Thank you for visiting nature.com. You are using a browser version with limited support for CSS. To obtain the best experience, we recommend you use a more up to date browser (or turn off compatibility mode in Internet Explorer). In the meantime, to ensure continued support, we are displaying the site without styles and JavaScript.
- View all journals
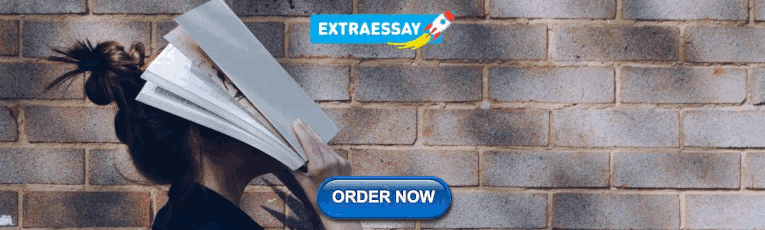
Biochemistry articles from across Nature Portfolio
Biochemistry is the study of the structure and function of biological molecules such as proteins, nucleic acids, carbohydrates and lipids. Biochemistry is also used to describe techniques suited to understanding the interactions and functions of biological molecules, including traditional techniques such as Western blotting, co-immunoprecipitation, and chromatography methods.
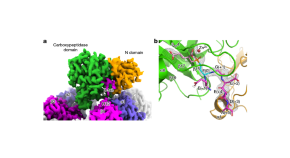
Cracking the tubulin code: enzyme structures offer clues to microtubule control
Cellular structural filaments called microtubules are made up of units of tubulin proteins. Various enzymes add modifications to and remove them from tubulin units; together, these modifications form a ‘tubulin code’ that controls microtubule function. Structures of an enzyme called CCP5 reveal how it deforms tubulin tails to recognize and remove modifications called single-glutamate branches.
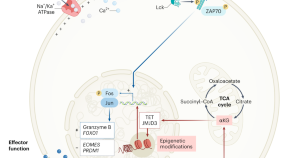
A pinch of salt boosts T cell function
Effector CD8 + T cells with cytotoxic ability are crucial for immunotherapy success. Two studies show that salt (NaCl) supplementation can enhance the effector function of CD8 + T cells and boost their antitumor responses.
- Karina L. Hajdu
- Lorène Rousseau
- Ping-Chih Ho
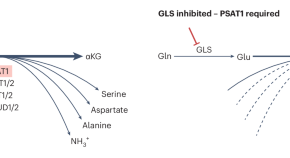
Affinity war: PSAT1 outcompetes the rest
Resistance to glutamine restriction is mediated by increased serine synthesis. The high affinity of serine synthesis enzyme PSAT1 for glutamate drives sustained glutamine utilization. Combined pathway targeting limits tumour growth in mouse breast cancer models.
- Richard Possemato
Related Subjects
- Biocatalysis
- Biogeochemistry
- Bioinorganic chemistry
- Biophysical chemistry
- Carbohydrates
- Chemical modification
- Enzyme mechanisms
- Glycobiology
- Histocytochemistry
- Immunochemistry
- Ion channels
- Metabolomics
- Neurochemistry
- Protein folding
- Proteolysis
- Structural biology
Latest Research and Reviews
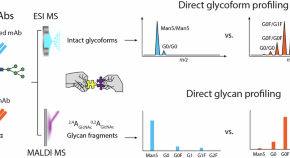
Direct glycosylation analysis of intact monoclonal antibodies combining ESI MS of glycoforms and MALDI-in source decay MS of glycan fragments
Glycoengineering of monoclonal antibodies (mAbs) has the potential to improve the efficacy of biopharmaceuticals, however, monitoring the glycoengineering process by glycosylation analysis often requires a multi-method approach. Here, the authors present a direct glycosylation analysis of intact mAbs by combining conventional ESI-MS of intact glycoforms and MALDI-in-source decay FT-ICR MS of glycan fragments.
- Isabella Senini
- Sara Tengattini
- Simone Nicolardi
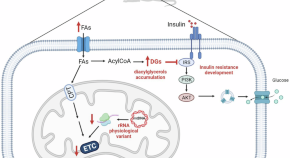
Haplotype variability in mitochondrial rRNA predisposes to metabolic syndrome
Detailed characterization of mtDNA haplotypes uncovers the impact of mitochondrial rRNA variations on mitochondrial translation and oxidative capacity and their metabolic consequences including insulin resistance development induced by high-fat diet.
- Petr Pecina
- Kristýna Čunátová
- Alena Pecinová
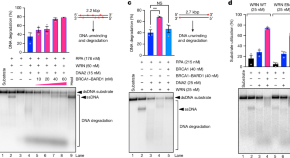
Mechanism of BRCA1–BARD1 function in DNA end resection and DNA protection
BRCA1–BARD1 directly promotes double-strand break repair by stimulating long-range DNA end resection pathways.
- Ilaria Ceppi
- Maria Rosaria Dello Stritto
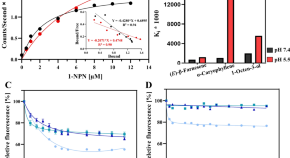
Revealing the pH-dependent conformational changes in sol g 2.1 protein and potential ligands binding
- Siriporn Nonkhwao
- Doungkamol Leaokittikul
- Sakda Daduang
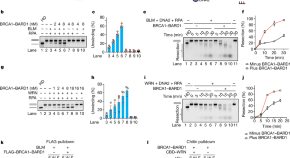
Promotion of DNA end resection by BRCA1–BARD1 in homologous recombination
Using highly purified protein factors, we provide evidence that BRCA1–BARD1 physically interacts with EXO1, BLM and WRN and upregulates the activity of all three resection pathways.
- Sameer Salunkhe
- James M. Daley
- Patrick Sung
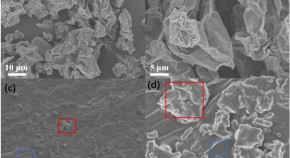
GO promotes detoxification of nicosulfuron in sweet corn by enhancing photosynthesis, chlorophyll fluorescence parameters, and antioxidant enzyme activity
- Zhihua Zhen
News and Comment
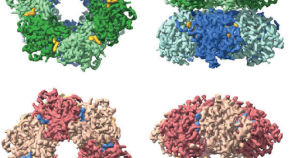
Measuring interactions in a circadian clock
An article in Nature Communications uses an Ising-like model to determine the interactions between monomers in a component of the cyanobacterial circadian clock.
- Zoe Budrikis
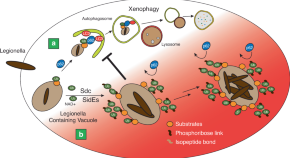
Legionella pneumophila evades host-autophagic clearance using phosphoribosyl-polyubiquitin chains
- Minhyeong Choi
- Minwoo Jeong
- Donghyuk Shin
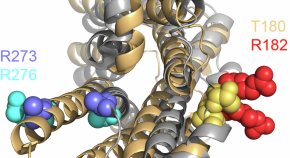
Opioid receptors: single molecule studies shed light on mechanisms of efficacy
- Cornelius Krasel
- Moritz Bünemann
Quick links
- Explore articles by subject
- Guide to authors
- Editorial policies

