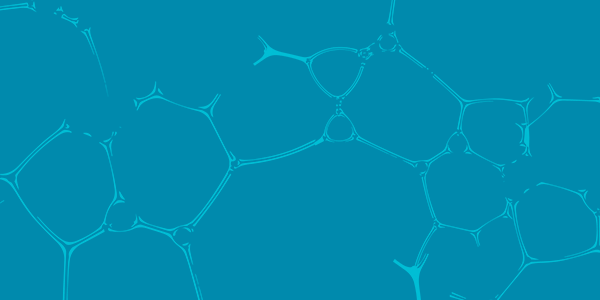
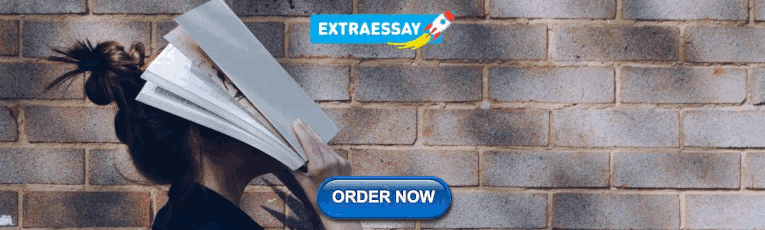
MRC Muscle Scale - MRC
The MRC scale for muscle power was first published in 1943 in a document called ‘Aids to the Investigation of Peripheral Nerve Injuries (War Memorandum No. 7)’. This became a standard text resource which was reprinted many times, and is referred to widely in a number of documents and papers. In the 1970s the document was republished with the title ‘Aids to the Examination of the Peripheral Nervous System (Memorandum No. 45)’.
The muscle scale grades muscle power on a scale of 0 to 5 in relation to the maximum expected for that muscle. In a recent comparison to an analogue scale the MRC scale is more reliable and accurate for clinical assessment in weak muscles (grades 0-3) while an analogue scale is more reliable and accurate for the assessment of stronger muscles (grades 4 and 5).
Permission to reuse the MRC Muscle Scale
The MRC Muscle Scale is licensed under the Open Government Licence .
© Crown Copyright
Aids to the Examination of the Peripheral Nervous System (Memorandum No. 45) is licensed under the Open Government Licence 3.0.
You must give appropriate credit (“Used with the permission of the Medical Research Council”) and indicate if changes were made. You may do so in any reasonable manner, but not in any way that suggests that the MRC endorses you or your use.
Aids to the examination of the peripheral nervous system – MRC Memorandum No.45 (superseding War Memorandum No.7)
Contact information
Ask a question, or get further information about any of the MRC scales. Email: [email protected]
For information about licensing
To view the full Open Government Licence, visit National Archives: Open Government Licence Version two .
Further context, best practice and guidance can be found in the National Archives: UK Government Licensing Framework .
LifeArc manages MRC’s intellectual property rights and commercialises findings by licensing them to industry. They can be contacted for support via the contact information on their website .
Last updated: 16 March 2023
This is the website for UKRI: our seven research councils, Research England and Innovate UK. Let us know if you have feedback or would like to help improve our online products and services .

MedicalCRITERIA.com
Unifying concepts, medical research council (mrc) scale for muscle strength.
The muscle scale grades muscle power on a scale of 0 to 5 in relation to the maximum expected for that muscle.
- Grade 5: Muscle contracts normally against full resistance.
- Grade 4: Muscle strength is reduced but muscle contraction can still move joint against resistance.
- Grade 3: Muscle strength is further reduced such that the joint can be moved only against gravity with the examiner’s resistance completely removed. As an example, the elbow can be moved from full extension to full flexion starting with the arm hanging down at the side.
- Grade 2: Muscle can move only if the resistance of gravity is removed. As an example, the elbow can be fully flexed only if the arm is maintained in a horizontal plane.
- Grade 1: Only a trace or flicker of movement is seen or felt in the muscle or fasciculations are observed in the muscle.
- Grade 0: No movement is observed.
- Grade 0: normal.
- Grade 1: no disability; minor sensory signs or areflexia.
- Grade 2: mild disability; ambulatory for >200 m; mild weakness in one or more limbs and sensory impairment.
- Grade 3: moderate disability; ambulatory for >50 m without stick; moderate weakness MRC Grade 4 and sensory impairment.
- Grade 4: severe disability; able to walk >10 m with support of stick; motor weakness MRC Grade 4 and sensory impairment.
- Grade 5: requires support to walk 5 m; marked motor and sensory signs.
- Grade 6: cannot walk 5 m, able to stand unsupported and able to transfer to wheelchair, able to feed independently.
- Grade 7: bedridden, severe quadriparesis; maximum strength MRC grade 3.
- Grade 8: respirator and/or severe quadriparesis; maximum strength MRC grade 2.
- Grade 9: respirator and quadriplegia.
- Grade 10: dead.
Modified Medical Research Council (mMRC) Dyspnea Scale
References:
- Medical Research Council. Aids to the examination of the peripheral nervous system, Memorandum no. 45, Her Majesty’s Stationery Office, London, 1981.
- Hahn AF, Bolton CF, Pillay N, et al. Plasma exchange therapy in chronic inflammatory demyelinating polyneuropathy. A double-blind, sham controlled, cross-over study. Brain 1996;119:1055–66. [Medline]
- Paternostro-Sluga T, Grim-Stieger M, Posch M, Schuhfried O, Vacariu G, Mittermaier C, Bittner C, Fialka-Moser V. Reliability and validity of the Medical Research Council (MRC) scale and a modified scale for testing muscle strength in patients with radial palsy. J Rehabil Med. 2008 Aug;40(8):665-71. [Medline]
Created: Mar 23, 2009.
Related Posts
The numeric rating scale (NRS) is a single 11-point numeric scale broadly validated across myriad patient…
The Hachinski ischemic score (HIS) is known to be a simple clinical tool, currently used…
Instructions: This scale is intended to record your own assessment of any sleep difficulty you…
Preoperative anxiety is a frequent and challenging problem with deleterious effects on the development of…
The Karnofsky Performance Status Scale (KPS) was designed to measure the level of patient activity…

Users Online
Medical disclaimer, recent posts.
- Diagnostic Criteria of Lipedema
- Diagnostic Criteria of Acute Adrenal Insufficiency
- Diagnostic Criteria for Peripartum Cardiomyopathy
- Diagnostic Criteria for Cannabis Use Disorder
- Psoriasis Area Severity Index (PASI) Score
- About Us (1)
- Allergy (3)
- Anesthesiology (5)
- Cardiology (55)
- Critical Care (17)
- Dermatology (7)
- Diabetes (14)
- Endocrinology and Metabolism (21)
- Epidemiology (6)
- Family Practice (7)
- Gastroenterology (63)
- Hematology (40)
- Immumology (6)
- Infectious Disease (44)
- Internal Medicine (1)
- Nephrology (17)
- Neurology (79)
- Nutrition (30)
- Obstetrics & Gynecology (21)
- Oncology (13)
- Ophthalmology (4)
- Orthopedic (5)
- Otolaryngology (5)
- Pathologic (1)
- Pediatrics (14)
- Pharmacology (3)
- Physical Therapists (7)
- Psychiatry (51)
- Pulmonary (23)
- Radiology (5)
- Rheumatology (52)
- Surgery (8)
- Urology (5)
Copyright by MedicalCriteria.com
Last Updated on 27 July, 2021 by Guillermo Firman

Muscle Power Assessment (MRC Scale)
- 📖 Geeky Medics OSCE Book
- ⚡ Geeky Medics Bundles
- ✨ 1300+ OSCE Stations
- ✅ OSCE Checklist PDF Booklet
- 🧠 UKMLA AKT Question Bank
- 💊 PSA Question Bank
- 💉 Clinical Skills App
- 🗂️ Flashcard Collections | OSCE , Medicine , Surgery , Anatomy
- 💬 SCA Cases for MRCGP
To be the first to know about our latest videos subscribe to our YouTube channel 🙌
Suggest an improvement
- Hidden Post Title
- Hidden Post URL
- Hidden Post ID
- Type of issue * N/A Fix spelling/grammar issue Add or fix a link Add or fix an image Add more detail Improve the quality of the writing Fix a factual error
- Please provide as much detail as possible * You don't need to tell us which article this feedback relates to, as we automatically capture that information for you.
- Your Email (optional) This allows us to get in touch for more details if required.
- Which organ is responsible for pumping blood around the body? * Enter a five letter word in lowercase
- Phone This field is for validation purposes and should be left unchanged.
The assessment of muscle power is a key part of a neurological examination of the upper or lower limbs. As a result, it is important to familiarise yourself with the Medical Research Council’s scale (MRC scale) of muscle power. The MRC scale of muscle strength uses a score of 0 to 5 to grade the power of a particular muscle group in relation to the movement of a single joint.
We have included the MRC scale below in several formats, to make it as accessible as possible:
- MRC Muscle Power Scale (PDF)
- MRC Muscle Power Scale (Image)
MRC Muscle Power Scale
0 | No contraction |
1 | Flicker or trace of contraction |
2 | Active movement, with gravity eliminated |
3 | Active movement against gravity |
4 | Active movement against gravity and resistance |
5 | Normal power |
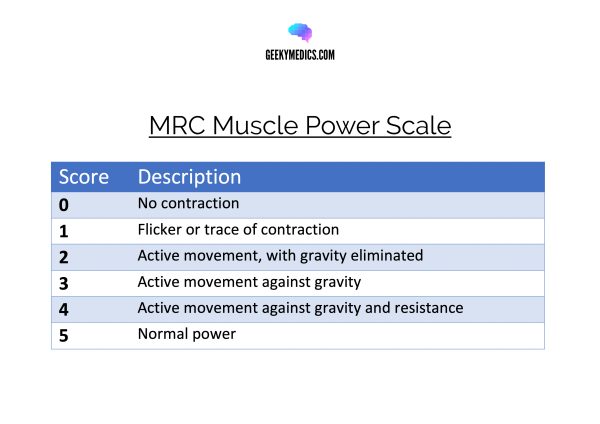
- Used with the permission of the Medical Research Council. Open Government Licence.
