanographic Commission, International Strategy for Disaster Reduction, and World Meteorological Organization, 2005).
CHALLENGES TO REDUCING THE NATION’S VULNERABILITY TO TSUNAMIS
Reducing the vulnerability of coastal settlements and infrastructure to tsunami risk poses some unique challenges. Although tsunamis can be devastating, as was seen during the 2004 Indian Ocean event, catastrophic tsunamis are relatively infrequent. This infrequency makes it more challenging to sustain the capacity to educate, warn, and prepare for this particular hazard. As discussed above, the history of tsunami warning and mitigation efforts in the United States shows that significant new funding is often made available only after a tsunami has devastated a coastal community and caused casualties. High funding levels and commitment to tsunami mitigation dissipate over time, leading to difficulties in maintaining efforts, knowledge, and lessons learned over time. Another challenge is the need to relay warnings from the fed-
eral government to state and local officials in just minutes (in the case of a near-field tsunami) or hours (in the case of a far-field tsunami). Sustaining the organizational preparedness and coordination across many jurisdictional boundaries presents a daunting challenge.
The committee recognizes that the nation’s tsunami detection, warning, and preparedness efforts originated in many diverse efforts distributed across several coastal states, and that attempts to integrate these distributed components into a coherent program have only recently begun. In particular, because tsunamis are rapid onset events, there is very little margin for error in the system before failure becomes catastrophic. An organization that operates in a low probability, high-risk environment, allowing few errors, is called an HRO (Roberts, 1990). HROs manifest a number of common properties: flexible and adaptable organizational structures, continually reinforced organizational learning, decision making that is both flexible and mobile, a strongly reinforced organizational culture, constant and effective communication, and trust among members of the system, particularly across organizations (Grabowski and Roberts, 1999; Grabowski et al., 2007). Because the committee identified the need for high-reliability operations in TWSs, the committee draws from the research literature on HROs (Roberts, 1990) and resilient systems (Hollnagel et al., 2008) to highlight particular characteristics that reduce the risks of failure in an idealized end-to-end warning system:
Situational Awareness in an Emergency: Because tsunamis are events that allow only minutes to hours for evacuation, a keen sense of situational awareness and the ability to respond quickly and effectively is required (Weick, 1990, 1993, 2003). HROs require decision making that is adaptable to change and surprise, and that is able to continually reassess needs across distributed organizations (Weick, 1993, 1998; Weick et al., 1999). Such is the case with the nation’s tsunami warning and preparedness efforts, where the TWCs, the state and local offices, and emergency managers and the affected public are geographically dispersed and often lack face-to-face contact. The dispersed and decentralized nature of the end-to-end tsunami warning and preparedness efforts make it a significant challenge to maintain awareness of the evolving situation during a crisis.
Learning and Training: To maintain situational awareness under changing conditions requires training. Therefore, an effective TWS requires that watchstanders, emergency managers, regulators, the public, and the media learn together, and engage in learning that enhances sense-making and developing alertness to small incidents that may cascade into much larger disasters (Weick, 1993; Farber et al., 2006). Because of the low frequency of tsunamis (e.g., California is issued an alert bulletin on average once every three years; Dengler, 2009), a TWS has few opportunities to learn from an event and therefore needs to learn from exercising the system through drills. Trial and error can be disastrous not only because disasters are rare, but also because in the absence of a major catastrophe to focus attention in the system, lessons learned from previous events may be forgotten or misapplied (March et al., 1991; Levitt and March, 1988; De Holan and Phillips, 2004). Learning in a high-reliability organization needs to be systematic, continually reinforced, measured, and made part of the system’s core values.
Fluid Organizational Structures: HRO structures are often adaptable and fluid, allowing the system to expand or contract in response to its environment (Roberts, 1990). TWSs with flexible organizational structures would be able to expand and contract resources in response to shifts and changes in environmental demands, disasters, or periods of slack resources. In the event of a tsunami, TWS managers need to grow effective, functioning response organizations in a period of less than 24 hours, and then adjust the organizational structures to the needs of the response (Tuler, 1988; Bigley and Roberts, 2001). The ability to provide varied organizational structures in response to environmental demands may be critical to the success of TWS organizations, similar to the way fire and emergency organizations expand and contract in response to fire demands (Grabowski and Roberts, 1999). Distributed information technology that connects the system responders can provide the technological glue that ties HRO members together, and fluid organizational structures can allow the organization to grow, expand, contract, and respond to changes in a dynamic, high tempo environment (Bigley and Roberts, 2001). Similar requirements for members and organizations in TWSs can be envisioned as tsunami conditions unfold.
Strong Organizational Culture: Schein (1992, 1996) defines “culture” as a set of basic tacit assumptions, that a group of people share, about how the world is and ought to be; it determines their perceptions, thoughts, feelings, and to some degree, their overt behavior. In many organizations, shared assumptions typically form around the functional units of the organization and are often based on members’ similar educational backgrounds or experiences (Grabowski and Roberts, 1996, 1997). HROs are characterized by strong cultures and norms that reinforce the organization’s mission and goals and that focus attention on procedures, policies, and reward structures consistent with the organization’s mission and safety (LaPorte and Consolini, 1991). HROs have cultures attentive to errors; cultures where closely held ideas about the organization, its mission, and member roles in reliability enhancement are articulated; cultures that encourage learning; and cultures where safe areas—for decision making, communication, and the like—are created as buffers (Weick, 1993). Constructs such as oversight and checks and balances reinforce the strong cultural norms of the HRO. Melding the varied cultures that integrate the system into a cohesive whole can be extremely difficult in distributed systems that are connected by linkages that can dissolve and wane as requirements, organizational structures, and political will change (Weick, 1987; Weick and Roberts, 1993; Grabowski and Roberts, 1999).
Managing decision making across organizations that report to different management structures is a challenge for highly dispersed efforts; this is certainly the case with U.S. tsunami detection, warning, and preparedness efforts. A particular challenge is that the federal government has responsibility to forecast and warn about potential hazards, yet local governments order evacuations. Failure to consider distributed decision making within groups and across multiple units can lead to lack of readiness for the next large-scale catastrophe; e.g., Hurricane Katrina (Roberts et al., 2005; Farber et al., 2006). Building good communication and trust aid in
effective decision making and can increase the likelihood of success in geographically dis-tributed organizations. Trust can be built by common training; opportunities for scientific and operational exchange; and workshops, conferences, exercises, and simulations that build community and coherence across distributed organizations.
TYING IT ALL TOGETHER: REPORT ROADMAP
In the following chapters, the committee assesses progress in the nation’s distributed tsunami preparedness, detection, and warning efforts and compares it to its vision of an idealized warning system ( Figure 1.3 ). Chapter 2 evaluates progress in hazard and vulnerability assessments and identifies potential improvements that could guide the nation’s tsunami risk-assessment efforts. Chapter 3 discusses education and outreach efforts and evaluates pre-event community and organizational preparedness and the coordination between the various entities at the local, state, and federal levels. Chapter 4 examines the technical hazard detection system, including the seismic and sea level sensor networks. Chapter 5 examines the TWCs’ operations and how technology and human capital are used to provide their functions. Appendices present supporting data on tsunami sources, hazard and evacuation maps, educational efforts, seismological methods, and several case-study tsunamis.
This page intentionally left blank.
Many coastal areas of the United States are at risk for tsunamis. After the catastrophic 2004 tsunami in the Indian Ocean, legislation was passed to expand U.S. tsunami warning capabilities. Since then, the nation has made progress in several related areas on both the federal and state levels. At the federal level, NOAA has improved the ability to detect and forecast tsunamis by expanding the sensor network. Other federal and state activities to increase tsunami safety include: improvements to tsunami hazard and evacuation maps for many coastal communities; vulnerability assessments of some coastal populations in several states; and new efforts to increase public awareness of the hazard and how to respond.
Tsunami Warning and Preparedness explores the advances made in tsunami detection and preparedness, and identifies the challenges that still remain. The book describes areas of research and development that would improve tsunami education, preparation, and detection, especially with tsunamis that arrive less than an hour after the triggering event. It asserts that seamless coordination between the two Tsunami Warning Centers and clear communications to local officials and the public could create a timely and effective response to coastal communities facing a pending tsuanami.
According to Tsunami Warning and Preparedness , minimizing future losses to the nation from tsunamis requires persistent progress across the broad spectrum of efforts including: risk assessment, public education, government coordination, detection and forecasting, and warning-center operations. The book also suggests designing effective interagency exercises, using professional emergency-management standards to prepare communities, and prioritizing funding based on tsunami risk.
READ FREE ONLINE
Welcome to OpenBook!
You're looking at OpenBook, NAP.edu's online reading room since 1999. Based on feedback from you, our users, we've made some improvements that make it easier than ever to read thousands of publications on our website.
Do you want to take a quick tour of the OpenBook's features?
Show this book's table of contents , where you can jump to any chapter by name.
...or use these buttons to go back to the previous chapter or skip to the next one.
Jump up to the previous page or down to the next one. Also, you can type in a page number and press Enter to go directly to that page in the book.
Switch between the Original Pages , where you can read the report as it appeared in print, and Text Pages for the web version, where you can highlight and search the text.
To search the entire text of this book, type in your search term here and press Enter .
Share a link to this book page on your preferred social network or via email.
View our suggested citation for this chapter.
Ready to take your reading offline? Click here to buy this book in print or download it as a free PDF, if available.
Get Email Updates
Do you enjoy reading reports from the Academies online for free ? Sign up for email notifications and we'll let you know about new publications in your areas of interest when they're released.
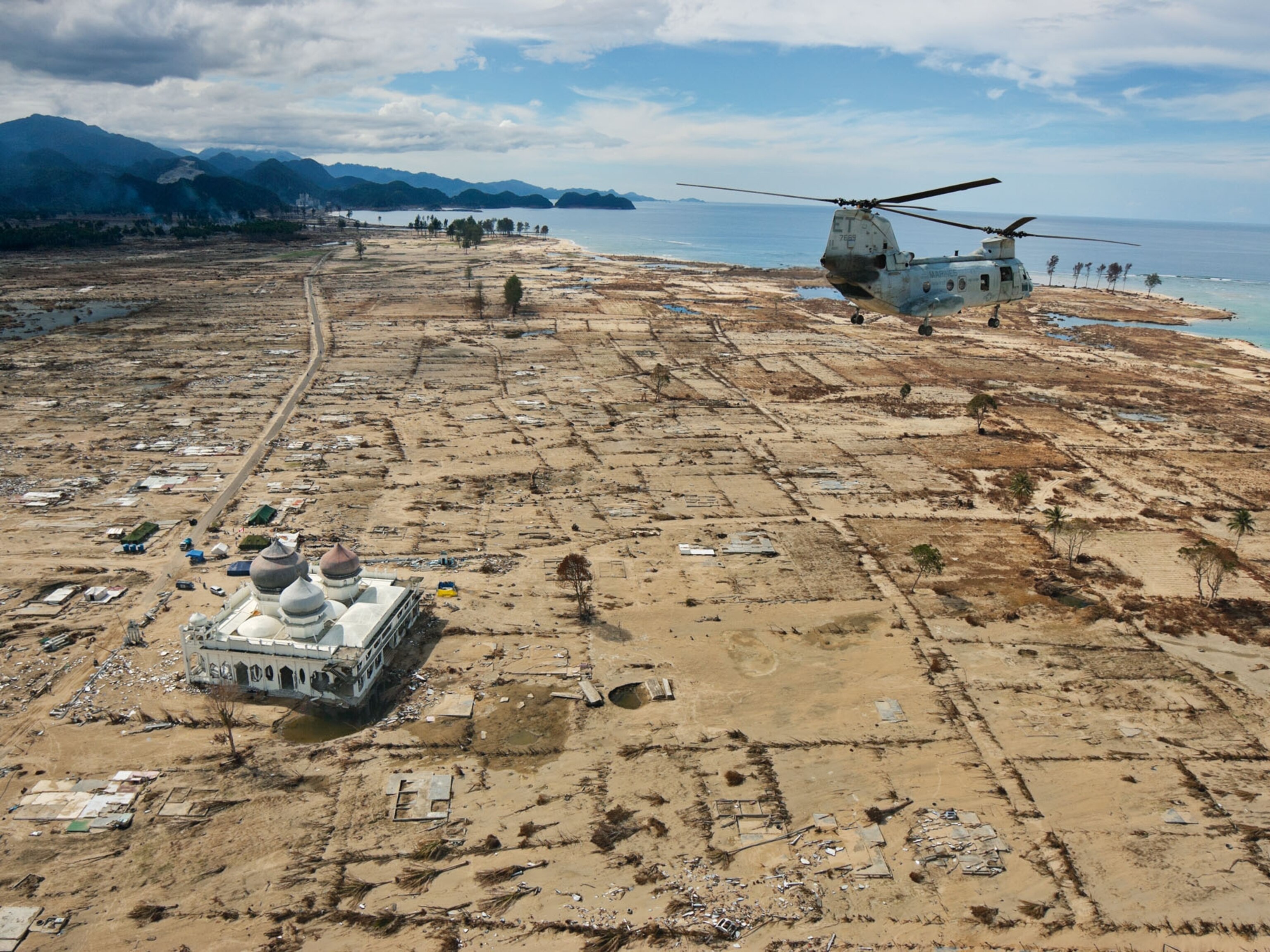
A U.S. Marine helicopter loaded with food flies over Lampuuk in northern Sumatra after a tsunami killed most of the village's 7,000 residents–and nearly 230,000 people on coastlines around the Indian Ocean.
Tsunami safety tips
These massive waves involve intense force and overwhelming volumes of water. Here's how to prepare.
With the ability to approach shores at 30 miles an hour and rise more than 100 feet high, tsunamis pose a deadly threat to coastal populations. The 2004 Indian Ocean tsunami and the 2011 Tohoku tsunami are two vivid and tragic examples of these waves' destructive power.
The most important step in staying safe during a tsunami—or any natural disaster, for that matter—is to know how vulnerable your area is in the first place. Many local governments map hazard areas and evacuation routes for communities at risk, while the U.S. National Weather Service offers a nationwide map with links to resources .
"Know what your risks are," says Kevin J. Richards, a natural hazards officer for the Hawaii Emergency Management Agency. "What's likely going to impact your home or your area?"
How to prepare
● Know the warning signs of a tsunami: rapidly rising or falling coastal waters, a loud roar from the ocean, or rumblings of an earthquake . “If people along the Indian Ocean coastline on December 26, 2004, were aware of and heeded these natural warnings, fewer people would have died,” says Rocky Lopes, administrator of the National Tsunami Hazard Mitigation Program . He adds, “A strong myth is that tsunamis always cause the ocean to recede before [the powerful] waves flood in. In some areas, particularly on islands, water recession may not happen.”
● Familiarize yourself with your government's warning system and subscribe to alerts. In the U.S., NOAA Weather Radio is a good resource.
● Map your evacuation route—not just for your home, but work, school or caregivers , or any place else where your family tends to be. Know how to get to safety on foot—roads may not always be feasible.
● Have your evacuation plan ready and rehearsed now. That way, Richards says, "You don't have to think about it. You just go and do it."
● Keep an emergency kit or "go bag" handy at home or in your car. The American Red Cross offers tips for stocking it here , including food, water, and a cell phone with chargers.
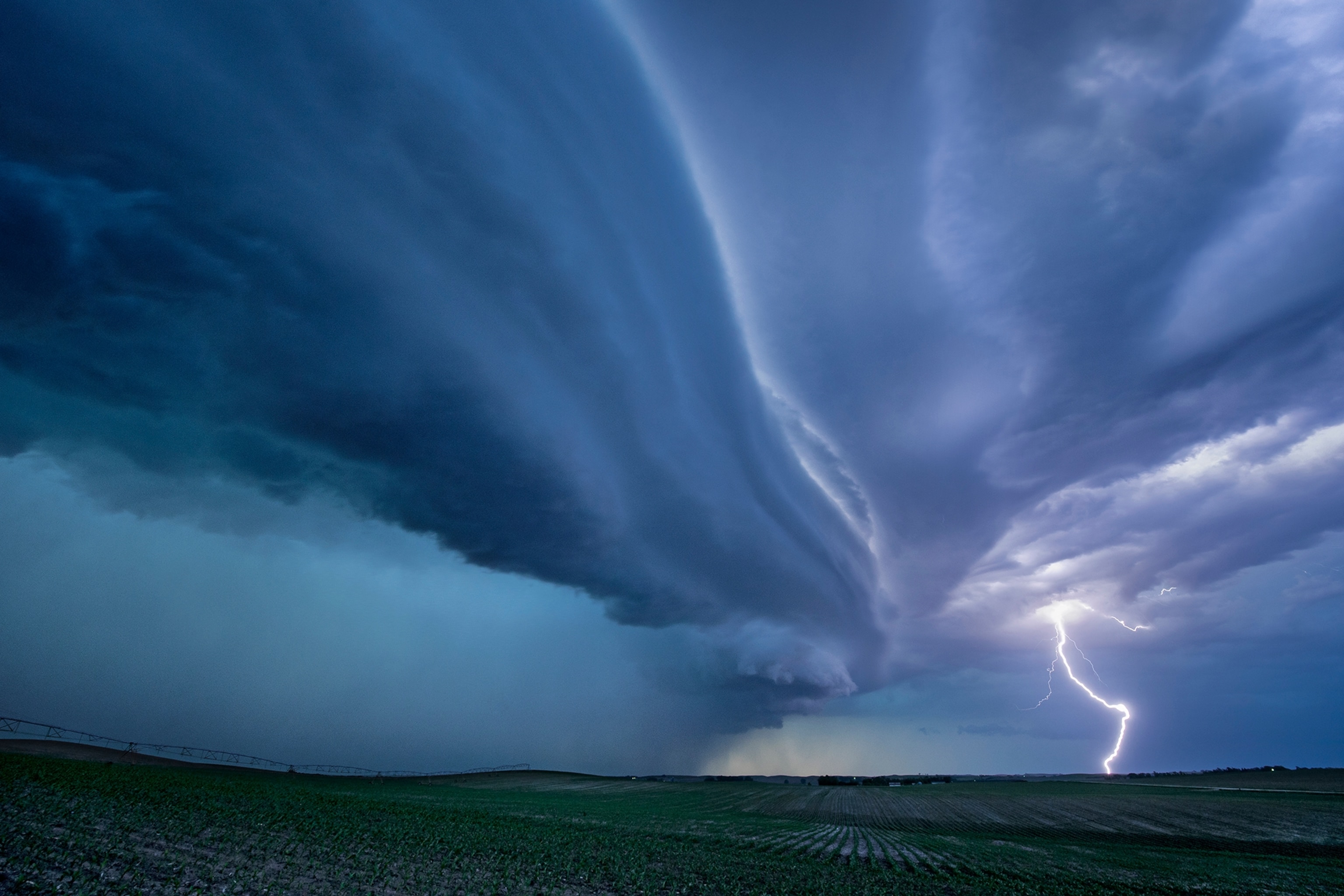
● Coordinate with loved ones on reuniting. "What happens when the family has to respond from separate areas?" Richards recommends asking. "How do you eventually come back together?"
During a tsunami
● If you're in a tsunami area and there is an earthquake, first drop to the floor, cover your head and neck, and hold on to something stable. If you're in a low-lying area, move inland as soon as possible.
● Listen for official warnings, but also listen and watch for natural signs. "Listen to the authorities, but do not wait for tsunami warnings and evacuation orders," counsels the U.S. site Ready.gov .
● People often mistake the distance they have to go to be safe. "Many people think they've got to go miles," Richards says, "when it might be just right behind Johnny's house." If you're a visitor staying in a tall concrete hotel, he adds, going above the fourth floor likely will be safer than evacuating.
● Stay put in a safe area until an official all-clear is given. The first wave of a tsunami may not be the last or the strongest and the danger can last for hours or even days, according to the National Weather Service .
After a tsunami
● Stay clear of damaged or flooded areas and downed power lines.
● Listen for further alerts and instructions about evacuation zones and shelters.
● Use texts and social media to communicate with loved ones, as phone systems are likely to be down or busy. The American Red Cross has a registry where you can list yourself as safe and well .
Follow these common-sense guidelines from the U.S. Centers for Disease Control and Prevention on food and water safety, including using bottled, boiled, or treated water and throwing away perishable foods left unrefrigerated for more than four hours.
For Hungry Minds
Related topics.
- EARTHQUAKES
You May Also Like
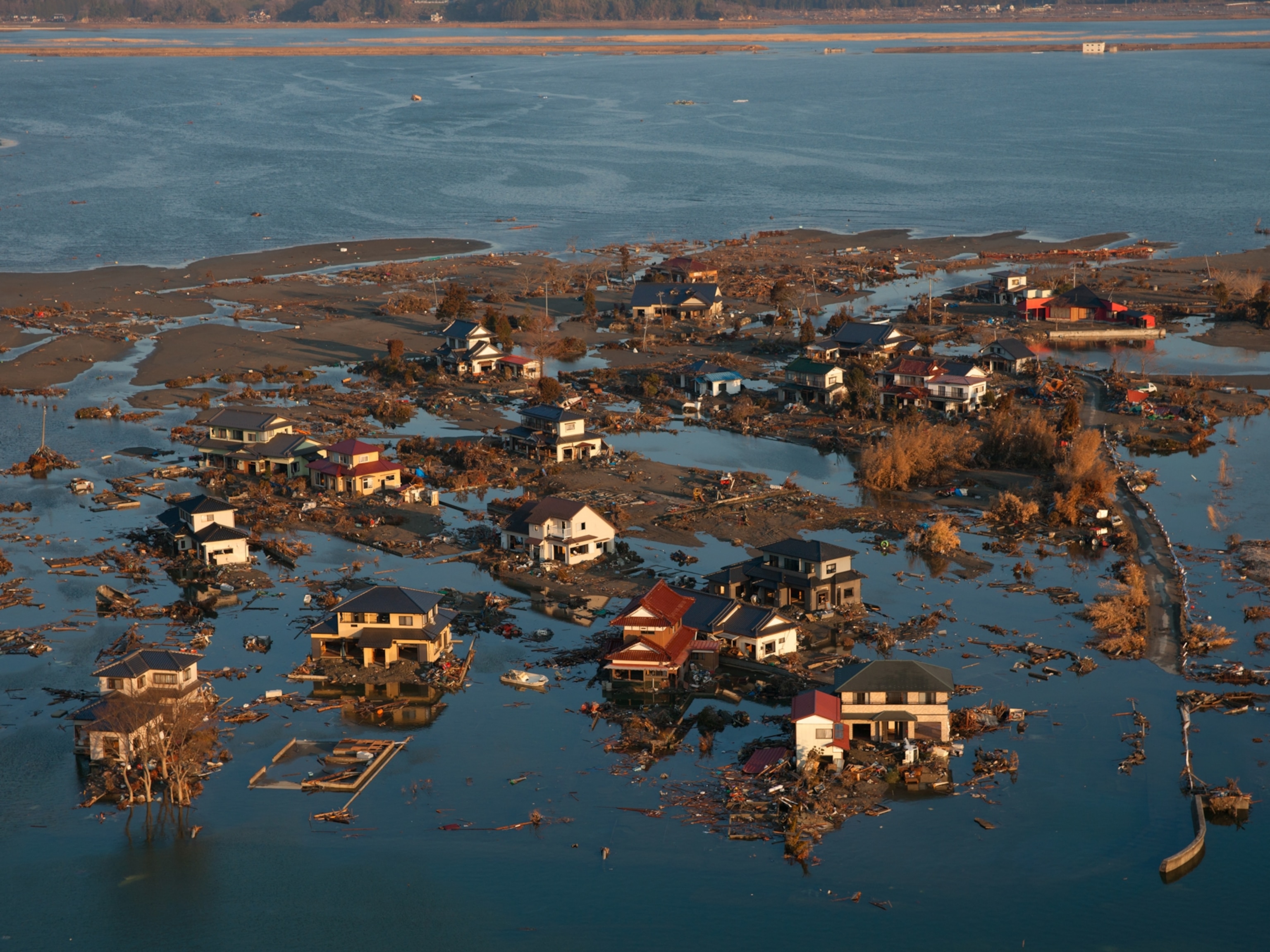
Japan's 2011 megaquake left a scar at the bottom of the sea. Scientists finally explored it.
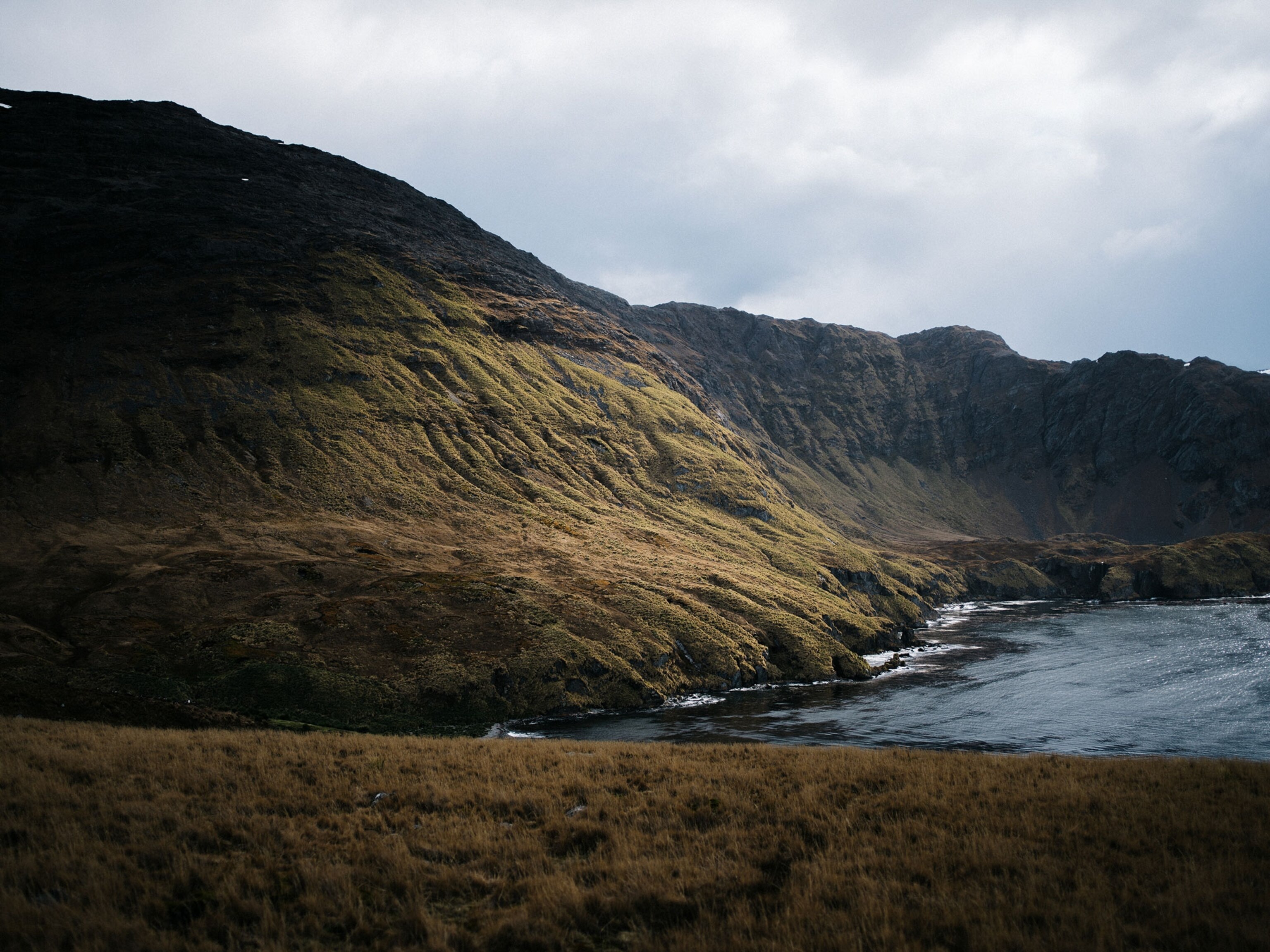
Source of mysterious global tsunami found near Antarctica
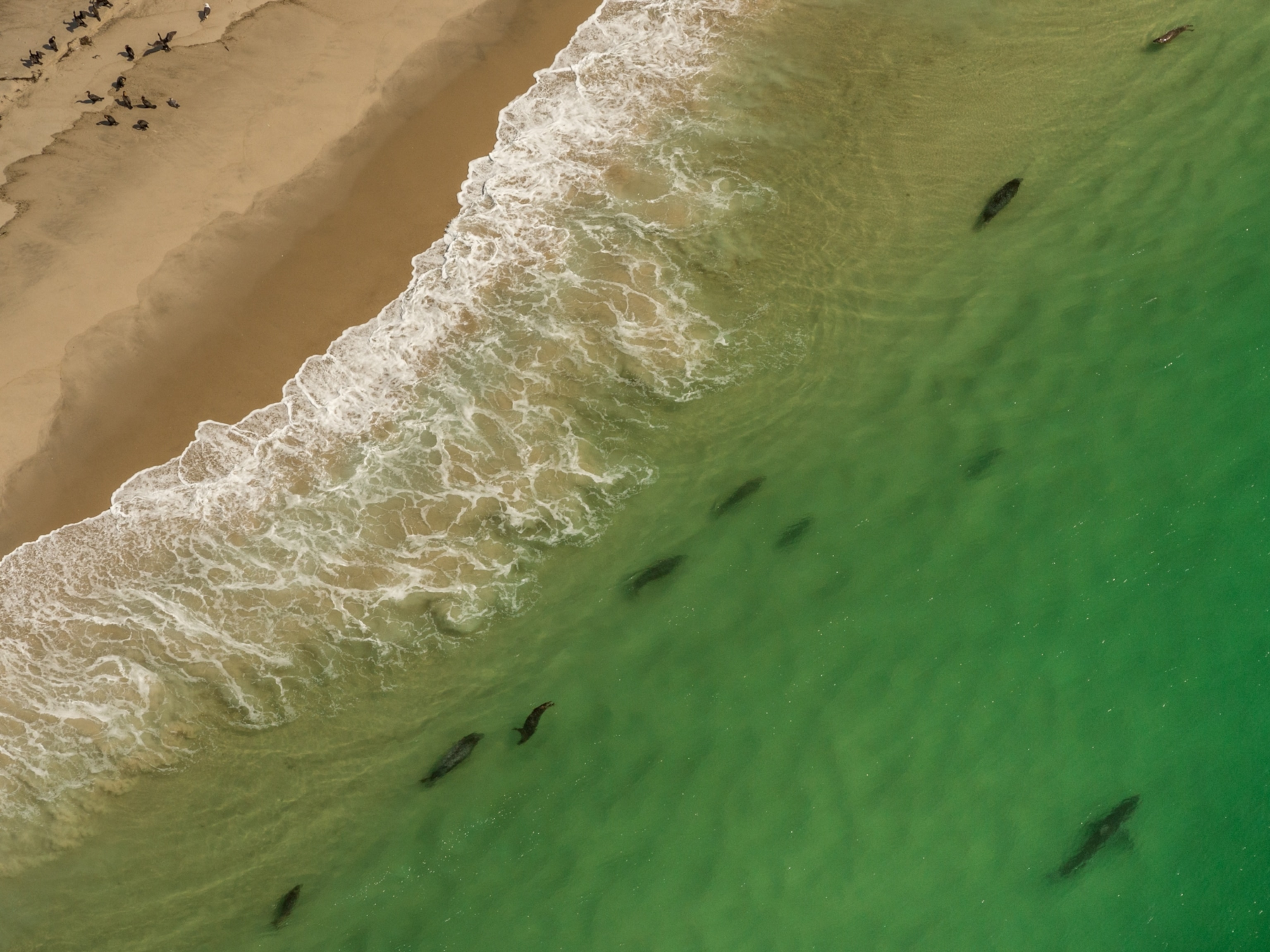
Cape Cod may have the highest density of great white sharks in the world
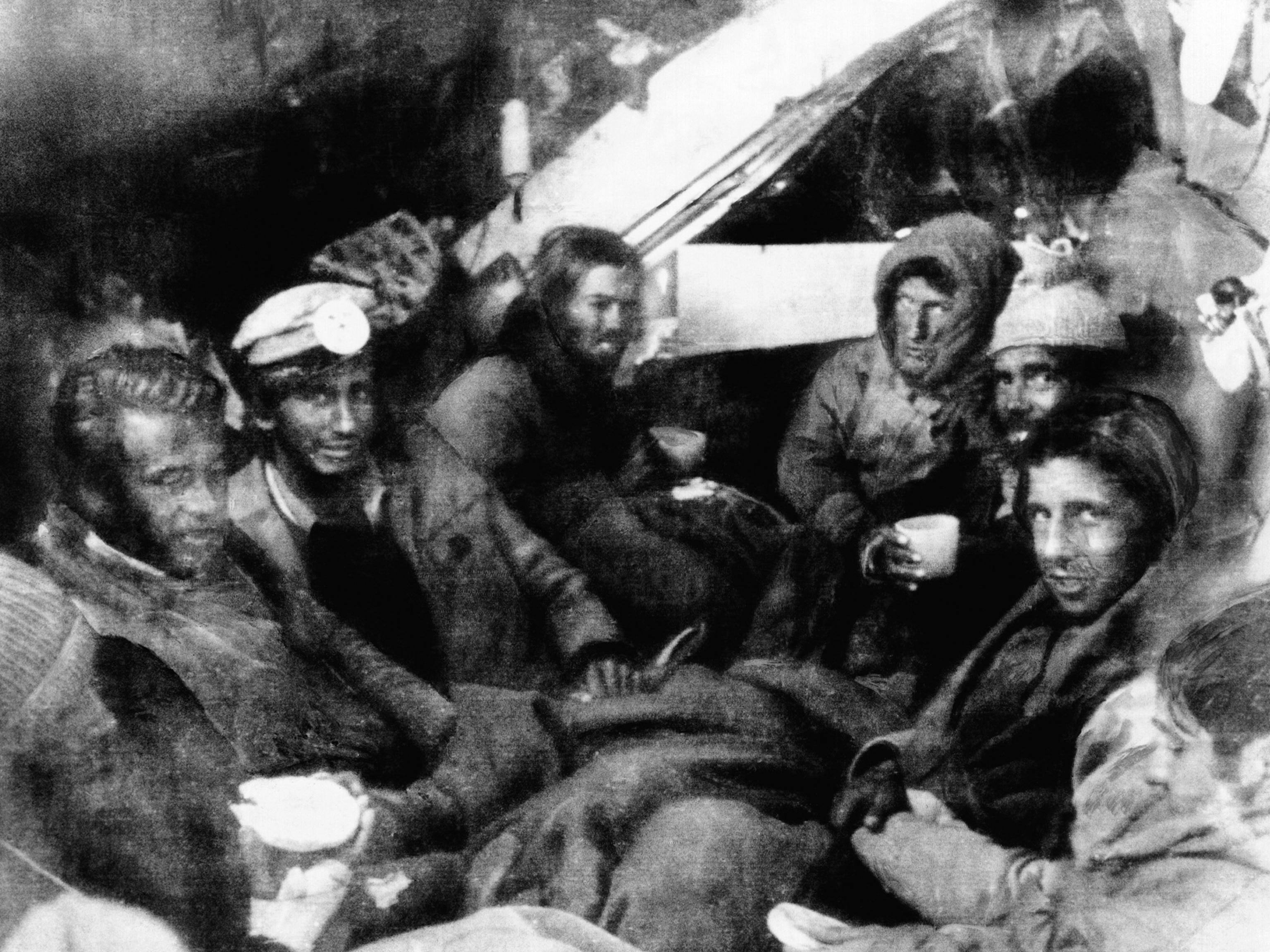
‘Society of the Snow’ is based on a true story. Here’s what really happened.
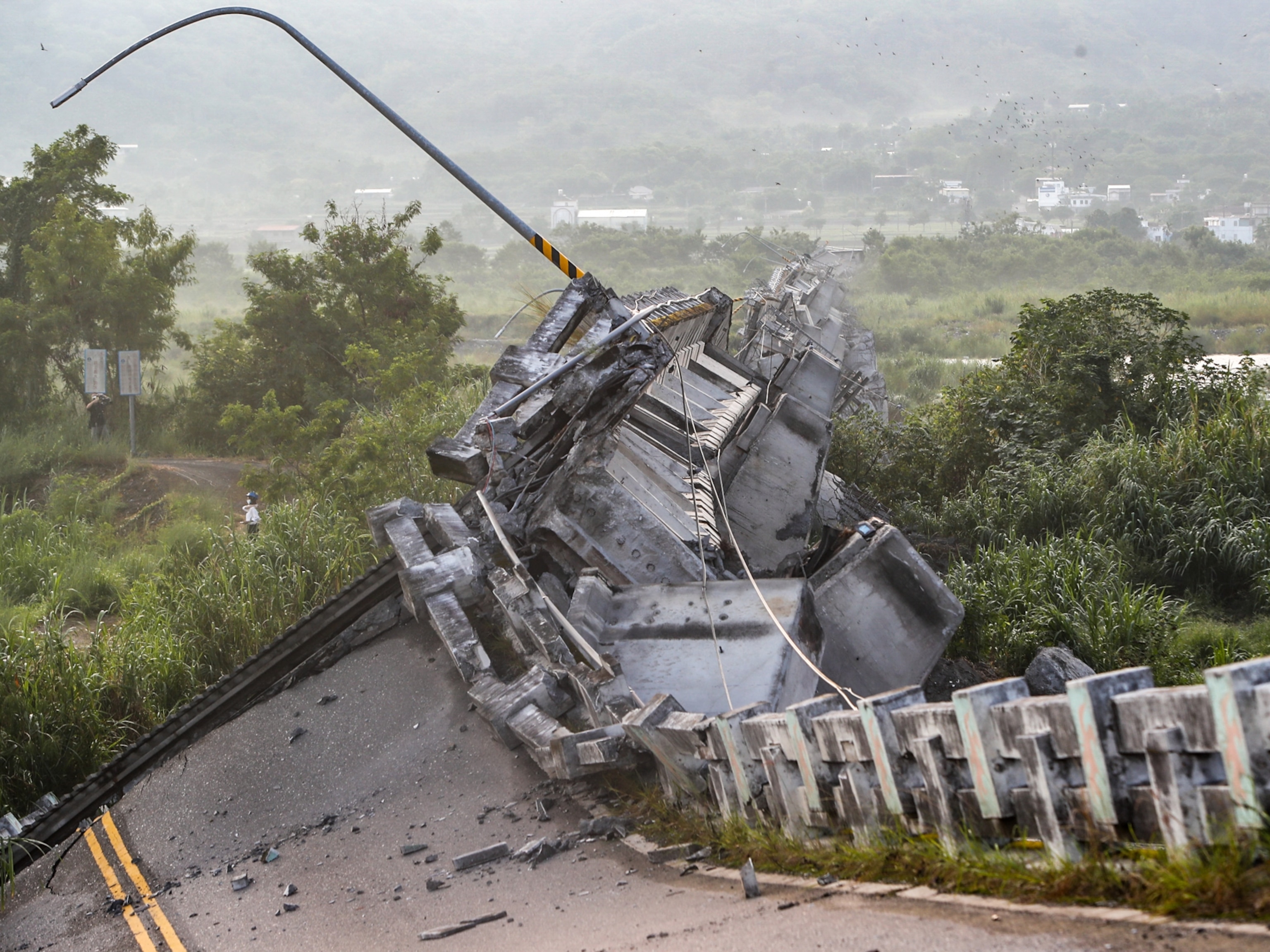
What causes earthquakes?
- Environment
- Perpetual Planet
History & Culture
- History & Culture
- History Magazine
- Mind, Body, Wonder
- Terms of Use
- Privacy Policy
- Your US State Privacy Rights
- Children's Online Privacy Policy
- Interest-Based Ads
- About Nielsen Measurement
- Do Not Sell or Share My Personal Information
- Nat Geo Home
- Attend a Live Event
- Book a Trip
- Inspire Your Kids
- Shop Nat Geo
- Visit the D.C. Museum
- Learn About Our Impact
- Support Our Mission
- Advertise With Us
- Customer Service
- Renew Subscription
- Manage Your Subscription
- Work at Nat Geo
- Sign Up for Our Newsletters
- Contribute to Protect the Planet
Copyright © 1996-2015 National Geographic Society Copyright © 2015-2024 National Geographic Partners, LLC. All rights reserved
Physics of Tsunamis
- © 2016
- Latest edition
- Boris W. Levin 0 ,
- Mikhail Nosov 1
Inst. Marine Geology & Geophysics, Russian Academy of Sciences, Yuzhno-Sakhalinsk, Russia
You can also search for this author in PubMed Google Scholar
Faculty of Physics, M.V.Lomonosov Moscow State University, Moskva, Russia
- Presents updated knowledge on physics of tsunamis and recent events plus advances in tsunami models
- Reflects significant progress in tsunami research, monitoring and mitigation over the last decade
- Presents a profound review of the methods of tsunami monitoring
- Includes supplementary material: sn.pub/extras
12k Accesses
59 Citations
3 Altmetric
This is a preview of subscription content, log in via an institution to check access.
Access this book