Information
- Author Services
Initiatives
You are accessing a machine-readable page. In order to be human-readable, please install an RSS reader.
All articles published by MDPI are made immediately available worldwide under an open access license. No special permission is required to reuse all or part of the article published by MDPI, including figures and tables. For articles published under an open access Creative Common CC BY license, any part of the article may be reused without permission provided that the original article is clearly cited. For more information, please refer to https://www.mdpi.com/openaccess .
Feature papers represent the most advanced research with significant potential for high impact in the field. A Feature Paper should be a substantial original Article that involves several techniques or approaches, provides an outlook for future research directions and describes possible research applications.
Feature papers are submitted upon individual invitation or recommendation by the scientific editors and must receive positive feedback from the reviewers.
Editor’s Choice articles are based on recommendations by the scientific editors of MDPI journals from around the world. Editors select a small number of articles recently published in the journal that they believe will be particularly interesting to readers, or important in the respective research area. The aim is to provide a snapshot of some of the most exciting work published in the various research areas of the journal.
Original Submission Date Received: .
- Active Journals
- Find a Journal
- Proceedings Series
- For Authors
- For Reviewers
- For Editors
- For Librarians
- For Publishers
- For Societies
- For Conference Organizers
- Open Access Policy
- Institutional Open Access Program
- Special Issues Guidelines
- Editorial Process
- Research and Publication Ethics
- Article Processing Charges
- Testimonials
- Preprints.org
- SciProfiles
- Encyclopedia
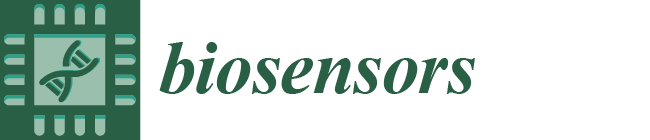
Article Menu
- Subscribe SciFeed
- Recommended Articles
- PubMed/Medline
- Google Scholar
- on Google Scholar
- Table of Contents
Find support for a specific problem in the support section of our website.
Please let us know what you think of our products and services.
Visit our dedicated information section to learn more about MDPI.
JSmol Viewer
Dna-based biosensors for the biochemical analysis: a review.
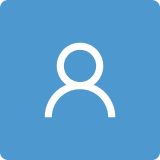
1. Introduction
2. functional dna strands-based biosensors, 2.1. dna aptamer biosensors.
Click here to enlarge figure
2.2. DNAzyme Biosensors
3. dna hybridization-based biosensors, 3.1. biosensor probes based on dna hairpin, 3.2. signal-enhanced biosensors based on dna hybridization, 4. dna templated biosensors, 4.1. dna tile assembly and its application, 4.2. dna origami assembly and its application, 5. summary and conclusions, 6. future perspective, author contributions, institutional review board statement, informed consent statement, conflicts of interest.
- Morán, M.C.; Nogueira, D.R.; Vinardell, M.P.; Miguel, M.G.; Lindman, B. Mixed protein–DNA gel particles for DNA delivery: Role of protein composition and preparation method on biocompatibility. Int. J. Pharm. 2013 , 454 , 192–203. [ Google Scholar ] [ CrossRef ] [ Green Version ]
- Hutton, J.R. Renaturation Kinetics and thermal stability of DNA in aqueous solutions of formamide and urea. Nucleic Acids Res. 1977 , 4 , 3537–3555. [ Google Scholar ] [ CrossRef ]
- Blake, R.D.; Delcourt, S.G. Thermal stability of DNA. Nucleic Acids Res. 1998 , 26 , 3323–3332. [ Google Scholar ] [ CrossRef ] [ Green Version ]
- Giesen, U.; Kleider, W.; Berding, C.; Geiger, A.; Ørum, H.; Nielsen, P.E. A formula for thermal stability (Tm) prediction of PNA/DNA duplexes. Nucleic Acids Res. 1998 , 26 , 5004–5006. [ Google Scholar ] [ CrossRef ]
- Li, F.; Zhang, H.; Dever, B.; Li, X.-F.; Le, X.C. Thermal Stability of DNA Functionalized Gold Nanoparticles. Bioconjug. Chem. 2013 , 24 , 1790–1797. [ Google Scholar ] [ CrossRef ]
- Jäger, S.; Rasched, G.; Kornreich-Leshem, H.; Engeser, M.; Thum, O.; Famulok, M. A Versatile Toolbox for Variable DNA Functionalization at High Density. J. Am. Chem. Soc. 2005 , 127 , 15071–15082. [ Google Scholar ] [ CrossRef ]
- Saccà, B.; Niemeyer, C.M. Functionalization of DNA nanostructures with proteins. Chem. Soc. Rev. 2011 , 40 , 5910–5921. [ Google Scholar ] [ CrossRef ]
- Wijaya, A.; Hamad-Schifferli, K. Ligand Customization and DNA Functionalization of Gold Nanorods via Round-Trip Phase Transfer Ligand Exchange. Langmuir 2008 , 24 , 9966–9969. [ Google Scholar ] [ CrossRef ] [ PubMed ]
- Kerman, K.; Kobayashi, M.; Tamiya, E. Recent trends in electrochemical DNA biosensor technology. Meas. Sci. Technol. 2003 , 15 , 1–11. [ Google Scholar ] [ CrossRef ] [ Green Version ]
- He, P.; Xu, Y.; Fang, Y. A Review: Electrochemical DNA Biosensors for Sequence Recognition. Anal. Lett. 2005 , 38 , 2597–2623. [ Google Scholar ] [ CrossRef ]
- Saidur, M.R.; Aziz, A.R.A.; Basirun, W.J. Recent advances in DNA-based electrochemical biosensors for heavy metal ion detection: A review. Biosens. Bioelectron. 2017 , 90 , 125–139. [ Google Scholar ] [ CrossRef ] [ PubMed ]
- Liang, G.; Man, Y.; Li, A.; Jin, X.; Liu, X.; Pan, L. DNAzyme-based biosensor for detection of lead ion: A review. Microchem. J. 2017 , 131 , 145–153. [ Google Scholar ] [ CrossRef ]
- Reder-Christ, K.; Bendas, G. Biosensor Applications in the Field of Antibiotic Research—A Review of Recent Developments. Sensors 2011 , 11 , 9450–9466. [ Google Scholar ] [ CrossRef ] [ PubMed ] [ Green Version ]
- Dehghani, S.; Nosrati, R.; Yousefi, M.; Nezami, A.; Soltani, F.; Taghdisi, S.M.; Abnous, K.; Alibolandi, M.; Ramezani, M. Aptamer-based biosensors and nanosensors for the detection of vascular endothelial growth factor (VEGF): A review. Biosens. Bioelectron. 2018 , 110 , 23–37. [ Google Scholar ] [ CrossRef ]
- Mehlhorn, A.; Rahimi, P.; Joseph, Y. Aptamer-Based Biosensors for Antibiotic Detection: A Review. Biosensors 2018 , 8 , 54. [ Google Scholar ] [ CrossRef ] [ Green Version ]
- Bishop, G.R.; Ren, J.; Polander, B.C.; Jeanfreau, B.D.; Trent, J.O.; Chaires, J.B. Energetic basis of molecular recognition in a DNA aptamer. Biophys. Chem. 2007 , 126 , 165–175. [ Google Scholar ] [ CrossRef ]
- Smirnov, I.; Shafer, R.H. Effect of Loop Sequence and Size on DNA Aptamer Stability. Biochemistry 2000 , 39 , 1462–1468. [ Google Scholar ] [ CrossRef ]
- Xia, T.; Yuan, J.; Fang, X. Conformational Dynamics of an ATP-Binding DNA Aptamer: A Single-Molecule Study. J. Phys. Chem. B 2013 , 117 , 14994–15003. [ Google Scholar ] [ CrossRef ]
- Minagawa, H.; Kataoka, Y.; Kuwahara, M.; Horii, K.; Shiratori, I.; Waga, I. A high affinity modified DNA aptamer containing base-appended bases for human β-defensin. Anal. Biochem. 2020 , 594 , 113627. [ Google Scholar ] [ CrossRef ]
- Tan, L.; Neoh, K.G.; Kang, E.-T.; Choe, W.-S.; Su, X. Affinity analysis of DNA aptamer—Peptide interactions using gold nanoparticles. Anal. Biochem. 2012 , 421 , 725–731. [ Google Scholar ] [ CrossRef ]
- Lai, J.-C.; Hong, C.-Y. Magnetic-Assisted Rapid Aptamer Selection (MARAS) for Generating High-Affinity DNA Aptamer Using Rotating Magnetic Fields. ACS Comb. Sci. 2014 , 16 , 321–327. [ Google Scholar ] [ CrossRef ]
- Keum, J.-W.; Bermudez, H. Enhanced resistance of DNA nanostructures to enzymatic digestion. Chem. Commun. 2009 , 7036–7038. [ Google Scholar ] [ CrossRef ]
- Xue, C.; Zhang, S.; Yu, X.; Hu, S.; Lu, Y.; Wu, Z.-S. Periodically Ordered, Nuclease-Resistant DNA Nanowires Decorated with Cell-Specific Aptamers as Selective Theranostic Agents. Angew. Chem. Int. Ed. 2020 , 59 , 17540–17547. [ Google Scholar ] [ CrossRef ]
- Ma, Y.; Ali, S.R.; Dodoo, A.S.; He, H. Enhanced Sensitivity for Biosensors: Multiple Functions of DNA-Wrapped Single-Walled Carbon Nanotubes in Self-Doped Polyaniline Nanocomposites. J. Phys. Chem. B 2006 , 110 , 16359–16365. [ Google Scholar ] [ CrossRef ]
- Li, J.; Fu, W.; Bao, J.; Wang, Z.; Dai, Z. Fluorescence Regulation of Copper Nanoclusters via DNA Template Manipulation toward Design of a High Signal-to-Noise Ratio Biosensor. ACS Appl. Mater. Interfaces 2018 , 10 , 6965–6971. [ Google Scholar ] [ CrossRef ]
- Liu, J. DNA-stabilized, fluorescent, metal nanoclusters for biosensor development. TrAC Trends Anal. Chem. 2014 , 58 , 99–111. [ Google Scholar ] [ CrossRef ] [ Green Version ]
- Blackwell, T.K.; Kretzner, L.; Blackwood, E.M.; Eisenman, R.N.; Weintraub, H. Sequence-Specific DNA Binding by the c-Myc Protein. Science 1990 , 250 , 1149–1151. [ Google Scholar ] [ CrossRef ]
- Paborsky, L.R.; McCurdy, S.N.; Griffin, L.C.; Toole, J.J.; Leung, L.L. The single-stranded DNA aptamer-binding site of human thrombin. J. Biol. Chem. 1993 , 268 , 20808–20811. [ Google Scholar ] [ CrossRef ]
- Muhammad, M.; Huang, Q. A review of aptamer-based SERS biosensors: Design strategies and applications. Talanta 2021 , 227 , 122188. [ Google Scholar ] [ CrossRef ]
- He, L.; Huang, R.; Xiao, P.; Liu, Y.; Jin, L.; Liu, H.; Li, S.; Deng, Y.; Chen, Z.; Li, Z.; et al. Current signal amplification strategies in aptamer-based electrochemical biosensor: A review. Chin. Chem. Lett. 2021 , 32 , 1593–1602. [ Google Scholar ] [ CrossRef ]
- Ekrami, E.; Pouresmaieli, M.; Shariati, P.; Mahmoudifard, M. A review on designing biosensors for the detection of trace metals. Appl. Geochem. 2021 , 127 , 104902. [ Google Scholar ] [ CrossRef ]
- Breaker, R.R.; Joyce, G.F. A DNA enzyme that cleaves RNA. Chem. Biol. 1994 , 1 , 223–229. [ Google Scholar ] [ CrossRef ]
- Yang, H.; Zhou, Y.; Liu, J. G-quadruplex DNA for construction of biosensors. TrAC Trends Anal. Chem. 2020 , 132 , 116060. [ Google Scholar ] [ CrossRef ]
- Wang, S. Construction of DNA Biosensors for Mercury (II) Ion Detection Based on Enzyme-Driven Signal Amplification Strategy. Biomolecules 2021 , 11 , 399. [ Google Scholar ] [ CrossRef ]
- Wang, F.; Zhang, Y.; Lu, M.; Du, Y.; Chen, M.; Meng, S.; Ji, W.; Sun, C.; Peng, W. Near-infrared band Gold nanoparticles-Au film “hot spot” model based label-free ultratrace lead (II) ions detection via fiber SPR DNAzyme biosensor. Sens. Actuators B Chem. 2021 , 337 , 129816. [ Google Scholar ] [ CrossRef ]
- Glick, G.D. Synthesis of a conformationally restricted DNA hairpin. J. Org. Chem. 1991 , 56 , 6746–6747. [ Google Scholar ] [ CrossRef ]
- Du, H.; Disney, M.D.; Miller, B.L.; Krauss, T.D. Hybridization-Based Unquenching of DNA Hairpins on Au Surfaces: Prototypical “Molecular Beacon” Biosensors. J. Am. Chem. Soc. 2003 , 125 , 4012–4013. [ Google Scholar ] [ CrossRef ]
- Du, H.; Strohsahl, C.M.; Camera, J.; Miller, B.L.; Krauss, T.D. Sensitivity and Specificity of Metal Surface-Immobilized “Molecular Beacon” Biosensors. J. Am. Chem. Soc. 2005 , 127 , 7932–7940. [ Google Scholar ] [ CrossRef ]
- Liu, A.; Wang, K.; Weng, S.; Lei, Y.; Lin, L.; Chen, W.; Lin, X.; Chen, Y. Development of electrochemical DNA biosensors. TrAC Trends Anal. Chem. 2012 , 37 , 101–111. [ Google Scholar ] [ CrossRef ]
- Seeman, N.C. Nucleic acid junctions and lattices. J. Theor. Biol. 1982 , 99 , 237–247. [ Google Scholar ] [ CrossRef ]
- Winfree, E.; Liu, F.; Wenzler, L.A.; Seeman, N.C. Design and self-assembly of two-dimensional DNA crystals. Nature 1998 , 394 , 539–544. [ Google Scholar ] [ CrossRef ] [ PubMed ]
- Lin, C.; Nangreave, J.K.; Li, Z.; Liu, Y.; Yan, H. Signal amplification on a DNA-tile-based biosensor with enhanced sensitivity. Nanomed. 2008 , 3 , 521–528. [ Google Scholar ] [ CrossRef ] [ PubMed ]
- Rothemund, P.W.K. Design of DNA origami. In Proceedings of the ICCAD-2005, IEEE/ACM International Conference on Computer-Aided Design, San Jose, CA, USA, 6–10 November 2005; pp. 471–478. [ Google Scholar ] [ CrossRef ]
- Douglas, S.M.; Dietz, H.; Liedl, T.; Högberg, B.; Graf, F.; Shih, W.M. Self-assembly of DNA into nanoscale three-dimensional shapes. Nature 2009 , 459 , 414–418. [ Google Scholar ] [ CrossRef ] [ PubMed ]
- Pei, H.; Zuo, X.; Pan, D.; Shi, J.; Huang, Q.; Fan, C. Scaffolded biosensors with designed DNA nanostructures. NPG Asia Mater. 2013 , 5 , 51. [ Google Scholar ] [ CrossRef ]
- Han, S.; Liu, W.; Yang, S.; Wang, R. Facile and Label-Free Electrochemical Biosensors for MicroRNA Detection Based on DNA Origami Nanostructures. ACS Omega 2019 , 4 , 11025–11031. [ Google Scholar ] [ CrossRef ] [ PubMed ] [ Green Version ]
- Sameiyan, E.; Bagheri, E.; Ramezani, M.; Alibolandi, M.; Abnous, K.; Taghdisi, S.M. DNA origami-based aptasensors. Biosens. Bioelectron. 2019 , 143 , 111662. [ Google Scholar ] [ CrossRef ] [ PubMed ]
- Song, S.; Wang, L.; Li, J.; Fan, C.; Zhao, J. Aptamer-based biosensors. TrAC Trends Anal. Chem. 2008 , 27 , 108–117. [ Google Scholar ] [ CrossRef ]
- Gong, L.; Zhao, Z.; Lv, Y.-F.; Huan, S.-Y.; Fu, T.; Zhang, X.-B.; Shen, G.-L.; Yu, R.-Q. DNAzyme-based biosensors and nanodevices. Chem. Commun. 2014 , 51 , 979–995. [ Google Scholar ] [ CrossRef ]
- Zhang, C.; Chen, J.; Sun, R.; Huang, Z.; Luo, Z.; Zhou, C.; Wu, M.; Duan, Y.; Li, Y. The Recent Development of Hybridization Chain Reaction Strategies in Biosensors. ACS Sens. 2020 , 5 , 2977–3000. [ Google Scholar ] [ CrossRef ]
- Li, N.; Du, M.; Liu, Y.; Ji, X.; He, Z. Multipedal DNA Walker Biosensors Based on Catalyzed Hairpin Assembly and Isothermal Strand-Displacement Polymerase Reaction for the Chemiluminescent Detection of Proteins. ACS Sens. 2018 , 3 , 1283–1290. [ Google Scholar ] [ CrossRef ]
- Xie, N.; Liu, S.; Yang, X.; He, X.; Huang, J.; Wang, K. DNA tetrahedron nanostructures for biological applications: Biosensors and drug delivery. Analyst 2017 , 142 , 3322–3332. [ Google Scholar ] [ CrossRef ]
- Loretan, M.; Domljanovic, I.; Lakatos, M.; Rüegg, C.; Acuna, G.P. DNA Origami as Emerging Technology for the Engineering of Fluorescent and Plasmonic-Based Biosensors. Materials 2020 , 13 , 2185. [ Google Scholar ] [ CrossRef ]
- Murphy, L. Biosensors and bioelectrochemistry. Curr. Opin. Chem. Biol. 2006 , 10 , 177–184. [ Google Scholar ] [ CrossRef ]
- Songa, E.A.; Okonkwo, J.O. Recent approaches to improving selectivity and sensitivity of enzyme-based biosensors for organophosphorus pesticides: A review. Talanta 2016 , 155 , 289–304. [ Google Scholar ] [ CrossRef ]
- Long, F.; Zhu, A.; Shi, H. Recent Advances in Optical Biosensors for Environmental Monitoring and Early Warning. Sensors 2013 , 13 , 13928–13948. [ Google Scholar ] [ CrossRef ] [ PubMed ] [ Green Version ]
- Metkar, S.K.; Girigoswami, K. Diagnostic biosensors in medicine—A review. Biocatal. Agric. Biotechnol. 2019 , 17 , 271–283. [ Google Scholar ] [ CrossRef ]
- Hu, Q.; Wu, J.; Chen, L.; Lou, X.; Xia, F. Recent Development of DNA-modified AIEgen Probes for Biomedical Application. Chem. Res. Chin. Univ. 2021 , 37 , 66–72. [ Google Scholar ] [ CrossRef ]
- Mannelli, I.; Minunni, M.; Tombelli, S.; Wang, R.; Michela Spiriti, M.; Mascini, M. Direct immobilisation of DNA probes for the development of affinity biosensors. Bioelectrochemistry 2005 , 66 , 129–138. [ Google Scholar ] [ CrossRef ]
- Wang, J. Survey and summary: From DNA biosensors to gene chips. Nucleic Acids Res. 2000 , 28 , 3011–3016. [ Google Scholar ] [ CrossRef ] [ PubMed ]
- Canoura, J.; Yu, H.; Alkhamis, O.; Roncancio, D.; Farhana, R.; Xiao, Y. Accelerating Post-SELEX Aptamer Engineering Using Exonuclease Digestion. J. Am. Chem. Soc. 2021 , 143 , 805–816. [ Google Scholar ] [ CrossRef ]
- Zhuo, Z.; Yu, Y.; Wang, M.; Li, J.; Zhang, Z.; Liu, J.; Wu, X.; Lu, A.; Zhang, G.; Zhang, B. Recent Advances in SELEX Technology and Aptamer Applications in Biomedicine. Int. J. Mol. Sci. 2017 , 18 , 2142. [ Google Scholar ] [ CrossRef ] [ PubMed ] [ Green Version ]
- Ellington, A.D.; Szostak, J.W. In vitro selection of RNA molecules that bind specific ligands. Nature 1990 , 346 , 818–822. [ Google Scholar ] [ CrossRef ] [ PubMed ]
- Liu, Y.; Tuleouva, N.; Ramanculov, E.; Revzin, A. Aptamer-based electrochemical biosensor for interferon gamma detection. Anal. Chem. 2010 , 82 , 8131–8136. [ Google Scholar ] [ CrossRef ] [ PubMed ] [ Green Version ]
- Chen, Y.; Pui, T.S.; Kongsuphol, P.; Tang, K.C.; Arya, S.K. Aptamer-based array electrodes for quantitative interferon-γ detection. Biosens. Bioelectron. 2014 , 53 , 257–262. [ Google Scholar ] [ CrossRef ]
- Pan, H.M.; Gonuguntla, S.; Li, S.; Trau, D. 3.33 Conjugated Polymers for Biosensor Devices. In Comprehensive Biomaterials II ; Ducheyne, P., Ed.; Elsevier: Oxford, UK, 2017; pp. 716–754. ISBN 978-0-08-100692-4. [ Google Scholar ] [ CrossRef ]
- Kabanov, A.V.; Felgner, P.L.; Seymour, L.W. Self-assembling complexes for gene delivery. Lab. Clin. Trial 1998 , 197–218. [ Google Scholar ] [ CrossRef ]
- Ho, H.-A.; Boissinot, M.; Bergeron, M.G.; Corbeil, G.; Doré, K.; Boudreau, D.; Leclerc, M. Colorimetric and Fluorometric Detection of Nucleic Acids Using Cationic Polythiophene Derivatives. Angew. Chem. Int. Ed. 2002 , 41 , 1548–1551. [ Google Scholar ] [ CrossRef ]
- Ho, H.-A.; Leclerc, M. Optical Sensors Based on Hybrid Aptamer/Conjugated Polymer Complexes. J. Am. Chem. Soc. 2004 , 126 , 1384–1387. [ Google Scholar ] [ CrossRef ]
- Geim, A.K. Graphene: Status and Prospects. Science 2009 , 324 , 1530–1534. [ Google Scholar ] [ CrossRef ] [ Green Version ]
- Nag, A.; Mitra, A.; Mukhopadhyay, S.C. Graphene and Its Sensor-Based Applications: A Review. Sens. Actuators Phys. 2018 , 270 , 177–194. [ Google Scholar ] [ CrossRef ]
- Suvarnaphaet, P.; Pechprasarn, S. Graphene-Based Materials for Biosensors: A Review. Sensors 2017 , 17 , 2161. [ Google Scholar ] [ CrossRef ] [ PubMed ] [ Green Version ]
- Kim, J.; Park, S.-J.; Min, D.-H. Emerging Approaches for Graphene Oxide Biosensor. Anal. Chem. 2017 , 89 , 232–248. [ Google Scholar ] [ CrossRef ]
- Mukherjee, S.; Meshik, X.; Choi, M.; Farid, S.; Datta, D.; Lan, Y.; Poduri, S.; Sarkar, K.; Baterdene, U.; Huang, C.-E. A Graphene and Aptamer Based Liquid Gated FET-like Electrochemical Biosensor to Detect Adenosine Triphosphate. IEEE Trans. Nanobioscience 2015 , 14 , 967–972. [ Google Scholar ] [ CrossRef ] [ PubMed ]
- Wang, Y.; Li, Z.; Wang, J.; Li, J.; Lin, Y. Graphene and Graphene Oxide: Biofunctionalization and Applications in Biotechnology. Trends Biotechnol. 2011 , 29 , 205–212. [ Google Scholar ] [ CrossRef ] [ PubMed ]
- Liu, J. Adsorption of DNA onto Gold Nanoparticles and Graphene Oxide: Surface Science and Applications. Phys. Chem. Chem. Phys. 2012 , 14 , 10485–10496. [ Google Scholar ] [ CrossRef ] [ Green Version ]
- Yu, S.H.; Lee, C.-S.; Kim, T.H. Electrochemical Detection of Ultratrace Lead Ion through Attaching and Detaching DNA Aptamer from Electrochemically Reduced Graphene Oxide Electrode. Nanomaterials 2019 , 9 , 817. [ Google Scholar ] [ CrossRef ] [ PubMed ] [ Green Version ]
- Koshland, D.E. 78. Koshland, D.E. 7 The Molecular Basis for Enzyme Regulation. In The Enzymes ; Boyer, P.D., Ed.; Academic Press: Cambridge, MA, USA, 1970; Volume 1, pp. 341–396. [ Google Scholar ] [ CrossRef ]
- Hasegawa, H.; Savory, N.; Abe, K.; Ikebukuro, K. Methods for Improving Aptamer Binding Affinity. Molecules 2016 , 21 , 421. [ Google Scholar ] [ CrossRef ] [ PubMed ]
- Kang, D.; Vallée-Bélisle, A.; Porchetta, A.; Plaxco, K.W.; Ricci, F. Re-engineering Electrochemical Biosensors To Narrow or Extend Their Useful Dynamic Range. Angew. Chem. Int. Ed. 2012 , 51 , 6717–6721. [ Google Scholar ] [ CrossRef ] [ PubMed ] [ Green Version ]
- Satış, H.; Özger, H.S.; Aysert Yıldız, P.; Hızel, K.; Gulbahar, Ö.; Erbaş, G.; Aygencel, G.; Guzel Tunccan, O.; Öztürk, M.A.; Dizbay, M.; et al. Prognostic value of interleukin-18 and its association with other inflammatory markers and disease severity in COVID-19. Cytokine 2021 , 137 , 155302. [ Google Scholar ] [ CrossRef ]
- Pepe, M.S.; Etzioni, R.; Feng, Z.; Potter, J.D.; Thompson, M.L.; Thornquist, M.; Winget, M.; Yasui, Y. Phases of Biomarker Development for Early Detection of Cancer. JNCI J. Natl. Cancer Inst. 2001 , 93 , 1054–1061. [ Google Scholar ] [ CrossRef ] [ Green Version ]
- Lu, Y.; Li, X.; Zhang, L.; Yu, P.; Su, L.; Mao, L. Aptamer-Based Electrochemical Sensors with Aptamer−Complementary DNA Oligonucleotides as Probe. Anal. Chem. 2008 , 80 , 1883–1890. [ Google Scholar ] [ CrossRef ]
- Mena, M.L.; Yanez-Sedeno, P.; Pingarron, J.M. A comparison of different strategies for the construction of amperometric enzyme biosensors using gold nanoparticle-modified electrodes. Anal. Biochem. 2005 , 336 , 20–27. [ Google Scholar ] [ CrossRef ]
- Zhao, Z.; Lei, W.; Zhang, X.; Wang, B.; Jiang, H. ZnO-Based Amperometric Enzyme Biosensors. Sensors 2010 , 10 , 1216–1231. [ Google Scholar ] [ CrossRef ] [ PubMed ]
- Delvaux, M.; Demoustier-Champagne, S. Immobilisation of glucose oxidase within metallic nanotubes arrays for application to enzyme biosensors. Biosens. Bioelectron. 2003 , 18 , 943–951. [ Google Scholar ] [ CrossRef ]
- Wilson, G.S.; Hu, Y.B. Enzyme based biosensors for in vivo measurements. Chem. Rev. 2000 , 100 , 2693–2704. [ Google Scholar ] [ CrossRef ]
- Secundo, F. Conformational changes of enzymes upon immobilisation. Chem. Soc. Rev. 2013 , 42 , 6250–6261. [ Google Scholar ] [ CrossRef ] [ PubMed ]
- Jesionowski, T.; Zdarta, J.; Krajewska, B. Enzyme immobilization by adsorption: A review. Adsorpt.-J. Int. Adsorpt. Soc. 2014 , 20 , 801–821. [ Google Scholar ] [ CrossRef ] [ Green Version ]
- Kruger, K.; Grabowski, P.J.; Zaug, A.J.; Sands, J.; Gottschling, D.E.; Cech, T.R. Self-splicing RNA: Autoexcision and autocyclization of the ribosomal RNA intervening sequence of tetrahymena. Cell 1982 , 31 , 147–157. [ Google Scholar ] [ CrossRef ]
- Santoro, S.W.; Joyce, G.F. A general purpose RNA-cleaving DNA enzyme. Proc. Natl. Acad. Sci. USA 1997 , 94 , 4262–4266. [ Google Scholar ] [ CrossRef ] [ PubMed ] [ Green Version ]
- Schildkraut, C.; Lifson, S. Dependence of the melting temperature of DNA on salt concentration. Biopolymers 1965 , 3 , 195–208. [ Google Scholar ] [ CrossRef ] [ PubMed ]
- Panjkovich, A.; Melo, F. Comparison of different melting temperature calculation methods for short DNA sequences. Bioinformatics 2005 , 21 , 711–722. [ Google Scholar ] [ CrossRef ] [ PubMed ]
- Wu, P.; Nakano, S.; Sugimoto, N. Temperature dependence of thermodynamic properties for DNA/DNA and RNA/DNA duplex formation. Eur. J. Biochem. 2002 , 269 , 2821–2830. [ Google Scholar ] [ CrossRef ] [ Green Version ]
- Weber, G. Mesoscopic model parametrization of hydrogen bonds and stacking interactions of RNA from melting temperatures. Nucleic Acids Res. 2013 , 41 , 30. [ Google Scholar ] [ CrossRef ] [ Green Version ]
- Brunet, A.; Salomé, L.; Rousseau, P.; Destainville, N.; Manghi, M.; Tardin, C. How does temperature impact the conformation of single DNA molecules below melting temperature? Nucleic Acids Res. 2018 , 46 , 2074–2081. [ Google Scholar ] [ CrossRef ] [ Green Version ]
- Kim, H.-K.; Rasnik, I.; Liu, J.; Ha, T.; Lu, Y. Dissecting metal ion–dependent folding and catalysis of a single DNAzyme. Nat. Chem. Biol. 2007 , 3 , 763–768. [ Google Scholar ] [ CrossRef ]
- Zhang, X.-B.; Kong, R.-M.; Lu, Y. Metal Ion Sensors Based on DNAzymes and Related DNA Molecules. Annu. Rev. Anal. Chem. 2011 , 4 , 105–128. [ Google Scholar ] [ CrossRef ] [ Green Version ]
- Hwang, K.; Hosseinzadeh, P.; Lu, Y. Biochemical and biophysical understanding of metal ion selectivity of DNAzymes. Inorganica Chim. Acta 2016 , 452 , 12–24. [ Google Scholar ] [ CrossRef ] [ Green Version ]
- Roth, A.; Breaker, R.R. An amino acid as a cofactor for a catalytic polynucleotide. Proc. Natl. Acad. Sci. USA 1998 , 95 , 6027–6031. [ Google Scholar ] [ CrossRef ] [ Green Version ]
- Huang, K.; Martí, A.A. Recent trends in molecular beacon design and applications. Anal. Bioanal. Chem. 2012 , 402 , 3091–3102. [ Google Scholar ] [ CrossRef ]
- Li, J.; Lu, Y. A Highly Sensitive and Selective Catalytic DNA Biosensor for Lead Ions. J. Am. Chem. Soc. 2000 , 122 , 10466–10467. [ Google Scholar ] [ CrossRef ]
- Liu, J.; Lu, Y. Improving Fluorescent DNAzyme Biosensors by Combining Inter- and Intramolecular Quenchers. Anal. Chem. 2003 , 75 , 6666–6672. [ Google Scholar ] [ CrossRef ]
- Zhao, X.-H.; Gong, L.; Zhang, X.-B.; Yang, B.; Fu, T.; Hu, R.; Tan, W.; Yu, R. Versatile DNAzyme-Based Amplified Biosensing Platforms for Nucleic Acid, Protein, and Enzyme Activity Detection. Anal. Chem. 2013 , 85 , 3614–3620. [ Google Scholar ] [ CrossRef ]
- Travascio, P.; Li, Y.; Sen, D. DNA-enhanced peroxidase activity of a DNA aptamer-hemin complex. Chem. Biol. 1998 , 5 , 505–517. [ Google Scholar ] [ CrossRef ] [ Green Version ]
- Teller, C.; Shimron, S.; Willner, I. Aptamer-DNAzyme Hairpins for Amplified Biosensing. Anal. Chem. 2009 , 81 , 9114–9119. [ Google Scholar ] [ CrossRef ]
- Li, H.; Chang, J.; Hou, T.; Li, F. HRP-Mimicking DNAzyme-Catalyzed in Situ Generation of Polyaniline To Assist Signal Amplification for Ultrasensitive Surface Plasmon Resonance Biosensing. Anal. Chem. 2017 , 89 , 673–680. [ Google Scholar ] [ CrossRef ]
- Whetton, A.D.; Preston, G.W.; Abubeker, S.; Geifman, N. Proteomics and Informatics for Understanding Phases and Identifying Biomarkers in COVID-19 Disease. J. Proteome Res. 2020 , 19 , 4219–4232. [ Google Scholar ] [ CrossRef ]
- DeKosky, S.T.; Kochanek, P.M.; Valadka, A.B.; Clark, R.S.B.; Chou, S.H.-Y.; Au, A.K.; Horvat, C.; Jha, R.M.; Mannix, R.; Wisniewski, S.R.; et al. Blood Biomarkers for Detection of Brain Injury in COVID-19 Patients. J. Neurotrauma 2020 , 38 , 1–43. [ Google Scholar ] [ CrossRef ]
- Kaur, M.; Tiwari, S.; Jain, R. Protein based biomarkers for non-invasive Covid-19 detection. Sens. Bio-Sens. Res. 2020 , 29 , 100362. [ Google Scholar ] [ CrossRef ]
- Bartlett, J.M.S.; Stirling, D. A Short History of the Polymerase Chain Reaction. In PCR Protocols ; Bartlett, J.M.S., Stirling, D., Eds.; Methods in Molecular Biology ™ ; Humana Press: Totowa, NJ, USA, 2003; pp. 3–6. ISBN 978-1-59259-384-2. [ Google Scholar ] [ CrossRef ]
- Kubista, M.; Andrade, J.M.; Bengtsson, M.; Forootan, A.; Jonák, J.; Lind, K.; Sindelka, R.; Sjöback, R.; Sjögreen, B.; Strömbom, L.; et al. The real-time polymerase chain reaction. Mol. Aspects Med. 2006 , 27 , 95–125. [ Google Scholar ] [ CrossRef ]
- Tomita, N.; Mori, Y.; Kanda, H.; Notomi, T. Loop-mediated isothermal amplification (LAMP) of gene sequences and simple visual detection of products. Nat. Protoc. 2008 , 3 , 877–882. [ Google Scholar ] [ CrossRef ]
- Hacia, J.G. Resequencing and mutational analysis using oligonucleotide microarrays. Nat. Genet. 1999 , 21 , 42–47. [ Google Scholar ] [ CrossRef ]
- Lequin, R.M. Enzyme Immunoassay (EIA)/Enzyme-Linked Immunosorbent Assay (ELISA). Clin. Chem. 2005 , 51 , 2415–2418. [ Google Scholar ] [ CrossRef ] [ Green Version ]
- Fang, X.; Liu, X.; Schuster, S.; Tan, W. Designing a Novel Molecular Beacon for Surface-Immobilized DNA Hybridization Studies. J. Am. Chem. Soc. 1999 , 121 , 2921–2922. [ Google Scholar ] [ CrossRef ]
- Fan, C.; Plaxco, K.W.; Heeger, A.J. Electrochemical interrogation of conformational changes as a reagentless method for the sequence-specific detection of DNA. Proc. Natl. Acad. Sci. USA 2003 , 100 , 9134–9137. [ Google Scholar ] [ CrossRef ] [ Green Version ]
- Rowe, A.A.; Chuh, K.N.; Lubin, A.A.; Miller, E.A.; Cook, B.; Hollis, D.; Plaxco, K.W. Electrochemical Biosensors Employing an Internal Electrode Attachment Site and Achieving Reversible, High Gain Detection of Specific Nucleic Acid Sequences. Anal. Chem. 2011 , 83 , 9462–9466. [ Google Scholar ] [ CrossRef ] [ Green Version ]
- Xiong, E.; Li, Z.; Zhang, X.; Zhou, J.; Yan, X.; Liu, Y.; Chen, J. Triple-Helix Molecular Switch Electrochemical Ratiometric Biosensor for Ultrasensitive Detection of Nucleic Acids. Anal. Chem. 2017 , 89 , 8830–8835. [ Google Scholar ] [ CrossRef ]
- Peterson, A.W.; Heaton, R.J.; Georgiadis, R.M. The effect of surface probe density on DNA hybridization. Nucleic Acids Res. 2001 , 29 , 5163–5168. [ Google Scholar ] [ CrossRef ] [ Green Version ]
- Hou, T.; Li, W.; Liu, X.; Li, F. Label-Free and Enzyme-Free Homogeneous Electrochemical Biosensing Strategy Based on Hybridization Chain Reaction: A Facile, Sensitive, and Highly Specific MicroRNA Assay. Anal. Chem. 2015 , 87 , 11368–11374. [ Google Scholar ] [ CrossRef ]
- Chen, Z.; Chengjun, S.; Zewei, L.; Kunping, L.; Xijian, Y.; Haimin, Z.; Yongxin, L.; Yixiang, D. Fiber optic biosensor for detection of genetically modified food based on catalytic hairpin assembly reaction and nanocomposites assisted signal amplification. Sens. Actuators B Chem. 2018 , 254 , 956–965. [ Google Scholar ] [ CrossRef ]
- Liu, Y.; Li, S.; Zhang, L.; Zhao, Q.; Li, N.; Wu, Y. Catalytic Hairpin Assembly-Assisted Rolling Circle Amplification for High-Sensitive Telomerase Activity Detection. ACS Omega 2020 , 5 , 11836–11841. [ Google Scholar ] [ CrossRef ]
- Chan, V.; Graves, D.J.; McKenzie, S.E. The biophysics of DNA hybridization with immobilized oligonucleotide probes. Biophys. J. 1995 , 69 , 2243–2255. [ Google Scholar ] [ CrossRef ] [ Green Version ]
- Ouldridge, T.E.; Šulc, P.; Romano, F.; Doye, J.P.K.; Louis, A.A. DNA hybridization kinetics: Zippering, internal displacement and sequence dependence. Nucleic Acids Res. 2013 , 41 , 8886–8895. [ Google Scholar ] [ CrossRef ] [ Green Version ]
- Liu, Y.; Kumar, S.; Taylor, R.E. Mix-and-match nanobiosensor design: Logical and spatial programming of biosensors using self-assembled DNA nanostructures. WIREs Nanomed. Nanobiotechnol. 2018 , 10 , e1518. [ Google Scholar ] [ CrossRef ] [ PubMed ]
- Fan, S.; Cheng, J.; Liu, Y.; Wang, D.; Luo, T.; Dai, B.; Zhang, C.; Cui, D.; Ke, Y.; Song, J. Proximity-Induced Pattern Operations in Reconfigurable DNA Origami Domino Array. J. Am. Chem. Soc. 2020 , 142 , 14566–14573. [ Google Scholar ] [ CrossRef ] [ PubMed ]
- Kallenbach, N.R.; Ma, R.-I.; Seeman, N.C. An immobile nucleic acid junction constructed from oligonucleotides. Nature 1983 , 305 , 829–831. [ Google Scholar ] [ CrossRef ]
- Liu, Y.; West, S.C. Happy Hollidays: 40th anniversary of the Holliday junction. Nat. Rev. Mol. Cell Biol. 2004 , 5 , 937–944. [ Google Scholar ] [ CrossRef ] [ PubMed ]
- Mao, C.; Sun, W.; Seeman, N.C. Designed Two-Dimensional DNA Holliday Junction Arrays Visualized by Atomic Force Microscopy. J. Am. Chem. Soc. 1999 , 121 , 5437–5443. [ Google Scholar ] [ CrossRef ]
- Du, S.M.; Seeman, N.C. The construction of a trefoil knot from a DNA branched junction motif. Biopolymers 1994 , 34 , 31–37. [ Google Scholar ] [ CrossRef ] [ PubMed ]
- Qi, J.; Li, X.; Yang, X.; Seeman, N.C. Ligation of Triangles Built from Bulged 3-Arm DNA Branched Junctions. J. Am. Chem. Soc. 1996 , 118 , 6121–6130. [ Google Scholar ] [ CrossRef ]
- Lin, C.; Liu, Y.; Rinker, S.; Yan, H. DNA Tile Based Self-Assembly: Building Complex Nanoarchitectures. Chem. Phys. Chem. 2006 , 7 , 1641–1647. [ Google Scholar ] [ CrossRef ]
- Kim, S.; Kim, J.; Qian, P.; Shin, J.; Amin, R.; Ahn, S.J.; LaBean, T.H.; Kim, M.K.; Park, S.H. Intrinsic DNA curvature of double-crossover tiles. Nanotechnology 2011 , 22 , 245706. [ Google Scholar ] [ CrossRef ] [ Green Version ]
- Sa-Ardyen, P.; Vologodskii, A.V.; Seeman, N.C. The Flexibility of DNA Double Crossover Molecules. Biophys. J. 2003 , 84 , 3829–3837. [ Google Scholar ] [ CrossRef ] [ Green Version ]
- Mao, C.; LaBean, T.H.; Reif, J.H.; Seeman, N.C. Logical computation using algorithmic self-assembly of DNA triple-crossover molecules. Nature 2000 , 407 , 493–496. [ Google Scholar ] [ CrossRef ]
- Liu, D.; Park, S.H.; Reif, J.H.; LaBean, T.H. DNA nanotubes self-assembled from triple-crossover tiles as templates for conductive nanowires. Proc. Natl. Acad. Sci. USA 2004 , 101 , 717–722. [ Google Scholar ] [ CrossRef ] [ PubMed ] [ Green Version ]
- Yan, H.; Yin, P.; Park, S.H.; Li, H.; Feng, L.; Guan, X.; Liu, D.; Reif, J.H.; LaBean, T.H. Self-assembled DNA Structures for Nanoconstruction. AIP Conf. Proc. 2004 , 725 , 43–52. [ Google Scholar ] [ CrossRef ]
- He, Y.; Ye, T.; Su, M.; Zhang, C.; Ribbe, A.E.; Jiang, W.; Mao, C. Hierarchical self-assembly of DNA into symmetric supramolecular polyhedra. Nature 2008 , 452 , 198–201. [ Google Scholar ] [ CrossRef ]
- Zhang, C.; Su, M.; He, Y.; Zhao, X.; Fang, P.; Ribbe, A.E.; Jiang, W.; Mao, C. Conformational flexibility facilitates self-assembly of complex DNA nanostructures. Proc. Natl. Acad. Sci. USA 2008 , 105 , 10665–10669. [ Google Scholar ] [ CrossRef ] [ PubMed ] [ Green Version ]
- Goodman, R.P.; Berry, R.M.; Turberfield, A.J. The Single-Step Synthesis of a DNA Tetrahedron. Chem. Commun. 2004 , 1372–1373. [ Google Scholar ] [ CrossRef ]
- Goodman, R.P.; Heilemann, M.; Doose, S.; Erben, C.M.; Kapanidis, A.N.; Turberfield, A.J. Reconfigurable, Braced, Three-Dimensional DNA Nanostructures. Nat. Nanotechnol. 2008 , 3 , 93–96. [ Google Scholar ] [ CrossRef ] [ PubMed ]
- Aldaye, F.A.; Sleiman, H.F. Modular Access to Structurally Switchable 3D Discrete DNA Assemblies. J. Am. Chem. Soc. 2007 , 129 , 13376–13377. [ Google Scholar ] [ CrossRef ] [ PubMed ]
- Zhou, Z.; Sohn, Y.S.; Nechushtai, R.; Willner, I. DNA Tetrahedra Modules as Versatile Optical Sensing Platforms for Multiplexed Analysis of miRNAs, Endonucleases, and Aptamer–Ligand Complexes. ACS Nano 2020 , 14 , 9021–9031. [ Google Scholar ] [ CrossRef ]
- Li, J.; Dai, J.; Jiang, S.; Xie, M.; Zhai, T.; Guo, L.; Cao, S.; Xing, S.; Qu, Z.; Zhao, Y.; et al. Encoding quantized fluorescence states with fractal DNA frameworks. Nat. Commun. 2020 , 11 , 2185. [ Google Scholar ] [ CrossRef ]
- Fan, Z.; Lin, Z.; Wang, Z.; Wang, J.; Xie, M.; Zhao, J.; Zhang, K.; Huang, W. Dual-Wavelength Electrochemiluminescence Ratiometric Biosensor for NF-κB p50 Detection with Dimethylthiodiaminoterephthalate Fluorophore and Self-Assembled DNA Tetrahedron Nanostructures Probe. ACS Appl. Mater. Interfaces 2020 , 12 , 11409–11418. [ Google Scholar ] [ CrossRef ] [ PubMed ]
- Song, P.; Shen, J.; Ye, D.; Dong, B.; Wang, F.; Pei, H.; Wang, J.; Shi, J.; Wang, L.; Xue, W.; et al. Programming bulk enzyme heterojunctions for biosensor development with tetrahedral DNA framework. Nat. Commun. 2020 , 11 , 838. [ Google Scholar ] [ CrossRef ] [ PubMed ] [ Green Version ]
- Wang, S.; Dong, Y.; Liang, X. Development of a SPR aptasensor containing oriented aptamer for direct capture and detection of tetracycline in multiple honey samples. Biosens. Bioelectron. 2018 , 109 , 1–7. [ Google Scholar ] [ CrossRef ] [ PubMed ]
- Nie, W.; Wang, Q.; Zou, L.; Zheng, Y.; Liu, X.; Yang, X.; Wang, K. Low-Fouling Surface Plasmon Resonance Sensor for Highly Sensitive Detection of MicroRNA in a Complex Matrix Based on the DNA Tetrahedron. Anal. Chem. 2018 , 90 , 12584–12591. [ Google Scholar ] [ CrossRef ] [ PubMed ]
- Diao, W.; Tang, M.; Ding, S.; Li, X.; Cheng, W.; Mo, F.; Yan, X.; Ma, H.; Yan, Y. Highly sensitive surface plasmon resonance biosensor for the detection of HIV-related DNA based on dynamic and structural DNA nanodevices. Biosens. Bioelectron. 2018 , 100 , 228–234. [ Google Scholar ] [ CrossRef ] [ PubMed ]
- Huang, R.; He, N.; Li, Z. Recent progresses in DNA nanostructure-based biosensors for detection of tumor markers. Biosens. Bioelectron. 2018 , 109 , 27–34. [ Google Scholar ] [ CrossRef ] [ PubMed ]
- Li, F.; Li, Q.; Zuo, X.; Fan, C. DNA framework-engineered electrochemical biosensors. Sci. China Life Sci. 2020 , 63 , 1130–1141. [ Google Scholar ] [ CrossRef ] [ PubMed ]
- Chidchob, P.; Sleiman, H.F. Recent advances in DNA nanotechnology. Curr. Opin. Chem. Biol. 2018 , 46 , 63–70. [ Google Scholar ] [ CrossRef ] [ PubMed ]
- Bu, N.-N.; Tang, C.-X.; He, X.-W.; Yin, X.-B. Tetrahedron-structured DNA and functional oligonucleotide for construction of an electrochemical DNA-based biosensor. Chem. Commun. 2011 , 47 , 7689–7691. [ Google Scholar ] [ CrossRef ]
- Li, M.; Xiong, C.; Zheng, Y.; Liang, W.; Yuan, R.; Chai, Y. Ultrasensitive Photoelectrochemical Biosensor Based on DNA Tetrahedron as Nanocarrier for Efficient Immobilization of CdTe QDs-Methylene Blue as Signal Probe with Near-Zero Background Noise. Anal. Chem. 2018 , 90 , 8211–8216. [ Google Scholar ] [ CrossRef ]
- Su, J.; Wu, F.; Xia, H.; Wu, Y.; Liu, S. Accurate cancer cell identification and microRNA silencing induced therapy using tailored DNA tetrahedron nanostructures. Chem. Sci. 2020 , 11 , 80–86. [ Google Scholar ] [ CrossRef ] [ PubMed ]
- Han, X.; Jiang, Y.; Li, S.; Zhang, Y.; Ma, X.; Wu, Z.; Wu, Z.; Qi, X. Multivalent aptamer-modified tetrahedral DNA nanocage demonstrates high selectivity and safety for anti-tumor therapy. Nanoscale 2019 , 11 , 339–347. [ Google Scholar ] [ CrossRef ] [ PubMed ]
- Bhuckory, S.; Kays, J.C.; Dennis, A.M. In Vivo Biosensing Using Resonance Energy Transfer. Biosensors 2019 , 9 , 76. [ Google Scholar ] [ CrossRef ] [ PubMed ] [ Green Version ]
- Rothemund, P.W.K. Folding DNA to create nanoscale shapes and patterns. Nature 2006 , 440 , 297–302. [ Google Scholar ] [ CrossRef ] [ PubMed ] [ Green Version ]
- Daems, D.; Pfeifer, W.; Rutten, I.; Saccà, B.; Spasic, D.; Lammertyn, J. Three-dimensional DNA origami as programmable anchoring points for bioreceptors in fiber optic surface plasmon resonance biosensing. ACS Appl. Mater. Interfaces 2018 , 10 , 23539–23547. [ Google Scholar ] [ CrossRef ] [ PubMed ]
- Rutten, I.; Daems, D.; Lammertyn, J. Boosting biomolecular interactions through DNA origami nano-tailored biosensing interfaces. J. Mater. Chem. B 2020 , 8 , 3606–3615. [ Google Scholar ] [ CrossRef ] [ PubMed ]
- Kong, G.; Xiong, M.; Liu, L.; Hu, L.; Meng, H.-M.; Ke, G.; Zhang, X.-B.; Tan, W. DNA origami-based protein networks: From basic construction to emerging applications. Chem. Soc. Rev. 2021 , 50 , 1846–1873. [ Google Scholar ] [ CrossRef ] [ PubMed ]
- Ge, Z.; Fu, J.; Liu, M.; Jiang, S.; Andreoni, A.; Zuo, X.; Liu, Y.; Yan, H.; Fan, C. Constructing Submonolayer DNA Origami Scaffold on Gold Electrode for Wiring of Redox Enzymatic Cascade Pathways. ACS Appl. Mater. Interfaces 2019 , 11 , 13881–13887. [ Google Scholar ] [ CrossRef ]
- Prodan, E.; Radloff, C.; Halas, N.J.; Nordlander, P. A Hybridization Model for the Plasmon Response of Complex Nanostructures. Science 2003 , 302 , 419–422. [ Google Scholar ] [ CrossRef ]
- Ding, S.-Y.; Yi, J.; Li, J.-F.; Ren, B.; Wu, D.-Y.; Panneerselvam, R.; Tian, Z.-Q. Nanostructure-based plasmon-enhanced Raman spectroscopy for surface analysis of materials. Nat. Rev. Mater. 2016 , 1 , 16021. [ Google Scholar ] [ CrossRef ]
- Jiang, R.; Li, B.; Fang, C.; Wang, J. Metal/Semiconductor Hybrid Nanostructures for Plasmon-Enhanced Applications. Adv. Mater. 2014 , 26 , 5274–5309. [ Google Scholar ] [ CrossRef ] [ PubMed ]
- Ming, T.; Chen, H.; Jiang, R.; Li, Q.; Wang, J. Plasmon-Controlled Fluorescence: Beyond the Intensity Enhancement. J. Phys. Chem. Lett. 2012 , 3 , 191–202. [ Google Scholar ] [ CrossRef ]
- Wiley, B.J.; Im, S.H.; Li, Z.-Y.; McLellan, J.; Siekkinen, A.; Xia, Y. Maneuvering the Surface Plasmon Resonance of Silver Nanostructures through Shape-Controlled Synthesis. J. Phys. Chem. B 2006 , 110 , 15666–15675. [ Google Scholar ] [ CrossRef ] [ PubMed ]
- Shaukat, A.; Anaya-Plaza, E.; Julin, S.; Linko, V.; Torres, T.; de la Escosura, A.; Kostiainen, M.A. Phthalocyanine–DNA origami complexes with enhanced stability and optical properties. Chem. Commun. 2020 , 56 , 7341–7344. [ Google Scholar ] [ CrossRef ] [ PubMed ]
- Wang, X.; Li, C.; Niu, D.; Sha, R.; Seeman, N.C.; Canary, J.W. Construction of a DNA Origami Based Molecular Electro-optical Modulator. Nano Lett. 2018 , 18 , 2112–2115. [ Google Scholar ] [ CrossRef ]
- Kuzyk, A.; Jungmann, R.; Acuna, G.P.; Liu, N. DNA Origami Route for Nanophotonics. ACS Photonics 2018 , 5 , 1151–1163. [ Google Scholar ] [ CrossRef ] [ PubMed ] [ Green Version ]
- Urban, M.J.; Dutta, P.K.; Wang, P.; Duan, X.; Shen, X.; Ding, B.; Ke, Y.; Liu, N. Plasmonic Toroidal Metamolecules Assembled by DNA Origami. J. Am. Chem. Soc. 2016 , 138 , 5495–5498. [ Google Scholar ] [ CrossRef ] [ PubMed ] [ Green Version ]
- Kuzyk, A.; Schreiber, R.; Zhang, H.; Govorov, A.O.; Liedl, T.; Liu, N. Reconfigurable 3D plasmonic metamolecules. Nat. Mater. 2014 , 13 , 862–866. [ Google Scholar ] [ CrossRef ] [ PubMed ] [ Green Version ]
- Hübner, K.; Pilo-Pais, M.; Selbach, F.; Liedl, T.; Tinnefeld, P.; Stefani, F.D.; Acuna, G.P. Directing Single-Molecule Emission with DNA Origami-Assembled Optical Antennas. Nano Lett. 2019 , 19 , 6629–6634. [ Google Scholar ] [ CrossRef ] [ Green Version ]
- Vogele, K.; List, J.; Pardatscher, G.; Holland, N.B.; Simmel, F.C.; Pirzer, T. Self-Assembled Active Plasmonic Waveguide with a Peptide-Based Thermomechanical Switch. ACS Nano 2016 , 10 , 11377–11384. [ Google Scholar ] [ CrossRef ] [ PubMed ]
- Prinz, J.; Schreiber, B.; Olejko, L.; Oertel, J.; Rackwitz, J.; Keller, A.; Bald, I. DNA Origami Substrates for Highly Sensitive Surface-Enhanced Raman Scattering. J. Phys. Chem. Lett. 2013 , 4 , 4140–4145. [ Google Scholar ] [ CrossRef ]
- Puchkova, A.; Vietz, C.; Pibiri, E.; Wünsch, B.; Sanz Paz, M.; Acuna, G.P.; Tinnefeld, P. DNA Origami Nanoantennas with over 5000-fold Fluorescence Enhancement and Single-Molecule Detection at 25 μM. Nano Lett. 2015 , 15 , 8354–8359. [ Google Scholar ] [ CrossRef ] [ PubMed ]
- Ijäs, H.; Nummelin, S.; Shen, B.; Kostiainen, M.A.; Linko, V. Dynamic DNA Origami Devices: From Strand-Displacement Reactions to External-Stimuli Responsive Systems. Int. J. Mol. Sci. 2018 , 19 , 2114. [ Google Scholar ] [ CrossRef ] [ PubMed ] [ Green Version ]
- Grossi, G.; Dalgaard Ebbesen Jepsen, M.; Kjems, J.; Andersen, E.S. Control of enzyme reactions by a reconfigurable DNA nanovault. Nat. Commun. 2017 , 8 , 992. [ Google Scholar ] [ CrossRef ] [ PubMed ] [ Green Version ]
- Kopperger, E.; List, J.; Madhira, S.; Rothfischer, F.; Lamb, D.C.; Simmel, F.C. A self-assembled nanoscale robotic arm controlled by electric fields. Science 2018 , 359 , 296–301. [ Google Scholar ] [ CrossRef ] [ Green Version ]
- Kuzyk, A.; Yang, Y.; Duan, X.; Stoll, S.; Govorov, A.O.; Sugiyama, H.; Endo, M.; Liu, N. A light-driven three-dimensional plasmonic nanosystem that translates molecular motion into reversible chiroptical function. Nat. Commun. 2016 , 7 , 10591. [ Google Scholar ] [ CrossRef ]
- Funck, T.; Liedl, T.; Bae, W. Dual Aptamer-Functionalized 3D Plasmonic Metamolecule for Thrombin Sensing. Appl. Sci. 2019 , 9 , 3006. [ Google Scholar ] [ CrossRef ] [ Green Version ]
- Lan, X.; Chen, Z.; Dai, G.; Lu, X.; Ni, W.; Wang, Q. Bifacial DNA Origami-Directed Discrete, Three-Dimensional, Anisotropic Plasmonic Nanoarchitectures with Tailored Optical Chirality. J. Am. Chem. Soc. 2013 , 135 , 11441–11444. [ Google Scholar ] [ CrossRef ] [ PubMed ]
- Chandrasekaran, A.R.; Pushpanathan, M.; Halvorsen, K. Evolution of DNA origami scaffolds. Mater. Lett. 2016 , 170 , 221–224. [ Google Scholar ] [ CrossRef ]
- Pound, E.; Ashton, J.R.; Becerril, H.A.; Woolley, A.T. Polymerase Chain Reaction Based Scaffold Preparation for the Production of Thin, Branched DNA Origami Nanostructures of Arbitrary Sizes. Nano Lett. 2009 , 9 , 4302–4305. [ Google Scholar ] [ CrossRef ]
- Liu, W.; Zhong, H.; Wang, R.; Seeman, N.C. Crystalline Two-Dimensional DNA-Origami Arrays. Angew. Chem. Int. Ed. 2011 , 50 , 264–267. [ Google Scholar ] [ CrossRef ] [ PubMed ] [ Green Version ]
- Yao, G.; Zhang, F.; Wang, F.; Peng, T.; Liu, H.; Poppleton, E.; Šulc, P.; Jiang, S.; Liu, L.; Gong, C.; et al. Meta-DNA structures. Nat. Chem. 2020 , 12 , 1067–1075. [ Google Scholar ] [ CrossRef ] [ PubMed ]
- Fu, Y.; Zeng, D.; Chao, J.; Jin, Y.; Zhang, Z.; Liu, H.; Li, D.; Ma, H.; Huang, Q.; Gothelf, K.V.; et al. Single-Step Rapid Assembly of DNA Origami Nanostructures for Addressable Nanoscale Bioreactors. J. Am. Chem. Soc. 2013 , 135 , 696–702. [ Google Scholar ] [ CrossRef ] [ PubMed ]
Category | Advantages | Disadvantages | Detection Object | |
---|---|---|---|---|
Functional DNA strand-based biosensors | DNA aptamer | Easily accessed; easily modified; adjustable affinity; more economic; more durable lifetime | Requires multi-round selection; easily attacked by the nucleic enzyme; potential biotoxicity | IFN-γ [ , ] |
Pb [ ] | ||||
Thrombin [ , ] | ||||
DNAzyme | High catalytic activity; small molecule detection with high sensitivity | Easily affected by temperature; needs oxidative substrate; the reaction product cannot be recycled to use | Pb [ ] | |
AMP, Lyso [ ] | ||||
Bleomycin [ ] | ||||
DNA hybridization-based biosensors | DNA hairpin | Detects nucleic acids with high selectivity; easily converts the hybridization process into physical signal change | Easily damaged by temperature | DNA [ , , ] |
HCR | High sensitivity, especially at the biosensing interface | Easily be triggered automatically by mistake | micro-RNA [ ] | |
CHA | More stable than HCR | Not as sensitive as HCR | micro-RNA [ ] | |
DNA template-based biosensors | DNA tiles | Effectively adjust the surface density of bioprobes; suitable for in vivo biosensing | Lack of the ability to form complex and large-scale patterns | mi-RNA 141 [ ] |
DNA origami | Control the arrange of bioprobes and materials with nanoscale accuracy; programmable nanostructure | Time-consuming annealing process; expensive; difficult to design | Oligonucleotides [ ] | |
Thrombin [ , ] |
MDPI stays neutral with regard to jurisdictional claims in published maps and institutional affiliations. |
Share and Cite
Hua, Y.; Ma, J.; Li, D.; Wang, R. DNA-Based Biosensors for the Biochemical Analysis: A Review. Biosensors 2022 , 12 , 183. https://doi.org/10.3390/bios12030183
Hua Y, Ma J, Li D, Wang R. DNA-Based Biosensors for the Biochemical Analysis: A Review. Biosensors . 2022; 12(3):183. https://doi.org/10.3390/bios12030183
Hua, Yu, Jiaming Ma, Dachao Li, and Ridong Wang. 2022. "DNA-Based Biosensors for the Biochemical Analysis: A Review" Biosensors 12, no. 3: 183. https://doi.org/10.3390/bios12030183
Article Metrics
Article access statistics, further information, mdpi initiatives, follow mdpi.
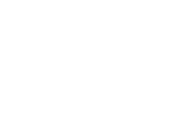
Subscribe to receive issue release notifications and newsletters from MDPI journals