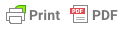
Other pages
- Product Bundles 🎉
- Join the Team 🙌
- Institutional Licence 📚
- OSCE Station Creator Tool 🩺
- Create and Share Flashcards 🗂️
- OSCE Group Chat 💬
- Newsletter 📰
- Advertise With Us
Join the community
134k followers
13k followers
290k followers
839k followers
MRC Scale | Muscle Strength Grading | Strength Testing
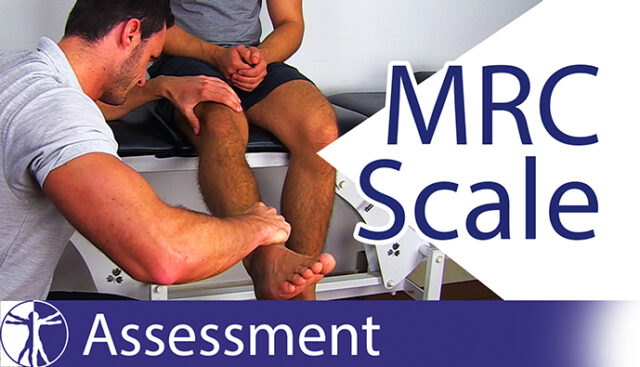
- Assessment E-Book
MRC stands for Medical Research Council which is the institution that sets up the standard for muscle strength testing.
The strength can be categorized on a level from zero to five. In our example, we will use the extension of the knee joint. So, the muscle which we are going to test is the Quadriceps.
The levels are as follows:
- Grade 0: The patient cannot activate the muscle, so no movement is observed. For grade 0, ask the patient to contract his quadriceps. He can do this by pushing the back of his knee into the bench. For grade 0, I will not see or feel a flicker or trace of contraction or movement.
- Grade 1: the patient can activate the muscle, without moving the limb. So only a trace or flicker of movement is seen or felt during palpation of the muscle. For grade 1, ask the patient to do the exact same thing, and this time, you will see or feel a muscle flicker or trace of movement.
- Grade 2: movement over the full range of motion can only occur if gravity is eliminated. In order to distinguish between grades 1 and 2, we have to bring our patient in side-lying position to eliminate gravity. Then, I will support the leg of my patient, bring it into full flexion and ask my patient to move into extension. If my patient is able to move through the full range of motion, this is a grade 2. If no movement is possible at all, we are talking about grade 1.
- Grade 3: the patient can overcome gravity and move through the full range of motion without resistance coming from the examiner. For grade 3, I’ll ask my patient to extend his knee against gravity.
- Grade 4: weakness with resistance. So your patient can move through the full range of motion with moderate resistance coming from the examiner. For grade 4, I will give moderate resistance against the extension of my patient’s knee.
- Grade 5: full strength. So your patient can move through the whole range of motion against full resistance coming from the examiner.And for grade 5, give full resistance against the extension of the patient’s knee. In order to distinguish between a grade 4 and 5, make sure to compare both legs.
Now that you’ve seen the basics of how to test according to the MRC scale, make sure to practice this with different joints and muscles and figure out a way of how to position your patient.
21 OF THE MOST USEFUL ORTHOPAEDIC TESTS IN CLINICAL PRACTICE
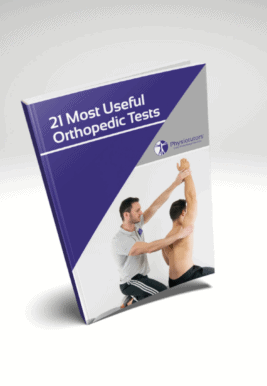
BUY THE FULL PHYSIOTUTORS ASSESSMENT BOOK
- 600+ Pages e-Book
- Interactive Content (Direct Video Demonstration, PubMed articles)
- Statistical Values for all Special Tests from the latest research
- Clinical Value Recommendation
- Detailed descriptions & searchable
- Currently on Version 6.0 – Free lifetime updates
- Available in 🇬🇧 🇩🇪 🇫🇷 🇪🇸 🇮🇹 🇵🇹 🇹🇷
- And much more!
ALL ORTHOPEDIC TESTS IN ONE PLACE
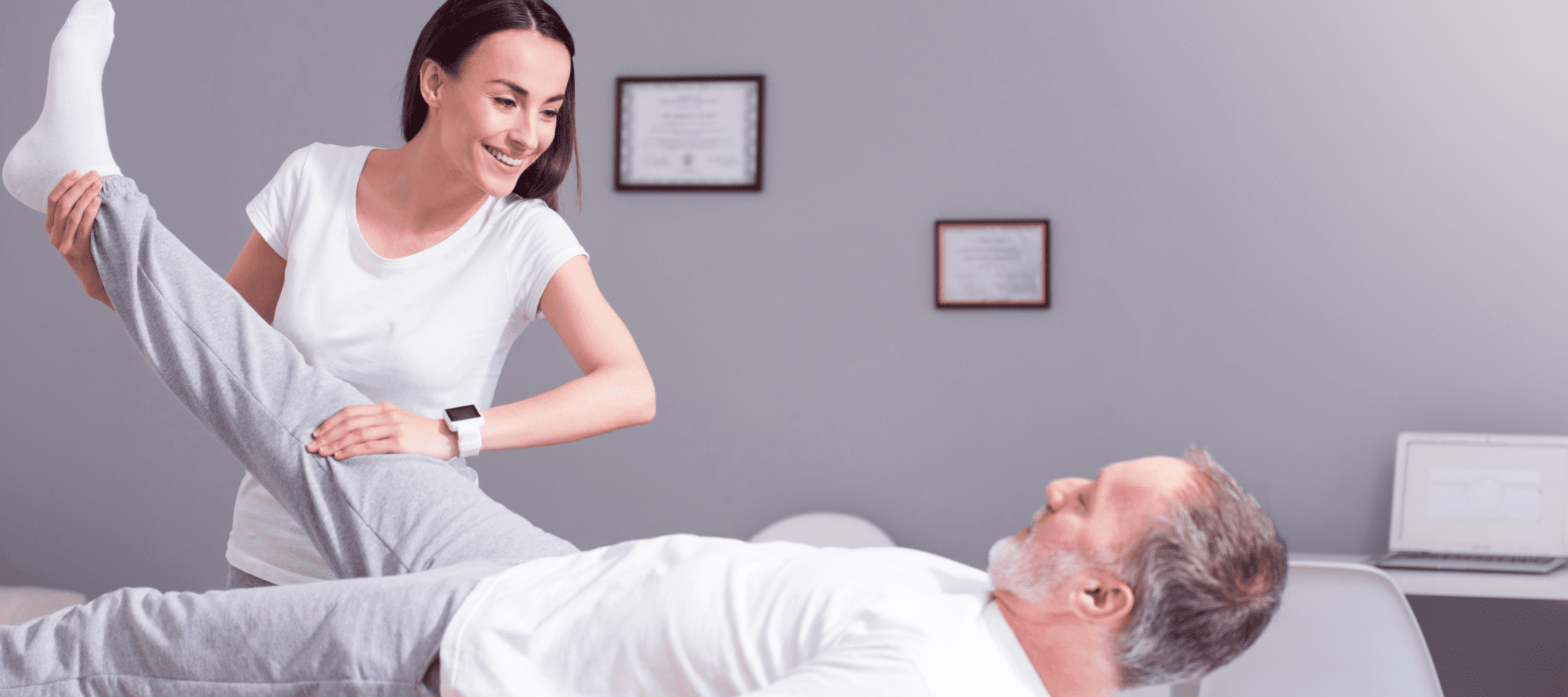
What customers have to say about the Assessment E-Book

Create your free account to gain access to this exclusive content and more!
To provide the best experiences, we and our partners use technologies like cookies to store and/or access device information. Consenting to these technologies will allow us and our partners to process personal data such as browsing behavior or unique IDs on this site and show (non-) personalized ads. Not consenting or withdrawing consent, may adversely affect certain features and functions.
Click below to consent to the above or make granular choices. Your choices will be applied to this site only. You can change your settings at any time, including withdrawing your consent, by using the toggles on the Cookie Policy, or by clicking on the manage consent button at the bottom of the screen.
Download our free physiotherapy app with all the knowledge you need.
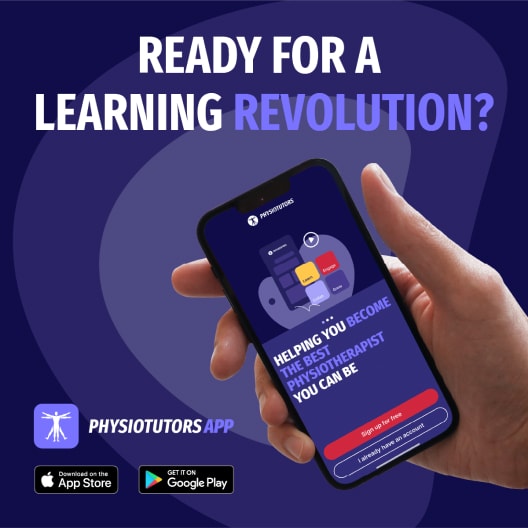
Europe PMC requires Javascript to function effectively.
Either your web browser doesn't support Javascript or it is currently turned off. In the latter case, please turn on Javascript support in your web browser and reload this page.

An official website of the United States government
The .gov means it’s official. Federal government websites often end in .gov or .mil. Before sharing sensitive information, make sure you’re on a federal government site.
The site is secure. The https:// ensures that you are connecting to the official website and that any information you provide is encrypted and transmitted securely.
- Publications
- Account settings
- My Bibliography
- Collections
- Citation manager
Save citation to file
Email citation, add to collections.
- Create a new collection
- Add to an existing collection
Add to My Bibliography
Your saved search, create a file for external citation management software, your rss feed.
- Search in PubMed
- Search in NLM Catalog
- Add to Search
Reliability and validity of the Medical Research Council (MRC) scale and a modified scale for testing muscle strength in patients with radial palsy
Affiliation.
- 1 Department of Physical Medicine and Rehabilitation, Medical University of Vienna, Austria. [email protected]
- PMID: 19020701
- DOI: 10.2340/16501977-0235
Objective: To assess the inter-rater and intra-rater reliability and validity of the original and a modified Medical Research Council scale for testing muscle strength in radial palsy.
Design: Prospective, randomized validation study.
Patients: Thirty-one patients with peripheral paresis of radial innervated forearm muscles were included.