- Available as EPUB and PDF
- Read on any device
- Instant download
- Own it forever
- Compact, lightweight edition
- Dispatched in 3 to 5 business days
- Free shipping worldwide - see info
- Durable hardcover edition
Tax calculation will be finalised at checkout
Other ways to access
Licence this eBook for your library
Institutional subscriptions
About this book
This second edition reflects significant progress in tsunami research, monitoring and mitigation within the last decade. Primarily meant to summarize the state-of-the-art knowledge on physics of tsunamis, it describes up-to-date models of tsunamis generated by a submarine earthquake, landslide, volcanic eruption, meteorite impact, and moving atmospheric pressure inhomogeneities. Models of tsunami propagation and run-up are also discussed. The book investigates methods of tsunami monitoring including coastal mareographs, deep-water pressure gauges, GPS buoys, satellite altimetry, the study of ionospheric disturbances caused by tsunamis and the study of paleotsunamis. Non-linear phenomena in tsunami source and manifestations of water compressibility are discussed in the context of their contribution to the wave amplitude and energy. The practical method of calculating the initial elevation on a water surface at a seismotectonic tsunami source is expounded. Potential and eddy traces ofa tsunamigenic earthquake in the ocean are examined in terms of their applicability to tsunami warning.
The first edition of this book was published in 2009. Since then, a few catastrophic events occurred, including the 2011 Tohoku tsunami, which is well known all over the world.
Similar content being viewed by others
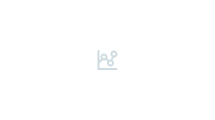
Introduction to “Global Tsunami Science: Past and Future, Volume III”
Tsunamis and tsunami warning: recent progress and future prospects.
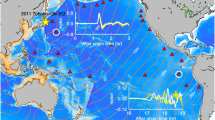
Progress and application of the synthesis of trans-oceanic tsunamis
- Tsunami Warning
- Historical Tsunami Database
- Tsunami Generation
- Co-Seismic Deformation
- Initial Elevation
- Long-Wave Theory
- Potential Theory
- Volcanic Eruption
- Meteotsunami
- Cosmogenic Tsunami
- Tsunami Propagation
- Tsunami Run-up
- Nonlinearity
- Numerical Simulation
- Measurements of Sea Level
- Tsunami Deposits
- Satellite Altimetry
- Ionospheric Disturbances
Table of contents (7 chapters)
Front matter, general information on tsunami waves, seaquakes, and other catastrophic phenomena in the ocean.
- Boris W. Levin, Mikhail A. Nosov
Source of a Tsunami of Seismotectonic Origin
Hydrodynamic processes at the source of a tsunami of seismotectonic origin: incompressible ocean, role of the compressibility of water and of nonlinear effects in the formation of tsunami waves, the physics of tsunami formation by sources of nonseismic origin, propagation of a tsunami in the ocean and its interaction with the coast, methods of tsunami wave registration, authors and affiliations, inst. marine geology & geophysics, russian academy of sciences, yuzhno-sakhalinsk, russia.
Boris W. Levin
Mikhail Nosov
About the authors
Bibliographic information.
Book Title : Physics of Tsunamis
Authors : Boris W. Levin, Mikhail Nosov
DOI : https://doi.org/10.1007/978-3-319-24037-4
Publisher : Springer Cham
eBook Packages : Earth and Environmental Science , Earth and Environmental Science (R0)
Copyright Information : Springer International Publishing Switzerland 2016
Hardcover ISBN : 978-3-319-24035-0 Published: 06 November 2015
Softcover ISBN : 978-3-319-33106-5 Published: 23 August 2016
eBook ISBN : 978-3-319-24037-4 Published: 22 October 2015
Edition Number : 2
Number of Pages : XIII, 388
Number of Illustrations : 53 b/w illustrations, 114 illustrations in colour
Topics : Natural Hazards , Oceanography , Geophysics and Environmental Physics
- Publish with us
Policies and ethics
- Find a journal
- Track your research
- CBSE Class 10th
- CBSE Class 12th
- UP Board 10th
- UP Board 12th
- Bihar Board 10th
- Bihar Board 12th
- Top Schools in India
- Top Schools in Delhi
- Top Schools in Mumbai
- Top Schools in Chennai
- Top Schools in Hyderabad
- Top Schools in Kolkata
- Top Schools in Pune
- Top Schools in Bangalore
Products & Resources
- JEE Main Knockout April
- Free Sample Papers
- Free Ebooks
- NCERT Notes
- NCERT Syllabus
- NCERT Books
- RD Sharma Solutions
- Navodaya Vidyalaya Admission 2024-25
- NCERT Solutions
- NCERT Solutions for Class 12
- NCERT Solutions for Class 11
- NCERT solutions for Class 10
- NCERT solutions for Class 9
- NCERT solutions for Class 8
- NCERT Solutions for Class 7
- JEE Main 2024
- MHT CET 2024
- JEE Advanced 2024
- BITSAT 2024
- View All Engineering Exams
- Colleges Accepting B.Tech Applications
- Top Engineering Colleges in India
- Engineering Colleges in India
- Engineering Colleges in Tamil Nadu
- Engineering Colleges Accepting JEE Main
- Top IITs in India
- Top NITs in India
- Top IIITs in India
- JEE Main College Predictor
- JEE Main Rank Predictor
- MHT CET College Predictor
- AP EAMCET College Predictor
- GATE College Predictor
- KCET College Predictor
- JEE Advanced College Predictor
- View All College Predictors
- JEE Main Question Paper
- JEE Main Cutoff
- JEE Main Advanced Admit Card
- JEE Advanced Admit Card 2024
- Download E-Books and Sample Papers
- Compare Colleges
- B.Tech College Applications
- KCET Result
- MAH MBA CET Exam
- View All Management Exams
Colleges & Courses
- MBA College Admissions
- MBA Colleges in India
- Top IIMs Colleges in India
- Top Online MBA Colleges in India
- MBA Colleges Accepting XAT Score
- BBA Colleges in India
- XAT College Predictor 2024
- SNAP College Predictor
- NMAT College Predictor
- MAT College Predictor 2024
- CMAT College Predictor 2024
- CAT Percentile Predictor 2023
- CAT 2023 College Predictor
- CMAT 2024 Admit Card
- TS ICET 2024 Hall Ticket
- CMAT Result 2024
- MAH MBA CET Cutoff 2024
- Download Helpful Ebooks
- List of Popular Branches
- QnA - Get answers to your doubts
- IIM Fees Structure
- AIIMS Nursing
- Top Medical Colleges in India
- Top Medical Colleges in India accepting NEET Score
- Medical Colleges accepting NEET
- List of Medical Colleges in India
- List of AIIMS Colleges In India
- Medical Colleges in Maharashtra
- Medical Colleges in India Accepting NEET PG
- NEET College Predictor
- NEET PG College Predictor
- NEET MDS College Predictor
- NEET Rank Predictor
- DNB PDCET College Predictor
- NEET Admit Card 2024
- NEET PG Application Form 2024
- NEET Cut off
- NEET Online Preparation
- Download Helpful E-books
- Colleges Accepting Admissions
- Top Law Colleges in India
- Law College Accepting CLAT Score
- List of Law Colleges in India
- Top Law Colleges in Delhi
- Top NLUs Colleges in India
- Top Law Colleges in Chandigarh
- Top Law Collages in Lucknow
Predictors & E-Books
- CLAT College Predictor
- MHCET Law ( 5 Year L.L.B) College Predictor
- AILET College Predictor
- Sample Papers
- Compare Law Collages
- Careers360 Youtube Channel
- CLAT Syllabus 2025
- CLAT Previous Year Question Paper
- NID DAT Exam
- Pearl Academy Exam
Predictors & Articles
- NIFT College Predictor
- UCEED College Predictor
- NID DAT College Predictor
- NID DAT Syllabus 2025
- NID DAT 2025
- Design Colleges in India
- Top NIFT Colleges in India
- Fashion Design Colleges in India
- Top Interior Design Colleges in India
- Top Graphic Designing Colleges in India
- Fashion Design Colleges in Delhi
- Fashion Design Colleges in Mumbai
- Top Interior Design Colleges in Bangalore
- NIFT Result 2024
- NIFT Fees Structure
- NIFT Syllabus 2025
- Free Design E-books
- List of Branches
- Careers360 Youtube channel
- IPU CET BJMC
- JMI Mass Communication Entrance Exam
- IIMC Entrance Exam
- Media & Journalism colleges in Delhi
- Media & Journalism colleges in Bangalore
- Media & Journalism colleges in Mumbai
- List of Media & Journalism Colleges in India
- CA Intermediate
- CA Foundation
- CS Executive
- CS Professional
- Difference between CA and CS
- Difference between CA and CMA
- CA Full form
- CMA Full form
- CS Full form
- CA Salary In India
Top Courses & Careers
- Bachelor of Commerce (B.Com)
- Master of Commerce (M.Com)
- Company Secretary
- Cost Accountant
- Charted Accountant
- Credit Manager
- Financial Advisor
- Top Commerce Colleges in India
- Top Government Commerce Colleges in India
- Top Private Commerce Colleges in India
- Top M.Com Colleges in Mumbai
- Top B.Com Colleges in India
- IT Colleges in Tamil Nadu
- IT Colleges in Uttar Pradesh
- MCA Colleges in India
- BCA Colleges in India
Quick Links
- Information Technology Courses
- Programming Courses
- Web Development Courses
- Data Analytics Courses
- Big Data Analytics Courses
- RUHS Pharmacy Admission Test
- Top Pharmacy Colleges in India
- Pharmacy Colleges in Pune
- Pharmacy Colleges in Mumbai
- Colleges Accepting GPAT Score
- Pharmacy Colleges in Lucknow
- List of Pharmacy Colleges in Nagpur
- GPAT Result
- GPAT 2024 Admit Card
- GPAT Question Papers
- NCHMCT JEE 2024
- Mah BHMCT CET
- Top Hotel Management Colleges in Delhi
- Top Hotel Management Colleges in Hyderabad
- Top Hotel Management Colleges in Mumbai
- Top Hotel Management Colleges in Tamil Nadu
- Top Hotel Management Colleges in Maharashtra
- B.Sc Hotel Management
- Hotel Management
- Diploma in Hotel Management and Catering Technology
Diploma Colleges
- Top Diploma Colleges in Maharashtra
- UPSC IAS 2024
- SSC CGL 2024
- IBPS RRB 2024
- Previous Year Sample Papers
- Free Competition E-books
- Sarkari Result
- QnA- Get your doubts answered
- UPSC Previous Year Sample Papers
- CTET Previous Year Sample Papers
- SBI Clerk Previous Year Sample Papers
- NDA Previous Year Sample Papers
Upcoming Events
- NDA Application Form 2024
- UPSC IAS Application Form 2024
- CDS Application Form 2024
- CTET Admit card 2024
- HP TET Result 2023
- SSC GD Constable Admit Card 2024
- UPTET Notification 2024
- SBI Clerk Result 2024
Other Exams
- SSC CHSL 2024
- UP PCS 2024
- UGC NET 2024
- RRB NTPC 2024
- IBPS PO 2024
- IBPS Clerk 2024
- IBPS SO 2024
- Top University in USA
- Top University in Canada
- Top University in Ireland
- Top Universities in UK
- Top Universities in Australia
- Best MBA Colleges in Abroad
- Business Management Studies Colleges
Top Countries
- Study in USA
- Study in UK
- Study in Canada
- Study in Australia
- Study in Ireland
- Study in Germany
- Study in China
- Study in Europe
Student Visas
- Student Visa Canada
- Student Visa UK
- Student Visa USA
- Student Visa Australia
- Student Visa Germany
- Student Visa New Zealand
- Student Visa Ireland
- CUET PG 2024
- IGNOU B.Ed Admission 2024
- DU Admission 2024
- UP B.Ed JEE 2024
- LPU NEST 2024
- IIT JAM 2024
- IGNOU Online Admission 2024
- Universities in India
- Top Universities in India 2024
- Top Colleges in India
- Top Universities in Uttar Pradesh 2024
- Top Universities in Bihar
- Top Universities in Madhya Pradesh 2024
- Top Universities in Tamil Nadu 2024
- Central Universities in India
- CUET Exam City Intimation Slip 2024
- IGNOU Date Sheet
- CUET Mock Test 2024
- CUET Admit card 2024
- CUET PG Syllabus 2024
- CUET Participating Universities 2024
- CUET Previous Year Question Paper
- CUET Syllabus 2024 for Science Students
- E-Books and Sample Papers
- CUET Exam Pattern 2024
- CUET Exam Date 2024
- CUET Cut Off 2024
- CUET Exam Analysis 2024
- IGNOU Exam Form 2024
- CUET 2024 Exam Live
- CUET Answer Key 2024
Engineering Preparation
- Knockout JEE Main 2024
- Test Series JEE Main 2024
- JEE Main 2024 Rank Booster
Medical Preparation
- Knockout NEET 2024
- Test Series NEET 2024
- Rank Booster NEET 2024
Online Courses
- JEE Main One Month Course
- NEET One Month Course
- IBSAT Free Mock Tests
- IIT JEE Foundation Course
- Knockout BITSAT 2024
- Career Guidance Tool
Top Streams
- IT & Software Certification Courses
- Engineering and Architecture Certification Courses
- Programming And Development Certification Courses
- Business and Management Certification Courses
- Marketing Certification Courses
- Health and Fitness Certification Courses
- Design Certification Courses
Specializations
- Digital Marketing Certification Courses
- Cyber Security Certification Courses
- Artificial Intelligence Certification Courses
- Business Analytics Certification Courses
- Data Science Certification Courses
- Cloud Computing Certification Courses
- Machine Learning Certification Courses
- View All Certification Courses
- UG Degree Courses
- PG Degree Courses
- Short Term Courses
- Free Courses
- Online Degrees and Diplomas
- Compare Courses
Top Providers
- Coursera Courses
- Udemy Courses
- Edx Courses
- Swayam Courses
- upGrad Courses
- Simplilearn Courses
- Great Learning Courses
Tsunami Essay
Giant waves caused by earthquakes or volcanic eruptions in the sea are known as tsunamis . The height of tsunami waves does not substantially rise as they approach the ocean's depths. However, as the waves move inland, the ocean's depth declines, causing them to rise to ever-higher heights. Although tsunamis majorly affect only coastal areas, they have a tremendous amount of destructive power and can have an impact on entire ocean basins. Here are a few sample essays on "Tsunami".
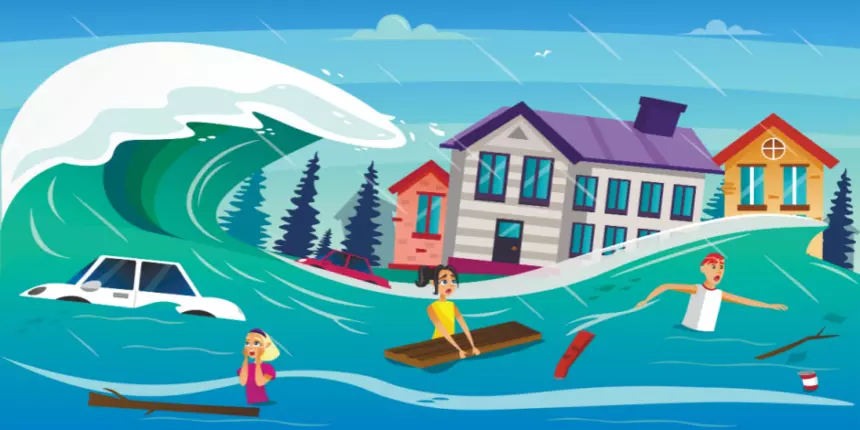
100 Words Essay On Tsunami
The first people to declare the existence of tsunamis were the Greeks. The Greeks considered a tsunami to be a ground quake. The only thing that separates tsunamis from earthquakes is that tsunamis happen in the ocean. Consequently, it is very difficult to limit the magnitude and spread of tsunamis. The ecology suffers significantly as a result of tsunamis. Buildings, ecosystems, livelihoods, and other things are destroyed.
Underwater earthquakes and volcanic eruptions play a crucial role in the development of tsunamis. Tsunamis are caused by various sources, including ground sinking, explosives, etc. Volcanic eruptions beneath the ocean's surface also cause tsunamis to occur. It is commonly known that tsunamis frequently happen in the Pacific Ocean.
200 Words Essay On Tsunami
The word "tsunami," meaning "harbour wave," is of Japanese origin. A tsunami is a series of lengthy water waves that are caused by ocean floor tremors and volcanic eruptions. A landslide will be the primary effect of the earthquake, barring any failure to produce a tsunami on the inside of the seas.
Warning Signs About Tsunamis
The ecology is destroyed by tsunamis , just like any other natural disaster. When a tsunami hits, the ocean waves accelerate to 420 km/h. There are warning signs advising individuals to get away if a tsunami is approaching in several places along the western coastline of the United States, which is vulnerable to tsunamis from the Pacific Ocean.
Based on details about the event that caused the tsunami, the topography of the sea floor, and the coastal landmass, computer simulations can roughly forecast the tsunami's arrival and damage.
Signals By Animals | Animals in the neighbourhood provide one of the first warnings. Before the flood comes, a lot of creatures recognise danger and seek higher ground. Marine life is impacted by tsunamis as well. The ability to predict earthquakes, tsunamis, and other natural disasters could be achieved by closely observing their behaviour.
Tsunami Warning Systems | The public can now be alerted to tsunamis before they reach the coasts in areas with a high risk of flooding by using the tsunami warning systems that are available nowadays.
500 Words Essay On Tsunami
A tsunami is a natural disaster that originates under water and is brought on by the waves that an earthquake causes to be generated in the ocean. The tsunami's impacts were initially reported by Greeks on Earth. They claim that earthquakes on land and tsunamis are identical. The sole distinction between a tsunami and an earthquake is that a tsunami results from an oceanic event. Because of this, it is practically impossible to manage the height and intensity of the tsunami.
Tsunami In India
In 2004, India suffered from a terrible tsunami. The tsunami's source was, though, close to Indonesia. It was estimated that 2 lakh people died due to the tsunami. The waves covered thousands of kilometres in places like Bangladesh, Sri Lanka, Thailand, India, Indonesia, and the Maldives.
In the Pacific Ocean, tsunamis dominate. They are likely to occur in a region with more massive bodies. A tsunami may be aided in its progression into a step-like wave by open bays and coastlines near extremely deep oceans.
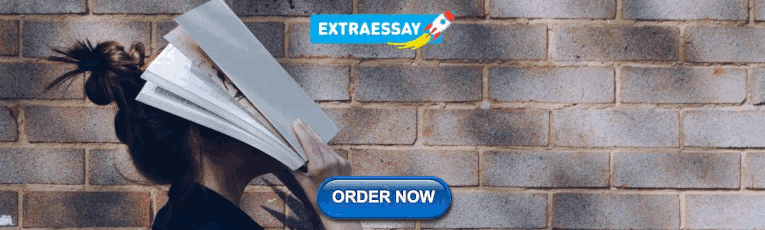
Controlling Destruction
Here are some steps that can be taken by the government to prevent Tsunami and especially the destruction it brings:-
Infrastructure | Government expenditures can go toward developing infrastructure that is robust, highly secure, and capable of withstanding a tsunami's impact. The height should be sufficient to prevent the tsunami's upper wave from conquering it. A tsunami-prone area can be protected from intensive development and habitational activity.
Warning Systems | The local government can install a quick and effective early warning system. This would assist in raising everyone's level of alertness. By doing so, it might be possible to reduce the loss of human life by getting more people to migrate or escape dangerous locations.
Awareness | It is vitally important to inform people about the effects of tsunamis and their potential consequences. They must be instructed on recognising and understanding the early tsunami warning signs. Under challenging circumstances, they must learn to stay fully prepared rather than panic and anxiously rush around.
Afforestation | Another alternative is to plant trees that can absorb the force of tidal waves, like mangroves, along the coast and its borders. These may lessen a tsunami's effects and limit the devastation they create.
My Experience Of Mock Drills
I remember mock drills were conducted in my school and high school as they were located near the coast of the Arabian sea. These mock drills were conducted to prepare the students for any emergency circumstances like tsunamis, earthquakes and fire disasters. We were taught how to escape in a planned manner which does not create a problematic situation. We were strictly instructed not to use lifts in such a scenario. During heavy rains, school was kept off since Tsunami is more likely to strike during rains.
Tsunamis are less common than other types of natural disasters. However, they may still cause significant damage. A tsunami's most severe consequence is the massive number of deaths. As a result, individuals have little time to flee or escape a tsunami's immediate, nearly silent impact. Tsunamis significantly harm the environment in addition to bringing severe degradation. Marine life suffers extreme damage.
Applications for Admissions are open.