An official website of the United States government
The .gov means it’s official. Federal government websites often end in .gov or .mil. Before sharing sensitive information, make sure you’re on a federal government site.
The site is secure. The https:// ensures that you are connecting to the official website and that any information you provide is encrypted and transmitted securely.
- Publications
- Account settings
Preview improvements coming to the PMC website in October 2024. Learn More or Try it out now .
- Advanced Search
- Journal List
- J Family Med Prim Care
- v.9(8); 2020 Aug
Comparison of test performance of biochemical parameters in semiautomatic method and fully automatic analyzer method
Suchitra kumari.
1 Department of Biochemistry, All India Institute of Medical Sciences (AIIMS), Bhubaneswar, Odisha, India
Jyotirmayee Bahinipati
2 Department of Biochemistry, Kalinga Institute of Medical Sciences (KIMS), Bhubaneswar, Odisha, India
Tapaswini Pradhan
Durgesh p. sahoo.
3 Department of CMFM, All India Institute of Medical Sciences (AIIMS), Bhubaneswar, Odisha, India
Background:
The primary health-care center (PHC) and community health center (CHC) are not well equipped with laboratory services. Semiauto analyzer-based reporting could be an effective modality, provided that the performance standard is comparable to that of the fully automatic analyzer. So, the objective of this study was to analyze the test results of biochemical parameters in semiauto and fully automatic analyzer and to compare the quality performance.
Materials and Methods:
One hundred forty-nine patients undergoing routine biochemical investigations in the department laboratory were enrolled in this study. Two millimeter of venous blood was collected from all the participants and processed for urea, cholesterol, triglyceride (TG), serum glutamate-oxaloacetate transaminase (SGOT) (aspartate aminotransferase), and serum glutamate-pyruvate transaminase (SGPT) (alanine aminotransferase) by using standard kits (ERBA) in semiauto analyzer (Transasia Erba Chem5X by Calbiotech Inc. USA, semiautomated clinical chemistry analyzer) and the fully automatic analyzer (Cobas Integra 400 Roche, Germany) method.
There was high variability in the distribution of urea, TG, SGOT, and SGPT values in both measurement methods, whereas cholesterol data followed a normal distribution (skewness: 1.522, 1.037; kurtosis: 2.373, 0.693 in semiauto and automated methods, respectively). A significant positive correlation between both the methods of assessment was observed in urea, cholesterol, TGs, SGOT, and SGPT. The mean difference for urea was –9.85 ± 23.997 (LOA: 37.189, –56.88), whereas it was highest for TG –24.34 ± 38.513 (LOA: 51.144, –99.829), suggesting that both methods can measure urea with less difference in absolute values, whereas for TG the measurement values are highly variable.
Conclusion:
The test performance of biochemical parameters such as urea, total cholesterol, TGs, SGOT, and SGPT taken by semiauto analyzer and fully automatic analyzer method of assessment were highly related and comparable.
Introduction
To ensure the reliability and accuracy of test results, the quality assurance is of paramount importance to provide the best possible patient care. The health outcomes depend on the accuracy of the testing and reporting as nowadays these test results are widely used in clinical and public health setups.[ 1 ] Hence, it is important to assure good laboratory performance by analyzing the complexity that involves many steps of activity and many people along with different laboratory processes and procedures.[ 2 ]
Clinical biochemistry is the most predominant diagnostic services in the field of laboratory Medicine and clinical medicine. It involves the measurement of substances in body fluids especially in the blood for the purpose of diagnosis, prevention, or treatment of disease.[ 3 ] These biochemical quantitative investigations give an accurate measure of extent of disease progression and hence help in greater understanding of the disease process. Reliability of the test performance depends on its accuracy, precision, specificity, and sensitivity of which precision and accuracy of the performance of analytical methods are the key measure of the quality performance.[ 4 ] Precision is measured by repeating the test run and it represents the reproducibility of an analytical method; accuracy being another important dimension defines how close the measured value is to the actual value.[ 5 ] The precision and accuracy of the test parameters should be acceptable for every biochemical method.[ 6 ] Specificity refers to the ability of an analytical method to determine solely the parameter of interest and sensitivity is the ability to detect even small quantities of the measured analyte.[ 7 ] Bias and imprecision are the major determinants of the test performance. Bias, an analytical characteristic, represents how the reported results differ from the actual value, whereas imprecision, or lack of reproducibility, is due to both physiological and analytical factors.[ 8 ] A number of factors broadly divided as preanalytical and analytical affect these determinants of quality performance. Deviation would result in laboratory errors, that is, use of unstable/deteriorated calibrators, unstable reagent blanks, error in calibration, or impure calibration material resulting in inadequate control on analytical variables causing systemic errors and inadequate control on preanalytical variables such as patient identity, sample collection and labeling, handling and transport, and fault in measuring devices causing the random errors.[ 9 ] Manual methods, semiauto analyzers, and fully automatic analyzer-based methods are in use in the Biochemistry laboratories. There has been a considerable increase in the demand of the biochemical parameters in clinical practice. To maintain the turnaround time and to meet the huge clinical need, mono-step methods (automated methods) are introduced to replace multistep cumbersome methods (Manual methods). Mono-step method using fully automatic chemistry analyzers performs many tests with the least manual involvements. The function of auto analyzer is to replace with automated devices the steps of pipetting and increase the accuracy and precision of the methods. Automation leads to reduction in variability of results and error of analysis as compared to manual means.[ 10 ]
Analytical methods used are the mainstay of the accuracy of the test results. Automation attains improved reproducibility but not improved accuracy. Hence, this study was conducted to analyze the test results of biochemical parameters in semiauto analyzer and fully automatic analyzer method and to compare the quality performance of the respective methodologies.
Materials and Methods
This was a hospital-based cross-sectional study conducted in the Department of Biochemistry from February 2017 to September 2019. One hundred forty-nine patients undergoing routine biochemical investigations in the department laboratory were enrolled in this study. After obtaining informed written consent, 2 mL of venous blood was collected from all the participants and processed for the biochemical analysis. The biochemical parameters such as urea, cholesterol, triglyceride (TG), serum glutamate-oxaloacetate transaminase (SGOT) (aspartate aminotransferase [AST]), and serum glutamate-pyruvate transaminase (SGPT) (alanine aminotransferase [ALT]) were estimated from the serum sample by using standard kits (ERBA) in semiauto analyzer (Transasia, Erba Chem5X, semiautomated clinical chemistry analyzer) and the fully automatic analyzer (Cobas Integra 400 Roche) method.
Estimation of urea by urease method (semiauto analyzer)
The reagent used contains: urease, glutamate dehydrogenase (GLDH), nicotinamide adenine dinucleotide (NADH), α-ketoglutaric acid, buffers, and stabilizers.

The rate of oxidation of NADH to NAD was measured at 340 nm by semiauto analyzer and was proportional to the urea concentration.[ 11 ]
Estimation of urea by full automatic analyzer (kinetic ureases and glutamate dehydrogenase method)
The serum sample was used to estimate the urea levels by full automatic analyzer. In the reaction, urea is hydrolyzed by urease to ammonia and carbon dioxide. GLDH catalyzes the condensation of ammonia and α-ketoglutarate to glutamate with the concomitant oxidation of reduced β-NADH to β-NAD. Change in the absorbance was directly proportional to the urea levels.[ 12 ]
Estimation of total cholesterol by semiauto analyzer (cholesterol oxidase and peroxidase method)
Cholesterol esterase (CHE) hydrolyzed the esterified cholesterol to free cholesterol. The free cholesterol was oxidized to form hydrogen peroxide (H 2 O 2 ), which further reacted with phenol and 4 amino antipyrine by peroxidase to form red-colored quinoneimine dye complex. The intensity of the color was directly proportional to the cholesterol in the serum sample.[ 13 ]

Estimation of total cholesterol by full automatic analyzer (cholesterol oxidase and peroxidase method)
Cholesterol esters in serum were hydrolyzed by CHE. The free cholesterol produced was oxidized by cholesterol oxidase (CHO) to cholest-4-en-3-one with the simultaneous production of H 2 O 2 , which oxidatively coupled with 4-aminoantipyrine and phenol in the presence of peroxidase to yield a chromophore. The red quinoneimine dye formed was measured at 540/600 nm as an increase in absorbance:[ 14 ]

Estimation of triacylglycerol by semiauto analyzer (enzymatic glycerol phosphate oxidase and peroxidase method)
Lipoprotein lipase hydrolyzed TGs to glycerol and free fatty acid. The glycerol formed with ATP in the presence of glycerol kinase formed glycerol 3 P, oxidized by glycerol phosphate oxidase to form H 2 O 2 , that reacted with phenolic compound and 4-amino antipyrine by the catalytic action of peroxidase to form a red-colored quinoneimine dye complex, intensity of which was directly proportional to the TGs present in the sample:[ 15 ]

Estimation of triacylglycerol by fully automatic analyzer (enzymatic glycerol phosphate oxidase and peroxidase: GPO POD method)
TGs in the sample were hydrolyzed by lipoprotein lipase to glycerol and free fatty acids. Glycerol kinase acted on glycerol to form glycerol 3 P, oxidized by glycerol phosphate oxidase to form H 2 O 2 , that reacted with chlorophenolic compound and 4-amino antipyrine by the catalytic action of peroxidase to form a red-colored benzoquinone mono imino phenazone complex.[ 16 ]
Estimation of SGOT by semiauto analyzer (International Federation of Clinical Chemistry [IFCC] kinetic method)
SGOT (AST) catalyzes the transfer of an amino group from L-aspartate to α-ketoglutarate. The rate of reaction was monitored using a coupling enzyme malate dehydrogenase (MDH), whereby the oxaloacetate formed was converted to malate in the presence of NADH. The oxidation of NADH was measured by monitoring the decrease in absorbance at 340 nm:[ 17 ]

Estimation of SGOT by fully automatic analyzer (IFCC kinetic method)
AST catalyzes the transfer of the amino group from L-aspartate to α-ketoglutarate to yield oxaloacetate and L-glutamate. MDH catalyzed the reduction of oxaloacetate with simultaneous oxidation of NADH + to NAD. The resulting rate of decrease in absorbance at 340 nm was directly proportional to the AST activity. Lactate dehydrogenase (LDH) was added to prevent interference from endogenous pyruvate which is normally present in serum.[ 17 ]
Estimation of SGPT by semiauto analyzer (IFCC kinetic method)
ALT or SGPT catalyzes the reversible transfer of an amino group from alanine to oxoglutarate forming glutamate and pyruvate. The pyruvate produced was reduced to lactate by LDH and NADH:[ 17 ]

Estimation of SGPT by fully automatic analyzer (IFCC kinetic method without pyridoxal phosphate)
ALT transfers the amino group from alanine to α-oxoglutarate to form pyruvate and glutamate. LDH catalyzed the reaction with pyruvate and NADH to produce lactate and NAD+. The decrease in absorbance due to the consumption of NADH was measured at 340 nm and was proportional to the ALT activity in the sample.[ 17 ]
The data generated by estimating these biochemical parameters by the semiauto analyzer and the fully automatic analyzer method were registered for statistical analysis.
Statistical analysis
Data were entered in Microsoft Excel 2013 and were analyzed using Statistical Package for the Social Sciences (SPSS SPSS by IBM) software program, version 20.0. Normality of data was assessed using skewness and kurtosis, normality plots, and statistical tests of normality such as Shapiro–Wilk and Kolmogorov–Smirnov tests. Quantitative data were represented in the form mean and standard deviation (SD). Correlation between two quantitative data was assessed using spearman's correlation as the data was not distributed normally.
Bland–Altman plot analysis was performed to assess the measures of agreement between two different methods of estimation of a biochemical parameter. Scatter plot was plotted between mean on the x -axis and difference of two measurement methods on the y -axis and limits of agreement were calculated using mean ± 1.96 SD of the differences between two measurements. A scatter that is evenly distributed above and below the zero line of no difference indicates that there is no systematic bias between the two methods and a scatter that is largely above or largely below the zero line of no difference or a scatter that increases or decreases with the mean value indicates a systematic bias between both methods. A Kendall's correlation coefficient between the means and the differences was obtained to confirm the uniformity of variance in the repeated measurements.
The intra-class correlation coefficient (ICC) was used to describe the relative extent to which two continuous measurements taken by two different methods of assessment are related. A high value of ICC of 0.95 indicates that 95% of the variance in the measurement is due to the true variance between the methods and 5% of the variance is due to measurement error or the variance within two methods.
This study was conducted on 149 patients and the biochemical parameters obtained were compared using semiauto and automated methods.
Table 1 represents the mean and SD, skewness, kurtosis of serum urea, cholesterol, TG, PT, and OT values along with correlation between two semiauto and automated methods. Skewness and kurtosis values in Table 1 indicated that there was a very high variability in the distribution of urea, TG, OT, and PT values in both measurement methods, whereas cholesterol data followed a normal distribution (skewness: 1.522, 1.037; kurtosis: 2.373, 0.693 in manual and automated methods, respectively). There was a significant positive correlation between both the methods of assessment in all the aforementioned five parameters.
Descriptive statistics, skewness, kurtosis, and correlation between two measurement methods
Mean | Std. deviation | Skewness | Kurtosis | Spearman correlation | |||
---|---|---|---|---|---|---|---|
Semiauto urea | 31.74 | 26.118 | 149 | 3.432 | 15.801 | 0.691 | 0.0001 |
Auto urea | 41.58 | 41.073 | 149 | 2.724 | 8.032 | ||
Semiauto cholesterol | 142.79 | 61.498 | 149 | 1.522 | 2.373 | 0.798 | 0.0001 |
Auto cholesterol | 161.30 | 67.555 | 149 | 1.037 | 0.693 | ||
Semiauto TG | 136.17 | 67.470 | 149 | 2.258 | 5.989 | 0.821 | 0.0001 |
Auto TG | 160.51 | 92.825 | 149 | 2.596 | 7.402 | ||
Semiauto OT | 42.42 | 76.576 | 149 | 4.533 | 20.42 | 0.653 | 0.0001 |
Auto OT | 56.95 | 102.384 | 149 | 4.187 | 17.455 | ||
Semiauto PT | 37.52 | 41.046 | 149 | 5.427 | 35.923 | 0.551 | 0.0001 |
Auto PT | 59.99 | 104.540 | 149 | 7.995 | 77.454 |
Table 1 and Figure 1 show that serum urea gave a correlation coefficient of r = 0.691; P = 0.0001 at 95% confidence interval with a regression equation of y = 0.8966 x + 32.859 (where y = measurements in automated urea, x = measurement in semiauto urea). Serum cholesterol gave a correlation coefficient of r = 0.798; P = 0.0001 at 95% confidence interval with a regression equation of y = 1.3144× =0.133 (where y = measurements in automated cholesterol, x = measurement in semiauto cholesterol). Serum TG gave a correlation coefficient of r = 0.821; P = 0.0001 at 95% confidence interval with a regression equation of y = 1.2835x – 14.26 (where y = measurements in automated TG, x = measurement in semiauto TG). Serum SGOT gave a correlation coefficient of r = 0.653; P = 0.0001 at 95% confidence interval with a regression equation of y = 1.1861x + 6.6351 (where y = measurements in automated SGOT, x = measurement in semiauto SGOT). Serum PT gave a correlation coefficient of r = 0.551; P = 0.0001 at 95% confidence interval with a regression equation of y = 2.1553x – 20.822 (where y = measurements in automated PT, x = measurement in semiauto PT). Hence, it can be summarized that the measurements in semiauto and automated methods showed significant positive correlation.