Methods: Wrist extension, finger extension and grip strength were evaluated by manual muscle testing. Dynamometric measurement of grip strength was performed. Pair-wise weighted kappa coefficients were calculated to determine inter-rater and intra-rater reliability. The 2 scores were compared using the signed-rank test. Spearman's correlation coefficients of the maximal relative force measurements with the median (over-raters) Medical Research Council and modified Medical Research Council scores were calculated to determine validity.
Results: Inter-rater agreement of the Medical Research Council scale (finger extension: 0.77; wrist extension: 0.78; grip strength: 0.78) and the modified Medical Research Council scale (finger extension: 0.81; wrist extension: 0.78; grip strength: 0.81) as well as intra-rater agreement of the Medical Research Council scale (finger extension: 0.86; wrist extension: 0.82; grip strength: 0.84) and the modified Medical Research Council scale (finger extension: 0.84, wrist extension: 0.81; grip strength: 0.88) showed almost perfect agreement. Spearman's correlation coefficients of the maximal relative force measurements with the median Medical Research Council and modified Medical Research Council score were both 0.78.
Conclusion: Medical Research Council and modified Medical Research Council scales are measurements with substantial inter-rater and intra-rater reliability in evaluating forearm muscles.
PubMed Disclaimer
Similar articles
- Inter- and intra-rater reliability of the Modified Ashworth Scale: a systematic review and meta-analysis. Meseguer-Henarejos AB, Sánchez-Meca J, López-Pina JA, Carles-Hernández R. Meseguer-Henarejos AB, et al. Eur J Phys Rehabil Med. 2018 Aug;54(4):576-590. doi: 10.23736/S1973-9087.17.04796-7. Epub 2017 Sep 13. Eur J Phys Rehabil Med. 2018. PMID: 28901119 Review.
- A new two-tier strength assessment approach to the diagnosis of weakness in intensive care: an observational study. Parry SM, Berney S, Granger CL, Dunlop DL, Murphy L, El-Ansary D, Koopman R, Denehy L. Parry SM, et al. Crit Care. 2015 Feb 26;19(1):52. doi: 10.1186/s13054-015-0780-5. Crit Care. 2015. PMID: 25882719 Free PMC article.
- Validity and reliability of the aneroid sphygmomanometer using a paediatric size cuff for craniocervical flexion test. Mahashabde R, Fernandez R, Sabnis S. Mahashabde R, et al. Int J Evid Based Healthc. 2013 Dec;11(4):285-90. doi: 10.1111/1744-1609.12048. Int J Evid Based Healthc. 2013. PMID: 24298922
- Assessment of limb muscle strength in critically ill patients: a systematic review. Vanpee G, Hermans G, Segers J, Gosselink R. Vanpee G, et al. Crit Care Med. 2014 Mar;42(3):701-11. doi: 10.1097/CCM.0000000000000030. Crit Care Med. 2014. PMID: 24201180 Review.
- Validation of an objective device for assessing circumductive wrist motion. Franko OI, Lal S, Pauyo T, Alexander M, Zurakowski D, Day C. Franko OI, et al. J Hand Surg Am. 2008 Oct;33(8):1293-300. doi: 10.1016/j.jhsa.2008.03.012. J Hand Surg Am. 2008. PMID: 18929191
- Deep Learning Model Coupling Wearable Bioelectric and Mechanical Sensors for Refined Muscle Strength Assessment. Li C, Wang T, Zhou S, Sun Y, Xu Z, Xu S, Shu S, Zhao Y, Jiang B, Xie S, Sun Z, Xu X, Li W, Chen B, Tang W. Li C, et al. Research (Wash D C). 2024 May 23;7:0366. doi: 10.34133/research.0366. eCollection 2024. Research (Wash D C). 2024. PMID: 38783913 Free PMC article.
- The independence of impairments in proprioception and visuomotor adaptation after stroke. Moore RT, Piitz MA, Singh N, Dukelow SP, Cluff T. Moore RT, et al. J Neuroeng Rehabil. 2024 May 18;21(1):81. doi: 10.1186/s12984-024-01360-7. J Neuroeng Rehabil. 2024. PMID: 38762552 Free PMC article.
- The Significance of Selected Myokines in Predicting the Length of Rehabilitation of Patients after COVID-19 Infection. Mińko A, Turoń-Skrzypińska A, Rył A, Mańkowska K, Cymbaluk-Płoska A, Rotter I. Mińko A, et al. Biomedicines. 2024 Apr 10;12(4):836. doi: 10.3390/biomedicines12040836. Biomedicines. 2024. PMID: 38672190 Free PMC article.
- Severe papilloedema with vision loss secondary to Guillain-Barré syndrome. Moloney NB, Ong J, Irani N. Moloney NB, et al. BMJ Case Rep. 2024 Apr 1;17(4):e259813. doi: 10.1136/bcr-2024-259813. BMJ Case Rep. 2024. PMID: 38565226
- External Validation of a Dynamic Prediction Model for Upper Limb Function After Stroke. Brunner IC, Andrinopoulou ER, Selles R, Lundquist CB, Pedersen AR. Brunner IC, et al. Arch Rehabil Res Clin Transl. 2023 Dec 21;6(1):100315. doi: 10.1016/j.arrct.2023.100315. eCollection 2024 Mar. Arch Rehabil Res Clin Transl. 2023. PMID: 38482101 Free PMC article.
Publication types
- Search in MeSH
Related information
Linkout - more resources, full text sources.
- MJS Publishing
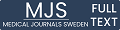
- Citation Manager
NCBI Literature Resources
MeSH PMC Bookshelf Disclaimer
The PubMed wordmark and PubMed logo are registered trademarks of the U.S. Department of Health and Human Services (HHS). Unauthorized use of these marks is strictly prohibited.
Select a Community
- MB 1 Preclinical Medical Students
- MB 2/3 Clinical Medical Students
- ORTHO Orthopaedic Surgery
Are you sure you want to trigger topic in your Anconeus AI algorithm?
You are done for today with this topic.
Would you like to start learning session with this topic items scheduled for future?
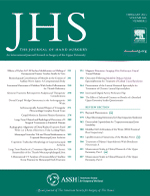
Use of the Medical Research Council muscle strength grading system in the upper extremity.
On a scale of 1 to 10, rate how much this article will change your clinical practice, will this article lead to more cost-effective healthcare, was this article biased (commercial or personal), what level of evidence do you think this article is.
Action | Numeric Key | Letter Key | Function Key |
---|---|---|---|
Show Bullets | S | Enter (frontside only) | |
20% | 1 | N | |
40% | 2 | H | |
60% | 3 | F | Enter (backside only) |
80% | 4 | E | |
100% | 5 | M | |
Previous Card | Left Arrow | ||
Next Card | N | Right Arrow | |
Toss | 0 | T |
Please Login to add comment
- Search Menu
- Sign in through your institution
- CNS Injury and Stroke
- Epilepsy and Sleep
- Movement Disorders
- Multiple Sclerosis/Neuroinflammation
- Neuro-oncology
- Neurodegeneration - Cellular & Molecular
- Neuromuscular Disease
- Neuropsychiatry
- Pain and Headache
- Advance articles
- Editor's Choice
- Author Guidelines
- Submission Site
- Why publish with this journal?
- Open Access
- About Brain
- Editorial Board
- Advertising and Corporate Services
- Journals Career Network
- Self-Archiving Policy
- Dispatch Dates
- Terms and Conditions
- Journals on Oxford Academic
- Books on Oxford Academic
Article Contents
Introduction, patients and methods, acknowledgements.
- < Previous
Modifying the Medical Research Council grading system through Rasch analyses
*The members of PeriNomS Study Group are provided in the Acknowledgements section.
- Article contents
- Figures & tables
- Supplementary Data
Els Karla Vanhoutte, Catharina Gerritdina Faber, Sonja Ingrid van Nes, Bart Casper Jacobs, Pieter Antoon van Doorn, Rinske van Koningsveld, David Reid Cornblath, Anneke Jelly van der Kooi, Elisabeth Aviva Cats, Leonard Hendrik van den Berg, Nicolette Claudia Notermans, Willem Lodewijk van der Pol, Mieke Catharina Elisabeth Hermans, Nadine Anna Maria Elisabeth van der Beek, Kenneth Craig Gorson, Marijke Eurelings, Jeroen Engelsman, Hendrik Boot, Ronaldus Jacobus Meijer, Giuseppe Lauria, Alan Tennant, Ingemar Sergio José Merkies, on behalf of the PeriNomS Study Group, Modifying the Medical Research Council grading system through Rasch analyses, Brain , Volume 135, Issue 5, May 2012, Pages 1639–1649, https://doi.org/10.1093/brain/awr318
- Permissions Icon Permissions
The Medical Research Council grading system has served through decades for the evaluation of muscle strength and has been recognized as a cardinal feature of daily neurological, rehabilitation and general medicine examination of patients, despite being respectfully criticized due to the unequal width of its response options. No study has systematically examined, through modern psychometric approach, whether physicians are able to properly use the Medical Research Council grades. The objectives of this study were: (i) to investigate physicians’ ability to discriminate among the Medical Research Council categories in patients with different neuromuscular disorders and with various degrees of weakness through thresholds examination using Rasch analysis as a modern psychometric method; (ii) to examine possible factors influencing physicians’ ability to apply the Medical Research Council categories through differential item function analyses; and (iii) to examine whether the widely used Medical Research Council 12 muscles sum score in patients with Guillain–Barré syndrome and chronic inflammatory demyelinating polyradiculoneuropathy would meet Rasch model's expectations. A total of 1065 patients were included from nine cohorts with the following diseases: Guillain–Barré syndrome ( n = 480); myotonic dystrophy type-1 ( n = 169); chronic inflammatory demyelinating polyradiculoneuropathy ( n = 139); limb-girdle muscular dystrophy ( n = 105); multifocal motor neuropathy ( n = 102); Pompe's disease ( n = 62) and monoclonal gammopathy of undetermined related polyneuropathy ( n = 8). Medical Research Council data of 72 muscles were collected. Rasch analyses were performed on Medical Research Council data for each cohort separately and after pooling data at the muscle level to increase category frequencies, and on the Medical Research Council sum score in patients with Guillain–Barré syndrome and chronic inflammatory demyelinating polyradiculoneuropathy. Disordered thresholds were demonstrated in 74–79% of the muscles examined, indicating physicians’ inability to discriminate between most Medical Research Council categories. Factors such as physicians’ experience or illness type did not influence these findings. Thresholds were restored after rescoring the Medical Research Council grades from six to four options (0, paralysis; 1, severe weakness; 2, slight weakness; 3, normal strength). The Medical Research Council sum score acceptably fulfilled Rasch model expectations after rescoring the response options and creating subsets to resolve local dependency and item bias on diagnosis. In conclusion, a modified, Rasch-built four response category Medical Research Council grading system is proposed, resolving clinicians’ inability to differentiate among its original response categories and improving clinical applicability. A modified Medical Research Council sum score at the interval level is presented and is recommended for future studies in Guillain–Barré syndrome and chronic inflammatory demyelinating polyradiculoneuropathy.