Aakash iACST Scholarship Test 2024
Get up to 90% scholarship on NEET, JEE & Foundation courses

ALLEN Digital Scholarship Admission Test (ADSAT)
Register FREE for ALLEN Digital Scholarship Admission Test (ADSAT)

JEE Main Important Physics formulas
As per latest 2024 syllabus. Physics formulas, equations, & laws of class 11 & 12th chapters

PW JEE Coaching
Enrol in PW Vidyapeeth center for JEE coaching

PW NEET Coaching
Enrol in PW Vidyapeeth center for NEET coaching

JEE Main Important Chemistry formulas
As per latest 2024 syllabus. Chemistry formulas, equations, & laws of class 11 & 12th chapters
Download Careers360 App's
Regular exam updates, QnA, Predictors, College Applications & E-books now on your Mobile

Certifications

We Appeared in

- Skip to primary navigation
- Skip to main content
- Skip to primary sidebar
UPSC Coaching, Study Materials, and Mock Exams
Enroll in ClearIAS UPSC Coaching Join Now Log In
Call us: +91-9605741000
Tsunami: Everything You Need to Know
Last updated on October 28, 2022 by ClearIAS Team
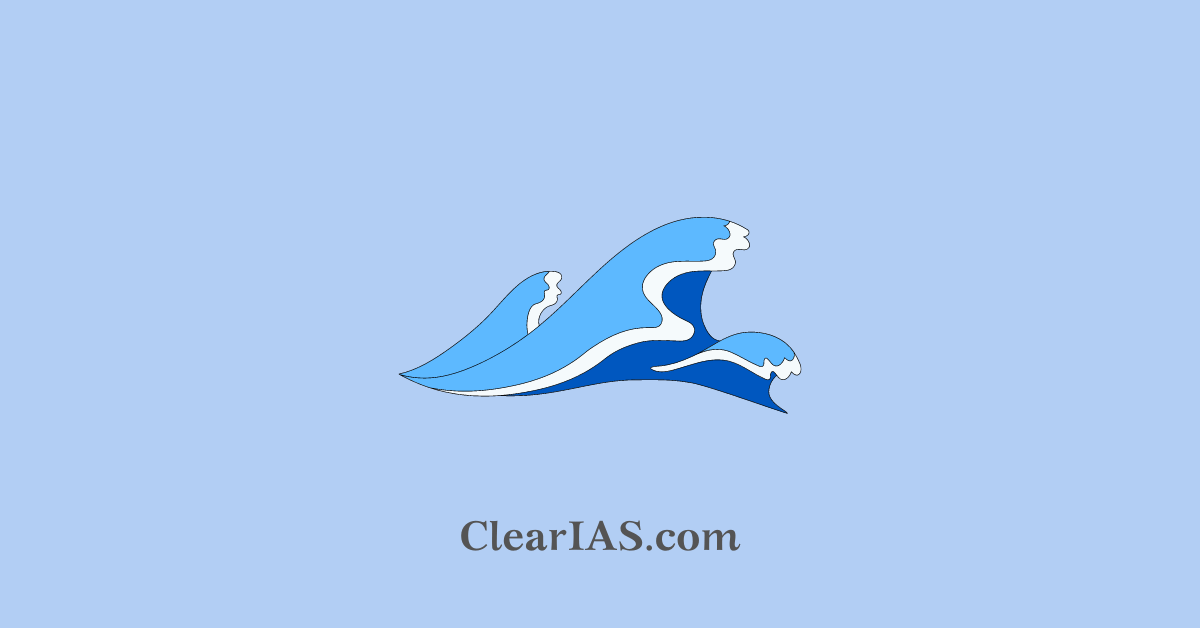
Based on the High-Powered Committee on Disaster Management’s report (2001), the term “tsunami” refers to an ocean wave that is caused by an ocean event, such as an earthquake, landslide, or volcanic eruption.
A tsunami is not just one wave; rather, it is a sequence of waves brought on by geological processes occurring near or below the ocean’s surface.
These waves have the potential to grow to tremendous sizes.
Table of Contents
Formation of Tsunamis
Several factors and incidents can lead to the formation of tsunamis, a few important of them are-
Undersea Earthquakes
Although landslides, volcanic eruptions, or even the impact of a sizable meteorite striking the water can all result in tsunamis, the most catastrophic tsunamis are produced by massive undersea earthquakes.
These destructive tsunami waves are produced by the vertical movement of the entire water column, and such a situation may arise as a result of sudden, jerky motions of fault blocks on the seabed.
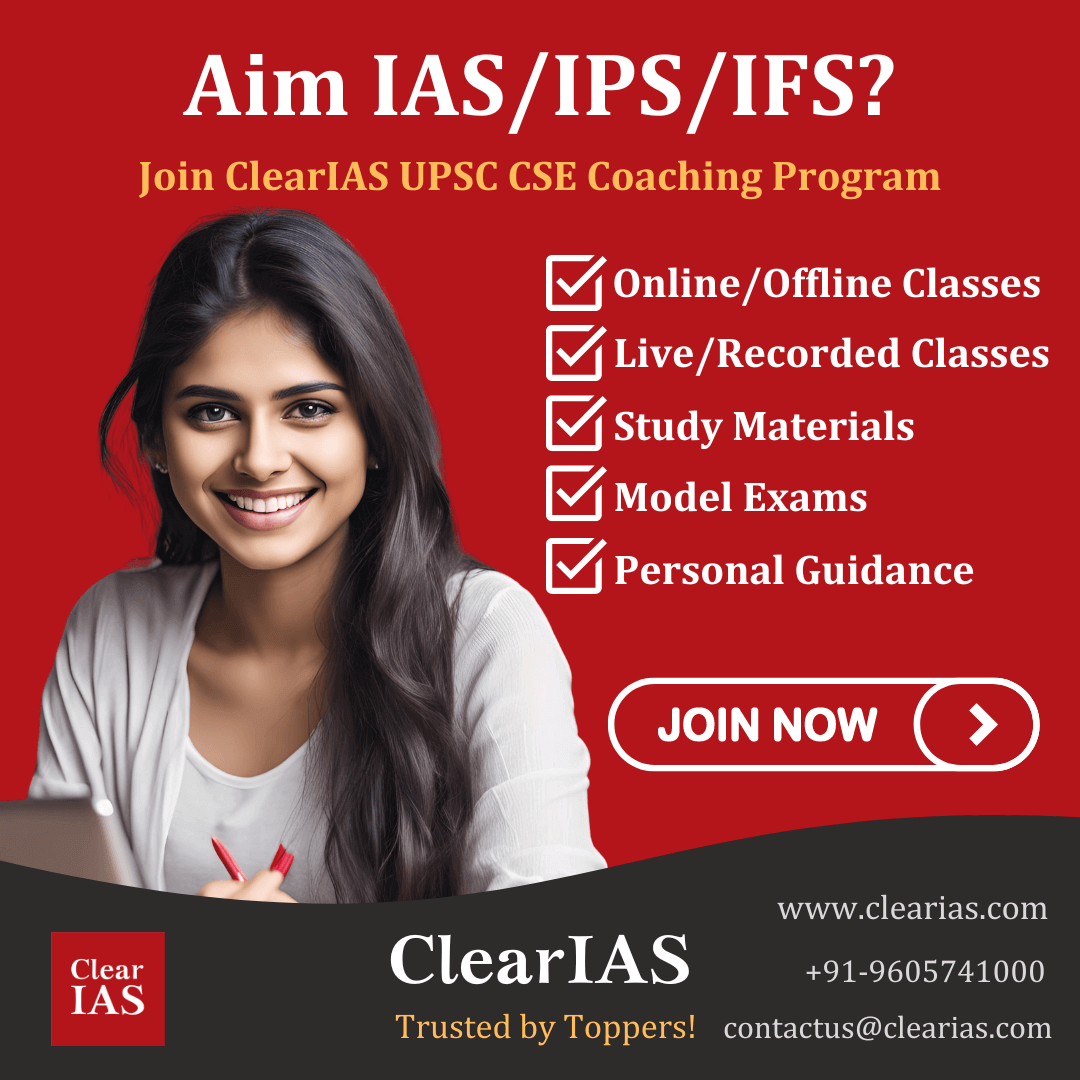
It should be emphasised that horizontal displacement of the sea floor usually does not cause a tsunami.
In addition, not all undersea earthquakes create tsunamis, as it depends upon the nature and degree of displacement of the seawater columns.
Other natural disasters like rock fall, landslides, and icefalls can cause the displacement of seawater which can lead to tsunami waves. These waves rapidly travel away from the source due to the dissipation of energy and create havoc in the nearby coastlines.
Volcanic Eruptions
When a powerful underwater volcanic eruption occurs, a significant amount of seawater is abruptly displaced, which results in the formation of tsunami waves.
Meteorites and Asteroids
In the event that meteorites and asteroids fall into the water, there is a chance that a tsunami could emerge.
Despite the extreme rarity of such an impact, the researcher gives the destructive potential of a tsunami brought on by a near-Earth asteroid.
Propagation of the Tsunamis
Tsunamis are made up of a series of extremely long waves that travel away from their point of origin on the ocean’s surface in all directions.
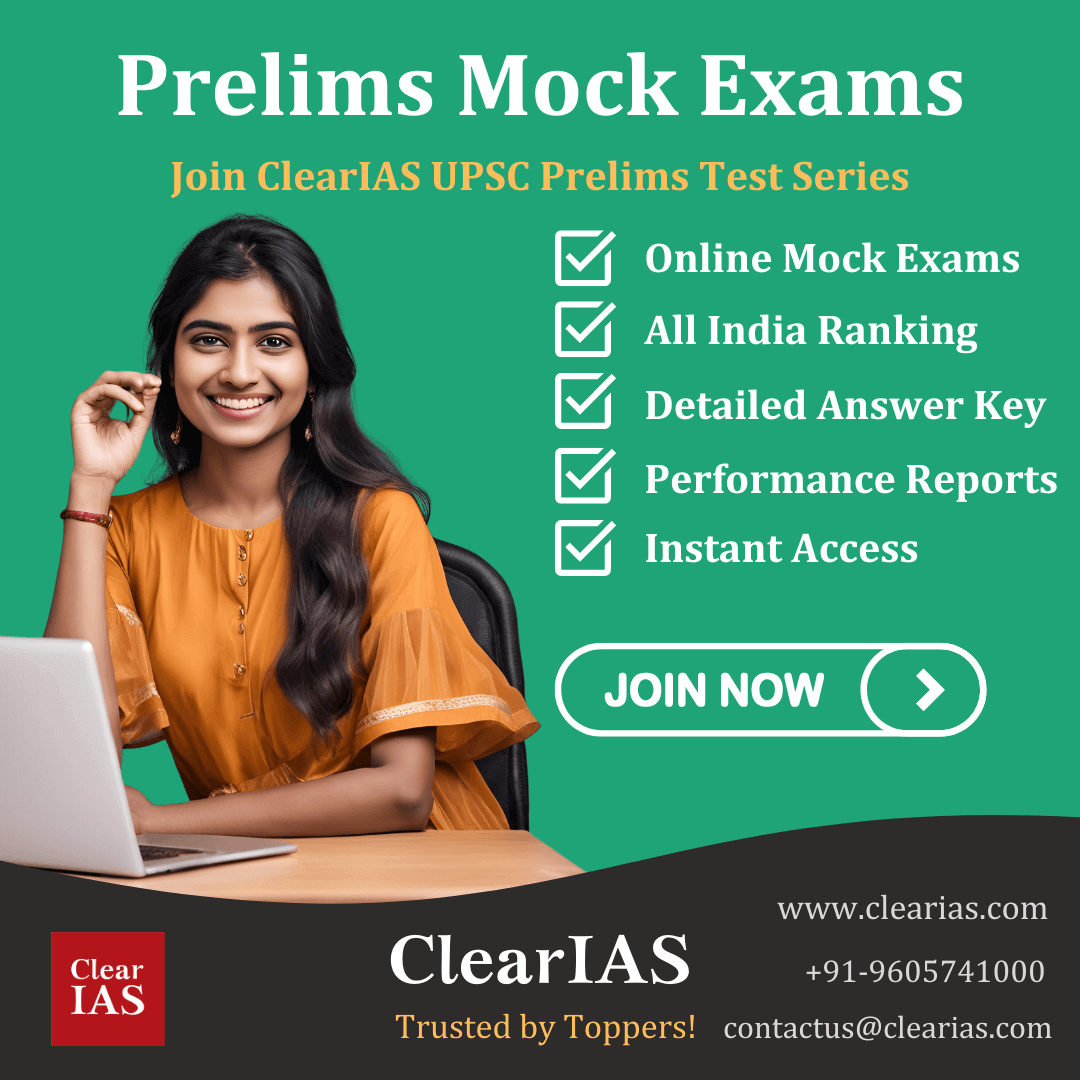
The tsunamis cannot be seen or heard because of their extremely long wavelength and very low amplitude in deep water. Therefore, as the killer waves pass by underneath boats at fast speeds, passengers cannot feel or see the tsunami waves.
As the tsunamis leave the deep water of the open ocean and travel towards the shallow water, they are transformed in two ways. They first experience a significant reduction in speed, then a massive increase in height.
Effects of Tsunamis
Residents of coastal areas are in serious danger from tsunamis. They emerge out of nowhere and strike the seashore like a water bomb with enormous, powerful waves that are travelling with great speed.
Loaded with enormous energy, the killer waves create havoc by flooding hundreds of metres inland, past the typical high water level.
Tsunami in the Indian Ocean
Multiple times, tsunamis have been caused in the Indian Ocean. On Indonesia’s Java and Sumatra islands in 1883, tsunamis generated by Krakatoa eruptions killed 36,000 people.
Damages Caused by Tsunami
Various types of damages caused by the tsunami are briefly described below.
Tsunamis always claim lakhs of lives.
The Andaman and Nicobar Islands in India were the most vulnerable because they were situated closest to the earthquake’s epicentre and the source of the tsunamis.
The coastal regions of Tamil Nadu were the primary targets of the tsunami attack on the Indian subcontinent.
Indonesia and Sri Lanka were the nations that recorded death tolls that were higher than those in India.
- Loss of Property
As a result of tsunami attacks, property worth millions of rupees gets damaged. Houses, public buildings, transportation systems, and other infrastructure components suffer damage that is practically irreparable.
In the tsunami of 26th December 2004, the coastal regions of India, which include sizable coastal tracts in Tamil Nadu, Andhra Pradesh, Pondicherry, and Kerala, have incurred billions of rupees worth of financial losses, according to preliminary findings of the Indian government.
- Physiographical Changes
According to reports, the tsunami on December 26, 2004, many islands’ water levels has risen, causing a number of their beaches to split in two. Indira Point, the southernmost point of the Indian Union, was nearly entirely washed out, reducing the coastline inward.
- The Motion of the Earth
According to a US Geological Survey, the earthquake’s enormous energy release caused the earth to tilt slightly. According to NASA’s geophysicist Richard Gross, the earthquake may have permanently accelerated the rotation of the planet due to a shift of mass toward its centre.
- A Decline in Soil Fertility and Agricultural Production
Vast, low-lying coastal areas were submerged by water, which made the soil more saline and decreases agricultural production. The soil’s extreme salinity renders the field unproductive.
- Effect on Marine Life
The marine life of the Ocean gets severely impacted by deadly tsunamis.
The coral reefs of the Andaman and Nicobar Islands archipelago were severely damaged or destroyed as a result of the tsunamis that occurred in 2004. It was possible that some of the archipelagic species might get extinct.
The destructive tsunamis caused severe losses in the fishing industry as well.
Large marine mammals including whales, dolphins, and other species were negatively impacted in their breeding, feeding, and other activities.
Tsunami Disaster Management and Mitigation
Oceanic waves known as tsunamis are so strong that it is impossible for humans to contain or control them. However, in the event that tsunamis are approaching, the following advice can be useful.
- Get off the beaches and head for higher ground.
- Stay away from rivers leading into the ocean.
- Wait for all clear as there can be several giant waves in succession.
Advance Warning about Tsunamis
The most effective strategy to protect vulnerable communities from the wrath of deadly tsunami waves is to provide them with advance notice of impending tsunamis. The common individual will benefit from the following advice in understanding the threat posed by tsunamis.
It is possible to predict the onslaught of tsunamis by studying their speed, wavelength and depth of the sea. Tsunamis often take some time to reach the seashore from their point of origin because, as they get closer to the shallow sea, their wavelength and speed are drastically reduced.
However, when they get to the shallow coastal seas, their energy is compressed, and they turn into deadly waves that destroy the coastal inhabitants.
Therefore, a timely warning of tsunamis a few hours in advance can prevent the loss of thousands of lives and millions of rupees worth of property.
Tsunami Warning System in India
Unfortunately, India and other Indian Ocean-rim countries did not have tsunami warning systems in 2004 when the deadly tsunamis hit the coasts of the Indian Ocean.
After the catastrophe, India decided to install its own tsunami warning system rather than becoming a part of the Pacific Warning System .
The system comprises a real-time network of seismic stations, bottom pressure recorders (BPR) and tide gauges to monitor earthquakes in the sea and tsunamis triggered by them.
Why the Problem is Consistent
Although tsunamis constitute a natural tragedy and man has hardly any control over such a powerful natural phenomenon yet man’s own misdeeds have made the situation very dangerous and helped in aggravating the fury of tsunamis.
For instance, no building is permitted within 500 metres of the coast. In India, it is required by law to keep this area of land undeveloped, yet many development projects (residential, commercial, recreational, etc.) are still carried out without regard for the law. The population in this region is most susceptible to tsunamis and other maritime dangers.
Mining sand and other minerals from the sea makes the concerned area highly vulnerable to tsunamis. The sand deposited on the sea coast absorbs much energy from the waves and saves much of the coastal areas from their fury. There is an urgent need to check unwanted construction and mining in ecologically sensitive coastal areas.
Article Written By: Priti Raj
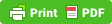
Aim IAS, IPS, or IFS?
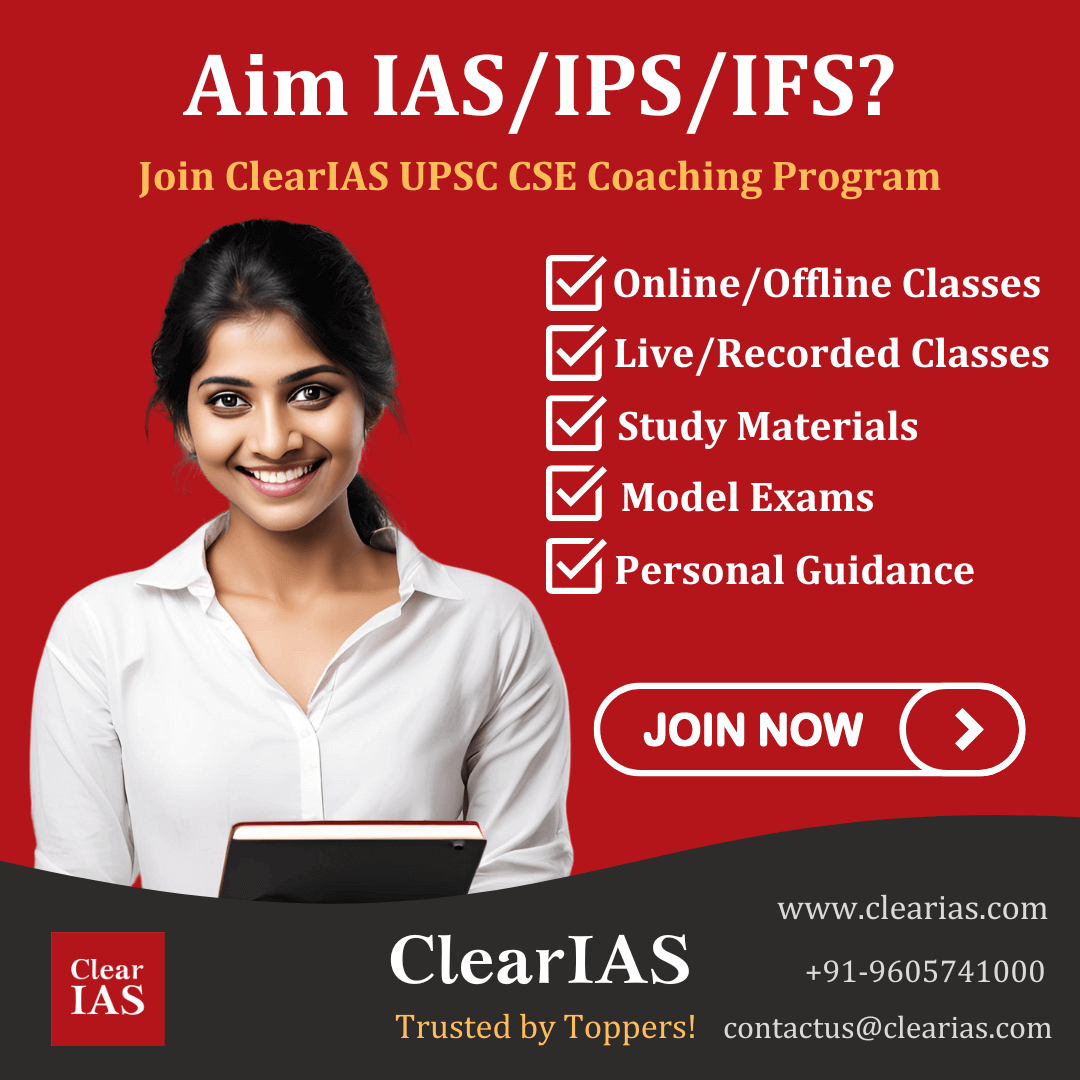
About ClearIAS Team
ClearIAS is one of the most trusted learning platforms in India for UPSC preparation. Around 1 million aspirants learn from the ClearIAS every month.
Our courses and training methods are different from traditional coaching. We give special emphasis on smart work and personal mentorship. Many UPSC toppers thank ClearIAS for our role in their success.
Download the ClearIAS mobile apps now to supplement your self-study efforts with ClearIAS smart-study training.
Reader Interactions
Leave a reply cancel reply.
Your email address will not be published. Required fields are marked *
Don’t lose out without playing the right game!
Follow the ClearIAS Prelims cum Mains (PCM) Integrated Approach.
Join ClearIAS PCM Course Now
UPSC Online Preparation
- Union Public Service Commission (UPSC)
- Indian Administrative Service (IAS)
- Indian Police Service (IPS)
- IAS Exam Eligibility
- UPSC Free Study Materials
- UPSC Exam Guidance
- UPSC Prelims Test Series
- UPSC Syllabus
- UPSC Online
- UPSC Prelims
- UPSC Interview
- UPSC Toppers
- UPSC Previous Year Qns
- UPSC Age Calculator
- UPSC Calendar 2024
- About ClearIAS
- ClearIAS Programs
- ClearIAS Fee Structure
- IAS Coaching
- UPSC Coaching
- UPSC Online Coaching
- ClearIAS Blog
- Important Updates
- Announcements
- Book Review
- ClearIAS App
- Work with us
- Advertise with us
- Privacy Policy
- Terms and Conditions
- Talk to Your Mentor
Featured on
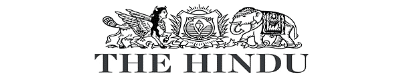
and many more...
Take ClearIAS Mock Exams: Analyse Your Progress
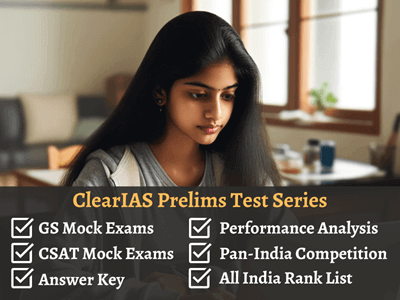
Analyse Your Performance and Track Your All-India Ranking
Ias/ips/ifs online coaching: target cse 2025.
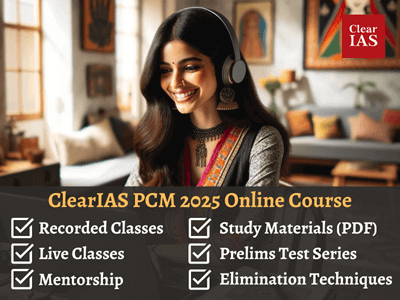
Are you struggling to finish the UPSC CSE syllabus without proper guidance?
Talk to our experts
1800-120-456-456
Disaster Management Essay
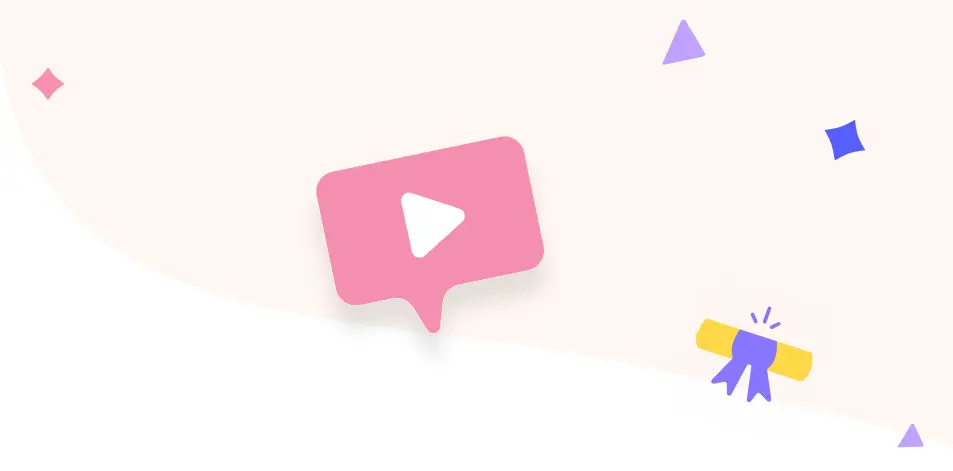
Essay on Disaster Management
Disaster Management is the arrangement and management of the resources following a disaster, be it natural or man-made. There are so many organizations who are dealing with various types of disastrous situations from the humanitarian aspect. Some disasters are just the consequences of human hazards and some are caused by natural calamity. However, we can prevent them by taking the necessary emergency measures to save and preserve lives. As natural disasters cannot be predicted, they can take place anywhere at any time.
Vedantu has provided an essay on Disaster management on this page. Students who have received an assignment to write an essay on Disaster Management or preparing an essay for examination can refer to this page to understand the pattern. Any student or parent can directly visit Vedantu site or download the app on the phone to get access to the study materials.
Disaster Management’ is the simple term of management which embraces loads of disaster-related activities. Disaster occurs frequently in some parts of the world. Japan is the best example of it. Japanese people are annoyed on Tsunamis and earthquakes. The local scene is not much different from the global one. No one could forget the cyclone in Orissa, Earthquake in Gujarat or even the Mumbai Terrorist Attack.
Natural and man-made are the two categories of the disaster. Natural disasters are those which occurred due to sudden changes in the environment or topography causing uncountable human as well as economic loss. Earthquakes, volcanic eruptions, floods and cloudbursts are few of the natural disasters. Manual intentional made disasters are man-made disasters. For example, Gas leakage, terrorist attacks, fire, oil spills. Man-made disasters are the result of human intentions or might be because of workmanship or technical errors. The count of man-made and natural disasters is rising rapidly.
Here are a few things which will help us to deal with earthquakes. The significant information about Natural calamities is predicted easier and is being shared within the public by the central bureau. Furthermore, earthquake-resistant structures are constructed considering, ‘Precaution is Better Than Cure’. Reflexes are made so strong that cover of solid platforms such as a table and chair should be taken as soon as the danger is sensible while the cover of trees, electric poles or buildings is avoided as far as possible. Keep in touch with local news during heavy rainy days. Any flood is preceded with significant time. Making proper use of divine buffer time for safety is advisable. Strategically planning of water reservoirs, land uses, tree plantation, rainwater harvesting techniques help us increase immunity power to fight against the drought.