Correlation between two measurement methods. (a) Correlation between manual and automated urea. (b) Correlation between manual and automated cholesterol. (c) Correlation between manual and automated triglyceride. (d) Correlation between manual OT and automated OT. (e) Correlation between manual and automated PT
After applying Bland–Altman analysis of agreement, on comparison between semiauto and automated methods, the mean difference was found to be less for urea –9.85 ± 23.997 (LOA: 37.189, –56.88) [ Table 2 ], whereas it was highest for TG –24.34 ± 38.513 (LOA: 51.144, –99.829), suggesting that both methods can measure urea with less difference in absolute values, whereas for TG the measurement values are highly variable [ Table 3 ]. The high intra-class correlation of 0.731 (PT) to 0.94 (TG) suggested the two continuous measurements taken by two different methods of assessment are highly related [ Figure 2 ].
Bland-Altman analysis of agreement between two measurement methods
Mean | Std. deviation | Limits of agreement (LOA) | Cronbach α | 95% CI | |||
---|---|---|---|---|---|---|---|
Upper | Lower | Upper | Lower | ||||
Difference urea=Semiauto-auto | -9.85 | 23.997 | 37.189 | -56.880 | 0.862 | 0.809 | 0.90 |
Difference Cholesterol=Semiauto-auto | -18.52 | 39.261 | 58.434 | -95.468 | 0.898 | 0.859 | 0.926 |
Difference TG=Semiauto-auto | -24.34 | 38.513 | 51.144 | -99.829 | 0.94 | 0.918 | 0.957 |
Difference OT=Semiauto-auto | -14.53 | 49.354 | 82.204 | -111.264 | 0.919 | 0.889 | 0.942 |
Difference PT=Semiauto-auto | -22.47 | 73.150 | 120.905 | -165.845 | 0.731 | 0.628 | 0.805 |
Correlation between the means and the differences to confirm the uniformity of variance
Kendall’s tau_b | Difference urea=manual-auto | |
---|---|---|
Mean urea=(manual + auto) /2 | Correlation coefficient | -0.386 |
0.0001 | ||
Difference cholesterol=manual-auto | ||
Mean cholesterol=(manual + auto) /2 | Correlation coefficient | -0.181 |
0.0001 | ||
Difference TG=manual-auto | ||
Mean TG=(manual + auto) /2 | Correlation coefficient | -0.224 |
0.0001 | ||
Difference OT=manual-auto | ||
Mean OT=(manual + auto) /2 | Correlation coefficient | -0.222 |
0.0001 | ||
Difference PT=manual-auto | ||
Mean PT=(manual + auto) /2 | Correlation coefficient | -0.231 |
0.0001 |

Agreement between manual and auto methods (a) Urea (b) Cholesterol (c) TG (d) OT (e) PT
The correlation between mean and difference of two methods showed weak correlation between two methods. Correlation coefficient was highest between urea (–0.386) and lowest between cholesterol (–0.181). The weak correlation coefficient suggests that both the methods are similar in measurement.
The medical requirements for performance of the biochemical parameters can best and most easily be described in terms of the total analytic error that represents both random and systematic components. The performance standard (PS), summarizes the medical specification for total analytic error.[ 18 ] The estimates of analytic error in the test method were compared with the defined allowable error (EA). If the errors observed in the test method are comparable to the medically allowable errors, then the method performs acceptably. If larger difference is observed, then the errors need to be decreased by appropriate modifications or else the method is unacceptable.[ 19 ]
In this study biochemical parameters obtained were compared using semiauto analyzer and automated methods. High variability in the distribution of urea, TG, SGOT, and SGPT values observed in both measurement methods as compared to cholesterol data indicating significant positive correlation between both the methods of assessment in all the above mentioned five parameters. This is in concordance with the previous study conducted by Swetha and Kavitha,[ 20 ] in which significant positive correlation at 95% confidence interval was documented in the SGOT and SGPT levels between semiauto and automated analyzers using the same analytical methodology. Ilanchezhian et al .[ 21 ] found lesser blood glucose values in glucometer as compared to chemical analyzer with lesser values of glucose in semiauto -analyzer as compared to auto analyzer that they attributed to changes in the temperature, humidity and transport conditions. Bland–Altman analysis of agreement, on comparison between semiautomatic and fully automated methods, the mean difference was found to be less for urea and highest for TG suggesting both methods can measure urea with less difference in absolute values, whereas for TG the measurement values are highly variable that could be attributed to certain variables such as sample capacity of the tubes, sample volume, dead volume, and throughput walkaway time.[ 22 ]
Many studies were done to compare the effectiveness of biochemical method with the molecular method with variable and contradictory results.[ 23 , 24 ]
In our study the high intra-class correlation of 0.731 (for SGPT) to 0.94 (for TG) suggested that two continuous measurements taken by two different methods of assessment are highly related, this satisfies the criteria that can be used to judge whether an analytical method has acceptable precision and accuracy. However, factors such as recovery, interference, and running in replicates must be taken into account, while conduction method-evaluation studies were taken into account to evaluate the performance of a new laboratory method. Analytical variations observed in this study could be due to testing methods and equipment, which may cause analyte values to be slightly different each time they are measured.
The district-level health services have an urgent need for improvement in diagnostic laboratory quality reporting by adopting latest technologies. Most of the peripheral health-care institutions are not equipped with fully automated chemistry analyzers. Semiauto analyzer-based biochemical reporting of routine parameters have comparable and dependable results, provided there is continuous, coordinated, and comprehensive care by primary care physicians and staff. The inferior quality of care reemphasizes the role of primary care physicians in the screening, diagnosis and treatment of common metabolic disorders. The district-level health-care facilities need reorganizations for better management of chronic disease management programs.
The test performance of biochemical parameters such as urea, total cholesterol, TG, SGOT, and SGPT taken by semiauto analyzer, and fully automatic analyzer method of assessment were highly related and comparable, with a significant positive correlation. Semiauto analyzer could be an efficient alternative in peripheral setups to provide quality biochemistry laboratory services.
Financial support and sponsorship
Conflicts of interest.
There are no conflicts of interest.
Click through the PLOS taxonomy to find articles in your field.
For more information about PLOS Subject Areas, click here .
Loading metrics
Open Access
Peer-reviewed
Research Article
Normative range of blood biochemical parameters in urban Indian school-going adolescents
Contributed equally to this work with: Khushdeep Bandesh, Punam Jha
Roles Data curation, Formal analysis, Investigation, Methodology, Validation, Visualization, Writing – original draft
Affiliations Genomics and Molecular Medicine Unit, CSIR–Institute of Genomics and Integrative Biology, New Delhi, India, Academy of Scientific and Innovative Research, CSIR–Institute of Genomics and Integrative Biology Campus, New Delhi, India
Roles Data curation, Formal analysis, Methodology, Software