In 2005, a historical essay tracing the history of scoring and summation of neuromuscular weakness as part of daily neurological practice was published by Dyck et al. (2005) . Mitchell and Lewis (1886) initiated the practice of alphanumerical scoring of neurological signs in the 19th century. However, it was Lovett, an orthopaedic surgeon, who introduced an ordinal scoring of muscle weakness that formed the basis for the Mayo Clinics and Medical Research Council (MRC) manual muscle testing grading systems, of which the MRC system is most widely used ( Medical Research Council, 1943 ; Dyck et al. , 2005 ). Its worldwide recognition is most probably due to its simplicity, and drawings illustrating how limb muscles should be tested. Through the decades, various versions have been published that aimed to improve the methods for muscle examination. The 2010 edition of Aids to the Investigation of Peripheral Nerve Injuries. Medical Research Council: Nerve Injuries Research Committee was recently presented on behalf of the guarantors of Brain , embracing a historical review and appreciation for its nurtures through the decades ( Compston, 2010 ). Despite being the most cardinal feature of daily neurological practice, the MRC scale has been respectfully criticized due to the unequal width of its categories, with Grades 1, 2 and 3 being too narrow, and 4 being too broad, often leading to attempts to modify the scale ( Brandsma et al. , 1995 ; Dyck et al. , 2005 ; Cuthbert and Goodheart, 2007 ; MacAvoy and Green, 2007 ; Merlini, 2010 ).
One of the most common sources of improper use of any outcome measure concerns the inconsistent use of the response options that correspond to the scales’ items ( Tennant and Conaghan, 2007 ). This results in what is known as ‘reversed or disordered thresholds’. The term threshold refers to the point between two adjacent response categories where either response is equally probable. In the case of the MRC scale, a threshold would be the point between two adjacent categories, such as between MRC Grades 2 and 3. Disordered thresholds occur when physicians have difficulty consistently discriminating between the MRC grades in patients with various degrees of muscle weakness. Surprisingly, no study has systematically examined the appropriateness of the MRC scale using modern psychometric techniques.
The objectives of this study were: (i) to examine the applicability and discriminative capacity of physicians using the MRC grades in patients with various neuromuscular illnesses with different degrees of muscle weakness. We questioned whether physicians could demonstrate a fairly uniform MRC grades’ ordered thresholds pattern along the Rasch scale continuum, since previous reports suggested human's inability to differentiate between more than four response options ( Andrich, 1996 ; Penta et al. , 2001 ); (ii) to investigate the influence of factors possibly affecting the proper use of the MRC grades in clinical practice (such as physician's clinical experience). For these two objectives, the Rasch method as a modern psychometric vehicle was used, solely focusing on threshold and item bias examinations ( Rasch, 1960 ; Tennant and Conaghan, 2007 ); and (iii) since Guillain–Barré syndrome and chronic inflammatory demyelinating polyradiculoneuropathy (CIDP) are potentially treatable illnesses and the MRC sum score has been often used as an outcome measure to determine efficacy in these illnesses, we have chosen to examine whether this multi-item scale would fulfil all Rasch model expectations in patients with Guillain–Barré syndrome and CIDP and if not, to propose changes to improve its use ( Kleyweg et al. , 1991 ; van der Meche and Schmitz, 1992 ; Merkies, 2001 ; van Koningsveld et al. , 2004 ; Hughes et al. , 2008 )
The MRC grades of various muscles were collected from different neuromuscular seminal studies published in the last two decades. Most of these studies have guided the worldwide neurological community in understanding the clinical and therapeutic pattern of these illnesses. A total of 1065 patients (Guillain–Barré syndrome: n = 480; myotonic dystrophy type 1: n = 169; CIDP: n = 139; limb-girdle muscular dystrophy: n = 105; multifocal motor neuropathy: n = 102; Pompe's disease: n = 62; and monoclonal gammopathy related polyneuropathy of undetermined significance: n = 8) were included ( Table 1 and Supplementary Table 1 ) ( van der Meché and Schmitz, 1992 ; van der Kooi et al. , 1996 ; de Die-Smulders et al. , 1998 ; Van den Berg-Vos et al. , 2002 ; van Koningsveld et al. , 2004 ; Hagemans et al. , 2005 ; Van Asseldonk et al. , 2005 ; Hughes et al. , 2008 ; Hermans et al. , 2010 ). The initial MRC data of all patients from the above-mentioned cohorts were selected for the purposes of the current study. All patients met their international criteria for their illness ( Asbury and Cornblath, 1990 ; AANA, 1991 ; Bushby and Beckmann, 1995 ; Hirschhorn and Reuser, 2001 ; EFNS/PNS, 2006 ; Prior, 2009 ). The diagnosis ‘monoclonal gammopathy related polyneuropathy of undetermined significance’ was established after excluding all possible causes for the gammopathy and polyneuropathy ( Miescher and Steck, 1996 ). For all studies, consent was obtained according to the Declaration of Helsinki and approval was obtained by the Ethical Committee of the institution in which the original study was performed.
Basic characteristics of patients with neuromuscular disorders
Study/disorder . | Patients examined ( ) . | Age, mean years (SD), range . | Gender | Symptoms duration, mean (SD), range (years) . | |
---|---|---|---|---|---|
Female (%) . | Male (%) . | ||||
INCAT study | 113 | 54.3 (15.1), 14–84 | 54 (47.8) | 59 (52.2) | 6.9 (3.1), 0.5–28 |
Dutch Guillain–Barré syndrome trial, 1992 | 147 | 47.5 (19.2), 5–81 | 71 (48.3) | 76 (51.7) | – |
Dutch Guillain–Barré syndrome trial, 2004 + Guillain–Barré syndrome pilot study, 1994 | 250 | 50.5 (20.1), 7–89 | 109 (43.6) | 141 (56.4) | – |
Myotonic dystrophy type-1 | 169 | 43.5 (11.5), 18–69 | 83 (49.1) | 86 (50.9) | 5.3 (6.9), 0–34 |
Multifocal motor neuropathy | 102 | 54.3 (12.1), 26–79 | 76 (74.5) | 26 (25.5) | 11.8 (8.2), 0.2–43 |
Pompe's disease | 62 | 48.1 (11.9), 25.1–71.7 | 29 (46.8) | 33 (53.2) | 7.9 (9.3), 0–30.5 |
Limb-girdle muscular dystrophy | 105 | 37.8 (15.6), 3–70 | 64 (61.0) | 41 (39.0) | 21.0 (14.5), 0–58 |
ICE CIDP | 117 | 51.6 (16.5), 18–83 | 40 (34.2) | 77 (65.8) | 5.3 (6.2), 0.2–34.3 |
Study/disorder . | Patients examined ( ) . | Age, mean years (SD), range . | Gender | Symptoms duration, mean (SD), range (years) . | |
---|---|---|---|---|---|
Female (%) . | Male (%) . | ||||
INCAT study | 113 | 54.3 (15.1), 14–84 | 54 (47.8) | 59 (52.2) | 6.9 (3.1), 0.5–28 |
Dutch Guillain–Barré syndrome trial, 1992 | 147 | 47.5 (19.2), 5–81 | 71 (48.3) | 76 (51.7) | – |
Dutch Guillain–Barré syndrome trial, 2004 + Guillain–Barré syndrome pilot study, 1994 | 250 | 50.5 (20.1), 7–89 | 109 (43.6) | 141 (56.4) | – |
Myotonic dystrophy type-1 | 169 | 43.5 (11.5), 18–69 | 83 (49.1) | 86 (50.9) | 5.3 (6.9), 0–34 |
Multifocal motor neuropathy | 102 | 54.3 (12.1), 26–79 | 76 (74.5) | 26 (25.5) | 11.8 (8.2), 0.2–43 |
Pompe's disease | 62 | 48.1 (11.9), 25.1–71.7 | 29 (46.8) | 33 (53.2) | 7.9 (9.3), 0–30.5 |
Limb-girdle muscular dystrophy | 105 | 37.8 (15.6), 3–70 | 64 (61.0) | 41 (39.0) | 21.0 (14.5), 0–58 |
ICE CIDP | 117 | 51.6 (16.5), 18–83 | 40 (34.2) | 77 (65.8) | 5.3 (6.2), 0.2–34.3 |
In the INCAT studies, a total of 113 patients were examined (Guillain–Barré syndrome, n = 83; CIDP, n = 22 and monoclonal gammopathy-related polyneuropathy of undetermined significance, n = 8).
ICE CIDP = immune globulin intravenous for CIDP; INCAT = inflammatory neuropathy cause and treatment.
Assessment scale
The MRC grading system provides the following grades: 0, paralysis; 1, only a trace or flicker of muscle contraction is seen or felt; 2, muscle movement is possible with gravity eliminated; 3, muscle movement is possible against gravity; 4, muscle strength is reduced, but movement against resistance is possible and 5, normal strength.
The MRC grades of the following six muscle pairs comprise the MRC sum score for Guillain–Barré syndrome and CIDP: upper arm abductors, elbow flexors, wrist extensors, hip flexors, knee extensors and foot dorsal flexors ( Kleyweg et al. , 1991 ). In the remaining cohorts (monoclonal gammopathy of undetermined significance related polyneuropathy, multifocal motor neuropathy, mytonic dystrophy type-1, Pompe's disease and limb-girdle muscular dystrophy), the muscles groups evaluated represented the clinical picture of each illness (see Supplementary Table 1 for available muscles per cohort).
Rasch analysis
Rationale for using rasch method.