National Disaster Management Authority (NDMA), American Red Cross, Federal Emergency Management Agency, International Association of Emergency Managers etc. are the best disaster management authorities. NDMA is a core body which obeys the law of Disaster Management. The reputed disaster managers are stuck in finding plans for rescuing from the loss of disaster. Moreover, to counteract the effect of disaster Rajya Sabha passed the ‘Disaster Management Act’ on 23 December 2005 which includes 11 chapters and 79 sections in it. Honourable Prime Minister of India Mr Narendra Modi holds the position of chairman of it.
Youngsters should motivate themselves to learn and practice plenty of disaster management techniques and arrange the camps regarding it. Today, everyone is fighting against one of the breathtaking disasters named COVID-19 which is as big as fighting in world war. Avoiding the crowd, wearing the mask are the basic precautions suggested by the World Health Organization (WHO) during this period of fighting. This disease spreads mostly amongst the people who come in close contact with the infected one. So, it's suggested to keep a safe distance of around 3 meters within the others. This is being termed as ‘Social Distancing’. Nature is not cruel nor is it human. Just small management skills help us withstand before, in and after disasters. Uncountable suggestions and instructions in disaster management but few which apply every time must be followed.
Stages of Disaster Management
With proper implementation and structured action, we can prevent or lessen the impact of natural or man-made disasters. There are certain stages involving the cycle for disaster management plan which incorporates policies and emergency responses required for a prospectively complete recovery. The stages are –
The most preferred way to deal with disasters is to be proactive in their prevention rather than rushing later for their cure. This implies recognition of potential hazards and working towards infrastructure to mitigate their impact. This stage in the management cycle involves setting up permanent measures to minimize disaster risk.
Setting up an evacuation plan in a school, training the teachers to lead the students towards safe structures in the event of earthquake, tornado or fire, planning a strong base for high raised sky-scrapers to prepare for earthquakes and designing a city in such a manner that reduces the risk of flooding are some examples of measures takes for disaster prevention.
Mitigation is the first and the foremost attempt to save human lives during the time of disaster or their recovery from the aftermath. The measures which are taken can be both structural and non-structural.
Structural mitigation measures could include transforming the physical characteristics of a building or the surroundings to curb the effect, for example, clearing out of the trees around your house, ensuring that storms don’t knock down the trees and send them crashing into the house. Non-structural measures could include amending the building or locality codes to enhance safety and prevent disasters.
Preparedness
Preparedness is a process that involves a social community where the trained, or the head of the community, businesses and institutions demonstrate the plan of action which is supposed to be executed during the event of a disaster. It is an ongoing continuous process with anticipation of a calamity, which involves training, evaluating and taking corrective action with the highest level of alertness. Some examples of such prevention measures are fire drills, shooter drills and evacuation rehearsals.
The response is the action taken after the disaster has occurred to retrieve some life from it. It includes short-term and long-term responses. In ideal situations, the disaster-management leader will coordinate the use of resources in the restoration process and minimize the risk of further property damage.
During this stage, the area of the calamity is cleared if it poses any further threat to human as well as environmental life. For example, evacuation of the city of Chernobyl, Ukraine, is a responsive action against a disaster.
The fifth and last stage in the process of the disaster management plan is the recovery stage. This can sometimes take years or decades to happen. The larger mass of a city is also sometimes part of the recovery from a disaster. The greatest and the most infamous example of this is the Hiroshima and Nagasaki nuclear attacks on Japan, it took the people of those cities years and decades to recover from that man-made calamity.
It took years of effort to stabilize the area and restore essential community or individual functions. The recovery stage prioritizes the basic essential needs of human survival like food, drinkable water, utilities, transportation and healthcare over less-essential services. Eventually, this stage is all about coordinating with individuals, communities and businesses to help each other to restore a normal or a new normal, as in the case of Covid-19.
How to Act as a Responsible Person During a Time of Disaster?
Some people have more experience than others with managing natural or man-made disasters and their prevention of them. Although this is that subject of life which should be studied and implemented by every business or community. As it is said rightly, “prevention is better than cure”, and any organization or an individual or a community can be hit by a disaster sooner or later, whether it's something as minor as a prolonged power cut or a life-threatening hurricane or an earthquake. Usually, the pandemics train us, as a social and political community, to deal with natural calamities and compel the organizations responsible for it, to build an infrastructure for its prevention.
To act responsibly and pro-actively during the event of a disaster, we have got to be prepared and equipped as a nation, individually and as a social community. To be well-educated and read with the aspects of disaster management is to be responsible for the handling of it.
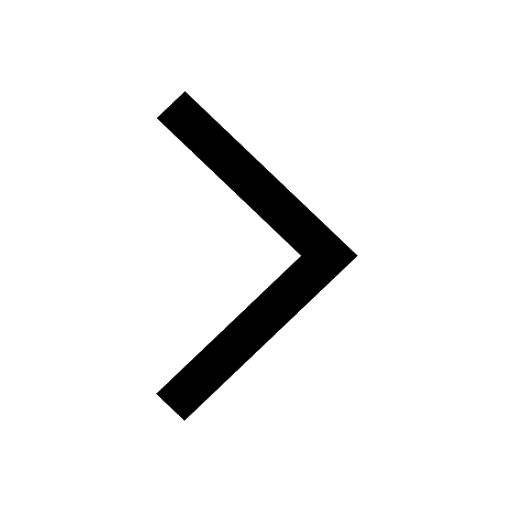
FAQs on Disaster Management Essay
1. What is Disaster Management?
In simpler words, disaster management can be defined as the arrangement of resources and precautions to deal with all humanitarian aspects during an emergency. Disasters are the consequences of natural or human hazards. Earthquakes, floods, volcanic eruptions, hurricanes are some of the deadliest natural disasters to name a few. Examples of man-made disasters are bomb blast, radiations, transport accidents, terrorist attacks etc.
2. What is the Main Aim of Disaster Management?
The main aim of disaster management is prevention, rescue and recovery from the trauma, and development.
3. How to Write an Essay on Disaster Management?
Disaster management refers to the response to an emergency situation to make it as normal as possible. While writing an essay on Disaster Management, you can start with an introduction, then go on with the definition, the types of disaster management, a little in-depth explanation along with examples, and finish it off with a conclusion.
4. Can I Get a Sample Essay on Disaster Management from Vedantu?
Yes, the essay mentioned on this page is about Disaster Management. This essay has been written by the experts of Vedantu keeping the understanding ability of the students of each class.
5. What are the career opportunities in the field of Disaster management?
People looking for career opportunities in the field of disaster management have many pathways to approach it. Some examples of the jobs relating to this line of work are crisis-management leader, disaster-assistance specialist and emergency-planning coordinator. These are the roles which call for varying levels of responsibility in preparing a city or a company for catastrophic events. The job roles can be approached with earning a master’s degree in emergency and crisis management.
Tsunami - Meaning, Characteristics & Mitigation Measures
The word ‘Tsunami’ literally means ‘harbour waves’. These are the series of waves and the characteristic feature that differentiates these waves are is their long wavelength.
The information about the Tsunami is important for the general studies paper of the most coveted IAS Exam .
Explore The Ultimate Guide to IAS Exam Preparation
Download The E-Book Now!
Aspirants would find this article helpful in preparing for the UPSC 2022 .
What is a Tsunami?
- The series of extremely long waves, Tsunami are very long wavelengths of water caused by a large and sudden displacement of the ocean due to earthquakes, volcanic eruptions etc.
- These are also called seismic sea waves and are one of the most powerful and destructive natural forces.
- When they reach the coast, they can cause dangerous coastal flooding and powerful currents that can last for several hours or days.
- Most tsunamis are caused by large earthquakes. Though, not all earthquakes cause tsunamis.
Know in detail about the Earthquake in the given link.
Characteristics of Tsunami
- Tsunamis are among Earth’s most infrequent hazards and most of them are small and nondestructive.
- Over deep water, the tsunami has very long wavelengths (often hundreds of kilometres long) when a tsunami enters shallow water, its wavelength gets reduced and the period remains unchanged, which increases the wave height.
- Tsunamis have a small amplitude (wave height) offshore. This can range from a few centimetres to over 30 m in height. However, most tsunamis have less than 3 m wave height.
- It radiates in all directions from the point of origin and covers the entire ocean.
- It generally consists of a series of waves, with periods ranging from minutes to hours.
- These are the waves generated by tremors and not by earthquakes themselves.
- There is no season for tsunamis and not all tsunamis act the same. It cannot be predicted where, when and how destructive it will be. A small tsunami in one place may be very large a few miles away.
- An individual tsunami may impact coasts differently. A tsunami can strike any ocean coast at any time. They pose a major threat to coastal communities. The effect of Tsunami would occur only if the epicentre of the tremor is below oceanic waters and the magnitude is sufficiently high.
- The speed of the wave in the ocean depends upon the depth of water. It is more in the shallow water than in the ocean deep. As a result of this, the impact of a tsunami is more near the coast and less over the ocean
Candidates can go through the following links to prepare well for the upcoming civil services examinations-
Causes of Tsunami
How are Tsunami generated?
A Tsunami can be generated only through the vertical movement of the seafloor. Most Tsunamis are generated by earthquakes. Volcanic eruption, underwater explosion, landslides and meteorite impacts are some other causes of Tsunami.
The details of causes of Tsunami is explained below-
Earthquake – Tsunami is generated by the earthquake because of the disturbance of the seafloor and is formed generally with vertical displacement. Most Tsunami is generated by earthquakes that occur along the subduction boundaries of plates along the ocean trenches. The size of the Tsunami is related to the size of the earthquake.
Underwater explosion – A Nuclear Testing by the US generated Tsunami in 1940 and 1950s in Marshall island.
Volcanic eruption – Volcanoes that occur along the Coastal waters can cause several effects that can cause a tsunami.
Landslides – Earthquake and volcanic eruptions generally generate landslides, these landslides when moving into the Oceans, bays and lakes can generate Tsunami.
Meteorite Impacts – Though no historic example as such of meteorite impact has caused Tsunami, the apparent impact of a meteorite about 5 million years ago produced Tsunami leaving deposits along the Gulf Coast of Mexico and the United States.
Tsunami is a geography topic. To aid your geography preparation for UPSC Exam, check the below-mentioned links:
- Geography NCERT Notes for UPSC
- Previous Year Geography Questions of UPSC Mains GS 1
- Download compilation of Geography Questions in UPSC Prelims
How Often Does Tsunami Occur?
- On average, every 15 years a tsunami occurs in the Pacific Ocean.
- Example a be taken from India itself which was the tsunami on Dec 26th, 2004 on the Indian Coast. It caused huge damage to life and property.
How is Tsunami different From a Wind – Generated Wave?
Tsunami should not be confused with the huge wind-generated waves. A wind-generated wave lasts for some seconds whereas a tsunami remains for minutes to hours. A tsunami can be disastrous which a wind-generated wave is not.
Tsunami Vulnerability in India
INCOIS is the body that is vigilant regarding tsunamis on the Indian coast. To know more about the Indian National Centre for Ocean Information Services – INCOIS .
The possible zones are Andaman – Sumatra or Makran (Pakistan).
Tsunami Risk, Hazard & Mitigation Measures
The main damage from the Tsunami comes from the destructive nature of waves. The second effect of the Tsunami includes debris acting as projectiles and the tertiary effect includes the loss of crops and water which leads to famine and disease.
Mitigation Measures
- Effective Planning
- The building of walls was done by Japan.
- Planting trees as done in Tamil Nadu by a village
- Proper relief and rehabilitation preparedness
- Awareness among the masses
UPSC exam-related preparation materials will be found through the links given below.
Aspirants can find complete information about upcoming Government Exams through the linked article .
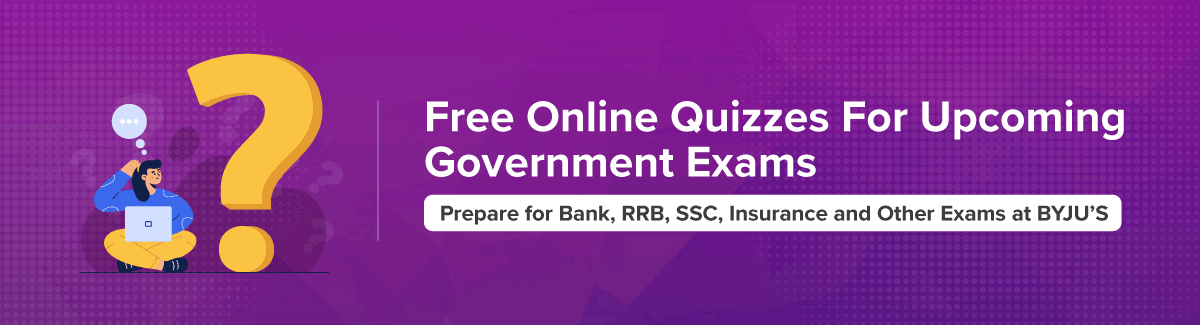
Leave a Comment Cancel reply
Your Mobile number and Email id will not be published. Required fields are marked *
Request OTP on Voice Call
Post My Comment