Roles Validation
Roles Resources
Affiliation Senior consultant endocrinologist and Scientific Advisor (Projects), International Life Sciences Institute-India, New Delhi, India
¶ Complete membership of the author group can be found in the Acknowledgments
Roles Writing – review & editing
* E-mail: [email protected] (DB); [email protected] (NT); [email protected] (VS)
Affiliations Academy of Scientific and Innovative Research, CSIR–Institute of Genomics and Integrative Biology Campus, New Delhi, India, GN Ramachandran Knowledge Center for Genome Informatics, CSIR–Institute of Genomics and Integrative Biology (CSIR–IGIB), Delhi, India
Affiliation Department of Endocrinology and Metabolism, All India Institute of Medical Sciences, New Delhi, India
Roles Conceptualization, Funding acquisition, Project administration, Supervision, Writing – review & editing
Affiliations Genomics and Molecular Medicine Unit, CSIR–Institute of Genomics and Integrative Biology, New Delhi, India, Academy of Scientific and Innovative Research, CSIR–Institute of Genomics and Integrative Biology Campus, New Delhi, India, Systems Genomics Laboratory, School of Biotechnology, Jawaharlal Nehru University, New Delhi, India
- Khushdeep Bandesh,
- Punam Jha,
- Anil K. Giri,
- Raman K. Marwaha,
- INDICO,
- Vinod Scaria,
- Nikhil Tandon,
- Dwaipayan Bharadwaj
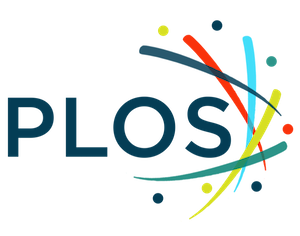
- Published: March 7, 2019
- https://doi.org/10.1371/journal.pone.0213255
- Reader Comments
Adolescence is the most critical phase of human growth that radically alters physiology of the body and wherein any inconsistency can lead to serious health consequences in adulthood. The timing and pace at which various developmental events occur during adolescence is highly diverse and thus results in a drastic change in blood biochemistry. Monitoring the physiological levels of various biochemical measures in ample number of individuals during adolescence can call up for an early intervention in managing metabolic diseases in adulthood. Today, only a couple of studies in different populations have investigated blood biochemistry in a small number of adolescents however, there is no comprehensive biochemical data available worldwide. In view, we performed a cross-sectional study in a sizeable group of 7,618 Indian adolescents (3,333 boys and 4,285 girls) aged between 11–17 years to inspect the distribution of values of common biochemical parameters that generally prevails during adolescence and we observed that various parameters considerably follow the reported values from different populations being 3.56–6.49mmol/L (fasting glucose), 10.60–199.48pmol/L (insulin), 0.21–3.22nmol/L (C–peptide), 3.85–6.25% (HbA 1 c), 2.49–5.54mmol/L (total cholesterol), 1.16–3.69mmol/L (LDL), 0.78–1.85mmol/L (HDL), 0.33–2.24mmol/L (triglycerides), 3.56–11.45mmol/L (urea), 130.01–440.15μmol/L (uric acid) and 22.99–74.28μmol/L (creatinine). Barring LDL and triglycerides, all parameters differed significantly between boys and girls (p< 0.001). Highest difference was seen for uric acid (p = 1.3 x10 -187 ) followed by C–peptide (p = 6.6 x10 -89 ). Across all ages during adolescence, glycemic and nitrogen metabolites parameters varied markedly with gender. Amongst lipid parameters, only HDL levels were found to be significantly associated with gender following puberty (p< 0.001). All parameters except urea, differed considerably in obese and lean adolescents (p< 0.0001). The present study asserts that age, sex and BMI are the essential contributors to variability in blood biochemistry during adolescence. Our composite data on common blood biochemical measures will benefit future endeavors to define reference intervals in adolescents especially when the global availability is scarce.
Citation: Bandesh K, Jha P, Giri AK, Marwaha RK, INDICO, Scaria V, et al. (2019) Normative range of blood biochemical parameters in urban Indian school-going adolescents. PLoS ONE 14(3): e0213255. https://doi.org/10.1371/journal.pone.0213255
Editor: Domenico Tricò, University of Pisa, ITALY
Received: June 4, 2018; Accepted: February 18, 2019; Published: March 7, 2019
Copyright: © 2019 Bandesh et al. This is an open access article distributed under the terms of the Creative Commons Attribution License , which permits unrestricted use, distribution, and reproduction in any medium, provided the original author and source are credited.
Data Availability: All relevant data are within the paper and its Supporting Information files.
Funding: The study was supported by the Department of Biotechnology [DBT], Government of India through GLUE grant project (N 1292) and BIOCHILD project (GAP0089). The work was also funded by Department of Science and Technology [DST], Government of India (DST/SR/PURSE PHASE II/11). Council of Scientific and Industrial Research CSIR-Senior Research Fellowship [KB] and Council of Scientific and Industrial Research CSIR-TWAS Postgraduate fellowship [PJ]. The funders had no role in study design, data collection and analysis, decision to publish, or preparation of the manuscript.
Competing interests: The authors have declared that no competing interests exist.
Introduction
Blood tests are routinely prescribed in healthcare systems to analyze the biochemistry to determine a diseased or a healthy state. A panel of blood tests commonly measures metabolic parameters–glycemic (glucose, insulin), lipids (total cholesterol, LDL, HDL, triglycerides), nitrogen metabolites (urea, creatinine, uric acid); important electrolytes (Na + , K + , Cl - , HCO 3 - ) and crucial enzymes (liver aminotransferases and phosphatases, etc.) [ 1 ]. These biochemical parameters vary considerably depending upon an individual's age, sex, ethnicity, dietary intake or physiological state of the body [ 2 – 8 ].
Age is a major factor that contributes to variation in blood biochemistry [ 9 ]. Ageing is a continuous dynamic process that leads to physiological, psychological and behavioral changes resulting in biological aberrations, metabolic dysfunction, cellular senescence or decline in function of organ systems [ 10 , 11 ]. Amongst various life stages–infancy, childhood, adolescence, adulthood and old age, adolescence is the critical one. It marks the period of transition from childhood to adulthood and is characterized by rapid biological, social and emotional changes [ 12 ] that severely impacts future health. This transition is largely governed by gender of an individual that gives rise to difference in hormonal levels among boys and girls at puberty [ 13 ]. On account of these multiple significant physiological changes, biochemical parameters tend to vary drastically. Administering the variability of these parameters during adolescence can layoff allied metabolic diseases in adulthood. Currently, a large body of studies have examined variability in blood biochemistry in children in different populations, however only a few inspected adolescents. In view, we analyzed physiological levels of common biochemical parameters in blood (glucose, insulin, C–peptide, HbA 1 c, total cholesterol {TC}, LDL, HDL, triglycerides, urea, creatinine and uric acid) and studied their variation with Body Mass Index (BMI) in several adolescents (N = 7,618), a number that has never been studied previously. The samples were collected as a part of another ongoing project beyond the intentions to define reference intervals. Therefore, we conveniently aimed to look at the mere dispersion of values of different parameters that normally exists during adolescence.
The physiological changes during adolescence always occur in a universal order however, their timing and pace vary depending upon environmental influences (e.g. food habits, lifestyle, and cultural systems) [ 14 – 17 ]. Today, several populations worldwide have encountered rapid urbanization swiftly changing the lifestyle of its people and hence predisposing them to several complex diseases. These changes together with the genetic makeup determine population specific normal range of values for common biochemical parameters. One such population that exhibits huge diversity on grounds of both genetic and lifestyle ways is Indians. Indians amounting to one-sixth of world's total population [ 18 ], comprise several endogamous groups that are genetically distinct and highly diverse in terms of dietary intake and ways of living [ 19 ]. Indian diets are typically carbohydrate rich compared to the dietary patterns favored in rest of the world [ 20 , 21 ], and indeed pose Indians at a high risk for metabolic diseases [ 21 – 24 ]. Therefore, we exclusively studied Indians at a younger age to institute an early intervention and management of highly prevalent metabolic diseases in the adult population.
Materials and methods
The study was carried out in accordance with the principles of the Helsinki Declaration and approved by Human Ethics Committee of All India Institute of Medical Sciences, New Delhi, India and CSIR–Institute of Genomics and Integrative Biology, New Delhi, India. A written informed consent was obtained from parents/guardians of each participant followed by a verbal consent from participants themselves.
Study population
We conducted the study at CSIR–Institute of Genomics and Integrative Biology, New Delhi, India. A total of 7,618 adolescents (3,333 boys and 4,285 girls) aged between 11–17 years were enrolled from various schools located in urban areas of Delhi–National Capital Region as a part of a study on childhood obesity conducted previously in our laboratory [ 25 ]. Average age in total sample group was observed to 13.73 years (± 1.80 years), 13.68 ±1.78 years in boys and 13.77 ±1.77 years in girls. Residing in north part of Indian subcontinent, all participants comprised Indo–European ethnicity and were selected through a pre–designed questionnaire assessing their age, dietary habits, state of birth, parent's state of origin and, past and present medical history. Participants who suffered from any chronic ailments, under medication, history of hospitalization in last six months and self–denial for blood withdrawal were excluded from the study.
The samples were categorized as Obese (OB), Overweight (OW) and Lean or Normal weight (NW) by calculating BMI z-scores and inspecting the previously established BMI percentiles for specific age and sex in children during adolescence (CDC Growth Charts) [ 26 , 27 ]. BMI at or above 95 th percentile at same age and sex was counted as Obese [ 26 ]. BMI below 95 th percentile but at or above 85 th percentile was considered Overweight and BMI in-between 85 th percentile and 5 th percentile was defined as Lean [ 26 ].
Sample collection and biochemical analysis
Participants were subjected to an overnight fast and their peripheral blood was collected by trained phlebotomists in three different vacutainers–serum separator tubes, sodium fluoride vacuette and potassium EDTA tubes manufactured by Becton Dickinson, New Jersey, USA. Samples were transported to laboratory over ice for processing within 2 hours of collection. Different biochemical parameters were measured using well-recognized robust analyzers–COBAS Integra 400 plus and COBAS e411. Details regarding assay methods for studied clinical parameters have been described previously [ 28 ].
Statistical analyses
As mentioned, we wanted to capture the natural spread of values for each parameter during adolescence, so no outliers were deleted. Thus, instead of presenting the mean values that are normally affected by the outliers, we rather looked at the median values and interquartile ranges (2.5 percentile and 97.5 percentile). Mann–Whitney U test was used for comparing difference among different groups that were analyzed. P value less than 0.05 was considered significant. R version 3.4.0 was used for statistical analysis ( http://www.R-project.org/ ). Although there were a small number of tests (11 parameters) that were performed between different groups, the observed differences were further reviewed after applying Bonferroni correction wherein the corrected p value was assumed to be 0.0045 (α/number of comparisons i.e. 0.05/{11}).
Power calculation
The power of the study was calculated using two different tools separately–OpenEpi, an open-source tool for computing epidemiologic statistics for public health [ 29 ] and G*Power 3, a robust power analysis program for the social, behavioral, and biomedical sciences [ 30 ]. Power of a two-sided U test of each parameter was computed at α level of 0.05 and 95% confidence intervals from the respective effect size in boys and girls (i.e. = {difference in group means}/{standard deviation}) ( S1 Table ).
For nearly all studied biochemical parameters, the power of the study was observed to be 100 percent (97 percent for TC) to detect genuine differences between various groups ( S1 Table ). For LDL and TG, overall comparison among all adolescent boys and girls lacked sufficient power, however their individual comparisons at specific age groups secured necessary power.
Glycemic parameters are strongly associated with gender and adolescence age
Normative range of plasma glycemic parameters in Indian adolescents was 3.56–6.49mmol/l (fasting glucose), 10.60–199.48pmol/l (insulin), 0.21–3.22nmol/l (C–peptide) and 3.85–6.25% (HbA 1 c) ( Table 1 ).
- PPT PowerPoint slide
- PNG larger image
- TIFF original image
https://doi.org/10.1371/journal.pone.0213255.t001
Plasma glucose.
Boys consistently showed significantly higher levels of fasting plasma glucose than girls across all ages during adolescence (p ≤ 0.001) ( Table 2 , S2 Table ). Mean value of plasma glucose was observed to be 4.91mmol/L in boys and 4.72mmol/L in girls (p = 6.2 x 10 −31 ) ( Table 1 ). Between subsequent ages, both boys and girls show consistent plasma glucose levels, except for age of 14 years, where plasma glucose levels in boys were observed to decline significantly than other ages during adolescence ( Table 2 ).
https://doi.org/10.1371/journal.pone.0213255.t002
Plasma insulin.
Fasting plasma insulin levels were remarkably higher in girls than boys (p = 5.1 x 10 −15 ) ( Table 1 ). This observation prevailed over the ages from 11 years to 14 years (p ≤ 10 −4 ), but at later ages of adolescence both boys and girls featured comparable plasma insulin levels ( Table 2 ). Highest insulin levels were observed to be 65.53pmol/L (median value) at age of 12 years in girls and 60.14pmol/L in boys at age of 16 years ( Table 2 ). Among boys, we noticed that plasma insulin levels change negligibly with age ( Table 2 , S3 Table ). However, in girls, plasma insulin levels change significantly between ages of 12 to 13 years (p = 0.01) and 16 to 17 years (p = 0.008) ( Table 2 , S4 Table ).
Alike plasma insulin, significantly higher levels of C–peptide were found in girls than boys across different ages in adolescence (p ≤ 10 −7 {11–16 years}, p = 0.046 {16–17 years}) ( Table 2 ). Mean C–peptide levels were 1.43nmol/L in girls in contrast to 1.03nmol/L in boys (p = 6.6 x 10 −89 ) ( Table 1 ). Furthermore, C–peptide levels varied significantly between ages of 11–12 years in both genders–boys (p = 4.73 x 10 −4 ) and girls (p = 1.88 x 10 −4 ) ( S3 and S4 Tables). Additionally, boys showed markedly different C–peptide levels between 16 and 17 years of age (p = 0.002) ( Table 2 ).
Mean levels of HbA 1 c were noted to be 5.24% in girls and 5.08% in boys (p = 3.0 x 10 −17 ) ( Table 1 ). With increasing age in adolescence, girls showed unusually higher HbA 1 c levels than boys (p < 10 −5 {11–13 years}, p = 0.01{15–16 years}) ( Table 1 ). Both boys and girls exhibited significantly different HbA 1 c levels between ages of 11 and 12 years (p = 0.02 and 0.003) ( S3 and S4 Tables). Additionally, girls aged 14 years displayed drastically lower HbA 1 c levels than their younger mates (p = 0.003) ( Table 2 ).
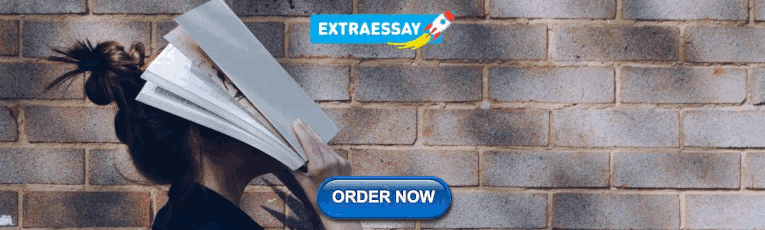
Amongst blood lipids only HDL varies robustly with gender and age throughout adolescence
In Indian adolescents, plasma levels for different lipids were commonly seen in the range of 2.49–5.54mmol/L (TC), 1.16–3.69mmol/L (LDL), 0.78–1.85mmol/L (HDL) and 0.33–2.24mmol/L (triglycerides) ( Table 1 ). Indian girls had significantly higher TC and HDL levels than boys (respective p = 1.3 x 10 −4 and 5.3 x 10 −11 ) ( Table 1 ).
Total Cholesterol.
Across all ages during adolescence, boys and girls revealed identical plasma TC levels except at age of 14 years, when girls have robustly higher levels than boys (p = 2.44 x 10 −4 ) ( Table 3 ). Barring the age of 14 years in boys, no significant change in levels of plasma TC was observed with increasing age during adolescence within each gender ( S3 and S4 Tables).
https://doi.org/10.1371/journal.pone.0213255.t003
Like TC, LDL levels were similar in boys and girls across different adolescence ages ( Table 3 ). Besides, no difference was seen with increasing age in either gender ( Table 3 ).
Indian boys and girls portrayed significantly distinct HDL levels from ages 14 years to 17 years (p < 0.001) ( Table 3 ), with girls having higher HDL levels than boys. HDL levels were found to be constant over adolescence period in both the genders ( Table 3 , S3 and S4 Tables).
Triglycerides.
Girls showed robustly higher triglyceride levels than boys at ages 11 and 12 years (p = 8.5 x 10 −4 and p = 0.01), but unexpectedly lower triglyceride levels at ages 15, 16 and 17 years (p < 0.01) ( Table 3 , S2 Table ). Within boys, triglyceride levels varied significantly between 14 and 15 years ( Table 3 , S3 and S4 Tables), conversely at 11–12 years among girls ( Table 3 , S3 and S4 Tables).
Nitrogen metabolites show marked difference among adolescent boys and girls
Plasma nitrogen metabolites were observed to be normally in the ranges of 3.56–11.45mmol/L (urea), 130.01–440.15μmol/L (uric acid) and 22.99–74.28μmol/L (creatinine) in Indian adolescents ( Table 1 ). Boys retained strikingly higher levels of all studied nitrogen metabolites than girls (p = 1.0 x 10 −39 {urea}; 1.3 x 10 −187 {uric acid} and 1.97 x 10 −28 {creatinine}).
Throughout adolescence (11–17 years), girls showed dramatically low levels of plasma urea than boys (p <10 −4 ) ( Table 4 ). Across teenage years, plasma urea levels differed suggestively from ages 11–12 years and 15–16 years in boys (respective p = 0.04, 0.006), however only between ages 15–16 years in girls (p = 0.007) ( S3 and S4 Tables).
https://doi.org/10.1371/journal.pone.0213255.t004
Plasma levels of uric acid were also remarkably low in girls than boys across all ages during adolescence (p < 0.005, 11 years and p < 10 −4 , 12 to 17 years) ( Table 4 ). With each successive year in age from 11 years to 15 years, plasma uric acid levels increased in boys (p ≤ 10 −4 ). On contrary, uric acid levels were seen to rise significantly only during transition from 11–12 years in girls (p = 0.005) ( S3 and S4 Tables).
Creatinine.
Together with urea and uric acid, Indian girls had apparently low levels of plasma creatinine than boys, with significant difference especially during the late adolescence period than boys (p < 10 −4 ) ( Table 1 ). In boys, creatinine levels were consistently found to be increased from age of 12 years to 16 years, with highest increment between 13 to 15 years (p < 10 −6 ) ( Table 4 , S3 Table ). Girls did not show a remarkable upsurge in creatinine levels during adolescence wherein levels increased only marginally from ages 11 to 15 years (p < 0.05).
BMI status significantly governs blood biochemistry during adolescence
We categorized our samples as per their BMI z-scores and observed that levels of all biochemical parameters except urea alter drastically among OB, OW and NW adolescents ( Table 5 ). Similar trend of association was also noted for all parameters in the respective BMI categories within each gender (Table S5a and S5b) barring FG, which however showed consistency when investigated individually in boys and girls.
https://doi.org/10.1371/journal.pone.0213255.t005
Rapid growth and development during childhood administer normal circulating levels of various biochemical markers. Abnormal levels of such markers are classical indications that can potentially signal an underlying pediatric disease or early signs of an adult onset disease. These levels are strongly influenced by their rate of growth, organ maturity, hormone responsiveness, nutrition and metabolism [ 31 , 32 ]. Additionally, children are immunologically naive and respond differently to infections, therefore adult normative values of various biochemical markers are not applicable in a pediatric setting. Given this background, we aimed to establish normative range of commonly studied blood biochemical parameters in Indian adolescents.
This is the first comprehensive study in 7,618 Indo–Europeans and a largest one worldwide ever conducted to examine blood biochemistry and its variation with gender and age during adolescence. Previously, Canadian Laboratory Initiative on Pediatric Reference Intervals (CALIPER) compiled health information from nearly 9000 community children of all ages from birth to 18 years but comprised only a handful of adolescents [ 33 ]. Likewise, the BCAMS Study in Chinese population inspected 3,223 schoolchildren aged 6–18 years [ 34 ]. Earlier studies investigating blood biochemistry specifically in adolescents were conducted in limited samples—Nigerians (N = 628) [ 35 ], Croatians (N = 998) [ 36 ] and Czech (N = 1,518) [ 37 ], which is much narrow than the present study. In India, a previous study examined a few surrogate markers for insulin resistance in 695 adolescents [ 38 ] and serum lipid profile in 4,245 children and adolescents aging from 6–17 years [ 39 ] but failed to deliver normative ranges for all common blood biochemical parameters assessed in a large sample set that could represent Indian population.
In accordance with Clinical and Laboratory Standards Institute (CLSI) guidelines [ 40 ], we studied various parameters in an interval between 0.025 and 0.975 fractiles of the distribution of results wherein 95% of the values resided. In adolescents, normative range for fasting plasma glucose of 3.56–6.49mmol/L was observed in Indians as against 3.77–8.27mmol/L in Nigerians [ 35 ] and 3.9–5.9mmol/L in Croatians [ 36 ]. For plasma insulin, Indian adolescents have normal levels within a range of 10.60–199.48pmol/L that is typically less than reported by a recent multiethnic study in Canada–CALIPER (15 – 345pmol/L) [ 33 ]. Mean plasma glucose and insulin levels were found to be 4.8mmol/L and 68.90pmol/L in the present study in lieu of previously reported levels of 5.19mmol/L and 87.5pmol/L in mere 695 Indian adolescents [ 38 ]. Besides, Indian boys featured considerably higher plasma glucose levels but lower plasma insulin than girls. This agrees with previous studies in normal weight adolescents from Czech [ 37 ] and Chinese populations [ 34 ] but confronts CALIPER data reporting similar insulin levels in both genders [ 33 ]. Girls are more insulin resistant than boys [ 41 ]. Adolescence period is largely predominated by puberty that is associated with a marked decrease in insulin sensitivity [ 42 ] that in turn is accompanied by compensatory increase in insulin secretion eventually leading to insulin resistance [ 43 ]. This provides a biological rationale for presence of remarkably high insulin and correspondingly low glucose levels in girls during adolescence.
Normative levels of C–peptide (0.21–3.22nmol/L) in Indians encompassed larger confines than reported levels in Canadians (0.26–2.24nmol/L) [ 33 ]. Besides insulin, Indian girls showed significantly higher C–peptide levels than boys. C–peptide, a product of insulin biosynthesis, is released in equimolar amount as mature insulin [ 44 ]. In view of higher secretion of insulin in girls, higher levels of C–peptide were anticipated [ 44 , 45 , 46 ]. Furthermore, mean HbA 1 c levels in Indians (5.16%) resembled Mexican–Americans (5.05%), non–Hispanic blacks (5.07%) and non–Hispanic whites (4.93%) [ 47 ]. Despite low glucose levels, we noticed unusually high HbA 1 c in girls during adolescence. Plasma glucose molecules attach non–enzymatically to hemoglobin in erythrocytes (HbA 1 c) [ 48 ]. Any variation in hemoglobin levels due to erythrocyte turnover will influence HbA 1 c values regardless of glucose levels. During adolescence, there is an upsurge in release of sex–specific hormones–estrogen in girls and testosterone in boys. Higher testosterone synthesis in boys at puberty results in greater production of hemoglobin [ 49 ]. HbA 1 c is the ratio of glycated hemoglobin to total hemoglobin content. Hence, a higher amount of total hemoglobin in boys will end up in lower HbA 1 c levels than girls which is what we also observed in our study.
Levels of total cholesterol in Indian adolescents were found to lie between 2.49–5.54mmol/L that is correspondent to well–established levels (2.9–5.8mmol/L) in children and adolescents from multiple ethnicities in Canada [ 33 ]. We observed mean levels of TC as 3.78mmol/L that is marginally lower than previously reported TC levels (4.85mmol/L) in Indian adolescents [ 38 ]. Further, presence of high TC levels in girls than boys during adolescence is also detected in Croatians where girls display normal TC levels within 3.3–6.2mmol/L as against 2.8–5.6mmol/L in boys [ 36 ]. Identification of high TC in girls is also noticed in Chinese adolescents [ 50 ]. In each gender, TC levels remained constant across different ages during adolescence. This finding is however inconsistent with the results in US adolescents that presented a decrease in TC levels with sexual maturation [ 51 ]. It is well–established that during puberty plasma lipid levels tend to differ by gender, ethnicity or both [ 51 ]. Correspondingly normal LDL levels in Indian adolescents were found to be similar between genders ranging from 1.16–3.69mmol/L. Similarity in LDL levels between girls and boys is also seen in Europeans ({Boys: 1.5–4.1mmol/L}; {Girls: 1.6–4.3mmol/L }) [ 36 ] and previously studied Indians ({Boys: mean levels = 2.20mmol/L}; {Girls: mean levels = 2.22mmol/L}) [ 38 ]. After the age of 13 years (peak pubertal period), girls showed higher LDL levels than boys. This nicely agrees with the observations in 14-year-old Chinese adolescents (girls: 1.03–3.41mmol/L; boys: 0.97–2.99mmol/L) [ 50 ]. Puberty is accompanied by increased BMI and subcutaneous adiposity owing to abrupt rise in estrogen levels [ 52 ]. Subcutaneous adiposity is a major covariate for LDL levels in adolescents (p < 0.001) [ 53 ] which fairly accounts for raised LDL levels in girls following puberty.
Normative range of HDL levels in Indians (0.78–1.85mmol/L) is lower than previously reported limits in Europeans (0.9–2.2mmol/L) [ 36 ]. In addition, mean HDL levels were observed to be like earlier stated levels in Indian adolescents (1.22mmol/L against 1.14mmol/L) [ 38 ]. During early years of adolescence, both boys and girls harbored similar HDL levels. However, at puberty, boys uniquely encountered a radical decline in HDL levels. Essentially, male puberty is characterized by boost in testosterone production that stimulates hepatic lipase activity [ 54 ], thereby reducing plasma HDL levels during the period [ 55 ]. On the other hand, observed TG levels in Indian adolescents (0.33–2.24mmol/L) correspond to normative TG levels in children and adolescents in Canada (0.5–2.23mmol/L) [ 33 ], however disperse over a wider range than Europeans (0.3–1.6mmol/L) [ 36 ]. Mean TG levels were slightly lower than our previously reported TG levels in lesser number of Indian adolescents (1.04mmol/L than 1.56mmol/L) [ 38 ]. At all successive ages after onset of puberty (14 years), high TG levels prevailed in Indian boys. Also, in Chinese boys, TG levels were reported to significantly increase from 0.79mmol/L at 12 years to 1.00mmol/L at 18 years of age [ 50 ]. Elevated TG levels in boys during adolescence occurs due to testosterone mediated decrease in Post Heparin (PH) Lipoprotein Lipase [ 56 ], an enzyme that catalyzes hydrolysis of TG [ 57 ].
Serum urea, uric acid and creatinine levels in Indian adolescents were observed to commonly reside within respective limits of 3.56–11.45mmol/L; 130.01–440.15μmol/L and 22.99–74.28μmol/L that are wider in comparison to 2.7–6.8mmol/L, 125–228μmol/L and 46–80μmol/L in Europeans [ 36 ]. All three studied nitrogen metabolites revealed exceptionally high levels in boys than girls throughout adolescence. Urea is a chief nitrogenous waste product of dietary proteins [ 58 ]. Protein rich foods largely comprise fish, meat, poultry and eggs. We recruited our samples from Northern part of India that is mainly dominated by vegetarian population [ 21 , 59 ]. Here, men are primary meat consuming people [ 21 , 59 ] and therefore vindicates high urea levels in boys. Similarly, dietary intake of high amounts of purine rich foods–red meat, sea foods, mushrooms, etc. end up in high uric acid levels in blood plasma [ 60 ]. At odds with fundamental influence of diet on plasma levels of urea and uric acid, creatinine is a waste product synthesized by muscles at a steady rate as a part of normal daily activity [ 61 ]. Men have higher muscle mass than women throughout their life [ 61 ] which corresponds to high creatinine production and elevated levels in blood.
Besides drastic variation in blood biochemistry due to multiple physiological events, a higher BMI further reinforces this variability during adolescence. We observed that all biochemical parameters vary considerably among Obese/ overweight and lean adolescents. Urea was noted to be an exception to the prevalent trend. The underlying rationale behind consistency of serum urea levels with obesity is though unknown, however urea levels has been previously been reported to remain unaltered with weight loss [ 62 ].
As is now evident that normal blood biochemistry indeed changes with ethnicity and food habits, our study therefore established a population specific normative range of common blood biochemical parameters in Indian adolescents and investigated its variability with gender, age and BMI. The age of onset of puberty varies widely among individuals owing to their genetic and environmental factors starting from as early as 8 years to as late as 16 years. The precise interpretation of our data is confined by lack of information about the age of onset of puberty in our cohort. Our findings will benefit medical practitioners assessing pediatric and adolescent health in piloting a timely intervention for chronic conditions and also serve as a platform for future studies to design proper reference intervals for adolescents in different world populations.
Supporting information
S1 table. statistical power of the study..
https://doi.org/10.1371/journal.pone.0213255.s001
S2 Table. Calculated p values for comparison of various biochemical parameters between boys and girls of same age group.
https://doi.org/10.1371/journal.pone.0213255.s002
S3 Table. Calculated p values for comparison of various biochemical parameters among boys of different age.
https://doi.org/10.1371/journal.pone.0213255.s003
S4 Table. Calculated p values for comparison of various biochemical parameters among girls of different age groups.
https://doi.org/10.1371/journal.pone.0213255.s004
S5 Table. Variation of biochemical parameters with BMI in boys and girls during adolescence.
https://doi.org/10.1371/journal.pone.0213255.s005
Acknowledgments
We thank all adolescents and their parents for participation and school authorities for cooperation in carrying out the study. We also thank all the members of INDICO Consortium: Viswanathan Mohan, Saurabh Ghosh, Abhay Sharma, Rubina Tabassum, Ganesh Chauhan, Anubha Mahajan, Om Prakash Dwivedi, Lakshmi Ramakrishnan, Radha Venkatesan, M. Chidambaram, D. Prabhakaran, K.S Reddy, Monisha Banerjee, Madhukar Saxena, Sandeep Mathur, Anil Bhansali, Viral Shah, S.V. Madhu, R.K. Marwaha, Pradeep Venkatesh, S.K. Aggarwal, Shantanu Sen Gupta, Sreenivas Chavali, Amitabh Sharma, Analabha Basu, Priyanka Banerjee, Anil K Giri, Shraddha Chakraborty, Yasmeen Kauser, Gauri Prasad, B. Abitha, Aditya Undru, Donaka Rajashekar, Vaisak Parekatt, Suki Roy and Anjali Singh for their support in sample collection, processing and data generation.
- View Article
- PubMed/NCBI
- Google Scholar
- 11. Nathan Wetherill Shock. Article—Human aging. Encyclopædia Britannica, inc. Date Published: November 23, 2017. Online at https://www.britannica.com/science/human-aging , Access Date: April 25, 2018.
- 13. The Editors of Encyclopaedia Britannica. Article—Puberty. Encyclopædia Britannica, inc. Date Published: April 05, 2018. Online at https://www.britannica.com/science/puberty , Accessed: 25 April 2018.
- 16. Antonishak J, Sutfin EL, Reppucci ND. Community Influence on Adolescent Development. In: Gullotta TP, Adams GR. (eds) Handbook of Adolescent Behavioral Problems. Springer, Boston, MA. 2005; 57–78.
- 18. Trading Economics. Online at https://tradingeconomics.com/india/population . Accessed: 29 May 2018.
- 19. Majumder PP, Mukherjee BN. Genetic Diversity and Affinities among Indian Populations: An Overview. In: Majumder PP. (eds) Human Population Genetics. Springer, Boston, MA. 1993; 255–275.
- 26. Centers for Disease Control and Prevention. CDC Growth Charts: United States. Available at: http://www.cdc.gov/growthcharts/ Accessed: December 2018.
- 32. Spear LP. The psychobiology of adolescence. In: Kline K, editor. Authoritative Communities: The Scientific Case for Nurturing the Child (The Search Institute Series on Developmentally Attentive Community and Society). Springer; New York, NY: 2007. pp. 263–280.
- 40. Wayne P. Defining, establishing, and verifying reference intervals in the clinical laboratory: approved guideline third edition. CLSI document C28-A3c 3rd ed Wayne (PA): Clinical and Laboratory Standards Institute. 2008.
- 59. Government of India, Sample Registration System Baseline Survey 2014. Online at https://data.gov.in/ , accessed 26 April 2018.
- Search Menu
- Sign in through your institution
- Advance Articles
- Collections
- Focus Collections
- Browse by cover
- High-Impact Research
- Founders' Reviews
- Author Guidelines
- Quick and Simple Author Support
- Focus Issues Call for Papers
- Submission Site
- Open Access Options
- Self-Archiving Policy
- Why Publish with Us?
- About Plant Physiology
- Editorial Board
- Advertising & Corporate Services
- Journals on Oxford Academic
- Books on Oxford Academic
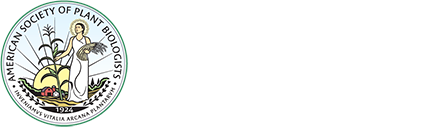
Article Contents
Materials and methods, acknowledgments, literature cited.
- < Previous
Biochemical and Genetic Analysis of the Effects of Amylose-Extender Mutation in Rice Endosperm
Corresponding author; e-mail [email protected] ; fax 81–92–642–3056.
Article, publication date, and citation information can be found at www.plantphysiol.org/cgi/doi/10.1104/pp.010127 .
- Article contents
- Figures & tables
- Supplementary Data
Aiko Nishi, Yasunori Nakamura, Naoki Tanaka, Hikaru Satoh, Biochemical and Genetic Analysis of the Effects of Amylose-Extender Mutation in Rice Endosperm, Plant Physiology , Volume 127, Issue 2, October 2001, Pages 459–472, https://doi.org/10.1104/pp.010127
- Permissions Icon Permissions
Biochemical analysis of amylose-extender ( ae ) mutant of rice ( Oryza sativa ) revealed that the mutation in the gene for starch-branching enzyme IIb (BEIIb) specifically altered the structure of amylopectin in the endosperm by reducing short chains with degree of polymerization of 17 or less, with the greatest decrease in chains with degree of polymerization of 8 to 12. The extent of such change was correlated with the gelatinization properties of the starch granules, as determined in terms of solubility in urea solution. The ae mutation caused a dramatic reduction in the activity of BEIIb. The activity of soluble starch synthase I (SSI) in the ae mutant was significantly lower than in the wild type, suggesting that the mutation had a pleiotropic effect on the SSI activity. In contrast, the activities of BEI, BEIIa, ADP-Glc pyrophosphorylase, isoamylase, isoamylase, pullulanase, and Suc synthase were not affected by the mutation. Therefore, it is stressed that the function of BEIIb cannot be complemented by BEIIa and BEI. These results strongly suggest that BEIIb plays a specific role in the transfer of short chains, which might then be extended by SS to form the A and B 1 chains of amylopectin cluster in rice endosperm.
Starch is composed of two types of molecule, namely amylose and amylopectin. Amylose is an essentially linear molecule composed of α(1→4)-linked glucosidic chains, whereas amylopectin is a highly branched glucan with α(1→6) glucosidic bonds that connect linear chains. The α-1,4 chains of amylopectin consist of A chains that carry no additional chains, B chains that carry A chains or other B chains, and a C chain that includes the reducing terminus ( Peat et al., 1952 ). Hizukuri (1986) proposed a cluster model for amylopectin. In this model, A and B 1 chains form a single cluster, whereas B 2 and B 3 chains extend to two and three clusters, respectively. Hanashiro et al. (1996) proposed that, in amylopectin, chains of degree of polymerization (DP) ≦12, 13 ≦ DP ≦ 24, 25 ≦ DP ≦ 36, and DP ≧ 37 correspond to A chains, B 1 chains, B 2 chains, and B 3 and longer chains, respectively.
Amylose is synthesized by ADP-Glc pyrophosphorylase (AGPase) and granule-bound starch synthase I (GBSSI), which is encoded by the Waxy gene. Amylopectin is synthesized by concerted reactions catalyzed by AGPase, soluble starch synthase (SS), starch-branching enzyme (BE), and starch-debranching enzyme. BE is the only enzyme that can introduce α-1,6-glucosidic linkages into α-polyglucans and, therefore, it plays an essential role in the biosynthesis of amylopectin. The BEs from various higher plants appear to be composed of two types, namely BEI and BEII from maize ( Zea mays ; Boyer and Preiss, 1978a ; Fisher and Boyer, 1983 ; Guan and Preiss, 1993 ; Guan et al., 1997 ), wheat ( Triticum aestivum ; Morell et al., 1997 ), and barley ( Hordeum vulgare ; Sun et al., 1997 ), and the A-type and B-type from pea ( Pisum sativum ; Burton et al., 1995 ; Martin and Smith, 1995 ) and potato ( Solanum tuberosum ; Larsson et al., 1996 , 1998 ). At least three isoforms of BE have been identified in rice ( Oryza sativa ) endosperm ( Mizuno et al., 1992 ; Nakamura et al., 1992 ). Yamanouchi and Nakamura (1992) resolved the BE of the developing endosperm, leaf blade, leaf sheath, culm, and root of rice into two types, BEI and BEII. The BEI type consists of only a single isoform, whereas the BEII type consists of multiple isoforms. The endosperm contains the two isoforms BEIIa (QEIIb) and BEIIb (QEIIa). BEIIb has been detected only in the endosperm, whereas BEIIa has been found in all organs.
Biochemical analysis of purified isoforms of BEI and BEII from maize endosperm indicated that BEI preferentially branches amylose-type, fewly branched polyglucans, as compared with BEII, whereas BEII has a higher capacity than BEI for branching amylopectin-type, highly branched glucans ( Guan and Preiss, 1993 ; Takeda et al., 1993 ; Guan et al., 1997 ). These observations strongly suggest that BEI and BEII might play distinct roles in the construction of amylopectin molecules. However, the significance of the multiple isoforms of BEII have not yet been clarified.
BEIIb-deficient mutants have been isolated from maize and pea. In maize, they have been designated as amylose-extender ( ae ) mutants and have detected in the endosperm ( Boyer and Preiss, 1978b ; Stinard et al., 1993 ). The corresponding rugosus ( r ) mutants in pea have been detected in the embryos ( Bhattacharyya et al., 1990 ). Starches from both ae and r mutants are characterized by a high amylose content ( Hilbert and MacMasters, 1946 ; Banks et al., 1974 ). The average chain length of amylopectin in ae maize endosperm is significantly longer than that of normal amylopectin ( Baba and Arai, 1984 ). In addition, the temperatures for the initiation of gelatinization of starch granules from ae maize and r pea are higher than those of the normal starches ( Yuan et al., 1993 ; Bogracheva et al., 1995 ). The starch granules from amylomaize are also very resistant to disintegration in a concentrated solution of urea ( Baba et al., 1983 ). These observations indicate that mutation of genes for BE leads to alterations in the structure of amylopectin and to rheological changes in starch. It has been reported that gelatinization properties are affected by the fine structure of amylopectin ( Sanders et al., 1990 ; Tester and Morrison, 1990 ; Yuan et al., 1993 ; Jane et al., 1999 ), but the relationships between starch-synthesizing enzymes, the fine structure of amylopectin, and gelatinization properties have not been fully clarified. Studies of BE-deficient mutations provide clues to the roles of BE in the synthesis of amylopectin.
The ae starch in rice endosperm has a higher gelatinization temperature and the amylopectin has longer α-1,4-glucan chains than those of the wild-type starch and amylopectin ( Yano et al., 1985 ). We do not understand, however, the way in which biochemical changes affect the structure of starch granules and the functional properties of starch. Mizuno et al. (1993) showed that BEIIb is deficient in ae rice mutants, whereas the level of the BEI transcript is apparently unaffected by the mutation. These observations suggest that the ae mutation might be useful in attempts to clarify not only the role of BEIIb in the biosynthesis of amylopectin in rice but also the effects of the fine structure of amylopectin on the physicochemical properties of starch.
In the present investigations, to eliminate the effects of amylose on the physicochemical properties of starch granules in ae mutant, we produced amylose-free ae mutant lines by crossing ae and waxy ( wx ) mutants. In addition, to examine the effect of the level of BEIIb on the synthesis of amylopectin, we performed reciprocal crosses to generate F 1 seeds with different doses of the Ae allele.
In the present study, we measured gelatinization properties of endosperm starches from ae and amylose-free ae mutant lines using various concentrations of urea in solution. We examined the relationship between the gelatinization properties of starch granules and the structure of amylopectin. To assess the metabolic role of BEIIb, we analyzed the effects of ae mutation on activities of other isoforms of BE, namely BEI and BEIIa, and of starch- and Suc-metabolizing enzymes, such as SS, AGPase, isoamylase, pullulanase, and Suc synthase.
In the present paper, the Ae protein of rice is referred to as starch-branching enzyme IIb (BEIIb) as is that of maize ( Preiss and Levi, 1980 ; Stinard et al., 1993 ). This enzyme in rice has also been referred to as QEIIa ( Yamanouchi and Nakamura, 1992 ) or RBE3 ( Mizuno et al., 1993 ). The other type of BEII, which is present in all rice tissues, including the developing endosperm and green leaves ( Yamanouchi and Nakamura, 1992 ), is referred to as BEIIa. This enzyme has been called QEIIb ( Yamanouchi and Nakamura, 1992 ) or RBE4 ( Mizuno et al., 1992 ).
Effects of the Concentration of Urea on Gelatinization of Samples of Endosperm Starch from ae Mutant and the Parental Rice Cultivar
Iodine-staining properties of endosperm starch from ae and amylose-free ae mutant lines
Line . | Genotype . | BEIIb . | GBSSI . | λ . | B.V. . | Apparent Amylose Content . |
---|---|---|---|---|---|---|
Kinmaze | 561 | 0.42 | 15.7 | |||
EM10 | – | 574 | 0.59 | 26.5 | ||
EM21 | – | 521 | 0.16 | 0.3 | ||
AMF44 | – | – | 553 | 0.28 | 7.5 |
Line . | Genotype . | BEIIb . | GBSSI . | λ . | B.V. . | Apparent Amylose Content . |
---|---|---|---|---|---|---|
Kinmaze | 561 | 0.42 | 15.7 | |||
EM10 | – | 574 | 0.59 | 26.5 | ||
EM21 | – | 521 | 0.16 | 0.3 | ||
AMF44 | – | – | 553 | 0.28 | 7.5 |
The B.V. (680) showed the absorbance at 680 nm of the starch-iodine complex.
The apparent amylose content was determined by the iodine method ( Juliano, 1971 ), on the base of calibration line, which was obtained from the blue values by changing the ratio of maize ( Zea mays ) amylose and rice amylopectin in the iodine solution.
AMF44 was a double-recessive mutant line, with the genotype aeae/wxwx , which was derived from a cross between the ae mutant EM10 and the wx mutant EM21. See text for details.

Effects of the concentration of urea on gelatinization of starch granules from the ae mutant EM10 and the wild-type cv Kinmaze and from lines with the ae and Ae genes on the waxy background. After incubation of starch granules in solutions of urea for 24 h, swelling was examined by measuring the volume of the swollen starch sediment. ■, Kinmaze (wild type, AeAe/WxWx ); ●, EM21 ( wx mutant, AeAe/wxwx ); ▵, EM10 ( ae mutant, aeae/WxWx ); ♦, AMF44 (double-recessive mutant, aeae/wxwx ). Results are means ± sd of results from three replicate experiments.
Effect of the ae Mutation on the Fine Structure of Amylopectin in the Mature Endosperm

Distribution of chain length of total α-polysaccharides from wild-type, ae , wx , and aeae/wxwx rice endosperm as determined by HPAEC-PAD. Amylopectin was debranched with isoamylase from P. amyloderamosa , reduced with sodium borohydride, and then fractionated on a Carbopac PA1 column. The α-1,4-glucan chains were eluted with a gradient of sodium hydroxide and sodium acetate and monitored with a PAD. A, The distribution of α-1,4-glucan chains in amylopectin from the wild type ( AeAe/WxWx ) and the ae mutant ( aeae/WxWx ). B, The difference in amylopectin chain lengths between the ae mutant and the wild type. The columns show the area of each peak for the ae mutant minus the area of the corresponding peak with the same DP for the wild type. C, The distribution of α-1,4-glucan chains in amylopectin from the wx mutant ( AeAe/wxwx ) and the double-recessive mutant ( aeae/wxwx ). D, The difference in amylopectin chain lengths between the double mutant and the wx mutant. The results show representatives from three experiments that gave similar results.
Dosage Effects of the Ae Locus
The ae mutation of rice is a mutation of a structural gene for the endosperm-specific branching enzyme BEIIb (RBE3; Mizuno et al., 1993 ). Because the endosperm is the triploid tissue, we can generate F 1 seeds with different doses of the Ae allele by making reciprocal crosses between the Kinmaze and EM10 lines. It seems likely that the gene dosage effect might be useful to understand the effects of the level of BEIIb protein on the proportion of short chains in amylopectin, on the gelatinization properties of starch granules in urea, and on the starch content.
Levels of the BEIIb