In health care, outcome measures often consist of ordinal multi-item questionnaires, based on the classical test theory ( DeVellis, 2006 ). Concerns have been raised about inappropriate analysis of the generated summed scores that are erroneously assumed to be at the interval level ( Wright, 1999 ; Svensson, 2001 ; DeVellis, 2006 ). The ability of a scale to provide fundamental measurements should be established before the more commonly reported psychometric attributes such as being simple, valid, reliable and responsive ( Tennant et al. , 2004 a ; Tennant and Conaghan, 2007 ). Modern scientific methods have been adopted to overcome the shortcomings of traditional measurements. One of the most widely used modern techniques is the Rasch method that transforms ordinal obtained scores into interval-level variables, and whose fit of data satisfies numerous checkpoints as part of model expectations ( Rasch, 1960 ; Tennant et al. , 2004 a ; Tennant and Conaghan, 2007 ).
In the current study setting, the Rasch model assumes that a patient with less weakness (thus more strength) will have a greater chance of receiving a higher MRC grade by the examining physician. A comprehensive description of the Rasch analysis specifically for neurologists is provided elsewhere ( Pallant and Tennant, 2007 ; Tennant and Conaghan, 2007 ; van Nes et al. , 2011 ). Briefly, the Rasch model shows what should be expected in response to ordinal items if interval scaling is to be achieved. For this, the following criteria should be fulfilled.
Thresholds examination: when using items with more than two response categories, as for the MRC grades, proper ordering of the response options should be verified using category probability curves for each muscle group examined, since this will reflect the ability of physicians to use the MRC in a correct way ( Shaw et al. , 1992 ). Ordered thresholds are where the transition (threshold) between categories map on to the underlying construct in the expected manner. Thus the transition between categories (e.g. 1–2 and 2–3) reflects increasing levels of muscle strength ( Fig. 1 , top). Disordered thresholds can occur when physicians use the response options inconsistently, and this inconsistency can be a source of misfit to model expectations. The difficulty discriminating between response options may be a result of too many options, or where the labelling of the options is confusing, both of which may lead to misinterpretation.
Fit statistics: fit statistics give an indication of how well the items fit the expected ordering required by the model. This ordering is a probabilistic version of Guttman Scaling ( Guttman, 1950 ). There are two general categories for detecting misfit: overall (summary) misfit, using the entire response matrix, and the individual fit (examining all items and all persons individually). At the summary level the overall mean residual values for both persons and items can be calculated. These values are expressed as a z -score with a mean of 0 and a SD of 1, values of which indicate perfect fit to model expectations ( Tennant et al. , 2004 b ; Pallant and Tennant, 2007 ). The summary item–trait interaction statistic reflects the fit of the observed data to the model's expectations and is represented by the chi-square. This statistic gives an indication of the invariance of the ordering of items across patients with different levels of muscle strength. A significant chi-square indicates a failure to retain this ordering. Besides the overall fit residuals, individual item–chi square and item and person residuals can be calculated ( Tennant et al. , 2004 b ; Pallant and Tennant, 2007 ; Vandervelde et al. , 2007 ).
Item bias: response to an item should not vary between groups (e.g. males versus females), given the same level of the underlying trait (e.g. muscle strength). We assessed item bias (differential item functioning) on the MRC data for various available person factors. A panel (I.S.J.M. and C.G.F.) have studied the range of the factors age, disease duration and physician's experience in the available cohorts. Subsequently, these factors were categorized into subgroups for item bias analyses, aiming for an equivalent distribution of participants among the subgroups (25–33% per subgroup).
Local dependency: local dependency arises when items are linked such that the response on one item is dependent upon the response to another. Item sets with correlations >0.3 are considered a source of misfit to the model ( Tennant and Conaghan, 2007 ).
Unidimensionality: the Rasch model assumes unidimensionality and consequently post hoc tests are included in the analysis to ensure that this assumption holds. These tests involve a comparison of person estimates (of muscle strength) based upon two sets of items identified from the first principal component analysis of the residuals. The estimates for every individual are compared by a t -test, and where <5% of these comparisons are significantly different, this is taken to support the assumption of unidimensionality ( Smith, 2002 ).
MRC response categories related thresholds explained and coded as ‘normal’ (green) or ‘abnormal’ (red)’. The first row shows the ideal graph representation for proper thresholds for the MRC grades. The first threshold at the intersection between MRC response options 0 and 1 corresponds to a 50% chance of choosing between these two adjacent categories. The thresholds should be ordered to obtain an ideal graph: Threshold 1 < Threshold 2 < Threshold 3 < Threshold 4 < Threshold 5. The second and third row give graphical examples of proper threshold ordering (coded as a green box) and disordered threshold (coded as a red box), respectively. T1–T5 = Thresholds 1–5, respectively.
Test procedure
Figure 2 presents a systematic ordering of the analyses performed in the current study. In Analyses 1 and 2 (MRC Rasch analyses for each cohort separately and MRC Rasch analyses after pooling data) the following were examined:
Step 1: the presence of ordered thresholds, thus determining whether the MRC grades for each muscle were ordered reflecting physicians’ ability to use these grades properly;
Step 2: in case of disordered thresholds: to seek for the most optimal modified MRC rescored categories that could serve as a unified tool in manual muscle scoring for all muscle groups. In order to rescore the MRC categories, the frequency distribution among the categories and the category probability curves were taken into account;
Step 3: the presence of possible item bias was examined to determine whether factors such as physician's experience in the neuromuscular field (i.e. would a more experienced physician apply the MRC grades more appropriately than a less experienced physician?) or possible differences between community and university based neurologists might influence the applicability of the MRC grades.
Study algorithm showing a systematic ordering of the analyses performed in the current study. First analyses (Analysis 1): initial MRC Rasch analysis for each individual cohort separately (thus performing a total of eight individual model analyses). Second analyses (Analysis 2): MRC Rasch analyses after pooling data at the muscle level from available cohorts. Third analyses (Analysis 3): MRC sum score Rasch analysis in patients with Guillain–Barré syndrome and CIDP. DM1 = myotonic dystrophy type-1; ICE = immune globulin intravenous for CISP; INCAT = inflammatory neuropathy cause and treatment; LGMD = limb-girdle muscular dystrophy; MMN = multifocal motor neuropathy.
Therefore, in Analyses 1 and 2, the Rasch method was applied only to examine the ability of physicians to use the MRC grading system in a proper way and to determine whether there were factors influencing its use. These analyses were not intended to create a formal Rasch-built MRC sum score for each cohort individually, since some of the cohort samples were relatively small, hence not fulfilling the basic requirements for proper Rasch modelling ( Linacre, 1994 ).
For Analysis 2, MRC data were pooled at the muscle level from the various available cohorts and resubjected to Rasch analysis, thereby controlling for diagnosis as a possible confounder and strengthening the category frequencies for the various muscles ( Linacre, 2002 ).
In Analysis 3 (MRC sum score Rasch analysis in Guillain–Barré syndrome/CIDP), the MRC 12 muscles sum score was analysed to determine whether Rasch model expectations would be met. The first two steps for Analyses 1 and 2 (see above) were also performed here. Subsequently, since there is no consensus regarding a fixed sequence of steps that must be followed when doing Rasch analyses, our rationale for the following steps were constantly driven by the biggest abnormality seen when studying all subjected data to Rasch, thereby focusing on all aspects that did not meet model expectations (misfit statistics, fit residuals disturbances, under-/overfitting, local dependency >0.3, and item bias). All steps needed were taken to create a unidimensional scale at the interval level.
Rasch general aspects, person factors and statistics
The MRC data of each muscle group were treated as if it was an ‘item’ that needed to be completed by the patients with response options from 0 to 5 (in the current study setting: a physician completed the ‘item’) using the Rasch Unidimensional Measurement Model 2020 software ( Andrich et al. , 2003 ).
In Analysis 1 (MRC Rasch in each cohort separately), the following person factors were taken into account ( Supplementary Table 2 ):
Ages: 1, <40 years; 2, 40–59 years and 3, ≥60 years;
gender: 0, female; 1, male;
type of disease: (a) inflammatory neuropathy-cause-and-treatment cohort: 1, Guillain–Barré syndrome; 2, CIDP; 3, gammopathy related polyneuropathy; (b) myotonic dystrophy cohort: 1, mild; 2, adult; 3, child/congenital type; and (c) limb-girdle dystrophy cohort: 1, sarcoglycanopathy; 2, calpainopathy; 3, limb-girdle type 1B, 2B and 2I; 4, unclassified;
duration of disease: (a) for all cohorts except limb-girdle patients: 1, <5 years; 2, 5–9 years; 3, 10–19 years; 4, ≥20 years; and (b) for limb-girdle cohort: 1, <10 years; 2, 10–19 years; 3, 20–29 years; 4, ≥30 years;
physician's experience in the neuromuscular field: for the inflammatory–neuropathy cause and treatment studies: 1, <3 years experience; 2, 3–5 years experience; 3, ≥6 years experience; the latter group constituting senior neuromuscular experts;
institution; for the Guillain–Barré syndrome trials: 0, community based; 1, university based hospital; and
country; for the Guillain–Barré syndrome cohort 2004: 1, The Netherlands; 2, Germany; 3, Belgium.
For Analyses 2 and 3 (MRC Rasch after pooling data and MRC sum score in Guillain–Barré syndrome/CIDP), the factors studied included (i) age category: 1, <40 years; 2, 40–59 years; 3, ≥60 years; (ii) gender: 0, female; 1, male; and (iii) type of disease: depending on the amount of illnesses being pooled together, each illness received a separate code.
For the MRC sum score analysis, the person separation index was also determined, which should be ≥0.7 for proper group comparison, and a minimum of 0.9 for clinical use ( Bland and Altman, 1997 ). The unrestricted partial credit Rasch model was used. Further analyses were undertaken using Stata 11.0 statistical software for Windows XP.
General aspects
A total of 1065 patients with various neuromuscular disorders were included from nine studies. Table 1 presents the patients’ characteristics. MRC data on 72 muscle groups were available ( Supplementary Table 1 , muscle groups assessed per cohort).
Analysis 1: initial MRC Rasch analyses for each cohort separately
Step 1: thresholds examination.
The obtained data (ordered thresholds coded ‘green’; disordered coded ‘red’; see Fig. 1 explaining these codes) for each muscle group in each cohort were summed, thereby creating a total of 210 muscle groups examined. A total of 165 (78.6%) muscle groups had disordered thresholds versus 45 (21.4%) with ordered thresholds. The disordered thresholds were particularly seen in the mid-response MRC category area (options 2 to 4).