IAS 2024 - Your dream can come true!
Download the ultimate guide to upsc cse preparation.
- Share Share
Register with BYJU'S & Download Free PDFs
Register with byju's & watch live videos.
Disaster Management Essay for Students and Children
500+ words essay on disaster management.
Nature has various manifestations both gentle as well as aggressive. We see how sometimes it is so calm while the other times it becomes fierce. The calm side is loved by everyone, of course, however, when the ferocious side is shown, devastation happens. As humans cannot control everything, certain things of nature are out of our control.

Similarly, when natural disasters happen, humans cannot control them. However, we can prevent them. In other words, whenever a calamitous situation arises that may disturb the life and ecosystem, we need emergency measures to save and preserve lives. As natural disaster are not predictable, they can take place anywhere at any time. To understand disaster management thoroughly, we need to first identify the types of disasters.
Types of Disasters
If we look at the disasters that have taken place earlier, we can easily say that nature is not merely responsible for them to happen. They happen due to other reasons too. This is why we have classified them in different categories. First comes the natural disasters which are caused by natural processes. They are the most dangerous disaster to happen which causes loss of life and damage to the earth. Some of the deadliest natural disasters are earthquakes , floods, volcanic eruptions, hurricanes, and more.

As no country is spared from any kind of disasters, India also falls in the same category. In fact, the geographical location of India makes it a very disaster-prone country. Each year, India faces a number of disasters like floods, earthquakes, tsunami, landslides, cyclones, droughts and more. When we look at the man-made disasters, India suffered the Bhopal Gas Tragedy as well as the plague in Gujarat. To stop these incidents from happening again, we need to strengthen our disaster management techniques to prevent destructive damage.
Get the huge list of more than 500 Essay Topics and Ideas
Disaster Management
Disaster management refers to the efficient management of resources and responsibilities that will help in lessening the impact of the disaster. It involves a well-planned plan of action so we can make effective efforts to reduce the dangers caused by the disaster to a minimum.
Most importantly, one must understand that disaster management does not necessarily eliminate the threat completely but it decreases the impact of the disaster. It focuses on formulating specific plans to do so. The National Disaster Management Authority (NDMA) in India is responsible for monitoring the disasters of the country. This organization runs a number of programs to mitigate the risks and increase the responsiveness.
Proper disaster management can be done when we make the citizens aware of the precautionary measures to take when they face emergency situations. For instance, everyone must know we should hide under a bed or table whenever there is an earthquake. Thus, the NDMA needs to take more organized efforts to decrease the damage that disasters are causing. If all the citizens learn the basic ways to save themselves and if the government takes more responsive measures, we can surely save a lot of life and vegetation.
{ “@context”: “https://schema.org”, “@type”: “FAQPage”, “mainEntity”: [{ “@type”: “Question”, “name”: “What are the types of disasters?”, “acceptedAnswer”: { “@type”: “Answer”, “text”: “There are essentially two types of disasters. One is natural disasters which include floods, earthquake, tsunami, volcanic eruptions and more. The other is man-made disaster including oil spills, fires” } }, { “@type”: “Question”, “name”: “How can we effectively manage disasters?”, “acceptedAnswer”: { “@type”: “Answer”, “text”:”We must make people aware of the consequences of disasters. Moreover, we can teach them actions and measures to take when they face emergency situations.”} }] }
Customize your course in 30 seconds
Which class are you in.

- Travelling Essay
- Picnic Essay
- Our Country Essay
- My Parents Essay
- Essay on Favourite Personality
- Essay on Memorable Day of My Life
- Essay on Knowledge is Power
- Essay on Gurpurab
- Essay on My Favourite Season
- Essay on Types of Sports
Leave a Reply Cancel reply
Your email address will not be published. Required fields are marked *
Download the App
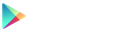
The Global Reach of the 26 December 2004 Sumatra Tsunami
V. titov, 1 a. b. rabinovich, 2,3 h. o. mofjeld, 1 r. e. thomson, 2 and f. i. gonzález 1, 1 noaa/pacific marine environmental laboratory, seattle, washington 2 institute of ocean sciences, department of fisheries and oceans canada, sidney, british columbia, canada 3 p. p. shirshov institute of oceanology, russian academy of sciences, moscow, russia, science, 309 (5743), 2045–2048, 2005. copyright ©2005 by the american association for the advancement of science. further electronic distribution is not allowed..
Numerical model simulations, combined with tide-gauge and satellite altimetry data, reveal that wave amplitudes, directionality, and global propagation patterns of the 26 December 2004 Sumatra tsunami were primarily determined by the orientation and intensity of the offshore seismic line source and subsequently by the trapping effect of mid-ocean ridge topographic waveguides.
- Entire Text
- Gallery of Figures
- Request a reprint through e-mail
to request a reprint.
PMEL Outstanding Papers
PMEL Publications Search
PMEL Homepage
- Search Menu
- Volume 238, Issue 1, July 2024 (In Progress)
- Volume 237, Issue 3, June 2024
- Advance Access
- Applied and Marine Geophysics
- General Geophysical Methods
- Geodynamics and Tectonics
- Geomagnetism and Electromagnetism
- Gravity, Geodesy and Tides
- Heat Flow and Volcanology
- Rock and Mineral Physics, Rheology
- 100 Influential Papers
- Advance Articles
- Express Letters
- Hunga Volcano Special Issue
- East Anatolia Fault Special Issue
- Special Issues
- Why Publish
- Author Guidelines
- Submission Site
- Read & Publish
- Developing Countries Initiative
- Author Resources
- Self-Archiving policy
- Rights and Permissions
- About Geophysical Journal International
- Editorial Board
- About the Royal Astronomical Society
- About the DGG
- Journals on Oxford Academic
- Books on Oxford Academic

Article Contents
1 introduction, 2 interseismic plate-coupling inversion, 3 coupling-based earthquake scenarios, 4 tsunami simulations in the western indian ocean, 5 discussion, 6 conclusions, acknowledgements, data availability.
- < Previous
Constraints from GPS measurements on plate coupling within the Makran subduction zone and tsunami scenarios in the western Indian Ocean

- Article contents
- Figures & tables
- Supplementary Data
Guo Cheng, William D Barnhart, David Small, Constraints from GPS measurements on plate coupling within the Makran subduction zone and tsunami scenarios in the western Indian Ocean, Geophysical Journal International , Volume 237, Issue 1, April 2024, Pages 288–301, https://doi.org/10.1093/gji/ggae046
- Permissions Icon Permissions
Plate-coupling estimates and previous seismicity indicate that portions of the Makran megathrust of southern Pakistan and Iran are partially coupled and have the potential to produce future magnitude 7+ earthquakes. However, the GPS observations needed to constrain coupling models are sparse and lead to an incomplete understanding of regional earthquake and tsunami hazard. In this study, we assess GPS velocities for plate coupling of the Makran subduction zone with specific attention to model resolution and the accretionary prism rheology. We use finite element model-derived Green's functions to invert for the interseismic slip deficit under both elastic and viscoelastic Earth assumptions. We use the model resolution matrix to characterize plate-coupling scenarios that are consistent with the limited spatial resolution afforded by GPS observations. We then forward model the corresponding tsunami responses at major coastal cities within the western Indian Ocean basin. Our plate-coupling results show potential segmentation of the megathrust with varying coupling from west to east, but do not rule out a scenario where the entire length of the megathrust could rupture in a single earthquake. The full subduction zone rupture scenarios suggest that the Makran may be able to produce earthquakes up to M w 9.2. The corresponding tsunami model from the largest earthquake event ( M w 9.2) estimates maximum wave heights reaching 2–5 m at major port cities in the northern Arabian Sea region. Cities on the west coast of India are less affected (1–2 m). Coastlines bounding eastern Africa, and the Strait of Hormuz, are the least affected (<1 m).
The Makran subduction zone (MSZ) of Iran and Pakistan currently accommodates northward subduction of the Arabian oceanic plate beneath continental Eurasia at a rate of ∼3.5 cm yr −1 over the last 3 Ma (Kopp et al . 2000 ; DeMets et al . 2010 ; Smith et al . 2012 ; Fig. 1 ). The MSZ stretches approximately 1000 km from west to east and is bounded by strike-slip fault systems: the right-lateral Minab–Zendan fault to the west and the left-lateral Ornach-Nal and Chaman faults to the east. These two strike-slip systems separate the Makran region from active continent–continent collisions forming the Himalaya and Zagros Mountain ranges. The distance between the active trench and volcanic arc in the MSZ varies from 400 to 600 km, owing to a shallow subduction angle (<5°; Quittmeyer & Jacob 1979 ; Byrne et al . 1992 ; Regard et al . 2010 ; Barnhart et al . 2014 ; Hayes et al . 2018 ). The active convergence between the Arabian and Eurasian plates also produces the world's largest accretionary prism in the MSZ, the Makran accretionary prism, which is divided into a 100–150 km wide submarine portion and a 150–200 km subaerial portion from south to north, respectively (Burg 2018 ). The notable width of the emergent portion of the accretionary prism likely results from shallow angle subduction, exceptionally high incoming sediment thickness (up to 7.5 km) at the trench (Smith et al . 2012 ) and extensive sediment underplating, evidenced by reflection seismic data (Fowler et al . 1985 ; Platt et al . 1985 ; Kopp et al . 2000 ).