Gene dosage effects of the Ae allele on the level and activity of BEIIb, chain length distribution, gelatinization properties, and grain morphology. A, Western-blotting analysis of BEIIb in mature rice kernels. Protein was extracted from 20 mg of rice powder. The immunoblot was developed with antiserum raised against BEIIb from rice endosperm ( Nakamura et al., 1992 ) at a dilution of 1:500. B, Native PAGE/activity stainings of BEs (left) and endogenous phosphorylase (right) in the endosperm of four genotypes. The migration and identification of each band corresponding to three BE isoforms (BEI, BEIIa, and BEIIb) and phosphorylase were according to our previous report ( Yamanouchi and Nakamura, 1992 ). The volumes of crude enzyme extract applied were 0.67 and 6.7 μL for BE and phosphorylase, respectively. Note that the phosphorylase band was not detected under the lower protein concentration (0.67 μL of crude extract). C, Differences in the distribution of α-1,4-glucan chains among the four genotypes. The columns show the peak areas for each glucan chain from each genotype minus that from the wild-type cv Kinmaze. The sd was given from three separate experiments. D, Effects of 4 m urea on the swelling of starch granules from the endosperm of four genotypes. Ten milligrams of rice powder in an Eppendorf tube was mixed with 0.5 mL of 4 m urea, and shaken for 24 h at 25°C. After centrifugation, samples were allowed to stand for 1 h. E, Kernels from the four genotypes. a through d, Results for the four genotypes, AeAeAe , AeAeae , Aeaeae , and aeaeae , respectively.
Gene dosage effects of the Ae allele on the various parameters of rice endosperm
Genotype . | Combination . | Relative BEIIb Content . | . | Concentration of Urea . | Grain Size . | |||
---|---|---|---|---|---|---|---|---|
Wt . | Length . | Width . | Thickness . | |||||
Kinmaze | 100 | 75.4 ± 0.8 (10) | 4 | 23.7 ± 1.6 (100) | 5.3 ± 0.2 | 3.0 ± 0.2 | 2.1 ± 0.2 | |
Kinmaze × EM10 | 74 | 74.1 ± 1.4 (8) | 4 | 23.4 ± 1.4 (99) | 5.2 ± 0.2 | 3.0 ± 0.2 | 2.1 ± 0.1 | |
EM10 × Kinmaze | 26 | 71.7 ± 0.3 (6) | 4 | 23.0 ± 1.1 (97) | 5.1 ± 0.1 | 2.8 ± 0.1 | 2.0 ± 0.2 | |
EM10 | 0 | 65.8 ± 1.7 | 8 | 15.5 ± 1.7 (65) | 4.8 ± 0.3 | 2.6 ± 0.2 | 1.7 ± 0.1 |
Genotype . | Combination . | Relative BEIIb Content . | . | Concentration of Urea . | Grain Size . | |||
---|---|---|---|---|---|---|---|---|
Wt . | Length . | Width . | Thickness . | |||||
Kinmaze | 100 | 75.4 ± 0.8 (10) | 4 | 23.7 ± 1.6 (100) | 5.3 ± 0.2 | 3.0 ± 0.2 | 2.1 ± 0.2 | |
Kinmaze × EM10 | 74 | 74.1 ± 1.4 (8) | 4 | 23.4 ± 1.4 (99) | 5.2 ± 0.2 | 3.0 ± 0.2 | 2.1 ± 0.1 | |
EM10 × Kinmaze | 26 | 71.7 ± 0.3 (6) | 4 | 23.0 ± 1.1 (97) | 5.1 ± 0.1 | 2.8 ± 0.1 | 2.0 ± 0.2 | |
EM10 | 0 | 65.8 ± 1.7 | 8 | 15.5 ± 1.7 (65) | 4.8 ± 0.3 | 2.6 ± 0.2 | 1.7 ± 0.1 |
The BEIIb content was determined from the intensity of the immunoreactive band (Fig. 3 A) with a densitometer.
The percentage of short chains with DP ≦ 17 within a cluster (DP ≦ 17/DP ≦ 24; Hanashiro et al., 1996 ) is given as the mean ± sd of results of three separate experiments. The difference from the aeaeae genotype (EM10) is indicated in parentheses.
The lowest concentration of urea for gelatinization of starch granules.
Details of grain size are given as means ± sd of results of more than seven separate sets of measurements of mature seeds. The kernel wt is given as a percentage of the wild-type wt in parentheses.
Distribution of Chain Length in Amylopectin
We observed a marked difference among the gene dosage groups in terms of the profiles of chain lengths in the isoamylolysates of amylopectin from rice endosperm (Fig. 3 C). Proportions of short chains, in particular those with DP ≦ 17, were remarkably depressed with decreases in the number of Ae alleles. All changes in the proportion of short chains of amylopectin were reproducible and reliable although the change was most marked in the nulliplex condition. Although a gene dosage effect of the Ae allele was apparent in the four genotypes, the extent of the gene dosage effect on the chain length distribution was not necessarily related to the effect on the level of BEIIb itself (Fig. 3 and Table II ). Hizukuri (1986) proposed that A and B 1 chains form a single cluster, and Hanashiro et al. (1996) classified A chains as those with DP ≦ 12 and B 1 chains as those with 12 ≦ DP ≦ 24. Based on this classification, we can define a single cluster as one that consists of chains with DP ≦ 24. The proportion of short chains with DP ≦ 17 within a single cluster was 75% in the wild type ( AeAeAe ), 74% in the duplex ( AeAeae ), 72% in the simplex ( Aeaeae ), and 66% in the nulliplex ( aeaeae ) genotype (Table II ). Thus, the proportion of short chains with DP ≦ 17 increased with increasing number of the Ae allele but the relation was not linear.
Gelatinization Properties
The starch granules from the wild type were gelatinized in 4 m urea, whereas those of EM10 were scarcely gelatinized at all in 4 m urea, as shown in Figure 1 . Although the granules of endosperm starch from the duplex ( AeAeae ) genotype were gelatinized in 4 m urea, the solubility, as determined by the volume of the sediment, was slightly lower than that of the wild type, corresponding to about 78% of the wild-type volume (Fig. 3 D). Starch granules from the simplex ( Aeaeae ) genotype were less gelatinized than those of the wild type, and the solubility was only 57% of the wild-type value (Fig. 3 D). These results are consistent with the gene dosage effects of the Ae allele on the chain length distribution of amylopectin.
Morphological Phenotype
Figure 3 E shows the dosage effects of the Ae allele on the phenotype of the entire mature kernel. The kernels of the ae mutant EM10 were markedly smaller than the wild type, and the dry weight was 65% of the wild-type dry weight (Table II ). The weights of the duplex ( AeAeae ) and simplex ( Aeaeae ) kernels were 99% and 97% of the wild-type weight, respectively. These results are also consistent with the gene dosage effects of the Ae allele on chain length distribution and gelatinization properties (Table II ).
In the F 2 population, the deficiency of BEIIb was always associated with the high resistance to gelatinization in urea and smaller kernels, and no segregants were found among them (data not shown). These results indicate that these characteristics, observed in the ae mutant line EM10, are caused by the ae gene. Thus, we can conclude that BEIIb, encoded by the Ae gene, plays a major role in the formation of short chains with DP ≦ 17 that are included in a single cluster of amylopectin, and, furthermore, that the reduction in proportion of short chains with DP ≦ 17 in the ae mutants is responsible for the insolubility of ae starch granules in a solution of urea.
Although the level of BEIIb increased approximately linearly with increasing numbers of Ae alleles, the proportion of short chains with DP ≦ 17, the gelatinization properties, and the starch content of kernels did not increase in parallel with the increase in number of the Ae allele. This observation suggests that BEIIb activity might not be the rate-limiting factor and that BEIIb might be present in excess relative to the rate of starch synthesis in the wild-type endosperm.
Effects of ae Mutation on Levels of Enzymes for the Metabolism of Starch and Suc
Effects of the ae mutation on the activities of enzymes involved in the metabolism of starch and Suc in developing rice endosperm
Enzyme . | Enzymatic Activity (nmol min per endosperm) . | |
---|---|---|
Kinmaze . | EM10 . | |
Starch synthase | ||
Citrate, glycogen | 4.78 ± 0.2 (100) | 2.71 ± 0.1 (57) |
Citrate, −glycogen | 3.78 ± 0.3 (100) | 1.30 ± 0.1 (34) |
−Citrate, glycogen | 0.70 ± 0.1 (100) | 0.21 ± 0.1 (30) |
AGPase | 44.8 ± 2.3 (100) | 56.7 ± 1.6 (127) |
Pullulanase | 14.6 ± 1.1 (100) | 14.5 ± 0.2 (99) |
Suc synthase | 28.5 ± 1.6 (100) | 35.7 ± 1.0 (125) |
Enzyme . | Enzymatic Activity (nmol min per endosperm) . | |
---|---|---|
Kinmaze . | EM10 . | |
Starch synthase | ||
Citrate, glycogen | 4.78 ± 0.2 (100) | 2.71 ± 0.1 (57) |
Citrate, −glycogen | 3.78 ± 0.3 (100) | 1.30 ± 0.1 (34) |
−Citrate, glycogen | 0.70 ± 0.1 (100) | 0.21 ± 0.1 (30) |
AGPase | 44.8 ± 2.3 (100) | 56.7 ± 1.6 (127) |
Pullulanase | 14.6 ± 1.1 (100) | 14.5 ± 0.2 (99) |
Suc synthase | 28.5 ± 1.6 (100) | 35.7 ± 1.0 (125) |
The values represent means ± sd of results of three separate experiments. The enzymatic activity obtained in each experiment was the mean of the results of three replicate incubations. The value as a percentage of the wild-type Kinmaze value is shown in parentheses.

Effects of the ae mutation on other starch-synthesizing enzymes. A, Native PAGE/activity staining of SS in the endosperm of ae mutant EM10 and the wild-type cv Kinmaze. Each lane contained 7.5 μL multiplied by the number given above the lane of the crude enzyme extract. The migration of each band corresponding to two SS isoforms (SSI and SSIII) was according to Abel et al. (1996) . B, SDS-PAGE of total protein in rice mature kernel. C, Western-blotting analysis of BEI in mature rice kernels. The immunoblot was developed with antiserum raised against BEI from rice endosperm ( Nakamura et al., 1992 ) at a dilution of 1:1,000.
Effect of ae Mutation on SSs
In an attempt to determine the isoform of SS that is affected by the ae mutation, the activity of each SS isoform was measured after the separation by native PAGE. Figure 4 A shows that the SSI isoform accounted for the bulk of the total SS activity in rice endosperm, and that the activity of SSI was distinctly lower (about 50%) in the mutant than that in the wild type. Figure 4 A shows that even in the absence of glycogen as glucan primer, 0.5 m citrate supported the SSI activity as in the case of SSI from maize endosperm ( Boyer and Preiss, 1981 ). This primer-independent SSI activity was markedly lower in EM10 than in Kinmaze (Table III and Fig. 4 A, left). The reduction of SSI activity was also observed in the presence of glycogen (Table III and Fig. 4 A, middle and right). Because BEIIa and BEIIb bands as well as the BEI band were separated from the SSI band, the reduction of SSI activity was not caused by the omission of BE isoforms (data not shown). The deduced SSIII isoform was also present in the rice endosperm, but its activity was to a markedly lesser extent than that of SSI. The SSIII activity in the mutant was at the same or slightly higher level as compared with that in the wild type. Therefore, the decrease in the total activity of SS caused by ae mutation was at least mainly due to decrease in the SSI activity.
Effects of ae Mutation on Levels of Transcripts for Starch-Synthesizing Enzymes