Step 2: rescoring MRC categories
A panel of neuromuscular and Rasch researchers studied the category probability curves and category frequencies of the MRC data for each muscle group. Subsequently, all muscle groups were systematically rescored in order to obtain the maximum uniform amount of response options, which turned out to be four categories (instead of six). Of the 210 muscle groups rescored, 182 (86.7%) had ordered thresholds and 28 (13.3%) were still disordered. Sixteen of these disordered muscle groups were distally located (finger spreaders, flexors and extensors, grip strength, wrist extensors and flexors, foot dorsal and plantar flexors). All disordered muscle groups except two were found in the two cohorts with the lowest number of patient's records (multifocal motor neuropathy, n = 102 and Pompe's disease, n = 62).
Results before and after rescoring the response options from six to four categories with corresponding threshold locations
A normal threshold ordering of the MRC grades is coded as ‘normal’; abnormal threshold is ‘abnormal’. See Fig. 1 , for examples, explaining these codes. Threshold location = location of the thresholds of adjacent MRC response options located on the created ruler (and expressed in logits).
Step 3: item bias examination
Eight selected person factors were used to examine possible item bias on the available muscle groups (see Supplementary Table 2 for available factors per cohort). Before rescoring, a total of 806 muscle groups (96.9%) were free of item bias, thus not being influenced by person factors like physicians’ experience. Item bias was only found in 26 muscles (3.1%; on person factor gender: 11 muscle groups had uniform differential item functioning, on disease type: eight had uniform, on disease duration: two uniform and one non-uniform, on physician's experience: two uniform, on country: one uniform, and on age: one muscle group had uniform differential item functioning). Differential item functioning findings did not change after rescoring at the individual cohort level.
Analysis 2: MRC Rasch analyses after pooling data
Similar findings were seen in the pooled data analyses. Of the 72 muscles examined, a total of 53 muscle groups (73.6%) had disordered threshold, particularly in the mid-categories ( Table 2 , ‘before rescoring’).
Equivalent to the findings of Analysis 1 and based on the location seen of the disordered thresholds (mid-categories 2–4), all muscle groups were systematically rescored to a modified MRC with four categories. Table 2 provides the data for the rescored MRC categories (see last four columns). Ordered thresholds were restored for all muscles except the masseter muscle. A modified version of the MRC grading system was created for clinical use with the following grades: 0, paralysis; 1, severe weakness (defined as >50% loss of strength); 2, slight weakness (<50% loss of strength); and 3, normal strength. A 50% cut-off was based on the following: having four modified response options as having three thresholds (three theoretical intersections between adjacent response options: Thresholds 1, 2 and 3); half of the distance between Threshold 3 (representing the intersection between modified MRC Grades 2 and 3; location 4.3 logits) and Threshold 1 (intersection between modified grades 0 and 1; location −2.98) for all 72 muscle groups is located at 0.66 logits [−2.98 (location Threshold 1) + 0.5 × 7.28 (0.5 × distances between Threshold 3 and Threshold 1)], which is close to the mean for Threshold 2 (intersection between the modified Grades 1 and 2): 0.46.
Differential item functioning was also performed on person factors age, gender and diagnosis ( Supplementary Table 3 ). Item bias was hardly seen on age and gender. On diagnosis, 33 muscle groups (45.8%) demonstrated differential item functioning ( Supplementary Table 3 ).
Analysis 3: MRC sum score Rasch analysis in patients with Guillain–Barré syndrome and chronic inflammatory demyelinating polyradiculoneuropathy
Step 0: general description of patients examined and initial findings.
A total of 619 patients from several cohorts [Guillain–Barré syndrome, n = 480; CIDP, n = 139; n = 272 females (43.9%) and n = 347 males (56.1%)] were available for these analyses ( van der Meche and Schmitz, 1992 ; The Dutch Guillain–Barré syndrome study group, 1994 ; Merkies, 2001 ; van Koningsveld et al. , 2004 ; Hughes et al. , 2008 ). The original MRC summed score failed to meet the model expectations. Misfit statistical findings for all three statistical parameters were initially seen ( Table 3 , ‘initial’ analysis).
Summary Rasch analyses statistics for the modification of MRC sum score in patients with Guillain−Barré syndrome and CIDP
Analysis . | Item fit residuals . | Person fit residuals . | Item-trait chi-square interaction | PSI . | Unidimensionality independent -test (95%CI) . | |
---|---|---|---|---|---|---|
. | Mean (SD) . | Mean (SD) . | DF . | -value . | . | . |
Initial | 0.147 (4.626) | −0.562 (1.749) | 108 | <0.00001 | 0.94 | 0.20 (0.183–0.218) |
Final | 0.341 (1.100) | −0.316 (1.094) | 55 | 0.0891 | 0.91 | NA |
Analysis . | Item fit residuals . | Person fit residuals . | Item-trait chi-square interaction | PSI . | Unidimensionality independent -test (95%CI) . | |
---|---|---|---|---|---|---|
. | Mean (SD) . | Mean (SD) . | DF . | -value . | . | . |
Initial | 0.147 (4.626) | −0.562 (1.749) | 108 | <0.00001 | 0.94 | 0.20 (0.183–0.218) |
Final | 0.341 (1.100) | −0.316 (1.094) | 55 | 0.0891 | 0.91 | NA |
In the final analysis, item and person fit residuals are acceptable, whereas chi-square is non-significant, indicating invariance across the trait. A person separation index of 0.91 indicates a reliable internal consistency. NA = not available; after performing split analyses, Rasch Unidimensional Measurement Model does not provide the opportunity to perform unidimensionality testing.
DF = degrees of freedom; PSI = person separation index.
Steps 1 and 2: thresholds examination and rescoring
Similar findings were seen here as the above-mentioned analyses. Eight muscle groups had disordered threshold. For uniformity, all 12 muscle groups were rescored to four response options, thereby restoring threshold ordering.
Step 3: local dependency and creating subsets
The following steps were driven by the strongest misfit seen to the Rasch model, which was found to be the strong local dependency findings of equivalent (right and left) muscle pairs (e.g. shoulder abductors right and left side; Spearman's correlations: ρ = 0.676–0.831). Therefore, six subsets of items were created, by combining the corresponding muscle pairs (left and right) with each other, improving the statistical parameters and resolving local dependency.
Step 4: unidimensionality examination
Based on the first principal components analysis, two comparison groups of subsets were formed with three positively loaded (arm muscle subsets) versus three negatively loaded (leg muscle subsets). The independent t -tests between these two groups suggested acceptable unidimensionality [ t -test (95% confidence interval): 0.065 (0.047–0.082)].
Step 5: item bias examination
Uniform differential item functioning was demonstrated on person factor ‘disease type’ for all created muscle subsets, except for the elbow flexors subset. Therefore, each subset of muscle pairs was split in order to obtain specific subsets for the patients with Guillain–Barré syndrome and CIDP, separately. After this, the model was free of any item bias and local dependency. All subsets of items, except the ‘foot dorsal flexors for patients with Guillain–Barré syndrome’, demonstrated fit statistics within required limits. The foot dorsal flexors in Guillain–Barré syndrome had a fit residual of +5.845 ( P = 0.000021), which disturbed Rasch model fitting ( Table 3 , final analysis for complete model fit after removing this item). However, for practical reasons the structure of the MRC sum score (composed by 12 muscles) was maintained, despite having skewed foot dorsal flexors in the Guillain–Barré syndrome subset of item. A high person separation index (0.91) was obtained for the final modified MRC sum score model.
Manual muscle testing has been used for more than seven decades for monitoring disease progression and response to therapy in various neuromuscular disorders ( van der Meche and Schmitz, 1992 ; van der Kooi et al. , 1996 ; de Die-Smulders et al. , 1998 ; Merkies, 2001 ; Van den Berg-Vos et al. , 2002 ; van Koningsveld et al. , 2004 ; Hagemans et al. , 2005 ; Van Asseldonk et al. , 2005 ; Hughes et al. , 2008 ; Hermans et al. , 2010 ) and the MRC grading system has been widely used for this purpose ( Dyck et al. , 2005 ; Compston, 2010 ). This study systematically examined the discriminatory capacity of the MRC grading system in a broad mixture of patients with neuromuscular illnesses, assessing a large number of muscles using the Rasch method. The original six response categories of the MRC grading system failed to differentiate among patients with various degrees of muscle weakness. Three-quarters of all muscles examined demonstrated disordered thresholds, especially in the mid-response categories (options 2–4). The inability of physicians to apply the apparently intuitive and easily applicable MRC grades in a proper way is consistent with reports criticizing the MRC system ( Dyck et al. , 2005 ; Schreuders et al. , 2006 ; MacAvoy and Green, 2007 ; Merlini, 2010 ). The current paper also shows that the observed disordered thresholds were generally independent of factors such as physicians’ experience, duration of illness or type of practice (university- versus community-based). The original MRC grading system inconsistencies were also ‘cross-validated’ throughout the neuromuscular cohorts, as the findings between the individual disease cohorts were equivalent.
After systematically rescoring all MRC grades to a modified four category response option, the accuracy of the MRC grading system increased by fulfilling ordered thresholds requirements. While this change from six to four response options might intuitively lower the ability to capture functional changes in a patient, from the current evidence, however, keeping the six responses will give a false sense of precision and potentially increase the error in assessment, which may lead to a false sense of clinically meaningful improvement when it may not exist.
The current paper shows the difficulties with the use of summed scores derived from various muscles tested in patients with Guillain–Barré syndrome and CIDP. However, after Rasch modelling, we were able to present a transformed modified MRC 12 muscle groups summed score for use in future clinical studies in these disorders ( Kleyweg et al. , 1991 ). The analyses revealed severe misfit of the foot dorsal flexors. However, since Guillain–Barré syndrome and CIDP are length-dependent neuropathies, we decided to keep this muscle group in the final model. The presented Rasch-built modified interval MRC sum score is considered a substantial improvement compared to the evaluation of muscle strength using ordinal based scores, which in essence are not suitable for performing adequate statistics. The modified interval MRC sum score for patients with CIDP should, however, be applied with some caution, because only 139 patients were assessed, which is lower than the proposed sample size requirements for a stable model ( Linacre, 1994 ). Also, the responsiveness of the Rasch-built modified interval MRC summed score for patients with Guillain–Barré syndrome and CIDP needs to be demonstrated in longitudinal studies, which is currently being investigated ( Liang, 1995 ). However, its personal separation index was high, indicating good ability of the modified scale to differentiate between groups of patients with various degrees for muscle weakness. Finally, since the differential item functioning findings on diagnosis ( Supplementary Table 3 ) demonstrate that neuromuscular illnesses may behave differently, it is conceivable that Rasch-built MRC sum scores are needed for specific illnesses such as multifocal motor neuropathy and other neuromuscular diseases. These efforts should be the focus of future studies.