(a) Topographic and bathymetric map of the western Indian Ocean region. Orange stars indicate major coastal cities: MC: Muscat; CB: Chah Bahar; GW: Gwadar; PN: Pasni; OM: Ormara; KA: Karachi; ST: Surat; MB: Mumbai; ML: Mangalore; KZ: Kozhikode; KO: Kochi; KC: Kuwait City; DM: Dammam; DH: Doha; AD: Abu Dhabi; DB: Dubai; MO; Mogadishu; DS: Dar es Salaam; MP: Maputo; DN: Durban. (b) Regional seismicity ( M w 4.5+, 1905–2022) and simplified structural map of the Makran subduction zone, modified from Burg ( 2018 ). Three rectangles along the coastal Makran indicate maximum estimated rupture areas for historic earthquake events (Byrne et al . 1992 ). Plate motion of Arabia relative to Eurasia (AR:EU) is shown by the solid arrow (DeMets et al . 2010 ). Structures and images are overlain on SRTM15+ relief model (Tozer et al . 2019 ).
Historically, the MSZ shows relatively low seismicity rates in comparison to other subduction zones in the world (Heidarzadeh et al . 2008 ; Mokhtari et al . 2019 ). However, several significant earthquakes have been reported in the MSZ, some of which were tsunamigenic. According to Byrne et al . ( 1992 ), four large earthquakes ( M w > 7.0) may have ruptured the plate boundary along the eastern part of the MSZ (Fig. 1 ), including the 1765 event that was felt strongly at Karachi in easternmost Makran, two coastal events in 1851 and 1864 affecting the town of Gwadar and the 1945 M w 8.0–8.3 event near Pasni that was followed by a large aftershock in 1947 to the south. The 1945 event is the largest instrumentally recorded earthquake in the subduction zone and is known to have caused a large tsunami, which is possibly the second deadliest tsunami event in the Indian Ocean basin killing over 4000 people (Heck 1947 ). The western section of the Makran, in contrast, has less documented seismic activity than the eastern MSZ and holds no clearly documented record of great subduction zone earthquakes. Only two events are reported to have occurred in the western Makran, in 1008 and 1483, but the latter event likely occurred within the Strait of Hormuz and thus may not be associated with megathrust rupture (Musson 2009 ; Rajendran et al . 2013 ). Additionally, some non-megathrust earthquakes of the Makran were tsunamigenic, such as the 2013 Baluchistan strike-slip earthquake ( M w 7.7), which triggered a landslide offshore Makran and led to a tsunami event (Baptista et al . 2020 ; Heidarzadeh & Satake 2015 ; Hoffmann et al . 2015 ).
Several megacities are located along the rim of the western Indian Ocean (Fig. 1 ). These cities include Mumbai (18+ million), Karachi (16+ million), Surat (6+ million), Dar es Salaam (6+ million), Mogadishu (2+ million) and major cities in the Persian Gulf (such as Dubai, Kuwait City and Doha, each with a population exceeding 2 million). Therefore, tsunamigenic earthquakes produced by the MSZ will potentially lead to high economic losses and human casualties in the western Indian Ocean, making assessment of the earthquake and tsunami potential of the Makran a critical component of regional risk analysis. Several plate-coupling models (i.e. the degree of interseismic strain accumulation) of the Makran have been proposed by previous studies. For example, Frohling & Szeliga ( 2016 ) found that the Makran megathrust is segmented, with a plate coupling of 58 per cent in eastern and western Makran, and a lower coupling ratio (31 per cent) along the central-western portion of the Makran. Lin et al . ( 2015 ) conducted Bayesian inversion of interferometric synthetic aperture radar (InSAR) observations from 2003 to 2010 for plate-coupling model in the eastern MSZ. They showed that high interseismic coupling occurs in the ruptured area of the 1945 M w 8.0–8.3 earthquake (Fig. 1 ), while lower coupling lies on both sides of the epicentral area. The inferred plate-coupling model may imply future occurrence of magnitude 7+ earthquakes, or a multisegment rupture event that would likely exceed magnitude 8 (Lin et al . 2015 ).
These previous plate-coupling studies in the MSZ invoked an elastic half-space mechanical model (Okada 1992 ), where the Earth behaves elastically during the interseismic period. However, in a viscoelastic Earth, the stresses induced by megathrust coupling are relaxed through viscous flow in the mantle during the decades to centuries long interseismic period (Wang et al . 2012 ). Several studies have revealed that the elastic Earth assumption tends to overestimate plate coupling in subduction zone setting as it ignores the effects introduced by viscous flow within the mantle wedge. Li et al . ( 2015 , 2018 ) conducted finite element modelling (FEM) to find that a purely elastic subduction zone model tends to overestimate the true coupling depth in North Chile and Cascadia. Li et al . ( 2020 ) showed that the assumption of a fully relaxed mantle may lead to underestimations of the coupling depth and/or plate coupling when ignoring the interseismic mantle relaxation. Furthermore, besides mantle viscoelasticity, simulations of post-seismic deformation following the 2013 Baluchistan earthquake show that the lower Makran accretionary wedge (12+ km depth) may undergo low-temperature viscoelastic deformation as well (Peterson et al . 2018 ; Cheng et al . 2022 ). Here, we conduct inversions of the GPS velocity data from Frohling & Szeliga ( 2016 ) for megathrust plate coupling of the MSZ using an FEM-derived Green's function under both elastic and viscoelastic Earth assumptions. We utilize the model resolution matrix derived from the Green's function to explore possible plate-coupling scenarios that the GPS observations could resolve. We then generate several megathrust earthquake scenarios based on these plate-coupling models and use them to forward model the corresponding tsunami responses at major coastal cities within the Persian Gulf, Arabian Sea and western Indian Ocean basin. The main goal of this study is to explore the population of subduction zone coupling scenarios, and by extension earthquake and tsunami scenarios, which are consistent with but incompletely resolved by the sparse geodetic observations currently available in the Makran.
2.1. Interseismic plate coupling: inversion and model resolution
Our analysis of coupling in the Makran focuses on modelling the resolution afforded by sparse campaign GPS measurements made prior to the 2013 M w 7.7 Baluchistan earthquake that occurred within the sub-aerial accretionary prism. We first use the fixed Eurasia GPS velocities from Frohling & Szeliga ( 2016 ) to invert for interseismic back-slip rate on the Makran megathrust. The back-slip model (Savage 1983 ) assumes that interseismic velocities or displacements reflect deformation due to coupling between two plates, such that the back-slip rate can be related to the magnitude of a slip deficit accrued on the plate interface that can later be released in an earthquake. Therefore, the slip deficit caused by fault coupling can be modelled as fault slip in the opposite sense to the coseismic slip (back-slip). The plate coupling is then defined as the ratio between back-slip rate during the modelled period, and the plate convergence rate at the trench. 11 out of 19 GPS stations from Frohling & Szeliga ( 2016 ) are used for the back-slip rate inversion (Fig. 1 ). The remaining eight stations lie either to the east or west of the MSZ where deformation is likely influenced by the strike-slip motion of the transverse fault systems. The GPS station coordinates and velocities were estimated using the GAMIT/GLOBK analysis package (Herring et al . 2010a , b ). Station coordinates and velocities were tied to the ITRF2008 global reference frame (Altamimi et al . 2011 ). The GPS velocities were rotated with respect to a fixed Eurasia reference frame using the pole-of-rotation parameters published in Altamimi et al . ( 2012 ).
We conduct the back-slip rate inversion based on a linear viscoelastic Makran accretionary prism model. The inverse problem describes the linear relationship between the back-slip rates on different fault patches and the surface displacement rates (i.e. GPS velocities) in the late stage of the interseismic period, when the post-seismic deformation from the previous large earthquake has diminished, and the surface deformation mainly results from interseismic megathrust loading. Thus, the inverse problem to solve for back-slip rate can be expressed as the linear system:
where G is the matrix of Green's functions relating unit back-slip rate on a fault patch at depth to velocities at a free surface, m is the unsolved back-slip rate vector and d is the data vector that contains the noisy GPS velocities. Following Barnhart & Lohman ( 2010 ), we apply least-squares inversion to solve for m , regularized by a minimum moment operator L , which minimizes slip on any given fault patch irrespective of the behaviour of adjacent patches. The regularized least-squares inversion minimizes the norm of
where L is an identity matrix, and λ is a weighting parameter that adjusts the degree to which the inversion is regularized. This regularization scheme is different from a spatial regularization scheme such as a Laplacian smoothing matrix. Therefore, the inverted slip distribution is not necessarily expected to be ‘smooth’ (i.e. constant spatial gradient between slip asperities). We compared the inversion results using minimum moment and Laplacian regularizations and found that due to the sparse distribution of the GPS stations, the Laplacian regularization introduces unrealistically high back-slip rate asperities downdip of the regional seismogenic zone ( Supporting Information Fig. S1 ). We explore the inversion results based on different λ values ( Supporting Information Fig. S2 ) and choose the best-fit λ value by identifying the corner of the L-curve (Harris & Segall 1987 ).
We construct the G matrix using FEM-derived Green's functions, following Masterlark ( 2003 ). We use the finite element meshing software Coreform-Cubit ( https://coreform.com/ ) to construct a layered viscoelastic 3-D subduction model, which is constrained by geophysical slab geometry (Craig & Copley 2014 ; Hayes et al . 2018 ), and material domains estimated from forward modelling of post-seismic deformation of the 2013 Baluchistan earthquake (Peterson et al . 2018 ; Cheng et al . 2022 ). The mesh geometry consists of four domains (Fig. 2 ): (1) an elastic upper wedge that extends to 12-km depth; (2) a Maxwell viscoelastic lower wedge that extends to a maximum depth of 40 km, which is the Moho depth reported in this region (Maggi et al . 2000 ; Shad Manaman et al . 2011 ; Abdollahi et al . 2018 ); (3) a 30 km thick elastic Arabian oceanic slab with megathrust geometry from the Slab2 model (Craig & Copley 2014 ; Hayes et al . 2018 ); and (4) a Maxwell viscoelastic oceanic and continental mantle at depths. To increase computational efficiency, instead of using the full megathrust mesh (10 350 patches in total), we discretize the plate interface into nonoverlapping patches with ∼30 km spacing (470 patches in total, Supporting Information Fig. S3 ). Each patch consists of sub-patches with a size on the scale of ∼15 km. We use open-source FEM software PyLith to assign material properties and derive the Green's functions (Aagaard et al . 2013 ). The materials properties for each domain are summarized in Supporting Information Table S1 . We set free displacement boundary condition at the ground surface. We fixed the displacements to be zero in the horizontal directions along the northern/southern boundaries and set the eastern/western boundaries to be free. We then prescribe unit back-slip rate (1 m yr −1 ) along strike and dip directions on one fault patch while keeping the slip rate zero on the remaining patches, and drive the simulation for 200 yr. The 200-yr simulation period is considerably longer than the Maxwell relaxation time of the viscoelastic materials (8 yr for the lower wedge, 50 yr for the mantle), which is an indication that the viscoelastic relaxation has stabilized and the simulation has reached the late stage of the interseismic period (Hu et al . 2004 ; Li et al . 2015 ). Additionally, we ignore any bias in the GPS velocity data that may be introduced by the prolonged post-seismic deformation of the 1945 Makran earthquake. For large (M > 8.5) subduction zone earthquakes, Li & Chen ( 2023 ) estimated the post-seismic phase to be in the range of 0.2–0.4 of their recurrence intervals. In the Makran region, the average recurrence time for magnitude 8 + earthquake is about 100–250 yr (Page et al . 1979 ). Therefore, although the post-seismic relaxation of the 1945 event may contribute to the GPS observations, we posit that this effect is small and the observed GPS velocities result solely from interseismic strain accumulation on the megathrust. We repeat the simulation process for every fault patch and calculate the surface velocities at the last time step, which are then flattened into column vectors and appended to the G matrix. For the elastic model, we calculate the G matrix following the same procedure as the viscoelastic model, but setting the material properties of the lower wedge and mantle domains to be elastic ( Supporting Information Table S1 ).

Makran subduction zone geometry and finite element mesh. Green domain: upper wedge. Blue domain: oceanic slab. Pink domain: lower wedge. Orange domain: oceanic and continental mantle.
After obtaining G , we solve for the back-slip rate vector m (eq. 3 ). We fix the rake to be 110°, a slip direction that both best explains the GPS observations based on a grid search procedure ( Supporting Information Fig. S4 ) and the relative plate motion resolved onto the subduction zone interface. We force the solution to be positive (slip along rake) and set the maximum allowable back-slip rate on each patch to be the plate convergence rate at the trench (fixed Eurasia reference frame, Altamimi et al . 2012 ). The regularized least squares solution can be written as
where m est is the estimated back-slip rate model. For each fault patch, we divide the interseismic back-slip rate model by the plate convergence rate at the trench to calculate the plate coupling. We translate the back-slip rate of each fault patch into the locking ratio by dividing the back-slip rate by the plate motion rate expected at the depth of each fault patch (Altamimi et al . 2012 ). We propagate the noise covariance structure of the data through the inversion and eq. ( 3 ) becomes:
where Ch is the inverse Cholesky factorization of the data covariance structure (Harris & Segall 1987 ).
2.2. Interseismic plate-coupling inversion: results
Fig. 3 shows the plate-coupling inversion results, as well as the model resolution matrices for elastic and viscoelastic models. The model resolution matrix ( R m ) is defined as
and reflects the degree of spatial averaging the inverted model has over the underlying true model (Menke 2018 ). To a first order, despite differences in the deeper part of the fault (below 20-km depth), both elastic and viscoelastic models exhibit similar magnitude and spatial distribution of plate coupling in the shallow portion (Figs 3a and c ), which likely results from the lack of GPS data in the region to capture the viscoelastic effects. According to Li et al . ( 2015 , 2018 ), viscoelastic subduction zone behaviours affect interseismic deformation over longer spatial wavelength than simple elastic half-space models and thus dominate in the GPS signals further inland from the trench. Therefore, the lack of GPS stations in inland Makran may cause the inversion results from the viscoelastic model to be indistinguishable from the elastic model.

Inverted interseismic plate-coupling models and the diagonal components of the model resolution matrices of the Makran megathrust resulting from (a and b) viscoelastic model and (c and d) elastic model. (e) L-curve between the fit to data (data norm) and the model size (model norm), and the selected corner ( λ ) for each coupling model. White arrows: GPS velocity data from Frohling & Szeliga ( 2016 ); pink arrows: forward-predicted velocities from the inversion; black solid lines: contours of the plate interface marked by depth in kilometer; black dash lines: boundaries between different fault segments; dark green lines: coastlines.
For both models, five regions with relatively high magnitudes of coupling are observed along strike at about 10-km depth. These coupled patches correspond to high values in the model resolution matrix (Figs 3b and d ), indicating that the plate coupling is high where coupling is well-resolved by the data. We observe along-strike variation in the coupling ratio over these five regions. The westernmost and two easternmost regions exhibit higher coupling with respect to the two central regions, indicating likely segmentation of the Makran megathrust with varying coupling from west to east (Fig. 3 ). This pattern is consistent with previous coupling estimates of the MSZ that suggested that the western and eastern segments have higher coupling (∼58 per cent), while the central segment is weakly coupled (∼31 per cent, Frohling & Szeliga 2016 ). Distributed high coupling extends below 20-km depth in our model. However, we find these estimates to likely be an artifact of the distribution of surface observations given that the model resolution matrix exhibits low values over the corresponding fault areas. Moreover, historic reviews of Makran seismicity suggest that, with the exception of intermediate depth normal faulting earthquakes, nearly all recorded earthquakes occur at depths shallower than 20 km (Jackson & McKenzie 1984 ; Byrne et al . 1992 ), further suggesting that this downdip ‘smear’ of high coupling is likely unreal, but rather an artifact resulting from the lack of GPS observations in the vicinity of the fault areas.
3.1. Model resolution
After undertaking inversions of the GPS observations for back slip rates, we explore the potential characteristics of back slip that cannot be resolved by the sparsely located geodetic measurements. An inverse model ( m est ) of back-slip rate (or fault slip, more generally) only reveals what aspects of the true, underlying back-slip rate distribution ( m true ) can be resolved given the location and quality of data, the imposed fault model (location, discretization), the imposed Earth structure, inversion regularization, and other sources of epistemic uncertainty. The resolution capabilities of an inverse problem need to be assessed in order to characterize the full range of potential underlying, true scenarios. Model resolution matrix ( R m ) gives what a true model looks like with the available data collection strategy.
We use the model resolution matrix to interrogate this relationship between our inverted back-slip rate distributions ( m est ) and the unknown true back-slip rate distribution ( m true ) in order to define a suite of possible true back-slip rate distributions. The relationship between the true underlying back-slip rate model ( m true ) and the inverted, estimated back-slip rate model ( m est ) is
Using eq. ( 6 ) and our inverted back-slip rate model ( m est ), we can then multiply any conjured, theoretical coupling scenario by the model resolution matrix, and then compare the output to our inverted coupling models and determine if the theoretical coupling scenario is possible given the model resolution. Fig. 4 illustrate the relationship between R m , m est and m true .

A general illustration of relationship between true back-slip rate ( m true ), model resolution matrix ( R m ) and inverted back-slip rate ( m est ) in back-slip rate inversion on a subduction interface. The model resolution matrix in this figure is constructed based on synthetic GPS velocities that are equally spaced on both land and seafloor.
To construct different scenarios of m true , we first divide the megathrust into three segments as previously described by varying coupling ratio (Fig. 3 ). We create in total of six back-slip rate scenarios on either one or multiple segments: east-only (E), central-only (C), west-only (W), east-central (EC), west-central (WC), and whole-fault (WCE). For each scenario, we assume that the interseismic back-slip rate extends to the trench and assign a series of combinations of downdip width and back-slip rate ( m true ). Since there is little information on the downdip limit of plate coupling, and the model resolution is low at depth (Fig. 3 ), we keep the downdip widths as narrow as possible. Therefore, the downdip widths estimated in this study reflect the shallowest plate-coupling limits that can be constrained by the GPS data. We then multiply m true by the R m from our viscoelastic inversion to calculate how the back-slip rate distribution would be resolved after the inversion ( m rsv ). In a trial-and-error manner, we calculate the root-mean-square error (RMSE) between m rsv and the inversion result m est to access whether the prescribed back-slip rate scenarios, under given model resolution, can produce the same back-slip rate pattern as the GPS inversion. Additionally, we conduct visual inspections to assess the fit between m rsv and m est within the ruptured segment(s) to ensure that actual back-slip rates are being fit, rather than noises (i.e. the downdip artifact shown in Fig. 3 ).
After obtaining different scenarios of m true , we divide them by the plate convergence rate at the trench to calculate the corresponding plate-coupling models. For each scenario, we use an earthquake scaling relationship to estimate an earthquake moment magnitude. This moment magnitude corresponds to a possible future earthquake where the entire coupled segment(s) is ruptured. We use the scaling relationship between fault area and magnitude described in Allen & Hayes ( 2017 ), which is developed based on earthquakes in subduction zone and offshore environments:
where M w is the moment magnitude, FA is fault area in square kilometres, and a and b are regression coefficients that are set to be −3.63 and 0.96, respectively. After solving for the seismic moment magnitude, we calculate the coseismic uniform slip magnitude following
where m o is the seismic moment release, D is the uniform fault slip, A is the summation of areas of all fault patches above the downdip limit and μ is shear modulus fixed at 40 GPa.
We further investigate scenarios where interseismic back-slip rate does not extend to the trench, but starts at certain depth downdip. To do this, for each scenario, we test combinations of back-slip rate and upper coupling depth limit while keeping the lower limit the same as before, and look for the best-fitting back-slip rate case, whose corresponding plate-coupling model and future earthquake slip magnitude are calculated following the same procedure as before.
3.2. Coupling-based earthquake scenarios: results
Figs 5 and 6 show the best-fitting plate-coupling models for all six back-slip rate scenarios under trench slip and blind slip conditions, respectively. Supporting Information Table S2 summarizes the source parameters for each back-slip rate scenario and the corresponding future earthquake. We observe several characteristics for different plate-coupling cases. In general, back-slip rate scenarios where locking extends to the trench produce trench-rupturing earthquakes of higher magnitudes than their blind-rupturing counterparts. For example, if the interseismic strain accumulation is released through an earthquake on the east-central segments, the magnitude of the earthquake is 9.0 and 8.7, under trench rupture and blind rupture conditions, respectively. We observe that single-segment coupling models, after being multiplied by the model resolution matrix, yield better fits to the GPS-inverted coupling model than the multisegment models within the corresponding segments. For instance, the east-only coupling model shows lower residuals than the whole-fault case within the eastern segment. Single-segment ruptures also produce lower magnitude than multisegment ruptures. Our results suggest that the Makran megathrust may be able to produce earthquakes up to M w 8.7 for single-segment rupture, and M w 9.2 for multisegment rupture. Additionally, the plate-coupling distributions for the east-only, central-only, and west-only back-slip rate cases indicate higher coupling in the eastern (50–60 per cent) and western (60–70 per cent) segments than the central segment (30–35 per cent, Fig. 7 ), which is again consistent with the results from Frohling & Szeliga ( 2016 ). Finally, when comparing the misfit between the GPS data and the forward-predicted velocities, we find that the prescribed back-slip scenarios yield good fit to data at stations close to the coupled patches. However, the westmost station exhibit large misfit exceeding the GPS uncertainty. This could result either from the spatially variable coupling on the western section or bias in the GPS velocity associated with the right-lateral motion on the nearby Minab–Zendan fault system.

Best-fitting single- and multisegment plate-coupling scenarios and the corresponding earthquake magnitudes under the trench slip condition. The first column shows the assigned plate coupling corresponding to each back-slip rate case. The second column shows the resolvable coupling (multiply the first column by the corresponding model resolution matrix). The third column shows the residual between the resolvable coupling and the inverted coupling shown in Fig. 3 . Each moment magnitude corresponds to an earthquake for the given coupling scenario assuming that the interseismic strain accumulation is fully released. White and pink arrows in the first column correspond to GPS velocity data and forward-predicted velocities, respectively.

Best-fitting single- and multisegment plate-coupling scenarios and the corresponding earthquake magnitudes under the blind slip condition.

The preferred coupling model of the Makran megathrust and its resolvability by the GPS data.
4.1. Tsunami simulations in the western Indian Ocean: methods
For each earthquake slip scenario, we calculate co-seismic ground/seafloor vertical displacements using Pylith . We use the same subduction zone geometry as before with the lower wedge exhibiting Maxwell rheology. We prescribe the slip distributions onto the megathrust and simulate the co-seismic surface static vertical displacements. Supporting Information Fig. S5 shows the resulting ground/seafloor uplift patterns. We then input these surface vertical displacements as initial condition for tsunami simulations using the open-source tsunami modelling code GeoClaw ( http://www.clawpack.org/geoclaw ). We ignore the time-dependent co-seismic slip evolution and assume that the earthquakes instantly deform the seafloor, as earthquake rupture propagates much faster than tsunami wave. We use the SRTM15 + relief model resampled at 1-arcminute pixel resolution for both topography and bathymetry inputs (Tozer et al . 2019 ). GeoClaw solves 2-D shallow water equations with finite volume technique (LeVeque et al . 2011 ), and employs adaptive mesh refinement (AMR) which automatically refines regions of higher tsunami complexity to finer mesh size according to user inputs. We use two levels of grid refinement with the coarsest set at 2-arcminute and the finest at 1-arcminute for the topography/bathymetry data set. For each earthquake scenario, we drive the tsunami simulation for 12 hours following the rupture. We then output time-series of estimated tsunami amplitudes (ETA) at 20 virtual tide gauges outside major port cities along coastlines bounding the Oman sea, the Strait of Hormuz, and southwestern Indian Ocean basin. Since the gauge points are located at different water depths, we apply Green's law to rescale the ETA to a common reference depth of 1 m following (Small & Melgar 2021 ).
4.2. Tsunami simulations in the western Indian Ocean: results
Fig. 8 illustrates the maximum tsunami wave heights calculated at the 20 tide gauge points for all six rupture scenarios under trench rupture and blind rupture conditions, respectively. Supporting Information Tables S3 and S4 summarize the maximum and peak-to-peak tsunami wave height results. In general, we observe spatial variation in the maximum and peak-to-peak tsunami wave heights in different regions of the western Indian Ocean basin. Fig. 9 shows the modelled ETA time-series resulting from the M w 9.2 whole-fault trench-rupturing earthquake, and Fig. 10 shows the corresponding tsunami snapshots. We group the gauge points into four spatial domains with variable maximum wave height ranges: the northern Arabian Sea region, the west coast of India, eastern Africa, and the Strait of Hormuz. Following the M w 9.2 rupture, coastal cities in the northern Arabian Sea region, including Muscat, Chah Bahar, Gwadar, Pasni, and Ormara, and Karachi, experience the most tsunami hazard with the maximum wave height reaching 2-5 m and peak-to-peak wave height reaching 4-9 m ( Supporting Information Tables S3 and S4 ). Cities on the west coast of India (i.e. Surat, Mumbai, Mangalore, Kozhikode, and Kochi) are less affected, with wave height reaching 1–1.5 m (peak-to-peak: 1-3 m). Coastal cities bounding eastern Africa (i.e. Mogadishu, Dar es Salaam, Maputo, and Durban), and the Strait of Hormuz (i.e. Kuwait City, Dammam, Doha, Abu Dhabi, Dubai), are the least affected, with the incoming maximum and peak-to-peak wave height less than 1 m.

Maximum tsunami wave heights at 20 synthetic gauge points (cyan circles) following single- and multisegment trench rupturing (red) and blind (green) earthquakes.

Modelled tsunami wave height time-series at gauge points offshore major coastal cities in the western Indian Ocean region following the M w 9.2 whole-fault trench-rupturing earthquake.