Northern-blot analyses of BEI, BEIIa, BEIIb, SSI, and SSIII transcripts in ae mutant EM10 and the wild-type cv Kinmaze. Total RNA from developing grains, stages I, II, III, and IV, were blotted and probed with specific RNA probes. The lower portion of the figure shows the ethidium bromide-stained RNA gel.
There have been a number of studies with BE-deficient mutants from various plant species. It is known that the ae mutant in maize ( Stinard et al., 1993 ) and the r mutant in pea ( Bhattacharyya et al., 1990 ) are caused by lesions in the gene coding for the BEII-type or BE-A-type enzyme. Mizuno et al. (1993) also showed that BEIIb is deficient in ae rice mutants. Our present investigations strongly suggest that the abnormalities of the endosperm starch of ae rice, such as the high affinity for iodine and the apparently high amylose content, are caused by an altered amylopectin structure that is due to mutation in a gene for BEIIb (Table I , Figs. 1-3 ). Rice ae mutants have been classified in the same type of BE-deficient mutants from other plant species; for example, ae in maize and r in pea, which are characterized by an apparent increase in the amount of amylose in the storage starch ( Banks et al., 1974 ; Jane et al., 1999 ). However, the blue value and λ-max after I 2 /KI staining of endosperm starch of the ae mutant with an amylose free waxy background were significantly higher than those of the wild type (Table I ), indicating that the apparently elevated amylose content of ae mutants of rice was not caused by an increase in the net amylose content but by the proposed abnormal structure of the amylopectin.
The structure of amylopectin from BE-deficient mutants is clearly distinct from the wild-type structure in various plants. Earlier analysis of chain length distribution showed that the proportion of short chains is markedly lower, whereas that of the longest chains is elevated in the amylopectins from maize ae endosperm ( Kasemsuwan et al., 1995 ) and pea r embryos ( Tomlinson et al., 1997 ). The present study specified the effect of ae mutation on the fine structure of amylopectin by showing that the proportion of short chains with DP ≦ 17 and, in particular, of chains with DP 8 to 12 was remarkably reduced in the amylopectin from the endosperm of rice ae mutant (Fig. 2 and Table II ). The specific role of BEIIb is also supported by the good correlations among the gene dosage effects of the Ae gene, the level of BEIIb itself, and the proportion of short chains with DP ≦ 17 (Fig. 3 , A–C). These results indicate that the proportion of outer chains, that is to say, mainly the A chains of a cluster, is specifically reduced in ae mutants of rice. The evidence is consistent with the reports that, in maize endosperm, BEI predominantly produces longer chains (DP ≧ 10), whereas BEII preferentially transfers short chains (3 ≦ DP ≦ 9; Guan and Preiss, 1993 ; Takeda et al., 1993 ; Guan et al., 1997 ).
Several investigations have reported that the side chains (A and B 1 chains) of a unit cluster of amylopectin form double helices although the minimum chain length for such a double helix might be approximately 10 Glc units, and the length of each double helix correlated with the gelatinization temperature ( Gidley and Bulpin, 1987 ; Cooke and Gidley, 1992 ; Gidley et al., 1995 ; Moates et al., 1997 ; Safford et al., 1998 ). The gelatinization properties in the gene dosage group were correlated with the extent of the decrease in the short chains with DP ≦ 17 (Fig. 3 , C and D). Thus, we conclude that the gelatinization behavior of starch granules in rice is predominantly, if not exclusively, determined by the proportion of short chains to long chains within a single cluster of amylopectin.
In rice endosperm, the activity of BEI is markedly higher than that of the total BEII (BEIIa and BEIIb) when measured by the phosphorylase a stimulation assay ( Yamanouchi and Nakamura, 1992 ). The present study showed that the activity of BEI in rice endosperm was unaffected by the ae mutation (Fig. 3 B), suggesting that the metabolic role of BEIIb cannot be compensated by the function of BEI. In cereal endosperm, there are two distinct forms of BEII, namely BEIIa and BEIIb ( Boyer and Preiss, 1978a ; Yamanouchi and Nakamura, 1992 ; Sun et al., 1998 ). BEIIb is specifically or predominantly found in the endosperm, whereas BEIIa is present in almost all tissues of maize ( Fisher et al., 1996 ; Gao et al., 1997 ), barley ( Sun et al., 1998 ), and rice ( Yamanouchi and Nakamura, 1992 ; Mizuno et al., 1993 ). Although there are some differences in terms of amino acid sequence between BEIIa and BEIIb, several investigators ( Guan and Preiss, 1993 ; Takeda et al., 1993 ; Mizuno et al., 2001 ) reported that the substrate specificities of the two enzymes are indistinguishable. The critical decreases in the proportion of short chains with DP ≦ 17 (Figs. 2 and 3 C), despite the maintenance of normal BEIIa activity (Fig. 3 B), indicate that the function of BEIIb cannot be compensated by BEIIa during the biosynthesis of amylopectin in rice endosperm.
In the ae mutant, both grain weight and the proportion of short chains with DP ≦ 17 were markedly lower than in the wild type (Table II ). Isoforms of BE in rice belong to the family of amylolytic enzymes ( Mizuno et al., 1992 ), cleaving α-1,4-glucosidic linkages and transferring the newly formed nonreducing ends to other α-1,4-linked chains ( Borovsky et al., 1976 ). Thus, the actions of BE result in an increase in the number of nonreducing ends to which Glc moieties from ADP-Glc are bound in the reaction catalyzed by the SS. Therefore, we speculate that the reduction in the activity of BEIIb in the ae mutants caused decreases in the number of reducing ends that, in turn, resulted in a decrease in the synthesis of starch.
The significant proportions of short chains in the amylopectin of wild-type japonica rice cv Kinmaze suggest that the activity of BEIIb for synthesizing short chains of the new amylopectin cluster is in excess of the amylopectin biosynthesis, and that the capacity of some form(s) of SS for elongation of short chains within the amylopectin cluster might be a rate-limiting factor in the endosperm. On the other hand, the activity of BEIIb is greatly depressed in ae mutants and the number of short chains transferred within the cluster is considered to be markedly reduced. These short chains are then fully elongated by SS(s). The result alters the pattern of side chains from the normal pattern to the ae -type pattern with a considerable decrease in the proportion of short chains (DP ≦ 17).
The activity of SS in the ae mutant EM10 was markedly decreased as compared with that of the wild type, whereas the activities of the other starch-synthesizing enzymes, such as AGPase, pullulanase, and Suc synthase, were unaffected (Table III ). The decrease in the total activity of SS induced by ae mutation was due to the decrease in SSI by approximately 50%, the major SS isoform in rice endosperm (Fig. 4 A). The result seems to be consistent with the previous report by Boyer and Preiss (1978b) that the ae mutation of maize causes a reduction in the activity of SSI, as well as in the activity of BEIIb, whereas the activity of SSII is unaffected in the mutant endosperm. These results might suggest that BEIIb is associated with SSI in maize and rice endosperm.
The gelatinization property is one of the most important rheological indicators of the cooking quality and processing characteristics of rice starch. Numerous investigations have shown that the rheological properties of starch, such as gelatinization, retrogradation, and pasting properties are affected by amylopectin structure ( Kalichevsky et al., 1990 ; Sanders et al., 1990 ; Tester and Morrison, 1990 ; Shi and Seib, 1992 ; Yuan et al., 1993 ; Lu et al., 1997 ; Safford et al., 1998 ; Jane et al., 1999 ). It has been noted that there is a correlation between the crystalline structure of starch and its rheological properties and that A chains play an important role of the formation of the crystalline structure. Jane et al. (1999) reported that the chain length and distribution of amylopectin branches determine the gelatinization temperature of starch, enthalpy changes, and pasting properties, and that the gelatinization temperature of starch increases with increasing the chain length. The results and the present studies support the view that alterations in amylopectin structure, in particular in short chains within clusters, might play a critical role in the rheological properties of starch. It is, therefore, likely that genetic modification of the gene for BEIIb will lead to the synthesis of novel types of starch as new materials for the food and starch industries.
Plant Materials
In this study, we used an amylose-extender ( ae ) mutant line EM10 that was generated by treatment of fertilized egg cells of japonica rice ( Oryza sativa ) cv Kinmaze ( Satoh and Omura, 1979 ) with N -methyl- N -nitrosourea. We also used an amylose-free ae mutant line, AMF44, in which the endosperm starch consists of only ae mutant-type amylopectin. AMF44 is a double-recessive mutant for ae and waxy ( wx ), with the aeae/wxwx genotype. It was derived from a cross between the ae mutant line EM10 and the wx mutant line EM21. The endosperm starch of EM21 contains no amylose and consists solely of amylopectin. The original parental cv Kinmaze and the wx mutant line EM21 were used as controls. For analysis of gene dosage effects, F 1 seeds with two and one copies of the Ae allele were generated by reciprocal crosses between Kinmaze and EM10.
Determination of Apparent Amylose Content
Twenty milligrams of starch was gelatinized by treatment with 2 mL of 1 n NaOH and stood for 24 h at room temperature. After addition of 4 mL of 1 n CH3COOH and 4 mL of distilled water, the solution was homogenized. An aliquot (0.8 mL) of the solution was taken and added by 0.2 mL of 0.2% (w/v) I 2 , 2% (w/v) KI, and 4 mL of distilled water. An A 680 (the blue value) and λ-max were measured. The apparent amylose content was determined according to the method of Juliano (1971) , on the base of calibration line, which was obtained from the blue value at 680 nm by changing the ratio of maize ( Zea mays ) amylose and rice amylopectin in the iodine solution.
Measurement of Gelatinization Properties
Five kernels of mature seeds of average size, which had been removed from embryos with forceps, were ground with a mortar and pestle, and 20 mg of the powder was mixed with 1 mL of a solution of 0 to 9 m urea, the pH of which had been adjusted to 6.0 with acetic acid, in an Eppendorf tube. The mixture was incubated at 25°C for 24 h. The suspension was centrifuged for 20 min at 8,000 g at room temperature and then allowed to stand for 1 h. The solubility of the granules in the urea solution was expressed in terms of the volume of the swollen sediment, which was calculated by subtracting the volume of supernatant from the urea solution (1 mL).
Determination of the Distribution of Lengths of α-1,4-Glucan Chains in α-Polysaccharides by HPAEC-PAD
The embryo and pericarp were removed from three dehulled grains of average size. The endosperms were ground with a mortar and pestle and 5 mg of the resulting powder were suspended in 5 mL of methanol and boiled for 10 min. The homogenate was centrifuged at 2,500 g for 5 min. The pelleted polyglucan was washed twice with 5 mL of 90% (v/v) methanol, suspended in 5 mL of distilled water, and then boiled for 60 min. An aliquot (1.0 mL) of the sample of gelatinized polyglucan was added to 50 μL of 600 m m sodium-acetate buffer (pH 4.4) and 10 μL of 2% (w/v) NaN 3 and then hydrolysis was achieved by addition of 10 μL of isoamylase from Pseudomonas amyloderamosa (1,400 units; Seikagaku Corporation, Tokyo) and incubation at 37°C for 24 h. The hydroxyl groups of the debranched glucans were reduced by treatment with 0.5% (w/v) of sodium borohydride under alkaline conditions for 20 h by the method of Nagamine and Komae (1996) .
The preparation was dried in vacuo at room temperature and allowed to dissolve in 20 μL of 1 m NaOH for 60 min. Then, the solution was diluted with 180 μL of distilled water. An aliquot (25 μL) of the preparation was injected into a BioLC (System model DX-500, Dionex, Sunnyvale, CA) equipped with a PAD and a Carbopac PA-1 column (4-mm i.d.× 25 cm). Size fractionation of α-1,4-glucans was performed with a linear gradient of sodium acetate (50–500 m m ) in 0.1 m NaOH at a flow rate of 1 mL min −1 .
Extraction of Proteins from Mature Seeds
The protein from mature seeds was analyzed for the gene dosage effect of the Ae allele on the level of BEIIb. Twenty milligrams of mature seed were powdered with a pair of pliers and added to 700 μL of sample buffer, which contained 4% (w/v) SDS, 4 m urea, 5% (v/v) 2-mercaptoethanol, and 0.125 m Tris-HCl (pH 6.8). After shaking at room temperature for 12 h, the sample was centrifuged at 10,000 g for 20 min at 4°C. An aliquot of the supernatant (10 μL) was taken and subjected to SDS-PAGE.
Western Blotting
SDS-PAGE was performed on a resolving gel (6 × 9 × 0.1 cm 3 ) prepared with 10% (w/v) acrylamide and 0.035% (w/v) bisacrylamide as described by Laemmli (1970) . The bands of proteins were blotted onto a polyvinylidenedifluoride membrane (Millipore, Bedford, MA) with a transblotter (Nihon Eido Co., Tokyo) and the membrane was incubated with rabbit antiserum that contained polyclonal antibodies raised against purified BEIIb and BEI from developing rice endosperm ( Nakamura et al., 1992 ). Immunoreactive proteins were detected by incubation with horseradish peroxidase-conjugated antibodies against rabbit IgG (Bio-Rad, Hercules, CA) and finally with 4-chloro-1-naphthol, as described by Towbin et al. (1979) .
Preparation of Enzymes Involved in the Metabolism of Starch and Suc
All the following procedures were carried out at 0°C to 4°C, unless otherwise noted.
For analysis of native-PAGE/activity stainings of BE and phosphorylase, an immature rice grain at the late-milking stage that was removed by the hull, pericarp, and embryo was homogenized with a mortar and pestle in 10-fold volume of a solution, which consisted of 50 m m HEPES ( N -2-hydroxyethylpiperazine- N '-2-ethanesulfonic acid)-NaOH (pH 7.4), 2 m m MgCl 2 , 50 m m 2-mercaptoethanol, and 12.5% (v/v) glycerol. The homogenate was centrifuged twice at 15,000 g for 15 min. The supernatant was used as the crude enzyme extract.
For analysis of native PAGE/activity staining of SS, an immature rice grain at the late-milking stage removed from the hull, pericarp, and embryo was homogenized with a mortar and pestle in the same volume of solution, which consisted of 50 m m Tris-HCl (pH 7.5), 2 m m EDTA-2Na, 5 m m dithiothreitol (DTT), 0.4 m m PMSF, and 10% (v/v) glycerol. The homogenate was centrifuged at 15,000 g for 15 min. The supernatant was used as the crude enzyme extract. The protein content was measured by the method of Bradford (1976) .
For enzyme assay of SS, five immature rice grains at the late-milking stage removed from the hull, pericarp, and embryo were homogenized with a mortar and pestle in 1 mL of the same buffer as described above, and the supernatant was used as the crude enzyme extract.
For assays of the other enzymes, five immature rice grains at the late-milking stage that were removed by the hull, pericarp, and embryo were homogenized with a mortar and pestle in 1 mL of solution, which consisted of 50 m m HEPES-NaOH (pH 7.4), 2 m m MgCl 2 , 50 m m 2-mercaptoethanol, and 12.5% (v/v) glycerol. The homogenate was centrifuged twice at 15,000 g for 15 min. The supernatant was used as the crude enzyme extract.
Native PAGE/Activity Stainings of BE and Phosphorylase
Native PAGE was performed on a slab gel prepared with 5% (w/v; resolving gel) and 3.3% (w/v; stacking gel) acrylamide by a modified version of the method described by Davis (1964) . Electrophoresis was carried out at 4°C at a constant current of 20 mA. After electrophoresis, the gel was rinsed with 20 mL of a solution of 50 m m HEPES-NaOH buffer (pH 7.4) and 20% (v/v) glycerol for 5 min on ice. The gel for detection of BE was incubated for 5 to 6 h at 30°C in 20 mL of the reaction mixture, which consisted of 50 m m HEPES-NaOH buffer (pH 7.4), 50 m m Glc-1-P, 2.5 m m AMP, 10% (v/v) glycerol, and rabbit muscle phosphorylase a (about 50 units; Sigma, St.Louis), whereas the gel for detection of phosphorylase was incubated for 5 to 6 h at 30°C in 20 mL of the same reaction mixture except that rabbit muscle phosphorylase a was omitted. The gels were placed in an iodine solution that consisted of 0.1% (w/v) I 2 and 1% (w/v) KI.
Native PAGE/Activity Staining of SS
Native PAGE was performed on a slab gel prepared with 7.5% (w/v; resolving gel) containing 0% or 0.8% (w/v) oyster glycogen (Type II; Sigma) and 3.3% (w/v; stacking gel) acrylamide by a modified version of the method described by Davis (1964) . Electrophoresis was carried out at 4°C at a constant current of 15 mA. After electrophoresis, the gel was rinsed twice with 35 mL of a solution of 100 m m Bicine-NaOH buffer (pH 7.5), 0.5 m m EDTA, 2 m m DTT, 10% (v/v) glycerol, and either in the presence or absence of 0.5 m citrate-Na buffer (pH 7.5) for 15 min on ice and then it was incubated for 12 h at 30°C in 35 mL of the reaction mixture, which consisted of 100 m m Bicine-NaOH buffer (pH 7.5), 0.5 m m EDTA, 2 m m DTT, 10% (v/v) glycerol, 1 m m ADP-Glc, and either in the presence or absence of 0.5 m citrate-Na buffer (pH 7.5). The gel was placed in an iodine solution that consisted of 0.1% (w/v) I 2 and 1% (w/v) KI.
Assays of Enzymatic Activities
The assay was conducted at 30°C under three different conditions in: (a) 0.5 m citrate-Na (pH 7.5), 50 m m Bicine-NaOH (pH 7.5), 1.7 m m ADP-Glc, 0.7 mg oyster glycogen (Type II, Sigma), 16.7 m m DTT, and the crude enzyme extract in a reaction volume of 300 μL; (b) 0.5 m citrate-Na (pH7.5), 50 m m Bicine-NaOH (pH 7.5), 1.7 m m ADP-Glc, 16.7 m m DTT, and the crude enzyme extract in a reaction volume of 300 μL; or (c) 50 m m Bicine-NaOH (pH 7.5), 1.7 m m ADP-Glc, 0.7 mg oyster glycogen, 16.7 m m DTT, and the crude enzyme extract in a reaction volume of 300 μL. Twenty minutes after the start of the reaction, enzymes were inactivated by placing the mixture in a boiling water bath for 2 min. Then, the mixture was supplemented with 100 μL of a solution of 50 m m HEPES-NaOH (pH 7.4), 10 m m phosphocreatine, 200 m m KCl, 10 m m MgCl 2 , and 10 μL of 5 mg mL −1 creatine phosphokinase (5 units; Type I, Sigma), and incubated for 30 min at 30°C to convert ADP to ATP. The resulting solution was heated in a boiling water bath for 2 min and then subjected to centrifugation at 15,000 g at 4°C for 10 min. The supernatant (300 μL) was mixed with a solution of 125 m m HEPES-NaOH (pH 7.4), 10 m m Glc, 20 m m MgCl 2 , and 150 μg NADP + . The enzymatic activity was recorded as the increase in A 340 after the addition of 1μL each of hexokinase (1.5 units; Roche Diagnostics, Tokyo) and G6P dehydrogenase (0.5 units; Type XV, Sigma).
The assay was conducted at 30°C in 100 m m HEPES-NaOH (pH 7.4), 3 m m 3-phosphoglycerate, 1.2 m m ADP-Glc, 3 m m sodium pyrophosphate, 5 m m MgCl 2 , 4 m m DTT, and the preparation of enzymes in a reaction mixture of 250 μL. Twenty minutes after the start of the reaction, enzymes were inactivated by placing the mixture in a boiling water bath for 2 min. After addition of 350 μL of distilled water, the mixture was subjected to centrifugation at 15,000 g at 2°C for 10 min. The supernatant (500 μL) was mixed with 0.15 mg NADP + . The enzymatic activity was recorded as the increase in A 340 after the addition of 1 μL each of phosphoglucomutase (0.4 units; Roche Diagnostics) and G6P dehydrogenase (0.5 units; Type XV, Sigma).
Pullulanase
The assay was conducted at 40°C in 50 m m MES (2- N -morpholinoethanesulfonic acid)-NaOH (pH 6.2), 2 mg pullulan, 20 m m CaCl 2 , and the preparation of enzyme in a reaction mixture of 200 μL. Twenty minutes after the start of the reaction, enzymes were inactivated by placing the mixture in a boiling-water bath for 2 min. Activity was determined as the increase in level of reducing sugars by measuring A 520 . The method was based on those described by Nelson (1944) and Somogyi (1952) .
Suc Synthase
The assay was conducted at 25°C in 50 m m HEPES-NaOH (pH 7.4), 7.5 m m UDP-Glc, 7.5 m m Fru, 15 m m MgCl 2 , and the preparation of enzyme in a reaction mixture of 140 μL. After 10 min, the reaction was terminated by placing the mixture in a boiling water bath for 2 min and then it was centrifuged at 13,000 g at 2°C for 5 min. The supernatant (100 μL) was mixed with a solution of 15 m m HEPES-NaOH (pH 8.0), 0.4 m m phosphoenolpyruvate, 5 m m KCl, 1 m m MgCl 2 , and 0.1 m m NADH. The enzymatic activity was determined as the increase in A 340 after the addition of 5 μL pyruvate kinase (2 units; Roche Diagnostics) and 1 μL lactate dehydrogenase (5.5 units; Roche Diagnostics).
Preparation of Total RNA from Rice Seeds and Northern-Blot Analysis
The following stages in grain development were used in northern-blot analysis: I, maximum grain length (fresh weight of grain: Kinmaze, 4.3 mg; EM10, 5.2 mg); II, about two-thirds grain width (Kinmaze, 9.6 mg; EM10, 9.5 mg); III, maximum grain width (Kinmaze, 14.5 mg; EM10, 14.0 mg); IV, and maximum grain thickness (Kinmaze, 20.2 mg; EM10, 17.5 mg).
Total RNA from rice developing seeds was prepared as follows ( Chang et al., 1993 ). Rice seeds were ground with a mortar and pestle in liquid nitrogen, and incubated at 65°C for 10 min in 7 mL of 2× cetyltrimethylammonium bromide (2% [w/v] cetyltrimethylammonium bromide, 0.1 m Tris [pH 9.5], 20 m m EDTA, 1.4 m NaCl, and 1% [w/v] β-mercaptoethanol) with vigorous shaking. The mixture was extracted two times with 7 mL of chloroform. Adding 0.25 volumes of 10 m LiCl to the aqueous phase and storing on ice for 3 h, the total RNA was selectively precipitated. After centrifugation, the total RNA pellet was resuspended in 500 μL Tris-EDTA buffer (pH 8.0), and extracted with an equal volume of chloroform. The total RNA was precipitated by adding 0.1 volumes of 3 m Na-acetate (pH 5.2) and 2 volumes of ethanol to the aqueous phase, then chilled at −70°C for 30 min, and collected by centrifugation and dissolved in water.
Twenty micrograms of total RNA was electrophoresed on a denaturing 1.2% (w/v) agarose gel containing 0.66 m formaldehyde and then transferred to a NYTRAN nylon membrane (Schleicher and Schuell, Keene, NH). The membrane was hybridized with a digoxygenin-labeled RNA probe and washed as recommended by the manufacturer (Roche Diagnostics).
A digoxygenin-labeled RNA probe was prepared according to the manufacturer (Roche Diagnostics). DNA fragment for the preparation of BEI, BEIIa, BEIIb, SSI, and SSIIIa gene-specific probes were amplified by PCR. The DNA fragments were amplified from a cDNA (expressed sequence tags) of rice cv Nipponbare using pairs of one forward primer (f), including Eco RI site at the 5′ end, and one reverse primer (r), including Kpn I site at the 5′ end. Genes, primers used, and amplified DNA fragment size were in the following: BEI gene, primer sbe1-f 5′-TACGAATTCCCCGAGGGAATGCCAGGAGTA-3′ and primer sbe1-r 5′-TACGGTACCAACTATACATAAAGTTCATAT-3′(441 bp); BEIIa gene, sbe2a-f 5′-TACGAATTCACTTAC-AGAGGACTAATGATC-3′ and sbe2a-r 5′-TACGGTACC-CTGTCGAACAGATTATTCATA-3′ (359 bp); BEIIb gene, sbe2b-f 5′-TACGAATTCTCCAGCGGAATGAGAACA-CCA-3′and sbe2b-r 5′-TACGGTACCCAAGATGTACAGA-AGTGCAGA-3′ (331 bp); SSI gene, ss1-f 5′-TACGAATT-CTTCATGGATCAACCATATGTC-3′ and ss1-r 5′-TACG-GTACCGTCTTCACCTTAAGACTCAAC-3′ (457 bp); and SSIII gene, ss3-f 5′-TACGAATTCAAGACCGGGCTCG-AGTTCTAG-3′ and ss3-r 5′-TACGGTACCACCTT-CATT-TTACTTGCAATT-3′(529 bp). After digesting with Eco RI and Kpn I, the fragments were cloned into pBluescript II SK+. The resulting plasmid was used for the in vitro production of digoxygenin-labeled specific RNA probe with T7 RNA polymerase after linearization with Eco RI.
The authors thank Dr. Akiko Kubo and Ms. Kazuko Kimura (National Institute of Agrobiological Resources) for growing the plant materials, and also thank Dr. Naoko Fujita (Akita Prefectural University) for technical advice for measurement of SS activity.
Abel GJW Springer F Willmitzer L Kossmann J Cloning and functional analysis of a cDNA encoding a novel 139 kDa starch synthase from potato ( Solanum tuberosum L.). Plant J 10 1996 981 991
Google Scholar
Adkins GK Greenwood CT Hourston DJ Studies on starches of high amylose content: XI. Some physicochemical properties of dispersions of amylomaize starch, and observations on the nature of high-amylose starches. Cereal Chem 47 1970 13 18
Baba T Arai Y Structural characterization of amylopectin and intermediate material in amylomaize starch granules. Agric Biol Chem 48 1984 1763 1775
Baba T Kim S-J Arai Y Treatment of amylomaize starch granules with urea: comparison with normal maize starch. Carbohydr Res 124 1983 344 348
Banks W Greenwood CT Muir DD Studies on starches of high amylose content: part 17. A review of current concepts. Starch 26 1974 289 300
Bhattacharyya MK Smith AM Ellis THN Hedley C Martin C The wrinkled-seed character of pea described by Mendel is caused by a transposon-like insertion in a gene encoding starch-branching enzyme. Cell 60 1990 115 122
Bogracheva TY Davydova NI Genin YV Hedley CL Mutant genes at the r and rb loci affect the structure and physico-chemical properties of pea seed starches. J Exp Bot 46 1995 1905 1913
Borovsky D Smith EE Whelan WJ On the mechanism of amylose branching by potato Q-enzyme. Eur J Biochem 62 1976 307 312
Boyer CD Preiss J Multiple forms of (1→4)-α-D-glucan, (1→4)-α-D-glucan-6-glycosyl transferase from developing Zea mays L. kernels. Carbohydr Res 61 1978a 321 334
Boyer CD Preiss J Multiple forms of starch branching enzyme of maize: evidence for independent genetic control. Biochem Biophys Res Commun 80 1978b 169 175
Boyer CD Preiss J Evidence for independent genetic control of the multiple forms of maize endosperm branching enzymes and starch synthases. Plant Physiol 67 1981 1141 1145
Bradford MM A rapid and sensitive method for the quantitation of microgram quantities of protein utilizing the principle of protein-dye binding. Anal Biochem 72 1976 248 254
Burton RA Bewley JD Smith AM Bhattacharyya MK Tatge H Ring S Bull V Hamilton WDO Martin C Starch branching enzymes belonging to distinct enzyme families are differentially expressed during pea embryo development. Plant J 7 1995 3 15
Chang S Puryear J Cairney J A simple and efficient method for isolation RNA from pine trees. Plant Mol Biol Rep 11 1993 113 116
Cooke D Gidley MJ Loss of crystalline and molecular order during starch gelatinisation: origin of the enthalpic transition. Carbohydr Res 227 1992 103 112
Davis BJ Disc electrophoresis II: method and application of human serum proteins. Ann NY Acad Sci 121 1964 404 427
Fisher MB Boyer CD Immunological characterization of maize starch branching enzymes. Plant Physiol 72 1983 813 816
Fisher DK Gao M Kim K-N Boyer CD Guiltinan MJ Allelic analysis of the maize amylose-extender locus suggests that independent genes encode starch-branching enzymes IIa and IIb. Plant Physiol 110 1996 611 619
Gao M Fisher DK Kim K-N Shannon JC Guiltinan MJ Independent genetic control of maize starch-branching enzymes IIa and IIb: isolation and characterization of Sbe2a cDNA. Plant Physiol 114 1997 69 78
Gidley MJ Bulpin PV Crystallisation of malto-oligosaccharides as models of the crystalline forms of starch: minimum chain-length requirement for the formation of double helices. Carbohydr Res 161 1987 291 300
Gidley MJ Cooke D Darke AH Hoffmann RA Russell AL Greenwell P Molecular order and structure in enzyme-resistant retrograded starch. Carbohydr Polym 28 1995 23 31
Guan H Li P Imparl-Radosevich J Preiss J Keeling P Comparing the properties of Escherichia coli branching enzyme and maize branching enzyme. Arch Biochem Biophys 342 1997 92 98
Guan HP Preiss J Differentiation of the properties of the branching isozymes from maize ( Zea mays ). Plant Physiol 102 1993 1269 1273
Hanashiro I Abe J Hizukuri S A periodic distribution of the chain length of amylopectin as revealed by high-performance anion-exchange chromatography. Carbohydr Res 283 1996 151 159
Hilbert GE MacMasters MM Pea starch, a starch of high amylose content. J Biol Chem 162 1946 229 238
Hizukuri S Polymodal distribution of the chain lengths of amylopectins, and its significance. Carbohydr Res 147 1986 342 347
Jane J Chen YY Lee LF McPherson AE Wong KS Radosavljevic M Kasemsuwan T Effects of amylopectin branch chain length and amylose content on the gelatinization and pasting properties of starch. Cereal Chem 76 1999 629 637
Juliano BO A simplified assay for milled-rice amylose. Cereal Sci Today 16 1971 334 340
Kalichevsky MT Orford PD Ring SG The retrogradation and gelation of amylopectins from various botanical sources. Carbohydr Res 198 1990 49 55
Kasemsuwan T Jane J Schnable P Stinard P Robertson D Characterization of the dominant mutant Amylose-extender ( Ae1–5180 ) maize starch. Cereal Chem 72 1995 457 464
Laemmli UK Cleavage of structural proteins during the assembly of the head of bacteriophage T4. Nature 227 1970 680 685
Larsson C-T Hofvander P Khoshnoodi J Ek B Rask L Larsson H Three isoforms of starch synthase and two isoforms of branching enzyme are present in potato tuber starch. Plant Sci 117 1996 9 16
Larsson C-T Khoshnoodi J Ek B Rask L Larsson H Molecular cloning and characterization of starch-branching enzyme II from potato. Plant Mol Biol 37 1998 505 511
Lu S Chen L-N Lii C-Y Correlations between the fine structure, physicochemical properties, and retrogradation of amylopectins from Taiwan rice varieties. Cereal Chem 74 1997 34 39
Martin C Smith AM Starch biosynthesis. Plant Cell 7 1995 971 985
Mizuno K Kawasaki T Shimada H Satoh H Kobayashi E Okumura S Arai Y Baba T Alteration of the structural properties of starch components by the lack of an isoform of starch branching enzyme in rice seeds. J Biol Chem 268 1993 19084 19091
Mizuno K Kimura K Arai Y Kawasaki T Shimada H Baba T Starch branching enzymes from immature rice seeds. J Biochem 112 1992 643 651
Mizuno K Kobayashi E Tachibana M Kawasaki T Fujimura T Funae K Kobayashi M Baba T Characterization of an isoform of rice starch branching enzyme, RBE4, in developing seeds. Plant Cell Physiol 42 2001 349 357
Moates GK Noel TR Parker R Ring SG The effect of chain length and solvent interactions on the dissolution of the B-type crystalline polymorph of amylose in water. Carbohydr Res 298 1997 327 333
Morell MK Blennow A Kosar-Hashemi B Samuel MS Differential expression and properties of starch branching enzyme isoforms in developing wheat endosperm. Plant Physiol 113 1997 201 208
Nagamine T Komae K Improvement of a method for chain-length distribution analysis of wheat amylopectin. J Chromatogr 732 1996 255 259
Nakamura Y Takeichi T Kawaguchi K Yamanouchi H Purification of two forms of starch branching enzyme (Q-enzyme) from developing rice endosperm. Physiol Plant 84 1992 329 335
Nelson N A photometric adaptation of Somogyi method for the determination of glucose. J Biol Chem 153 1944 375 380
Peat S, Whelan WJ, Thomas GJ (1952) Evidence of multiple branching in waxy maize starch. J Chem Soc 4546–4548
Preiss J Levi C Starch biosynthesis and degradation. Carbohydrates: Structure and Function, The Biochemistry of Plants Preiss J 3 1980 371 423 Academic Press New York
Safford R Jobling SA Sidebottom CM Westcott RJ Cooke D Tober KJ Strongitharm BH Russell AL Gidley MJ Consequences of antisense RNA inhibition of starch branching enzyme activity on properties of potato starch. Carbohydr Polym 35 1998 155 168
Sanders EB Thompson DB Boyer CD Thermal behavior during gelatinization and amylopectin fine structure for selected maize genotypes as expressed in four inbred lines. Cereal Chem 67 1990 594 602
Satoh H Omura T Induction of mutation by the treatment of fertilized egg cell with N -methyl- N -nitrosourea in rice. J Fac Agr Kyushu Univ 24 1979 165 174
Shi Y-C Seib PA The structure of four waxy starches related to gelatinization and retrogradation. Carbohydr Res 227 1992 131 145
Somogyi M Notes on sugar determination. J Biol Chem 195 1952 19 23
Stinard PS Robertson DS Schnable PS Genetic isolation, cloning, and analysis of a Mutator -induced, dominant antimorph of the maize amylose extender1 locus. Plant Cell 5 1993 1555 1566
Sun C Sathish P Ahlandsberg S Deiber A Jansson C Identification of four starch-branching enzymes in barley endosperm: partial purification of forms I, IIa, and IIb. New Phytol 137 1997 215 222
Sun C Sathish P Ahlandsberg S Jansson C The two genes encoding starch-branching enzymes IIa and IIb are differentially expressed in barley. Plant Physiol 118 1998 37 49
Takeda Y Guan H-P Preiss J Branching of amylose by the branching isoenzymes of maize endosperm. Carbohydr Res 240 1993 253 263
Takeda Y Hizukuri S Juliano BO Structure of rice amylopectins with low and high affinity for iodine. Carbohydr Res 168 1987 79 88
Tester RF Morrison WR Swelling and gelatinization of cereal starches: II. Waxy rice starches. Cereal Chem 67 1990 558 563
Tomlinson KL Lloyd JR Smith AM Importance of isoforms of starch-branching enzyme in determining the structure of starch in pea leaves. Plant J 11 1997 31 43
Towbin H Staehelin T Gorden J Electrophoretic transfer of proteins from polyacrylamide gels to nitrocellulose sheets: procedure and some applications. Proc Natl Acad Sci USA 76 1979 4350 4354
Yamanouchi H Nakamura Y Organ specificity of isoforms of starch branching enzyme (Q-enzyme) in rice. Plant Cell Physiol 33 1992 985 991
Yano M Okuno K Kawakami J Satoh H Omura T High amylose mutants of rice, Oryza sativa L. Theor Appl Genet 69 1985 253 257
Yuan RC Thompson DB Boyer CD Fine structure of amylopectin in relation to gelatinization and retrogradation behavior of maize starches from three wx -containing genotypes in two inbred lines. Cereal Chem 70 1993 81 89
Author notes
Month: | Total Views: |
---|---|
February 2021 | 2 |
March 2021 | 15 |
April 2021 | 29 |
May 2021 | 21 |
June 2021 | 44 |
July 2021 | 47 |
August 2021 | 48 |
September 2021 | 39 |
October 2021 | 39 |
November 2021 | 27 |
December 2021 | 34 |
January 2022 | 34 |
February 2022 | 34 |
March 2022 | 46 |
April 2022 | 35 |
May 2022 | 19 |
June 2022 | 25 |
July 2022 | 16 |
August 2022 | 45 |
September 2022 | 32 |
October 2022 | 32 |
November 2022 | 36 |
December 2022 | 22 |
January 2023 | 21 |
February 2023 | 17 |
March 2023 | 26 |
April 2023 | 19 |
May 2023 | 20 |
June 2023 | 29 |
July 2023 | 30 |
August 2023 | 25 |
September 2023 | 30 |
October 2023 | 28 |
November 2023 | 20 |
December 2023 | 34 |
January 2024 | 48 |
February 2024 | 23 |
March 2024 | 41 |
April 2024 | 32 |
May 2024 | 33 |
June 2024 | 24 |
July 2024 | 53 |
August 2024 | 39 |
September 2024 | 15 |
Email alerts
Citing articles via.
- Recommend to Your Librarian
- Advertising & Corporate Services
- Awards & Funding
- Plant Science Today
- Plant Biology Meeting
- Meeting Management Services
- Plant Science Research Weekly
- Taproot: A Plantae Podcast
Affiliations
- Online ISSN 1532-2548
- Print ISSN 0032-0889