In conclusion, the original MRC manual muscle testing grading system failed to meet the Rasch model expectations in various neuromuscular disorders, despite being the standard metric in neurology worldwide. Modification of this grading system to four response categories (0, paralysis; 1, severe weakness; 2, slight weakness; and 3, normal strength) may significantly enhance the ability of clinicians to differentiate degrees of weakness with greater precision and accuracy. Based on this, we have developed a Rasch-built interval MRC summed score for use in future clinical studies evaluating patients with Guillain–Barré syndrome and CIDP. Future studies are warranted to improve the solidness of our neurological assessments.
We thank Professor S. Waxman from the Yale University, USA who helped us to increase the transparency and reading of the manuscript. The members of PeriNomS Study Group are as follows: A.A. Barreira, Brazil; D. Bennett, UK; P.Y.K. van den Bergh, Belgium; V. Bril, Canada; G. Devigili, Italy; R.D. Hadden, UK; A.F. Hahn, Canada; H.-P. Hartung, Germany; R.A.C. Hughes, UK; I. Illa, Spain; H. Katzberg, Canada; A.J. van der Kooi, The Netherlands; J.-M. Léger, France; R.A. Lewis, USA; M.P.T. Lunn, UK; O.J.M. Nascimento, Brazil; E. Nobile-Orazio, Italy; L. Padua, Italy; J. Pouget, France; M.M. Reilly, UK, I. van Schaik, The Netherlands; B. Smith, USA; M. de Visser, The Netherlands; D. Walk, USA
Abbreviations
chronic inflammatory demyelinating polyradiculoneuropathy
Medical Research Council
Google Scholar
Google Preview
Author notes
- myotonic dystrophy
- neuromuscular diseases
- glycogen storage disease type ii
- guillain-barre syndrome
- muscular dystrophy, limb girdle
- polyneuropathy, demyelinating, inflammatory, chronic
- polyneuropathies
- psychometrics
- monoclonal gammopathy of undetermined significance
- neurological rehabilitation
- multifocal motor neuropathy
- medical research
- muscle strength
- grading system
- rasch model
Supplementary data
Month: | Total Views: |
---|---|
December 2016 | 3 |
January 2017 | 6 |
February 2017 | 21 |
March 2017 | 19 |
April 2017 | 28 |
May 2017 | 7 |
June 2017 | 14 |
July 2017 | 6 |
August 2017 | 15 |
September 2017 | 8 |
October 2017 | 10 |
November 2017 | 11 |
December 2017 | 38 |
January 2018 | 41 |
February 2018 | 67 |
March 2018 | 50 |
April 2018 | 47 |
May 2018 | 63 |
June 2018 | 46 |
July 2018 | 22 |
August 2018 | 29 |
September 2018 | 37 |
October 2018 | 39 |
November 2018 | 63 |
December 2018 | 51 |
January 2019 | 45 |
February 2019 | 57 |
March 2019 | 67 |
April 2019 | 133 |
May 2019 | 74 |
June 2019 | 65 |
July 2019 | 47 |
August 2019 | 65 |
September 2019 | 81 |
October 2019 | 65 |
November 2019 | 56 |
December 2019 | 59 |
January 2020 | 82 |
February 2020 | 71 |
March 2020 | 85 |
April 2020 | 62 |
May 2020 | 73 |
June 2020 | 108 |
July 2020 | 112 |
August 2020 | 99 |
September 2020 | 133 |
October 2020 | 147 |
November 2020 | 115 |
December 2020 | 100 |
January 2021 | 152 |
February 2021 | 157 |
March 2021 | 195 |
April 2021 | 174 |
May 2021 | 146 |
June 2021 | 112 |
July 2021 | 116 |
August 2021 | 125 |
September 2021 | 130 |
October 2021 | 117 |
November 2021 | 143 |
December 2021 | 127 |
January 2022 | 144 |
February 2022 | 150 |
March 2022 | 138 |
April 2022 | 177 |
May 2022 | 102 |
June 2022 | 147 |
July 2022 | 127 |
August 2022 | 124 |
September 2022 | 137 |
October 2022 | 147 |
November 2022 | 161 |
December 2022 | 143 |
January 2023 | 122 |
February 2023 | 111 |
March 2023 | 129 |
April 2023 | 176 |
May 2023 | 137 |
June 2023 | 90 |
July 2023 | 60 |
August 2023 | 70 |
September 2023 | 120 |
October 2023 | 98 |
November 2023 | 139 |
December 2023 | 113 |
January 2024 | 188 |
February 2024 | 167 |
March 2024 | 196 |
April 2024 | 162 |
May 2024 | 159 |
June 2024 | 125 |
July 2024 | 34 |
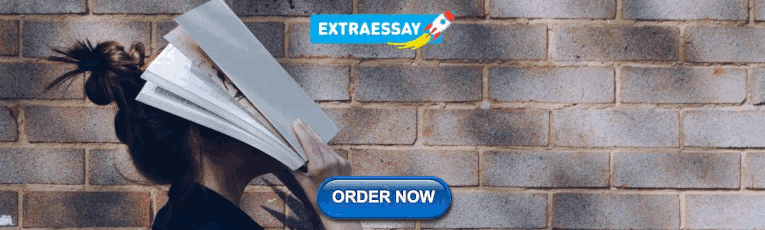
Email alerts
Citing articles via, looking for your next opportunity.
- Contact the editorial office
- Guarantors of Brain
- Recommend to your Library
Affiliations
- Online ISSN 1460-2156
- Print ISSN 0006-8950
- Copyright © 2024 Guarantors of Brain
- About Oxford Academic
- Publish journals with us
- University press partners
- What we publish
- New features
- Open access
- Institutional account management
- Rights and permissions
- Get help with access
- Accessibility
- Advertising
- Media enquiries
- Oxford University Press
- Oxford Languages
- University of Oxford
Oxford University Press is a department of the University of Oxford. It furthers the University's objective of excellence in research, scholarship, and education by publishing worldwide
- Copyright © 2024 Oxford University Press
- Cookie settings
- Cookie policy
- Privacy policy
- Legal notice
This Feature Is Available To Subscribers Only
Sign In or Create an Account
This PDF is available to Subscribers Only
For full access to this pdf, sign in to an existing account, or purchase an annual subscription.
17 Best Medical schools in Saint Petersburg
Updated: February 29, 2024
- Art & Design
- Computer Science
- Engineering
- Environmental Science
- Liberal Arts & Social Sciences
- Mathematics
Below is a list of best universities in Saint Petersburg ranked based on their research performance in Medicine. A graph of 271K citations received by 35.9K academic papers made by 17 universities in Saint Petersburg was used to calculate publications' ratings, which then were adjusted for release dates and added to final scores.
We don't distinguish between undergraduate and graduate programs nor do we adjust for current majors offered. You can find information about granted degrees on a university page but always double-check with the university website.
1. St. Petersburg State University
For Medicine
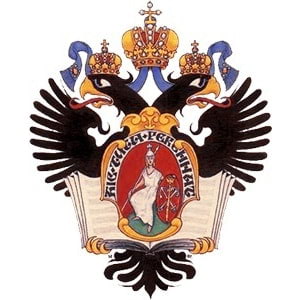
2. Pavlov First Saint Petersburg State Medical University
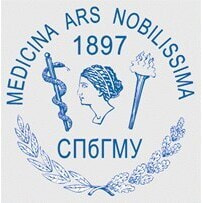
3. Saint Petersburg State Pediatric Medical Academy
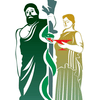
4. ITMO University
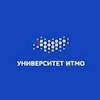
5. Peter the Great St.Petersburg Polytechnic University
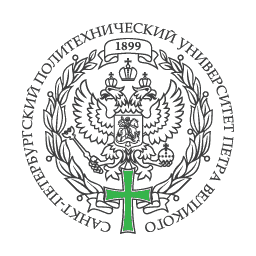
6. Saint Petersburg State Institute of Technology
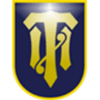
7. Saint Petersburg State Electrotechnical University
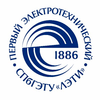
8. Leningrad State University
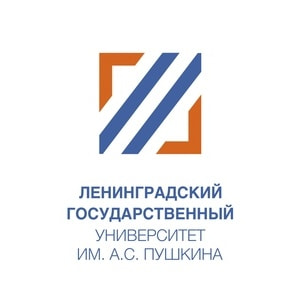
9. St. Petersburg State University of Architecture and Civil Engineering
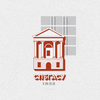
10. Saint-Petersburg Mining University
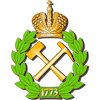
11. St. Petersburg State University of Aerospace Instrumentation
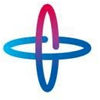
12. St. Petersburg State University of Economics
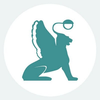
13. European University at St. Petersburg
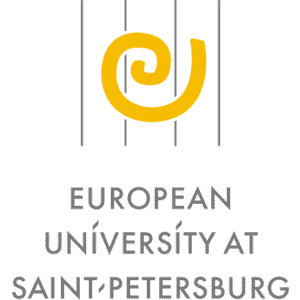
14. Baltic State Technical University "Voenmeh"
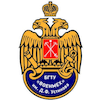
15. Bonch-Bruevich St. Petersburg State University of Telecommunications
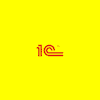
16. Russian State Hydrometeorological University
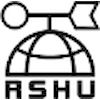
17. St. Petersburg State University of Civil Aviation
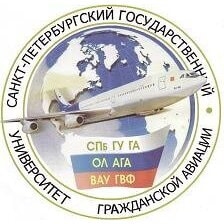
Universities for Medicine near Saint Petersburg
University | City | ||
---|---|---|---|
174 | 9 | Lappeenranta | |
269 | 1 | Tartu | |
270 | 3 | Tartu | |
296 | 5 | Joensuu | |
299 | 1 | Helsinki | |
306 | 8 | Espoo | |
316 | 4 | Tallinn | |
323 | 2 | Tallinn | |
354 | 6 | Jyvaskyla | |
395 | 3 | Tampere |
Medicine subfields in Saint Petersburg

- News & Insights
- Education System
- Grading System
- Credentials
- Universities
Grading System for Saint Petersburg State University
Matching grading scales:, russia/ussr most common.