Modelled tsunami wave propagation after the M w 9.2 whole-fault trench-rupturing earthquake. Solid circles indicate gauge point locations offshore major coastal cities.
5.1. Seismic potential of the Makran megathrust
Our preferred plate-coupling model (Fig. 7 ) suggests that coupling varies along the strike of the subduction zone. We divide the MSZ into three regions based on the average coupling ratio, which are spatially similar to the three segments defined by Frohling & Szeliga ( 2016 ). The eastern segment, spanning from west of Pasni, Pakistan (63.2°E) to the eastern end of the domain (66.2°E), exhibits approximately 50 per cent coupling. This region hosted the 1945 M w 8.0–8.3 megathrust earthquake (Byrne et al . 1992 ). The central segment spans from west of Chah Bahar, Iran (60°E) to the west of Pasni (63.2°E) and shows a reduced plate coupling of ∼30 per cent. Historically, this section of the fault has experienced few major earthquakes, with only two coastal events recorded in 1851 and 1864 (Fig. 1 ). Lastly, the western segment, from west of Chah Bahar (60°E) to the western end of the domain (57.2°E), is approximately 60 per cent coupled. However, the coupling of this segment is not well-resolved when compared to the other two segments, as the back-slip rate inversion is noisy downdip of the regional seismogenic zone. This likely results from low GPS station coverage in the region, or neglecting the stress partitioning contributed by the Minab–Zendan fault system to the west.
Segmentation of the megathrust implies variation in degree of earthquake hazard potential along the MSZ. Following the asperity model of Lay & Kanamori ( 1981 ), strong plate coupling corresponds to larger asperity size and is more likely to produce larger events, whereas weak coupling indicates smaller asperity size and likely results in smaller events. Higher plate coupling is also an indication of faster interseismic strain accumulation on the megathrust, where subduction zone earthquakes yield shorter recurrence intervals than those of the same magnitudes but produced on weakly coupled plate interfaces. We calculate the recurrence intervals for all earthquake scenarios by dividing the uniform co-seismic slip magnitudes by the back-slip rates ( Supporting Information Table S2 ). The results show that, under trench-rupture condition, the recurrence intervals for an M w 8.7 earthquake that ruptures the eastern, central and western segments are approximately 373, 664 and 381 yr, respectively, with unknown uncertainties. This change in recurrence interval correlates to the variation in plate coupling from east to west, where the eastern and western segments are stronger coupled than the central segment, resulting in shorter recurrence intervals for earthquakes of a given magnitude. Therefore, the eastern and western Makran are likely able to host larger, more frequent earthquake events than the central Makran.
5.2. Tsunami hazard potential in the western Indian Ocean basin
Our coupling-based tsunami simulation indicates variations in expected tsunami wave height along the coastline bounding the western Indian Ocean basin. In the largest earthquake scenario where the entire megathrust is rupture to the trench, the northern Arabian Sea region experiences the most severe tsunami hazard with the maximum wave height reaching several coastal cities at 2–5 m within the first hour after the earthquake ( Supporting Information Table S3 , Figs 9 and 10 ). The peak tsunami wave reduces to 1–1.5 m height and arrives at the west coast of India after 4 hr. The east coast of Africa and the Strait of Hormuz are the least affected by the M w 9.2 earthquake in Makran, where the incoming maximum wave height is less than 1 m. For all 20 virtual gauge points, we define and calculate a simple index, termed the tsunami hazard score (THS), to assess the tsunami hazard potential at each location considering all 12 possible earthquake scenarios in Makran. The THS is defined as the weighted sum of number of events exceeding given maximum tsunami wave height thresholds at a gauge point for all 12 earthquake events, normalized between 0 and 100:
where N i is the number of events producing maximum wave height larger than W i metres (from 1 to 4.5 m, 0.5-m increment). Supporting Information Table S5 shows the THS summary for all 20 coastal cities. Again, cities in the northern Arabian Sea region yield the highest THS (i.e. having the highest tsunami potential from earthquakes in Makran). Coastal cities in western India yield lower THS values, and cities in eastern Africa and the Strait of Hormuz have the lowest score. Specifically, Muscat, Char Bhar and Gwadar are the three cities with highest THS values. Muscat is the capital and the most populated city in Oman. Char Bhar and Gwadar are the two of the fastest-growing commercial port cities in the region. Tsunami events of maximum wave height of 3–5 m at these locations can cause severe destruction to coastal infrastructures and port facilities, and result in significant human casualties and economic losses.
Tsunami height records and observations in the Makran are incomplete. The 1945 M w 8.0–8.3 earthquake is the first instrumentally recorded event, and the only event for which observations of tsunami wave heights along different coasts are available (Heidarzadeh et al . 2008 ). According to Berninghausen ( 1966 ), the wave heights were at about 12–15 m at Pasni and Ormara, and 1.4 m at Karachi. Ambraseys & Melville ( 2005 ) reported the wave height to be about 4–5 m in Pasni and 1.5 m in Karachi. Adams et al . ( 2018 ) detided a Karachi tide-gauge record and reported the water level to be ∼0.5 m above ambient tide. Page et al . ( 1979 ) reported a wave height reaching 7–10 m along several parts of the Makran coast. Since the 1945 earthquake ruptured in the eastern segment of the megathrust, we compare our predicted maximum tsunami wave heights from the east-only trench-rupture scenario to the field observations. Our model suggests a maximum wave height of 1.28 m in Karachi, which is in reasonable agreement with the field observations. However, the wave height predictions at Pasni and Ormara are much lower (1.15 and 1.32 m, respectively) than the 12–15 m reported by previous studies. This discrepancy between modelling results and field observations is consistent with other numerical models of this earthquake (Heidarzadeh et al . 2008 ; Heidarzadeh & Satake 2015 ; Qiu et al . 2022 ). Heidarzadeh et al . ( 2008 ) proposed three possible explanations for this discrepancy: (1) the reports of 12–15 m wave heights were incorrect and an exaggeration; (2) the large wave heights resulted from submarine landslides triggered by the earthquake; and (3) the elevated wave heights could be produced by large displacements on splay faults near the trench, which are not considered in our models. Qiu et al . ( 2022 ) conducted tsunami simulations of the 1945 megathrust rupture with additional displacements on splay faults. Their results indicate amplification of tsunami wave height when considering the presence of splay faults.
Although we cannot currently resolve megathrust coupling in the MSZ with the resolution that can be accomplished in other global subduction zones, this study serves to provide a synopsis of what is possible given our current data availability. By developing coupling scenarios that are consistent with the model resolution of our inverse problem, we are able to hypothesize several important characteristics of coupling in the MSZ and how future great earthquakes may play out:
The plate-coupling distribution that can be directly inferred from available GPS observations is insufficient to uniquely explain the history of great earthquakes in the Makran; thus, there is significant coupling that cannot be directly resolved with current observations.
The megathrust of the MSZ is likely segmented with variable plate coupling along strike, in agreement with previous studies. The eastern and western segments correspond to approximately 50 and 60 per cent coupling, respectively. The central segment exhibits lower coupling (about 30 per cent) than the other two segments.
Our investigation suggests that, based on the inverted plate coupling, the Makran megathrust may be able to produce earthquakes up to M w 8.7 for single-segment rupture, and M w 9.2 for multisegment rupture.
Population centres along the western Indian Ocean basin may be exposed to tsunami hazard as a result of Makran great earthquakes. The northern Arabian Sea region experiences the most severe tsunami hazard with the maximum wave height reaching several coastal cities at 2–5 m. The west coast of India is less affected with wave height reduced to 1–1.5 m. The east coast of Africa and the Strait of Hormuz are the least affected, for which the incoming wave height is less than 1 m.
This work was supported by NSF Award EAR 1917500. High-performance computational resources were provided by the University of Iowa, Iowa City. Any use of trade, firm or product names is for descriptive purposes only and does not imply endorsement by the U.S. Government.
The GPS velocity data used to invert for interseismic plate coupling were imported from Frohling & Szeliga ( 2016 ). Several figures were generated using the Generic Mapping Tool (Wessel et al . 2013 ).
Aagaard B.T. , Knepley M.G. , Williams C.A. 2013 . A domain decomposition approach to implementing fault slip in finite-element models of quasi-static and dynamic crustal deformation . J. geophys. Res. , 118 ( 6 ), 3059 – 3079 . 10.1002/jgrb.50217
Google Scholar
Abdollahi S. , Ardestani V.E. , Zeyen H. , Shomali Z.H. 2018 . Crustal and upper mantle structures of Makran subduction zone, SE Iran by combined surface wave velocity analysis and gravity modeling . Tectonophysics , 747–748 , 191 – 210 . 10.1016/j.tecto.2018.10.005
Adams L.M. , Atwater B.F. , Hasan H. 2018 . Karachi tides during the 1945 Makran tsunami . Geosci. Lett. , 5 ( 1 ), 25 . doi: 10.1186/s40562-018-0121-z . 10.1186/s40562-018-0121-z
Allen T.I. , Hayes G.P. 2017 . Alternative rupture-scaling relationships for subduction interface and other offshore environments . Bull. seism. Soc. Am. , 107 ( 3 ), 1240 – 1253 . 10.1785/0120160255
Altamimi Z. , Collilieux X. , Métivier L. 2011 . ITRF2008: an improved solution of the international terrestrial reference frame . J. Geod. , 85 ( 8 ), 457 – 473 . 10.1007/s00190-011-0444-4
Altamimi Z. , Métivier L. , Collilieux X. 2012 . ITRF2008 plate motion model . J. geophys. Res. , 117 ( B7 ). doi: 10.1029/2011JB008930 . 10.1029/2011JB008930
Ambraseys N.N. , Melville C.P. 2005 . A History of Persian Earthquakes . Cambridge Univ. Press .
Google Preview
Baptista M.A. , Miranda J.M. , Omira R. , El Hussain I. 2020 . Study of the 24 September 2013 Oman Sea tsunami using linear shallow water inversion . Arabian Journal of Geosciences , 13 ( 14 ), 606 . doi: 10.1007/s12517-020-05632-z . 10.1007/s12517-020-05632-z
Barnhart W.D. , Hayes G.P. , Samsonov S.V. , Fielding E.J. , Seidman L.E. 2014 . Breaking the oceanic lithosphere of a subducting slab: the 2013 Khash, Iran earthquake . Geophys. Res. Lett. , 41 ( 1 ), 32 – 36 . 10.1002/2013GL058096
Barnhart W.D. , Lohman R.B. 2010 . Automated fault model discretization for inversions for coseismic slip distributions . J. geophys. Res. , 115 ( B10 ). doi: 10.1029/2010JB007545 . 10.1029/2010JB007545
Berninghausen W..H. 1966 . Tsunamis and seismic seiches reported from regions adjacent to the Indian Ocean . Bull. seism. Soc. Am. , 56 ( 1 ), 69 – 74 . 10.1785/BSSA0560010069
Burg J.-P. 2018 . Geology of the onshore Makran accretionary wedge: synthesis and tectonic interpretation . Earth Sci. Rev. , 185 , 1210 – 1231 . 10.1016/j.earscirev.2018.09.011
Byrne D.E. , Sykes L.R. , Davis D.M. 1992 . Great thrust earthquakes and aseismic slip along the plate boundary of the Makran Subduction Zone . J. geophys. Res. , 97 ( B1 ), 449 – 478 . 10.1029/91JB02165
Cheng G. , Barnhart W.D. , Li S. 2022 . Power-law viscoelastic flow of the lower accretionary prism in the Makran subduction zone following the 2013 Baluchistan earthquake . J. geophys. Res. , 127 ( 11 ), e2022JB024493 . doi: 10.1029/2022JB024493 . 10.1029/2022JB024493
Craig T.J. , Copley A. 2014 . An explanation for the age independence of oceanic elastic thickness estimates from flexural profiles at subduction zones, and implications for continental rheology . Earth planet. Sci. Lett. , 392 , 207 – 216 . 10.1016/j.epsl.2014.02.027
DeMets C. , Gordon R.G. , Argus D.F. 2010 . Geologically current plate motions . Geophys. J. Int. , 181 ( 1 ), 1 – 80 . 10.1111/j.1365-246X.2009.04491.x
Fowler S.R. , White R.S. , Louden K.E. 1985 . Sediment dewatering in the Makran accretionary prism . Earth planet. Sci. Lett. , 75 ( 4 ), 427 – 438 . 10.1016/0012-821X(85)90186-4
Frohling E. , Szeliga W. 2016 . GPS constraints on interplate locking within the Makran subduction zone . Geophys. J. Int. , 205 ( 1 ), 67 – 76 . 10.1093/gji/ggw001
Harris R.A. , Segall P. 1987 . Detection of a locked zone at depth on the Parkfield, California, segment of the San Andreas Fault . J. geophys. Res. , 92 ( B8 ), 7945 – 7962 . 10.1029/JB092iB08p07945
Hayes G.P. , Moore G.L. , Portner D.E. , Hearne M. , Flamme H. , Furtney M. , Smoczyk G.M. 2018 . Slab2, a comprehensive subduction zone geometry model . Science , 362 ( 6410 ), 58 – 61 . 10.1126/science.aat4723
Heck N.H. 1947 . List of seismic sea waves* . Bull. seism. Soc. Am. , 37 ( 4 ), 269 – 286 . 10.1785/BSSA0370040269
Heidarzadeh M. , Pirooz M.D. , Zaker N.H. , Yalciner A.C. , Mokhtari M. , Esmaeily A. 2008 . Historical tsunami in the Makran subduction zone off the southern coasts of Iran and Pakistan and results of numerical modeling . Ocean Eng. , 35 ( 8 ), 774 – 786 . 10.1016/j.oceaneng.2008.01.017
Heidarzadeh M. , Satake K. 2015 . New insights into the source of the Makran tsunami of 27 November 1945 from tsunami waveforms and coastal deformation data . Pure appl. Geophys. , 172 ( 3 ), 621 – 640 . 10.1007/s00024-014-0948-y
Herring T.A. , King R.W. , McClusky S.C. 2010a . GAMIT reference manual, GPS analysis at MIT release 10.4 . Tech. Rep., Dept. Earth Atmos. Planet. Sci. Mass. Inst. Technol., Cambridge, MA .
Herring T.A. , King R.W. , McClusky S.C. 2010b . GLOBK: global Kalman filter VLBI and GPS analysis program, release 10.4 . Tech. Rep., Dept. Earth Atmos. Planet. Sci. Mass. Inst. Technol., Cambridge, MA .
Hoffmann G. , Grützner C. , Reicherter K. , Preusser F. 2015 . Geo-archaeological evidence for a Holocene extreme flooding event within the Arabian Sea (Ras al Hadd, Oman) . Quat. Sci. Rev. , 113 , 123 – 133 . 10.1016/j.quascirev.2014.09.033
Hu Y. , Wang K. , He J. , Klotz J. , Khazaradze G. 2004 . Three-dimensional viscoelastic finite element model for postseismic deformation of the great 1960 Chile earthquake . J. geophys. Res. , 109 ( B12 ). doi: 10.1029/2004JB003163 . 10.1029/2004JB003163
Jackson J. , McKenzie D. 1984 . Active tectonics of the Alpine–Himalayan Belt between western Turkey and Pakistan . Geophys. J. R. Astron. Soc. , 77 ( 1 ), 185 – 264 . 10.1111/j.1365-246X.1984.tb01931.x
Kopp C. , Fruehn J. , Flueh E.R. , Reichert C. , Kukowski N. , Bialas J. , Klaeschen D. 2000 . Structure of the Makran subduction zone from wide-angle and reflection seismic data . Tectonophysics , 329 ( 1 ), 171 – 191 . 10.1016/S0040-1951(00)00195-5
Lay T. , Kanamori H. 1981 . An asperity model of large earthquake sequences , in Earthquake Prediction , pp. 579 – 592 ., American Geophysical Union (AGU) . doi: 10.1029/ME004p0579 . 10.1029/ME004p0579
LeVeque R.J. , George D.L. , Berger M.J. 2011 . Tsunami modelling with adaptively refined finite volume methods* . Acta Numer. , 20 , 211 – 289 . 10.1017/S0962492911000043
Li S. , Chen L. 2023 . How long can the postseismic and interseismic phases of great subduction earthquake sustain? Toward an integrated earthquake-cycle perspective . Geophys. Res. Lett. , 50 ( 11 ), e2023GL103976 . doi: 10.1029/2023GL103976 . 10.1029/2023GL103976
Li S. , Fukuda J. , Oncken O. 2020 . Geodetic evidence of time-dependent viscoelastic interseismic deformation driven by megathrust locking in the southwest Japan subduction zone . Geophys. Res. Lett. , 47 ( 4 ), e2019GL085551 . doi: 10.1029/2019GL085551 . 10.1029/2019GL085551
Li S. , Moreno M. , Bedford J. , Rosenau M. , Oncken O. 2015 . Revisiting viscoelastic effects on interseismic deformation and locking degree: a case study of the Peru–North Chile subduction zone . J. geophys. Res. , 120 ( 6 ), 4522 – 4538 . 10.1002/2015JB011903
Li S. , Wang K. , Wang Y. , Jiang Y. , Dosso S.E. 2018 . Geodetically inferred locking state of the Cascadia megathrust based on a viscoelastic earth model . J. geophys. Res. , 123 ( 9 ), 8056 – 8072 . 10.1029/2018JB015620
Lin Y.N. , Jolivet R. , Simons M. , Agram P.S. , Martens H.R. , Li Z. , Lodi S.H. 2015 . High interseismic coupling in the Eastern Makran (Pakistan) subduction zone . Earth planet. Sci. Lett. , 420 , 116 – 126 . 10.1016/j.epsl.2015.03.037
Maggi A. , Jackson J.A. , Priestley K. , Baker C. 2000 . A re-assessment of focal depth distributions in southern Iran, the Tien Shan and northern India: do earthquakes really occur in the continental mantle? . Geophys. J. Int. , 143 ( 3 ), 629 – 661 . 10.1046/j.1365-246X.2000.00254.x
Masterlark T. 2003 . Finite element model predictions of static deformation from dislocation sources in a subduction zone: sensitivities to homogeneous, isotropic, Poisson-solid, and half-space assumptions . J. geophys. Res. , 108 ( B11 ). doi: 10.1029/2002JB002296 . 10.1029/2002JB002296
Menke W. 2018 . Geophysical Data Analysis: Discrete Inverse Theory . Academic Press .
Mokhtari M. , Ala Amjadi A. , Mahshadnia L. , Rafizadeh M. 2019 . A review of the seismotectonics of the Makran subduction zone as a baseline for tsunami hazard assessments . Geosci. Lett. , 6 ( 1 ), 13 . doi: 10.1186/s40562-019-0143-1 . 10.1186/s40562-019-0143-1
Musson R.M.W. 2009 . Subduction in the Western Makran: the historian's contribution . J. Geol. Soc. , 166 ( 3 ), 387 – 391 . 10.1144/0016-76492008-119
Okada Y. 1992 . Internal deformation due to shear and tensile faults in a half-space . Bull. seism. Soc. Am. , 82 ( 2 ), 1018 – 1040 . 10.1785/BSSA0820021018
Page W.D. , Alt J.N. , Cluff L.S. , Plafker G. 1979 . Evidence for the recurrence of large-magnitude earthquakes along the Makran coast of Iran and Pakistan . Tectonophysics , 52 ( 1 ), 533 – 547 . 10.1016/0040-1951(79)90269-5
Peterson K.E. , Barnhart W.D. , Li S. 2018 . Viscous accretionary prisms: viscoelastic relaxation of the Makran accretionary prism following the . 2013 Baluchistan, Pakistan earthquake . J. geophys. Res. , 123 ( 11 ), 10 107 – 10 123 . 10.1029/2018JB016057
Platt J.P. , Leggett J.K. , Young J. , Raza H. , Alam S. 1985 . Large-scale sediment underplating in the Makran accretionary prism, southwest Pakistan . Geology , 13 ( 7 ), 507 – 511 . 10.1130/0091-7613(1985)13<507:LSUITM>2.0.CO;2
Qiu Q. , Zhou Z. , Lin J. , Zhang F. , Chen Z. , Yang X. 2022 . Great earthquake and tsunami potential in the eastern Makran subduction zone: new insights from geodetic and structural constraints . Tectonophysics , 837 , 229462 . 10.1016/j.tecto.2022.229462
Quittmeyer R.C. , Jacob K.H. 1979 . Historical and modern seismicity of Pakistan, Afghanistan, northwestern India, and southeastern Iran . Bull. seism. Soc. Am. , 69 ( 3 ), 773 – 823 . 10.1785/BSSA0690030773
Rajendran C.P. , Rajendran K. , Shah-hosseini M. , Beni A.N. , Nautiyal C.M. , Andrews R. 2013 . The hazard potential of the western segment of the Makran subduction zone, northern Arabian Sea . Nat. Hazards , 65 ( 1 ), 219 – 239 . 10.1007/s11069-012-0355-6
Regard V. et al. 2010 . The transition between Makran subduction and the Zagros collision: recent advances in its structure and active deformation . Geol. Soc. London Spec. Publ. , 330 ( 1 ), 43 – 64 .
Savage J.C. 1983 . A dislocation model of strain accumulation and release at a subduction zone . J. geophys. Res. , 88 ( B6 ), 4984 – 4996 . 10.1029/JB088iB06p04984
Shad Manaman N. , Shomali H. , Koyi H. 2011 . New constraints on upper-mantle S-velocity structure and crustal thickness of the Iranian plateau using partitioned waveform inversion . Geophys. J. Int. , 184 ( 1 ), 247 – 267 . 10.1111/j.1365-246X.2010.04822.x
Small D.T. , Melgar D. 2021 . Geodetic coupling models as constraints on stochastic earthquake ruptures: an example application to PTHA in Cascadia . J. geophys. Res. , 126 ( 7 ), e2020JB021149 . doi: 10.1029/2020JB021149 . 10.1029/2020JB021149
Smith G. , McNeill L. , Henstock T.J. , Bull J. 2012 . The structure and fault activity of the Makran accretionary prism . J. geophys. Res. , 117 ( B7 ). doi: 10.1029/2012JB009312 . 10.1029/2012JB009312
Tozer B. , Sandwell D.T. , Smith W.H.F. , Olson C. , Beale J.R. , Wessel P. 2019 . Global bathymetry and topography at 15 arc sec: SRTM15+ . Earth Space Sci. , 6 ( 10 ), 1847 – 1864 . 10.1029/2019EA000658
Wang K. , Hu Y. , He J. 2012 . Deformation cycles of subduction earthquakes in a viscoelastic Earth . Nature , 484 , 327 – 332 . 10.1038/nature11032
Wessel P. , Smith W.H.F. , Scharroo R. , Luis J. , Wobbe F. 2013 . Generic Mapping Tools: improved version released . EOS, Trans. Am. geophys. Un. , 94 ( 45 ), 409 – 410 . 10.1002/2013EO450001
Supplementary data
Fig. S1 . Inverted plate-coupling models using (a) minimum moment and (b) Laplacian regularization.
Fig. S2 . Inverted interseismic viscoelastic plate-coupling models of the Makran megathrust and the diagonal components of the model resolution matrices using varying smoothing coefficient. White arrows: GPS velocity data; Pink arrows: forward-predicted velocity from the inversion; Black solid lines: contours of the plate interface marked by depth in kilometer; Black dash lines: boundaries between different fault segments. Dark green lines: coastlines.
Fig. S3 . Example of 3 nonoverlapping fault patches for inversion approach. When calculating the surface deformation resulting from slipping of each patch, we apply unit slip to the nodes located in the patch of interest while applying zero slip to all the other nodes on the fault.
Fig. S4 . Misfit between the observed and predicted GPS velocities using different rake values in the back-slip rate inversion.
Fig. S5 . Co-seismic static ground/seafloor vertical displacements resulting from single- and multi-segment earthquakes under both trench rupture and blind rupture conditions.
Table S1 . Four model domains and their material properties used in the generation of Green’s functions for both elastic and viscoelastic models.
Table S2 . Source parameters for all back-slip scenarios and the corresponding future earthquake cases.
Table S3 . Maximum tsunami wave height response (in meters) at the 20 tide gauge points (cities) resulting from 12 rupture scenarios under both trench rupture and blind rupture conditions.
Table S4 . Peak-to-peak tsunami wave height response (in meters) at the 20 tide gauge points (cities) resulting from 12 rupture scenarios under both trench rupture and blind rupture conditions.
Table S5 . Occurrence of events larger than certain maximum wave height thresholds and Tsunami Hazard Score (THS) at the 20 tide gauge points (cities).
Email alerts
Astrophysics data system, citing articles via.
- Recommend to your Library
- Advertising and Corporate Services
- Journals Career Network
Affiliations
- Online ISSN 1365-246X
- Print ISSN 0956-540X
- Copyright © 2024 The Royal Astronomical Society
- About Oxford Academic
- Publish journals with us
- University press partners
- What we publish
- New features
- Open access
- Institutional account management
- Rights and permissions
- Get help with access
- Accessibility
- Advertising
- Media enquiries
- Oxford University Press
- Oxford Languages
- University of Oxford
Oxford University Press is a department of the University of Oxford. It furthers the University's objective of excellence in research, scholarship, and education by publishing worldwide
- Copyright © 2024 Oxford University Press
- Cookie settings
- Cookie policy
- Privacy policy
- Legal notice
This Feature Is Available To Subscribers Only
Sign In or Create an Account
This PDF is available to Subscribers Only
For full access to this pdf, sign in to an existing account, or purchase an annual subscription.
Effect on People Who Have Been Through Tsunami Essay
Introduction, post traumatic stress disorder, symptoms of ptsd, the tsunami and the psychological effects, post-tsunami management of ptsd, the theory of psychoanalysis, reference list.
The Tsunami disaster that occurred towards the end of 2004 resulted in a huge burden on the community both in physical terms and psychological trauma. A big number of the population lost their lives and property worth millions of dollars was destroyed. In addition, members of the families that were diseased were left with psychological trauma that affected them after the event.
The community and government were left with a major challenge of how to cope with the physical and psychological stress that was quite evident. Many individuals were not only in need of material assistance, but also psychosocial care to help them cope with the psychological trauma they went through.
Most of the individuals suffered from anxiety disorders, depressive disorders, as well as post traumatic stress disorders (PTSD). PTSD is a disorder people go through after experiencing a dangerous event. This is a severe disorder and can last for a few days to months. The severity depends on the intensity of the dangerous event as well as the surrounding circumstances.
PTSD is also experienced by solders after taking part in wars and the symptoms can affect them for the rest of their lives. Most of the Tsunami victims experienced PTSD which affected their family life, social life as well as their work. Most of them had to shelter in camps since most of their houses were destroyed. It is in these camps that they received both physical and psychological help.
This paper will look at what is meant by PTSD and the symptoms of this disorder. It also gives an overview of the Tsunami disaster including the physical and psychological effects of the dangerous events. It will go further to highlight the recovery response that was initiated to help the Tsunami PTSD patients and conclude by looking at the psychoanalysis theory developed by Sigmund Freud.