- Copyright © 2024 American Society of Plant Biologists
- About Oxford Academic
- Publish journals with us
- University press partners
- What we publish
- New features
- Open access
- Institutional account management
- Rights and permissions
- Get help with access
- Accessibility
- Advertising
- Media enquiries
- Oxford University Press
- Oxford Languages
- University of Oxford
Oxford University Press is a department of the University of Oxford. It furthers the University's objective of excellence in research, scholarship, and education by publishing worldwide
- Copyright © 2024 Oxford University Press
- Cookie settings
- Cookie policy
- Privacy policy
- Legal notice
This Feature Is Available To Subscribers Only
Sign In or Create an Account
This PDF is available to Subscribers Only
For full access to this pdf, sign in to an existing account, or purchase an annual subscription.
Advertisement
Understanding the biochemical, physiological, molecular, and synthetic biology approaches towards the development of C 4 rice ( Oryza sativa L.)
- Published: 25 January 2024
Cite this article
- Jyoti Prakash Sahoo ORCID: orcid.org/0000-0002-5009-491X 1 ,
- Debashis Mahapatra 2 ,
- Manaswini Mahapatra 1 ,
- Tuward J. Dweh 1 ,
- Salma Kayastha 1 ,
- Pranaya Pradhan 3 ,
- Swapan Kumar Tripathy 4 ,
- Kailash Chandra Samal 5 ,
- Abinash Mishra 6 ,
- Manasi Dash 6 &
- Spandan Nanda 7
196 Accesses
Explore all metrics
Crop yields must drastically increase to meet the demands of a rapidly increasing global population. One current option being investigated is the augmentation of photosynthetic capacity by incorporating the C 4 photosynthetic pathway into C 3 crops such as rice to significantly increase production. Crops with improved photosynthetic mechanisms would make better use of the solar radiation that can be converted into yield. This will help to boost grain yield, reduce water loss, and increase nitrogen use efficiency, particularly in hot and dry conditions. Identifying and manipulating the genes required to install C 4 photosynthesis in rice is a big challenge. However, the international C 4 rice collaboration, is formed to test the possibility of incorporating the C 4 mechanism into rice. This article summarizes the parameters that must be changed in rice in order for the C 4 pathway to be successfully introduced. It also examines the biochemistry, physiology, molecular biology, and synthetic biology approaches between the C 3 and C 4 photosynthetic processes.
This is a preview of subscription content, log in via an institution to check access.
Access this article
Subscribe and save.
- Get 10 units per month
- Download Article/Chapter or eBook
- 1 Unit = 1 Article or 1 Chapter
- Cancel anytime
Price includes VAT (Russian Federation)
Instant access to the full article PDF.
Rent this article via DeepDyve
Institutional subscriptions
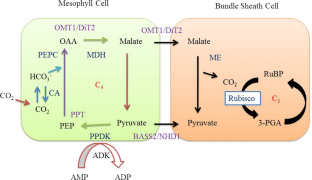
Similar content being viewed by others
Developing C4 Rice for Higher Photosynthetic Efficiency and Environmental Stress Tolerance
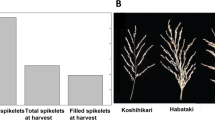
Photosynthesis and food security: the evolving story of C 4 rice
Pyramiding of three c 4 specific genes towards yield enhancement in rice, data availability.
Not applicable.
Akita S (1994) The eco-physiological aspect of raising the yield plateau of irrigated rice in the tropics. In: Cassman KG (ed) Breaking the yield barrier, 1993. International Rice Research Institute
Google Scholar
Andreou AI, Nakayama N (2018) Mobius assembly: a versatile golden-gate framework towards universal DNA assembly. PLoS ONE 13(1):e0189892
Article PubMed PubMed Central Google Scholar
Bandyopadhyay A, Datta K, Zhang J, Yang W, Raychaudhuri S, Miyao M, Datta SK (2007) Enhanced photosynthesis rate in genetically engineered indica rice expressing pepc gene cloned from maize. Plant Sci 172(6):1204–1209
Article CAS Google Scholar
Benhua J, Suqin Z, Demao J (2004) Photosynthetic C_ (4)-microcycle in transgenic rice plant lines expressing the maize C_ (4)-photosynthetic enzymes. Zuo Wu Xue Bao 30(6):536–543
Borba R, Reyna-Llorens I, Dickinson PJ, Steed G, Gouveia P, Gorska A, Hibberd JM (2023) Compartmentation of photosynthesis gene expression between mesophyll and bundle sheath cells of C 4 maize is dependent on time of day. Biorxiv. 2023–04
Broncano LS, Pukacz KR, Reichel-Deland V, Schlüter U, Triesch S, Weber AP (2023) Photorespiration is the solution, not the problem. J Plant Physiol 282:153928
Article Google Scholar
Brown NJ, Parsley K, Hibberd JM (2005) The future of C4 research–maize, flaveria or cleome? Trends Plant Sci 10(5):215–221
Article CAS PubMed Google Scholar
Bruckner M, Fischer G, Tramberend S, Giljum S (2015) Measuring telecouplings in the global land system: a review and comparative evaluation of land footprint accounting methods. Ecol Econ 114(C):11–21
Burgess AJ, Wang P (2023) Not all Calvin’s are equal: differential control of the Calvin cycle in C 3 versus C 4 plants. Plant Physiol 191(2):817–819. https://doi.org/10.1093/plphys/kiac531
Chen H, Lin Y, Zhang Q (2009) Review and prospect of transgenic rice research. Chin Sci Bull 54(22):4049–4068
Cui H (2021) Challenges and approaches to crop improvement through C 3 -to-C 4 engineering. Front Plant Sci 12:715391
Dai X, Tu X, Du B, Dong P, Sun S, Wang X, Li P (2022) Chromatin and regulatory differentiation between bundle sheath and mesophyll cells in maize. Plant J 109(3):675–692
Danila FR, Thakur V, Chatterjee J, Bala S, Coe RA, Acebron K, Quick WP (2021) Bundle sheath suberisation is required for C 4 photosynthesis in a Setaria viridis mutant. Commun Biol 4(1):254
Article CAS PubMed PubMed Central Google Scholar
Dash SSS, Lenka D, Sahoo JP, Tripathy SK, Samal KC, Lenka D, Panda RK (2022) Biochemical characterization of maize ( Zea mays L.) hybrids under excessive soil moisture stress. Cereal Res Commun. https://doi.org/10.1007/s42976-021-00241-2
DiMario RJ, Quebedeaux JC, Longstreth DJ, Dassanayake M, Hartman MM, Moroney JV (2016) The cytoplasmic carbonic anhydrases β CA2 and β CA4 are required for optimal plant growth at low CO 2 . Plant Physiol 171(1):280–293
Döring F, Streubel M, Bräutigam A, Gowik U (2016) Most photorespiratory genes are preferentially expressed in the bundle sheath cells of the C 4 grass Sorghum bicolor. J Exp Bot 67(10):3053–3064
Drincovich MF, Casati P, Andreo CS (2001) NADP-malic enzyme from plants: a ubiquitous enzyme involved in different metabolic pathways. FEBS Lett 490(1–2):1–6
Edwards GE, Walker D (1983) C 3 , C 4 : Mechanisms and cellular and environmental regulation of photosynthesis. University of California Press, Berkeley
Engler C, Youles M, Gruetzner R, Ehnert TM, Werner S, Jones JD, Marillonnet S (2014) A golden gate modular cloning toolbox for plants. ACS Synth Biol 3(11):839–843
Ermakova M, Danila FR, Furbank RT, Von Caemmerer S (2020) On the road to C 4 rice: advances and perspectives. Plant J 101(4):940–950
Ermakova M, Arrivault S, Giuliani R, Danila F, Alonso-Cantabrana H, Vlad D, Furbank RT (2021) Installation of C 4 photosynthetic pathway enzymes in rice using a single construct. Plant Biotechnol J 19(3):575–588
Feng Z, Mao Y, Xu N, Zhang B, Wei P, Yang DL, Zhu JK (2014) Multigeneration analysis reveals the inheritance, specificity, and patterns of CRISPR/Cas-induced gene modifications in Arabidopsis. Proc Natl Acad Sci 111(12):4632–4637
Fukayama H, Hatch MD, Tamai T, Tsuchida H, Sudoh S, Furbank RT, Miyao M (2003) Activity regulation and physiological impacts of maize C 4 -specific phospho enol pyruvate carboxylase overproduced in transgenic rice plants. Photosynth Res 77:227–239
Galmes J, Kapralov MV, Andralojc PJ, Conesa MA, Keys AJ, Parry MAJ, Flexas J (2014) Expanding knowledge of the RuBisCo kinetics variability in plant species: environmental and evolutionary trends. Plant Cell Environ 37(9):1989–2001
Gunn LH, Martin Avila E, Birch R, Whitney SM (2020) The dependency of red Rubisco on its cognate activase for enhancing plant photosynthesis and growth. Proc Natl Acad Sci 117(41):25890–25896
Guo S, Zhu M, Du J, Wang J, Lu X, Jin Y, Zhang Y (2023) Accurate phenotypic identification and genetic analysis of the ear leaf veins in maize ( Zea mays L.). Agronomy 13(3):753
Hasan R, Ohnuki Y, Kawasaki M, Taniguchi M, Miyake H (2005) Differential sensitivity of chloroplasts in mesophyll and bundle sheath cells in maize, an NADP-malic enzyme-type C 4 plant, to salinity stress. Plant Prod Sci 8(5):567–577
Häusler RE, Hirsch HJ, Kreuzaler F, Peterhänsel C (2002) Overexpression of C 4 -cycle enzymes in transgenic C 3 plants: a biotechnological approach to improve C 3 -photosynthesis. J Exp Bot 53(369):591–607
Article PubMed Google Scholar
Hibberd JM, Sheehy JE, Langdale JA (2008) Using C 4 photosynthesis to increase the yield of rice—rationale and feasibility. Curr Opin Plant Biol 11(2):228–231
Horton P, Murchie EH (2000) C 4 photosynthesis in rice: some lessons from studies of C 3 photosynthesis in field-grown rice. Studies in Plant Science, vol 7. Elsevier, pp 127–144
Horvath P, Barrangou R (2010) CRISPR/Cas, the immune system of bacteria and archaea. Science 327:167–170. https://doi.org/10.1126/science.1179555
Hossain M, Narciso J (2002) Global rice market: trends and perspectives. Bangladesh Develop Stud 28(4):1–28. https://www.jstor.org/stable/40795663
IRRI (2018) Rice of the future gets financial boost. Available at: https://www.irri.org/news-and-events/news/rice-future-gets-financial-boost . Accessed 20 June 2023
Ishikawa C, Hatanaka T, Misoo S, Miyake C, Fukayama H (2011) Functional incorporation of sorghum small subunit increases the catalytic turnover rate of RuBisCo in transgenic rice. Plant Physiol 156(3):1603–1611
Islam MA, Sarkar M, Rouf A, Rahman MC, Rahaman M (2021a) Assessing the level of comparative advantage for the boro rice production in Bangladesh: a time series analysis. J Bangladesh Agric Univ 19(3):361–370
Islam MS, Magid ASIA, Chen Y, Weng L, Ma J, Arafat MY, Li Y (2021b) Effect of calcium and iron-enriched biochar on arsenic and cadmium accumulation from soil to rice paddy tissues. Sci Total Environ 785:147163
Jiao DM, Li X, Ji BH (2005) Photoprotective effects of high level expression of C 4 phosphoenolpyruvate carboxylase in transgenic rice during photoinhibition. Photosynthetica 43(4):501–508
Kajala K, Covshoff S, Karki S, Woodfield H, Tolley BJ, Dionora MJA, Quick WP (2011) Strategies for engineering a two-celled C 4 photosynthetic pathway into rice. J Exp Bot 62(9):3001–3010
Karki S, Rizal G, Quick WP (2013) Improvement of photosynthesis in rice ( Oryza sativa L.) by inserting the C 4 pathway. Rice 6(1):1–8
Karpilov YS (1960) The distribution of radioactive carbon 14 amongst the products of photosynthesis of maize. Trudy Kazansk Sel’shokoz Inst 41:15–24
CAS Google Scholar
Kebeish R, Niessen M, Thiruveedhi K, Bari R, Hirsch HJ, Rosenkranz R, Stabler N, Schonfeld B, Kreuzaler F, Peterhansel C (2007) Chloroplastic photorespiratory bypass increases photosynthesis and biomass production in Arabidopsis thaliana . Nat Biotechnol 25(5):593–599
Khamari B, Hasmi SK, Samal KC, Sahoo JP, Senapati AK, Ranasingh N, Beura SK, Sala T (2022) Impact of microbial rivals and natural alterations on root decay and plant development in sesame. Indian Phytopathol. https://doi.org/10.1007/s42360-022-00552-2
Kinsman EA, Pyke KA (1998) Bundle sheath cells and cell-specific plastid development in Arabidopsis leaves. Development 125(10):1815–1822
Kortschak HP, Hartt CE, Burr GO (1965) Carbon dioxide fixation in sugarcane leaves. Plant Physiol 40(2):209–213
Kortschak HP, Hartt CE, Burr GO (1957) Abstracts of the annual meeting of the Hawaiian Academy of Science, p 21
Ku SB, Edwards GE (1978) Oxygen inhibition of photosynthesis. Temperature dependence of quantum yield and its relation to O 2 /CO 2 solubility ratio. Planta 140(1):1–6
Ku MS, Cho D, Li X, Jiao DM, Pinto M, Miyao M, Matsuoka M (2007) Introduction of genes encoding C4 photosynthesis enzymes into rice plants: physiological consequences. In: Novartis Foundation Symposium 236‐Rice Biotechnology: Improving Yield, Stress Tolerance and Grain Quality: Rice Biotechnology: Improving Yield, Stress Tolerance and Grain Quality: Novartis Foundation Symposium, Wiley, Chichester, vol 236, pp 100–116
Lee JH, Won HJ, Oh ES, Oh MH, Jung JH (2020) Golden gate cloning-compatible DNA replicon/2A-mediated polycistronic vectors for plants. Front Plant Sci 11:559365
Leegood RC (2008) Roles of the bundle sheath cells in leaves of C 3 plants. J Exp Bot 59(7):1663–1673
Leegood RC, Lea PJ, Adcock MD, Haeusler RD (1995) The regulation and control of photorespiration. J Exp Bot 46:1397–1414
Lin MT, Salihovic H, Clark FK, Hanson MR (2022) Improving the efficiency of Rubisco by resurrecting its ancestors in the family Solanaceae. Sci Adv 8(15):6871
Liu X, Li X, Zhang C, Dai C, Zhou J, Ren C, Zhang J (2017) Phosphoenolpyruvate carboxylase regulation in C 4 -PEPC-expressing transgenic rice during early responses to drought stress. Physiol Plant 159(2):178–200
Lowder LG, Zhang D, Baltes NJ, Paul JW 3rd, Tang X, Zheng X et al (2015) A CRISPR/Cas9 toolbox for multiplexed plant genome editing and transcriptional regulation. Plant Physiol 169:971–985. https://doi.org/10.1104/pp.15.00636
Ma X, Zhang Q, Zhu Q, Liu W, Chen Y, Qiu R et al (2015) A robust CRISPR/Cas9 system for convenient, high-efficiency multiplex genome editing in monocot and dicot plants. Mol Plant 8:1274–1284. https://doi.org/10.1016/j.molp.2015.04.007
Mallona I, Egea-Cortines M, Weiss J (2011) Conserved and divergent rhythms of crassulacean acid metabolism-related and core clock gene expression in the cactus Opuntia ficus-indica . Plant Physiol 156(4):1978–1989
Maroco JP, Ku MSB, Lea PJ, Dever LV, Leegood RC, Furbank RT, Edwards GE (1998) Oxygen requirement and inhibition of C 4 photosynthesis: an analysis of C 4 plants deficient in the C 3 and C 4 cycles. Plant Physiol 116(2):823–832
Matsuoka M, Kyozuka J, Shimamoto K, Kano-Murakami Y (1994) The promoters of two carboxylases in a C 4 plant (maize) direct cell-specific, light-regulated expression in a C 3 plant (rice). Plant J 6(3):311–319
Miyao M, Masumoto C, Miyazawa SI, Fukayama H (2011) Lessons from engineering a single-cell C 4 photosynthetic pathway into rice. J Exp Bot 62(9):3021–3029
Mubarak ANM, Burgess AJ, Pyke K, Quick WP, Murchie EH (2023) Mass screening of rice mutant populations at low CO 2 for identification of lowered photorespiration and respiration rates. Front Plant Sci 14:1125770
Nagalla SY, Samal KC, Behera L, Sahoo JP, Mishra D, Sahoo AR, Praveena J, Nargave CS (2022) Morpho-quantitative and biochemical characterization of Chia ( Salvia hispanica L.) seeds to understand its benefits and to increase its adaptability. Environ Conserv J. https://doi.org/10.36953/ECJ.10882265
Narawatthana S, Phansenee Y, Thammasamisorn BO, Vejchasarn P (2023) Multi-model genome-wide association studies of leaf anatomical traits and vein architecture in rice. Front Plant Sci 14:1107718
Nikolopoulos D, Liakopoulos G, Drossopoulos I, Karabourniotis G (2002) The relationship between anatomy and photosynthetic performance of heteromeric leaves. Plant Physiol 129(1):235–243
Nomura M, Higuchi T, Ishida Y, Ohta S, Komari T, Imaizumi N, Miyao-Tokutomi M, Matsuoka M, Tajima S (2005) Differential expression pattern of C 4 bundle sheath expression genes in rice, a C 3 plant. Plant Cell Physiol 46(5):754–761
O'Donnelly K (2015) Towards increasing the efficiency of Rubisco, through the use of carbonic anhydrase mimetics. Available at: http://hdl.handle.net/10044/1/34317 . Accessed 16 Dec 2023. https://doi.org/10.25560/34317
Ogren WL (1984) Photorespiration: pathways, regulation, and modification. Annu Rev Plant Physiol 35(1):415–442
Pathak H, Tripathi R, Jambhulkar NN, Bisen JP, Panda BB (2020) Eco-regional-based-rice farming for enhancing productivity, profitability, and sustainability, NRRI research bulletin no. 22
Peterhansel C (2011) Best practice procedures for the establishment of a C 4 cycle in transgenic C 3 plants. J Exp Bot 62(9):3011–3019
Peterson RB, Schultes NP, McHale NA, Zelitch I (2016) Evidence for a role for NAD (P) H dehydrogenase in concentration of CO 2 in the bundle sheath cell of Zea mays . Plant Physiol 171(1):125–138
Pradhan B, Panda D, Bishi SK, Chakraborty K, Muthusamy SK, Lenka SK (2022) Progress and prospects of C 4 trait engineering in plants. Plant Biol 24(6):920–931
Priyadarshini L, Samal KC, Sahoo JP, Mohapatra U (2020) Morphological, biochemical and molecular characterization of some promising potato ( Solanum tuberosum L) cultivars of Odisha. J Pharmacog Phytochem 9(5):1657–1664
Quick WP (2013) Engineering a C4 rice. In: Muralidharan K, Siddiq EA (eds) International dialogue on perception and prospects of designer rice. Society for Advancement of Rice Research, Directorate of Rice Research, Hyderabad, pp 329–335
Retta MA, Yin X, Ho QT, Watté R, Berghuijs HN, Verboven P, Nicolaï BM (2023) The role of chloroplast movement in C 4 photosynthesis: a theoretical analysis using a three-dimensional reaction–diffusion model for maize. J Exp Bot 74:erad138
Romanova AK (2005) Physiological and biochemical aspects and molecular mechanisms of plant adaptation to the elevated concentration of atmospheric CO 2 . Russ J Plant Physiol 52:112–126
Sage RF (2001) Environmental and evolutionary preconditions for the origin and diversification of the C 4 photosynthetic syndrome. Plant Biol 3(03):202–213
Sage RF (2004) The evolution of C 4 photosynthesis. New Phytol 161(2):341–370
Sage TL, Sage RF (2009) The functional anatomy of rice leaves: implications for refixation of photorespiratory CO 2 and efforts to engineer C 4 photosynthesis into rice. Plant Cell Physiol 50(4):756–772
Sage RF, Zhu XG (2011) Exploiting the engine of C 4 photosynthesis. J Exp Bot 62(9):2989–3000
Sahoo JP, Sharma V (2018) Impact of LOD score and recombination frequencies on the microsatellite marker based linkage map for drought tolerance in kharif rice of Assam. Int J Curr Microbiol App Sci 7(8):3299–3304
Sahoo JP, Singh SK, Saha D (2018) A review on linkage mapping for drought stress tolerance in rice. J Pharmacogn Phytochem 7(4):2149–2157
Sahoo JP, Sharma V, Verma RK, Chetia SK, Baruah AR, Modi MK, Yadav VK (2019) Linkage analysis for drought tolerance in kharif rice of Assam using microsatellite markers. Indian J Tradit Knowl 18(2):371–375
Sahoo JP, Behera L, Sharma SS, Praveena J, Nayak SK, Samal KC (2020a) Omics studies and systems biology perspective towards abiotic stress response in plants. Am J Plant Sci 11(12):2172
Sahoo JP, Mohapatra U, Mishra P (2020b) An outlook on metabolic pathway engineering in crop plants. Arch Agric Environ Sci 5(3):431–434
Sahoo JP, Mohapatra U, Mishra AP, Samal K (2020c) Turmeric (Haldi)-A strapping strategy for enhancing the immune system to reduce the effect of SARS-CoV-2. Food Sci Rep 1(8):10–12
Sahoo JP, Behera L, Praveena J, Sawant S, Mishra A, Sharma SS, Samal KC (2021a) The golden spice turmeric ( Curcuma longa ) and its feasible benefits in prospering human health—a review. Am J Plant Sci 12(3):455–475
Sahoo JP, Mishra AP, Samal KC, Dash AK (2021b) Insights into the antibiotic resistance in Biofilms—a review. Environ Conserv J 22(3):59–67
Sahoo JP, Mohapatra U, Saha D, Mohanty IC, Samal KC (2022a) Linkage disequilibrium mapping: a journey from traditional breeding to molecular breeding in crop plants. Indian J Tradit Knowl 21(2):434–442
Sahoo JP, Samal KC, Tripathy SK, Lenka D, Mishra P, Behera L, Behera B (2022b) Understanding the genetics of Cercospora leaf spot (CLS) resistance in mung bean ( Vigna radiata L. Wilczek). Trop Plant Pathol 47:1–15. https://doi.org/10.1007/s40858-022-00525-w
Samal KC, Sahoo JP, Behera L, Dash T (2021) Understanding the BLAST (Basic local alignment search tool) Program and a step-by-step guide for its use in life science research. Bhartiya Krishi Anusandhan Patrika 36(1):55–61
Samal KC, Ramakrishna B, Sahoo JP, Behera L (2022) Molecular characterization and validation of micro-satellite markers linked to powdery mildew disease resistance in mini core germplasm of urdbean [ Vigna mungo (L.) Hepper]. Res Biotica 4(2):62–68
Scarpella E, Marcos D, Friml J, Berleth T (2006) Control of leaf vascular patterning by polar auxin transport. Genes Dev 20(8):1015–1027
Schreiber L (2010) Transport barriers made of cutin, suberin and associated waxes. Trends Plant Sci 15(10):546–553
Serra O, Geldner N (2022) The making of suberin. New Phytol 235(3):848–866
Sharwood RE, Ghannoum O, Kapralov MV, Gunn LH, Whitney SM (2016a) Temperature responses of Rubisco from Paniceae grasses provide opportunities for improving C 3 photosynthesis. Nature Plants 2(12):1–9
Sharwood RE, Sonawane BV, Ghannoum O, Whitney SM (2016b) Improved analysis of C 4 and C 3 photosynthesis via refined in vitro assays of their carbon fixation biochemistry. J Exp Bot 67(10):3137–3148
Shu Y, Huang G, Zhang Q, Peng S, Li Y (2023) Reduction of photosynthesis under P deficiency is mainly caused by the decreased CO 2 diffusional capacities in wheat ( Triticum aestivum L.). Plant Physiol Biochem 198:107680
Shukla V, Barberon M (2021) Building and breaking of a barrier: suberin plasticity and function in the endodermis. Curr Opin Plant Biol 64:102153
Slewinski TL, Anderson AA, Zhang C, Turgeon R (2012) Scarecrow plays a role in establishing Kranz’s anatomy in maize leaves. Plant Cell Physiol 53(12):2030–2037
Sriraj P, Gurjar D (2022) Breeding effects in conversion of C 3 rice plant to C 4 rice plant. Pharma Innov 11(4):897–900
Suzuki S, Murai N, Kasaoka K, Hiyoshi T, Imaseki H, Burnell JN, Arai M (2006) Carbon metabolism in transgenic rice plants that express phosphoenolpyruvate carboxylase and/or phosphoenolpyruvate carboxykinase. Plant Sci 170(5):1010–1019
Takeuchi Y, Akagi H, Kamasawa N, Osumi M, Honda H (2000) Aberrant chloroplasts in transgenic rice plants expressing a high level of maize NADP-dependent malic enzyme. Planta 211(2):265–274
Tolley BJ, Sage TL, Langdale JA, Hibberd JM (2012) Individual maize chromosomes in the C 3 plant oat can increase bundle sheath cell size and vein density. Plant Physiol 159(4):1418–1427
Tsuchida H, Tamai T, Fukayama H, Agarie S, Nomura M, Onodera H, Miyao M (2001) High level expression of C4-specific NADP-malic enzyme in leaves and impairment of photoautotrophic growth in a C3 plant, rice. Plant Cell Physiol 42(2):138–145
Turkan I, Uzilday B, Dietz KJ, Bräutigam A, Ozgur R (2018) Reactive oxygen species and redox regulation in mesophyll and bundle sheath cells of C 4 plants. J Exp Bot 69(14):3321–3331
Ueno O (2001) Ultrastructural localization of photosynthetic and photorespiratory enzymes in epidermal, mesophyll, bundle sheath, and vascular bundle cells of the C 4 dicot Amaranthus viridis . J Exp Bot 52(358):1003–1013
Ueno O (2011) Structural and biochemical characterization of the C 3 –C 4 intermediate Brassica gravitate and relatives, with particular reference to the cellular distribution of RuBisCo. J Exp Bot 62(15):5347–5355
Van Lun M, Hub JS, Van DSD, Andersson I (2014) CO 2 and O 2 distribution in RuBisCo suggest the small subunit functions as a CO 2 reservoir. J Am Chem Soc 136(8):3165–3171
von Caemmerer S, Evans JR (2010) Enhancing C3 photosynthesis. Plant Physiol 154(2):589–592
Von Caemmerer S, Quick WP, Furbank RT (2012) The development of C 4 rice: current progress and future challenges. Science 336(6089):1671–1672
Wang P, Fouracre J, Kelly S, Karki S, Gowik U, Aubry S, Shaw MK, Westhoff P, Slamet-Loedin IH, Quick WP, Hibberd JM, Langdale JA (2013) Evolution of GOLDEN2-LIKE gene function in C 3 and C 4 plants. Planta 237(2):481–495
Wang S, Tholen D, Zhu XG (2017) C 4 photosynthesis in C 3 rice: a theoretical analysis of biochemical and anatomical factors. Plant Cell Environ 40(1):80–94
Weber APM, Von CS (2010) Plastid transport and metabolism of C 3 and C 4 plants: comparative analysis and possible biotechnological exploitation. Curr Opin Plant Biol 13(3):257–265
Xiao Y, Sloan J, Hepworth C, Fradera-Soler M, Mathers A, Thorley R, Zhu XG (2023) Defining the scope for altering rice leaf anatomy to improve photosynthesis: a modelling approach. New Phytol 237(2):441–453
Yeh SY, Lin HH, Chang YM, Chang YL, Chang CK, Huang YC, Ku MS (2022) Maize Golden2-like transcription factors boost rice chloroplast development, photosynthesis, and grain yield. Plant Physiol 188(1):442–459
Zhang BJ, Ling LL, Wang RF, Jiao DM (2009) Photosynthetic characteristics and effect of ATP in transgenic rice with phosphoenolpyruvate carboxylase and pyruvate orthophosphate dikinase genes. Photosynthetica 47(1):133–136
Zhang Y, Malzahn AA, Sretenovic S, Qi Y (2019) The emerging and uncultivated potential of CRISPR technology in plant science. Nature Plants 5(8):778–794
Zhou Y, Gunn LH, Birch R, Andersson I, Whitney SM (2023) Grafting Rhodobacter sphaeroides with red algae Rubisco to accelerate catalysis and plant growth. Nature Plants 9:978–986. https://doi.org/10.1038/s41477-023-01436-7
Zhu H, Li C, Gao C (2020) Applications of CRISPR–Cas in agriculture and plant biotechnology. Nat Rev Mol Cell Biol 21(11):661–677
Download references
Author information
Authors and affiliations.
Faculty of Agriculture and Allied Sciences, C.V. Raman Global University, Bhubaneswar, Odisha, 752054, India
Jyoti Prakash Sahoo, Manaswini Mahapatra, Tuward J. Dweh & Salma Kayastha
Department of Plant Physiology, College of Agriculture, Odisha University of Agriculture & Technology, Bhubaneswar, 751003, India
Debashis Mahapatra
NICRA Project, Krishi Vigyan Kendra, Odisha University of Agriculture and Technology, Puri, 752014, India
Pranaya Pradhan
College of Agriculture, Odisha University of Agriculture & Technology, Bhawanipatna, 766001, India
Swapan Kumar Tripathy
College of Horticulture, Odisha University of Agriculture & Technology, Chiplima, 768025, India
Kailash Chandra Samal
Department of Genetics and Plant Breeding, College of Agriculture, Odisha University of Agriculture & Technology, Bhubaneswar, 751003, India
Abinash Mishra & Manasi Dash
Department of Chemistry, College of Basic Science and Humanities, Odisha University of Agriculture & Technology, Bhubaneswar, 751003, India
Spandan Nanda
You can also search for this author in PubMed Google Scholar
Contributions
JPS was involved in conceptualization, data curation, formal analysis, investigation, methodology, resources, software, visualization, and writing—original draft; DM, MM, TJD, SK, PP, AM, and SN contributed to writing—reviewing and editing; JPS, SKT, KCS, and MD were responsible for project administration, supervision, and validation. All authors read and approved the final manuscript.
Corresponding author
Correspondence to Jyoti Prakash Sahoo .
Ethics declarations
Conflict of interest.
The authors declare no competing interests.
Ethical approval
Additional information.
Communicated by Tibor Janda.
Rights and permissions
Springer Nature or its licensor (e.g. a society or other partner) holds exclusive rights to this article under a publishing agreement with the author(s) or other rightsholder(s); author self-archiving of the accepted manuscript version of this article is solely governed by the terms of such publishing agreement and applicable law.
Reprints and permissions
About this article
Sahoo, J.P., Mahapatra, D., Mahapatra, M. et al. Understanding the biochemical, physiological, molecular, and synthetic biology approaches towards the development of C 4 rice ( Oryza sativa L.). CEREAL RESEARCH COMMUNICATIONS (2024). https://doi.org/10.1007/s42976-024-00489-4
Download citation
Received : 25 October 2023
Accepted : 02 January 2024
Published : 25 January 2024
DOI : https://doi.org/10.1007/s42976-024-00489-4
Share this article
Anyone you share the following link with will be able to read this content:
Sorry, a shareable link is not currently available for this article.
Provided by the Springer Nature SharedIt content-sharing initiative
- Bundle sheath cell
- Chloroplast
- Mesophyll cell
- Photorespiration
- Photosynthesis
- Find a journal
- Publish with us
- Track your research
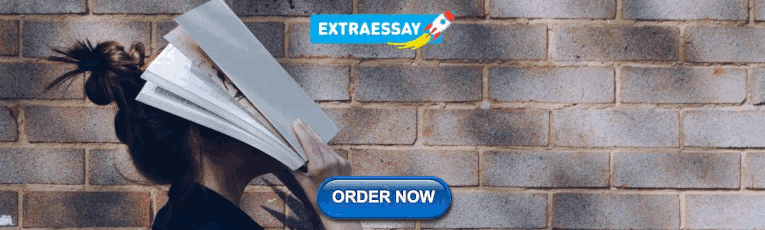
IMAGES
VIDEO
COMMENTS
Discover a faster, simpler path to publishing in a high-quality journal. PLOS ONE promises fair, rigorous peer review, broad scope, and wide readership - a perfect fit for your research every time.. Learn More Submit Now
Introduction Analysis of blood for the evaluation of clinically relevant biomarkers requires precise collection and sample handling by phlebotomists and laboratory staff. An important consideration for the clinical application of metabolomics are the different anticoagulants utilized for sample collection. Most studies that have characterized differences in metabolite levels in various blood ...
Explore the latest full-text research PDFs, articles, conference papers, preprints and more on BIOCHEMICAL ANALYSIS. Find methods information, sources, references or conduct a literature review on ...
This paper focuses on one of the most commonly encountered materials in our society, namely paper. Paper is an inherently complex material, yet its use provides for chemical analysis approaches that are elegant in their simplicity of execution. In the first half of the previous century, paper in scientific research was used mainly for filtration and chromatographic separation. While its use ...
The journal's title declares its broad scope: methods for the basic biological sciences that include biochemistry, molecular genetics, cell biology, proteomics, immunology, bioinformatics and wherever the frontiers of research take the field. The emphasis is on methods from the strictly analytical to the more preparative that would include ...
Analytical biochemistry articles from across Nature Portfolio. Analytical biochemistry is the study of biochemical components found in a cell or other biological sample. This scientific discipline ...
Biochemistry is the study of the structure and function of biological molecules such as proteins, nucleic acids, carbohydrates and lipids. Biochemistry is also used to describe techniques suited ...
The current volume is another in this series which is designed to try to meet the need in the field of biochemical analysis. The topics to be included are chemical, physical, microbiological, and if necessary, animal assays, as well as basic techniques and instrumentation for the determination of enzymes, vitamins, hormones, lipids ...
A Feature Paper should be a substantial original Article that involves several techniques or approaches, provides an outlook for future research directions and describes possible research applications. Feature papers are submitted upon individual invitation or recommendation by the scientific editors and must receive positive feedback from the ...
About this book. Biochemical analysis is a rapidly expanding field and is a key component of modern drug discovery and research. Methods of Biochemical Analysis provides a periodic and authoritative review of the latest achievements in biochemical analysis. Founded in 1954 by Professor David Glick, Methods of Biochemical Analysis provides a ...
The FASP method utilizes a molecular weight cutoff (MWCO) membrane to filter out digested peptides above a certain molecular weight for downstream analysis and allows for all sample preparation steps in a proteomic analysis to be carried out in a single 96-well microplate [153, 154, 155]. Building off of the microplate-based diagnostic assay ...
The data generated by estimating these biochemical parameters by the semiauto analyzer and the fully automatic analyzer method were registered for statistical analysis. Statistical analysis Data were entered in Microsoft Excel 2013 and were analyzed using Statistical Package for the Social Sciences (SPSS SPSS by IBM) software program, version 20.0.
Description. Techniques for Biochemical Analysis provides researchers with a practical guide for investigating a variety of different biomolecules. It includes a range of tried and tested protocols, outlining the principles upon which each technique is founded, as well as providing instructions on equipment setup and use, buffer preparation ...
International Journal of Research Publications. Volume-54, Issue-1,June 2020. ISSN number 2708-3578 (Online) Accepted and Published Manuscript. A Mini-Review on Commonly used Biochemical Tests for ...
With certain methods of analysis, for example, flame emission spectrophotometry and use of an indirect ion-selective electrode, where dilution of the specimen in a large volume of solution is required, pseudohyponatraemia may occur in patients with severe triglyceridaemia or paraproteinaemia and very rarely may arise secondary to severe ...
The molecular microbiology methods have revolutionized the bacterial identification process. It is not only faster and accurate but also precise and broadly used. The current chapter describes several biochemical and molecular methods for bacterial identification. Various methods such as Gram's staining, coagulase test, catalase test, oxidase ...
Biochemistry. Biochemical analysis is usually conducted in frozen muscle tissue or in cultured fibroblasts. A detailed, step-by-step procedure for the measurement of the activities of complexes I-IV has been published286 and should facilitate comparison of data among laboratories, thus far a thorny issue.
Adolescence is the most critical phase of human growth that radically alters physiology of the body and wherein any inconsistency can lead to serious health consequences in adulthood. The timing and pace at which various developmental events occur during adolescence is highly diverse and thus results in a drastic change in blood biochemistry. Monitoring the physiological levels of various ...
Abstract. Biochemical analysis of amylose-extender(ae) mutant of rice (Oryza sativa) revealed that the mutation in the gene for starch-branching enzyme IIb (BEIIb) specifically altered the structure of amylopectin in the endosperm by reducing short chains with degree of polymerization of 17 or less, with the greatest decrease in chains with degree of polymerization of 8 to 12.
However, in a study, theoretical analysis of biochemical and anatomical factors is performed for C 4 photosynthesis in C 3 rice (Wang et al. 2017). However, researchers have identified potential ancestors of C 3 Rubiscos with superior kinetics, showcasing promising attributes for aiding plants in adapting to climate change (Lin et al. 2022).
is used in the experimental analysis. 1.2. Biochemical analysis of millets Total nitrogen content was determined by the standard Kjeldahl method followed by digestion, distillation than titration procedure then protein percent is calculated by standard 2. Fat content of millet was determined
In this study, we assessed the biochemical constituents such as soluble protein, proline, nitrate reductase and catalase activity at five different growth stages (vegetative, flowering, grain development, grain maturity and harvest) of barnyard millet and foxtail millet. The proline accumulation was highest at grain developme nt stage in all ...
Abstract and Figures. The present study was aimed to evaluate biochemical and phytotochemical of dry leaves of Stevia rebaudiana. Biochemical analysis indicated that Stevia leaves are a good ...