Post-traumatic stress disorder is an anxiety people go through after experiencing a dangerous event. When people are in danger, they are normally engraved with fear and uncertainties of the outcomes of such an event. This is a health response and it is meant to help the body as it defends itself against danger. However, a person who experiences post traumatic stress (PTSD) may have a damaged reaction. Individuals under PTSD most of the time feel strained and terrified even when out of danger.
They fear for their lives and most of them suffer from sleepless nights because they are even afraid of sleeping (Myers, 2010). The occurrence of the dangerous event keeps recurring on people’s mind causing anxiety and depression. If a person is not treated early, he may suffer from PTSD for the rest of his life and may even lead to untimely death.
Any person can be a victim of PTSD; it affects children, the youths, and even old people. It normally affects people who have gone through a dangerous situation or witnessed a dangerous event. Events that can cause PTSD include war, sexual assault, motor accidents, and natural disasters such as earthquakes, fires, the tsunami, and many others.
However, it is worth noting that not every person that experiences PTSD has actually experienced a dangerous event. There are people who get PTSD after receiving bad news, for instances, news about the death of a loved one or a friend.
The symptoms of PTSD vary with the kind of danger. These symptoms can be classified into three classes as follows:
- Re-experiencing symptoms- this includes flashbacks about the dangerous event, bad dreams, or terrifying thoughts. These symptoms may interfere with a person’s daily routine because of lack of concentration. Some people even day dream and may bust out crying, shouting or running for help. Symptoms may be re-experienced where object or even prevailing situations may elicit the signs (Myers, 2010). These symptoms require professional help to deal with because a person experiencing them live with self denial and does not accept what he is going through. They can last for 4-6 weeks or even months after the event but this depends on a person’s willingness to accept assistance and the desire to change.
- Avoidance symptoms- a person experiencing PTSD tries to keep away from any object or situation that will be a constant reminder of the event. Others even withdraw themselves from the society and their participation in social activities diminishes. At times, they may be overcome by feel emotions and start carrying without a reason. Other people experience depression, worry or remorse, while others may tend to shun away from activities that they found enjoyable before the event took place. The first step in helping a person with these symptoms is to remove all objects that may act as a constant reminder of the event and also to change his environment so that he is able to see life from another angle.
- Hyper arousal symptoms- they include feeling tense, insomnia, or being angered easily. These symptoms are hardly triggered by objects that remind a person about a dangerous event but they are rather constant. They make a person to feel stressed and angry most of the time which may not be pleasant especially in a work place (Myers, 2010). These are the most severe symptoms since they do not only affect the individual psychology but also that of the people around him. It is very normal to go through these symptoms after going experiencing a dangerous event. Some people experience them for a short period of time while others may experience them for months if not years.
A great tsunami occurred on December 24, 2004 and affected a large population from some parts of East Africa and South Asia. It was a natural disaster that did not only take the lives of many people but also destroyed a lot of property. In Sri Lanka (which was the most hit region) alone, 31, 187 people were reported dead, more than four thousand were reported missing, over twenty thousand had been injured and almost half a million people were displaced (Tull, 2009).
As it is normal in many dangerous events, the tsunami was not an exemption as many people were left with psychological problems especially those who witnessed the disaster in Sri Lanka. A study that was carried out about 4 weeks after the tsunami discovered that about 39% of children were suffering from PTSD (Daily News Sri Lanka, 2005). A similar study found out that more than 40% of adolescents and about 20% of adult women showed symptoms of PTSD 4 months later (Daily News Sri Lanka, 2005).
However, the researches do not provide enough information about the long-term effects of the crisis but according to WHO a bigger percentage of people gradually developed symptoms of PTSD due to the event. To verify this claim, an international research group was formed to study the post-tsunami effect.
A study was carried out in Peraliya in Sri Lanka district about one year after the disaster. This is an area where more than 2, 000 people had lost their lives and proximately 450 families had been displaced, and the entire society was in a mess.
A part from the natural disaster, many people had gone through many psychological effects. For instance, about 80% feared for their life because they could still visualize the event that took place. More than 50% had already lost at least one member of the family and they had constant flashback of the event while approximately 80% had lost a friend.
On the other hand, part of the population that was interviewed explained how some of their family members and friends had been seriously injured as a result of the crisis and they could spend sleepless nights. Out of all the participants of the study, 21% experienced PTSD, 16% suffered from severe depression, 30% had relentless anxiety, while more than 22% had somatic signs (Tull, 2009).
Some of the symptoms that were common among many victims of the Tsunami were tension, fear, anxiety, confusion, flashbacks, plus other permanent emotional scars. Other people expressed pessimistic thoughts, withdrawal from social activities, among other physical symptoms.
Some people were able to cope with these psychological effects while others needed professional care. Some of the disaster survivors developed psychiatric disorders including but not limited to anxiety disorders, depression disorders, and PTSD. Most of the victims suffering from PTSD depressed and could not understand what was happening to them (Daily News Sri Lanka, 2005).
They resorted to crying a lot to at least try to ease the pain although this did not work. Some of them wished to die because they could not carry the burden that was imposed on them. It was difficult for family members trying to help those suffering from PTSD because they too were still in agony. It is not always easy to witness the death of a loved one and lead a normal life. What was happening to the post-tsunami victims was normal and it is associated to all dangerous events.
Many people can recover from PTSD on their own if they are given proper education, they are supported, and their lifestyles modified. The first step in helping a person under PTSD is to help him come into terms with the condition he is going through and to reassure him that what he is going through is normal and is as a result of the traumatic event that he experienced.
Also support from family members, friends, and other professionals helps a person to recover quickly from PTSD. Some anxiety management strategies such as breathing techniques and involvement in social activities have also been known to play a big part in the recovery process. However, patients with severe symptoms of PTSD such as recurrent flashbacks, and lack of sleep and those not responding to PTSD treatment should be referred to a psychiatrist (WHO, 2005).
Most of people who were displaced from tsunami crisis lived in relief camps. Following the disaster, many people were concerned with what had happened and were ready to offer their help in order to help the victims recover from the psychological trauma they went through.
A large number of volunteers all over the world visited these camps to sympathize with the victims. They carried their toolboxes of psychosocial and trauma recovery activities which added confusion and amusement to the people who benefited from their activities as well as the authorities that were concerned with the recovery process.
Psychosocial and mental health programs were initiated in the relief camps by the United States agencies, non-governmental, and governmental institutions to help the victims come into terms with the event.
These programs revived political support, which accelerated the recovery process and many PTSD patients were able to benefit from these programs. In addition, the president of Sri Lanka supported psychological intervention process both financially and psychologically.
He gave these processes the first priority among all the programs that started as part of post-tsunami recovery plan. He realized that if those victims continued to suffer psychologically, no amount of material support would help them come out of the crisis. The president went further to caution all authorities involved in the recovery program to make sure that all personnel hired to conduct the post-trauma counseling were qualified and had enough experience (WHO, 2005).
This is for the reason that some people may be so much willing to offer their help but such assistance may sometimes not be professional and may be only based on belief which can engrave the problem instead of solving it. The acronym PTSD and the word psychosocial were used too often and they became part of the post-tsunami recovery plan. They even became the widely used terms by both politicians and news columnists.
As a team, we also took part in helping the internally displaced persons from the Tsunami. We organized a small fund rising within the school compound where we collected money, clothing as well as food. We visited the victims at the camps and donated some of the material things we had managed to collect and went a head to talk with then.
We played with the children who looked depressed to help them come to terms with their predicament. Since we could not offer any professional psychological help, we just had a general talk with some of the victims and assured them that, they are people out there who care for them and a ready to offer a should to cry on.
From research, it has been found that psychoanalysis forms part of the recovery treatment for people under PTSD. This theory was first developed by Sigmund Freud. He is one of the ancient psychologists. The aim of this theory was to study the psychological functioning of human beings and their behavior. Fraud established clinical procedures that would be used to treat mentally ill persons. According to him, individual personality is developed from childhood experiences.
The aim of this therapy was to convey reserved thoughts and mind-set into consciousness in order to free the patient from suffering recurring fuzzy emotions. These reserved thoughts and feelings are brought to consciousness by encouraging a conversation between the doctor and the patient. Patients are encouraged to talk freely and express their dreams and experiences (Asiado, 2007).
According to Freud, psychoanalysis is a process used to treat individuals suffering from psychological problems. He called it “The doctor’s ‘treatment’ and it involves eliciting repressed memories from the patient by interpreting the responses to his questions” (Asiado, 2007).
He observed that a person suffering from psychology problems would be encouraged to re-live previous experience and this would be used as a technique for treatment. The theory involves intervention such as confronting and illuminating the patient’s pathological suspicions, desires, and guilt. Through analysis of individual’s conflicts, psychoanalysis treatment can be used to prove that patients’ unconsciousness is the worst threat of causing symptoms.
This progression assists in determining solutions for the reticent conflicts. In the psyche, the interpretation of Dreams, Freud proposed that dreams can be used to demonstrate the judgment of the unconscious mind. He described dreams as the noble road to the unconscious. In the unconscious mind painful memories are repressed but can be accessed through psychoanalysis treatment.
PTSD can affect, children, adolescent, and even adults. It knows no borders and affects everyone including soldiers who are believed to be the ‘hardest’ persons. Some of the symptoms of PTSD include: recurrent flashbacks, anxiety, depression, insomnia, bad dreams, and pessimistic thought.
They are people who exhibit these symptoms for a very short period of time while others continue to suffer for months if not years. An example of a dangerous event that left many people with PTSD is the great Tsunami of 2004. Apart from the physical damage that resulted from this event, many people suffered from psychological disorders including PTSD.
Children, adolescents, and adults were displaced and were sheltered in relief camps. Some of the symptoms exhibited by the individuals suffering from PTSD included flashbacks, depression, stress, anxiety, loss of self control, pessimist thoughts, and many more. Most of the people who were displaced were sheltered in relief camps where they received both local and international visitors who sympathized with their predicament.
This is where many non-governmental, governmental and U.S. agencies offered their assistance to the victims. They were given both material support and psychological support. Psychological intervention programs were initiated with the support of the president of Sri Lanka to help the people who were suffering from PTSD. The psychological treatment which they received was based on the psychoanalysis theory developed by Sigmund Freud.
Asiado, T. (2007). Sigmund Freud and His Couch . Web.
Daily News, Sri Lanka (2005). Editorial: The Primacy of Mental Health Protection. (12 February 2005). Colombo 10: Lake House Press.
Myers, D.G. (2010). Psychology (9th ed.). New York, NY: Worth Publishers
Tull, M. (2009). The Psychological Impact of the 2004 Tsunami . Web.
WHO (2005). Psychosocial Care of Tsunami-Affected Populations . PDF File. Web.
- Chicago (A-D)
- Chicago (N-B)
IvyPanda. (2018, July 20). Effect on People Who Have Been Through Tsunami. https://ivypanda.com/essays/effect-on-people-who-have-been-through-tsunami/
"Effect on People Who Have Been Through Tsunami." IvyPanda , 20 July 2018, ivypanda.com/essays/effect-on-people-who-have-been-through-tsunami/.
IvyPanda . (2018) 'Effect on People Who Have Been Through Tsunami'. 20 July.
IvyPanda . 2018. "Effect on People Who Have Been Through Tsunami." July 20, 2018. https://ivypanda.com/essays/effect-on-people-who-have-been-through-tsunami/.
1. IvyPanda . "Effect on People Who Have Been Through Tsunami." July 20, 2018. https://ivypanda.com/essays/effect-on-people-who-have-been-through-tsunami/.
Bibliography
IvyPanda . "Effect on People Who Have Been Through Tsunami." July 20, 2018. https://ivypanda.com/essays/effect-on-people-who-have-been-through-tsunami/.
- Tsunami Disasters in Okushiri Island
- Tsunami Funding: On Assistance to the Victims of the December 2004 Tsunami
- Tsunami Geological Origin
- The Fears Within: What Do You See in the Mirror?
- Catatonic Depression: Etiology and Management
- Treatment of Bipolar Disorder
- Defining Disabilities in Modern World
- Psychology of Behavior: Anxiety Disorders
- Work & Careers
- Life & Arts
Become an FT subscriber
Try unlimited access Only $1 for 4 weeks
Then $75 per month. Complete digital access to quality FT journalism on any device. Cancel anytime during your trial.
- Global news & analysis
- Expert opinion
- Special features
- FirstFT newsletter
- Videos & Podcasts
- Android & iOS app
- FT Edit app
- 10 gift articles per month
Explore more offers.
Standard digital.
- FT Digital Edition
Premium Digital
Print + premium digital, ft professional, weekend print + standard digital, weekend print + premium digital.
Essential digital access to quality FT journalism on any device. Pay a year upfront and save 20%.
- Global news & analysis
- Exclusive FT analysis
- FT App on Android & iOS
- FirstFT: the day's biggest stories
- 20+ curated newsletters
- Follow topics & set alerts with myFT
- FT Videos & Podcasts
- 20 monthly gift articles to share
- Lex: FT's flagship investment column
- 15+ Premium newsletters by leading experts
- FT Digital Edition: our digitised print edition
- Weekday Print Edition
- Videos & Podcasts
- Premium newsletters
- 10 additional gift articles per month
- FT Weekend Print delivery
- Everything in Standard Digital
- Everything in Premium Digital
Complete digital access to quality FT journalism with expert analysis from industry leaders. Pay a year upfront and save 20%.
- 10 monthly gift articles to share
- Everything in Print
- Make and share highlights
- FT Workspace
- Markets data widget
- Subscription Manager
- Workflow integrations
- Occasional readers go free
- Volume discount
Terms & Conditions apply
Explore our full range of subscriptions.
Why the ft.
See why over a million readers pay to read the Financial Times.
International Edition
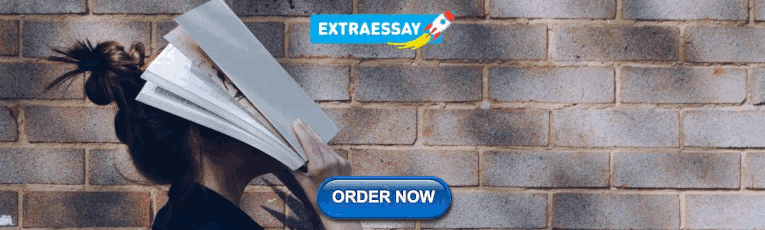
IMAGES
VIDEO
COMMENTS
Tsunami Disaster in Asia, held in Phuket, Thailand, May 4-6, 2005,1 and the Third International Conference on Early Warning, held in Bonn, Germany, March 27-29, 2006.2 2. Laying the groundwork for tsunami response management Several important international and regional meetings, most of them taking place around the time of
This paper includes a brief overview of current gaps and challenges in tsunami risk communication and management, visualized through a schematic figure (see Fig. 1 and Table 1). Fig. 1 illustrates an integrated risk governance process [28, 29] adopted in this paper and described in detail in the following. Table 1 provides a summary of different stages of "understanding risk" and "risk ...
Summary. Tsunamis are natural hazards that have caused massive destruction and loss of life in coastal areas worldwide for centuries. Major programs promoting tsunami safety, however, date from the early 20th century and have received far greater emphasis following two major events in the opening decade of the 21st century: the Indian Ocean Tsunami of December 26, 2004, and the Great East ...
With its Intergovernmental Oceanographic Commission joined by 150 Member States, and its expertise in the culture and education fields, UNESCO coordinates actions by governments, scientists, the private sector, civil society and other UN organizations. Together, we created the tsunami warning system. We map the ocean depths, identify species ...
The ideal system incorporates risk assessment, public education, tsunami detection, warning management, and public response . Protecting and warning the public begins with an understanding of the tsunami risk envi-Page 31 Share Cite. Suggested Citation:"1 Introduction." National Research Council. 2011.
Introduction. To reduce the effects and minimize possible dangers and losses as was experienced in the 2004 Tsunami, all organizations and societies will need to be prepared for possible disasters and emergencies and create systems that should deal with these disasters and emergencies. The saving of lives during a disaster and emergency ...
During a tsunami. If you're in a tsunami area and there is an earthquake, first drop to the floor, cover your head and neck, and hold on to something stable. If you're in a low-lying area, move ...
The manual is based on papers and presentations prepared for a programme of training workshops conducted in countries of the Indian Ocean, Southeast Asia and South China Sea Region during 2008-2010, part of the project entitled "Strengthening Tsunami Warning and Emergency Responses: Training Workshops on the Development of Standard Operating ...
At 07:59 Local Time (00:59 UTC) on December 26, 2004, a Mw = 9.3 megathrust earthquake occurred along 1300 km of the oceanic subduction zone located 100 km west of Sumatra and the Nicobar and Andaman Islands in the eastern Indian Ocean (1). Highly destructive waves were generated by up to.
This second edition reflects significant progress in tsunami research, monitoring and mitigation within the last decade. Primarily meant to summarize the state-of-the-art knowledge on physics of tsunamis, it describes up-to-date models of tsunamis generated by a submarine earthquake, landslide, volcanic eruption, meteorite impact, and moving atmospheric pressure inhomogeneities.
Tsunami's Reasons and Effects Essay. For many inhabitants of the Earth, a tsunami threat looks like an abstract and very exotic danger. However, the vagaries of nature in recent years are such that it is quite difficult to feel completely protected from such a danger. Moreover, even in a small lake, under a certain confluence of circumstances ...
500+ Words Essay on Tsunami. Tsunami is a phenomenon where a series of strong waves that are responsible for the surge in water sometimes reach the heights in many meters. This is a natural disaster that is caused due to the volcano eruption in the ocean beds. Also, a phenomenon like landslides and earthquakes contributes to reasons for a tsunami.
Essay # 1. Meaning of Tsunami: ADVERTISEMENTS: When a large earthquake happens beneath an ocean floor, it can change the level of the floor suddenly, raising and lowering it substantially. This produces a large disturbance in the sea. The size and energy of disturbance depends on the magnitude of the earth quake.
Australia Tsunami Warning System (ATWS) After the occurrence of Indian Ocean tsunami in 2004, the system was established. Australia Bureau of Meteorology, Geosciences Australia and Emergency Management of Australia were involved in its establishment. They all aimed at providing timely tsunami warning system to all Australian population.
At 07:59 local time (00:59 UTC) on 26 December 2004, a moment magnitude (M) 9.3 megathrust earthquake occurred along 1300 km of the oceanic subduction zone located 100 km west of Sumatra and the Nicobar and Andaman Islands in the eastern Indian Ocean [ Stein and Okal, 2005 ]. Highly destructive waves were generated by up to 10-m vertical ...
500 Words Essay On Tsunami. A tsunami is a natural disaster that originates under water and is brought on by the waves that an earthquake causes to be generated in the ocean. The tsunami's impacts were initially reported by Greeks on Earth. They claim that earthquakes on land and tsunamis are identical. The sole distinction between a tsunami ...
Based on the High-Powered Committee on Disaster Management's report (2001), the term "tsunami" refers to an ocean wave that is caused by an ocean event, such as an earthquake, landslide, or volcanic eruption. A tsunami is not just one wave; rather, it is a sequence of waves brought on by geological processes occurring near or below the ...
Disaster Management Essay. Disaster Management' is the simple term of management which embraces loads of disaster-related activities. Disaster occurs frequently in some parts of the world. Japan is the best example of it. Japanese people are annoyed on Tsunamis and earthquakes. The local scene is not much different from the global one.
The series of extremely long waves, Tsunami are very long wavelengths of water caused by a large and sudden displacement of the ocean due to earthquakes, volcanic eruptions etc. These are also called seismic sea waves and are one of the most powerful and destructive natural forces. When they reach the coast, they can cause dangerous coastal ...
500+ Words Essay on Disaster Management. Nature has various manifestations both gentle as well as aggressive. We see how sometimes it is so calm while the other times it becomes fierce. The calm side is loved by everyone, of course, however, when the ferocious side is shown, devastation happens. As humans cannot control everything, certain ...
Why in News. According to experts from the Indian National Centre for Ocean Information System (INCOIS), India is much safer against tsunami threats than it was in 2004, due to the establishment of a state-of-the-art tsunami early warning system at INCOIS.. Key Points. Indian Tsunami Early Warning System: The Indian Tsunami Early Warning System (ITEWS) was established in 2007 and is based at ...
Abstract. Numerical model simulations, combined with tide-gauge and satellite altimetry data, reveal that wave amplitudes, directionality, and global propagation patterns of the 26 December 2004 Sumatra tsunami were primarily determined by the orientation and intensity of the offshore seismic line source and subsequently by the trapping effect of mid-ocean ridge topographic waveguides.
4.2. Tsunami simulations in the western Indian Ocean: results. Fig. 8 illustrates the maximum tsunami wave heights calculated at the 20 tide gauge points for all six rupture scenarios under trench rupture and blind rupture conditions, respectively. Supporting Information Tables S3 and S4 summarize the maximum and peak-to-peak tsunami wave ...
In the past two years, according to reports, it has retracted more than 11,000 papers. Wiley and other publishers, such as Springer Nature, are battling an epidemic of fakery. But battle it they must.
An example of a dangerous event that left many people with PTSD is the great Tsunami of 2004. Apart from the physical damage that resulted from this event, many people suffered from psychological disorders including PTSD. Children, adolescents, and adults were displaced and were sheltered in relief camps. Some of the symptoms exhibited by the ...
This paper discusses recent challenges in BCEAO monetary policy, from a recent spike in inflation, the persistent erosion of external reserves, and strains in the regional financial market. In response to these shocks, the BCEAO operated via both policy rates and liquidity management, including by shifting from fixed to variable rate auctions. The paper finds that the conduct of monetary ...
Oligarch Bidzina Ivanishvili has sought to steer Caucasus nation back into Russia's orbit
The result is a revisionist history of the cold war that downplays ideology as Moscow's guiding motive. This marks quite a departure from most cold war histories, which pay more attention to ...