Grade | Scale | Grade Description | US Grade |
---|---|---|---|
5.00 | Excellent (отлично) | A | |
4.00 - 4.99 | Good (хорошо) | B | |
3.00 - 3.99 | Satisfactory (удовлетворительно) | C | |
P | Credit (зачёт) | S | |
2.00 - 2.99 | Unsatisfactory (неудовлетворительно) | F |

An official website of the United States government
The .gov means it’s official. Federal government websites often end in .gov or .mil. Before sharing sensitive information, make sure you’re on a federal government site.
The site is secure. The https:// ensures that you are connecting to the official website and that any information you provide is encrypted and transmitted securely.
- Publications
- Account settings
Preview improvements coming to the PMC website in October 2024. Learn More or Try it out now .
- Advanced Search
- Journal List
- Front Genet
Truncating Variant in Myof Gene Is Associated With Limb-Girdle Type Muscular Dystrophy and Cardiomyopathy
Artem kiselev.
1 Department of Molecular Biology and Genetics, Almazov National Medical Research Centre, Saint Petersburg, Russia
2 Department of Molecular Medicine and Surgery, Centre for Molecular Medicine, Karolinska Institutet, Stockholm, Sweden
Anastasia Knyazeva
Alexey sergushichev.
3 Computer Technologies Laboratory, ITMO University, Saint Petersburg, Russia
Renata Dmitrieva
Aleksandr khudiakov, john jorholt.
4 Department of Women’s and Children’s Health, Karolinska Institutet, Stockholm, Sweden
Natalia Smolina
Ksenia sukhareva, yulia fomicheva, evgeny mikhaylov.
5 Arrhythmia Department, Almazov National Medical Research Centre, Saint Petersburg, Russia
Lubov Mitrofanova
Alexander predeus.
6 Bioinformatics Institute, Saint Petersburg, Russia
Gunnar Sjoberg
Dmitriy rudenko, thomas sejersen, anna lindstrand.
7 Clinical Genetics, Karolinska University Laboratory, Karolinska University Hospital, Stockholm, Sweden
Anna Kostareva
Associated data.
The dataset supporting the conclusions of this article is available in GEO repository ( {"type":"entrez-geo","attrs":{"text":"GSE119027","term_id":"119027"}} GSE119027 ) https://www.ncbi.nlm.nih.gov/geo/query/acc.cgi?acc=GSE119027 and can be found in the Supplementary Material .
Even though genetic studies of individuals with neuromuscular diseases have uncovered the molecular background of many cardiac disorders such as cardiomyopathies and inherited arrhythmic syndromes, the genetic cause of a proportion of cardiomyopathies associated with neuromuscular phenotype still remains unknown. Here, we present an individual with a combination of cardiomyopathy and limb-girdle type muscular dystrophy where whole exome sequencing identified myoferlin ( MYOF )—a member of the Ferlin protein family and close homolog of DYSF —as the most likely candidate gene. The disease-causative role of the identified variant c.[2576delG; 2575G>C], p.G859QfsTer8 is supported by functional studies in vitro using the primary patient’s skeletal muscle mesenchymal progenitor cells, including both RNA sequencing and morphological studies, as well as recapitulating the muscle phenotype in vivo in zebrafish. We provide the first evidence supporting a role of MYOF in human muscle disease.
Introduction
Approximately 25% of genes associated with cardiomyopathy also cause neuromuscular disorders, and genetic studies of neuromuscular diseases have contributed substantially to uncover the molecular background of cardiac disorders ( Bondue et al., 2018 ). The list of those genes was substantially extended due to availability of next-generation sequencing; however, the genetic cause of a proportion of cardiomyopathies associated with neuromuscular phenotype remains unknown.
Myoferlin is a member of the Ferlin protein family with a role in vesicle trafficking, membrane fusion, and repair ( Posey et al., 2011 ). Two of these protein family members, Dysferlin (DYSF) and Otoferlin (OTOF), are well known in connection to human genetic disorders: DYSF gene was linked to limb-girdle muscle dystrophy 2B (LGMD2B) (MIM#253601) and Miyoshi myopathy (MIM#254130), while OTOF was reported as a causative gene for non-syndromic hearing loss (MIM#601071). Eventhough DYSF pathogenic variants are mainly associated with LGMD2B and Miyoshi myopathy, subtle cardiac dysfunction have also been described in patients with DYSF mutations ( Wenzel et al., 2007 ; Choi et al., 2010 ; Nishikawa et al., 2016 ), and supported by a number of experimental studies ( Han et al., 2007 ; Wang et al., 2015 ). These studies suggest a potential role for the Ferlin family of proteins in the development of cardiac and skeletal muscle disorders.
Myoferlin ( MYOF ) was first cloned in 2000 by Davis et al. and mainly described in connection to cancer cell invasion ( Blomme et al., 2016 ; Zhang et al., 2018 ). In spite of extensive research, no phenotype linked to MYOF mutation has been reported yet ( Davis et al., 2000 ; Bonne et. al., 2017 ). Nevertheless, in vitro studies showed that MYOF has a role in myoblast fusion, with loss of MYOF in the mouse model supporting this finding, as MYOF -null mice present with smaller myofibers due to reduced cell fusion ( Doherty et al., 2005 ). Here, we present a clinical case of cardiomyopathy associated with limb-girdle type muscular dystrophy. Target sequencing of cardiomyopathy-associated genes did not identify any pathogenic variants. Utilizing whole exome sequencing (WES), we uncovered MYOF as the most likely candidate gene. Morphological and expression studies of the patient’s cells as well as a zebrafish knockdown model strongly support a role for MYOF variants in cardiac and skeletal muscle disorders.
Materials and Methods
Ethics approval and consent to participate.
The study was performed according to the Declaration of Helsinki, and approval was obtained from the Ethical Review Boards of Karolinska Institute and Almazov National Medical Research Centre, approval number 2016/54. Written informed consent was obtained from both the patient and healthy donors prior to the investigation, including a consent for publication. All procedures with zebrafish were performed in accordance with standard operating procedures approved by the Stockholm Ethical Board for Animal Experiments (permit number 13063-2017).
Availability of Data and Materials
Genetic testing.
Target sequencing of 108 cardiomyopathy-associated genes ( Supplementary Material, Table S1 ) and WES were performed as previously described ( Kostareva et al., 2016 ; Kiselev et al., 2018 ) using a targeted panel of 108 cardiomyopathy-associated genes ( Supplementary Material, Table S1 ). For MYOF gene, the {"type":"entrez-nucleotide","attrs":{"text":"NM_133337","term_id":"1676439709","term_text":"NM_133337"}} NM_133337 reference sequence was used. After target and WES, variant classification was performed according to the guidelines from the American College of Medical Genetics ( Richards et al., 2015 ).
Skeletal Muscle Mesenchymal Progenitor Cell Purification and Separation
A muscle biopsy taken from the patient’s m. deltoideus was used for morphological examination and skeletal muscle mesenchymal progenitor cell (SM-MPC) isolation. Control cells were obtained from the hip muscles of healthy donors (n = 13). Skeletal muscle mesenchymal progenitor cells were isolated enzymatically, using an adaptation of published protocols ( Danoviz and Yablonka-Reuveni, 2012 ; Smolina et al., 2015 ). In brief, muscle tissue was placed into enzyme solution, mechanically disrupted with scissors and digested for 60 min at 37 C in 5 ml filtered 0.1% collagenase I (C0130, Sigma, Germany). To remove collagenase and cell debris after digestion, the cell suspension was centrifuged for 5 min at 1,000× g and the supernatant containing enzyme solution was discarded. To release stem cells from the fibers, the pellet was resuspended using sterile pipette tips in 2.5 ml of washing media (DMEM supplemented with 10% horse serum; Gibco, USA). After the resuspension, the fibers were let to settle for 5 min and then the supernatant containing stem cells was replaced to a fresh tube. To increase yield, this step was repeated twice. The double-collected supernatant was filtered through a 40-μm nylon cell strainer and centrifuged for 10 min at 1,000 × g ; the resultant supernatant was discarded and the pellet of cells was placed in proliferation media (DMEM supplemented with 10% FCS) on cell culture dishes and cultured until 80% confluence. Cell staining and Western blot analysis were performed using anti-myoferlin antibody (Abcam 76746). MYOF protein expression levels were quantified as relative densitometric units normalized to Ponceau S staining. Densitometry was performed in NIH ImageJ software (USA). Aggregates area after immunocytochemistry using anti-MYOF antibody was measured using ZEN software (Carl Zeiss, Germany). At least four fields for each condition were analyzed. Distribution of aggregate size was analyzed using R-Studio version 1.0.153 with R version 3.0.1. Statistical analysis was performed using GraphPad Prism version 5.00 for Windows (GraphPad Software, www.graphpad.com ). For comparison of two groups, the Mann–Whitney test was used. P < 0.05 was considered statistically significant.
Flow Cytometry Analysis
The myogenic nature of purified cells was evaluated by flow cytometry analysis performed on CytoFLEX (Beckman Coulter) as previously described ( Awaya et al., 2012 ; Lapan and Gussoni, 2012 ); the following panel of antibodies was used to determine immunophenotype: anti-CD56 PC7 (Beckman Coulter, USA, {"type":"entrez-protein","attrs":{"text":"A21692","term_id":"90395","term_text":"pir||A21692"}} A21692 ), anti-CD146 PE (Beckman Coulter, USA, A07483), anti-CD166 PE (Beckman Coulter, USA, A22361), anti-CD73 PE (BD Pharmingen, USA, 550257), anti-CD105 APC (R&D Systems, USA, FAB1097A-100), and anti-CD45 PC5 (Beckman Coulter, USA, A07785). Data were analyzed using the CytExpert 2.0 (Beckman Coulter). The phenotypic characteristics of the obtained cells are illustrated in the Supplementary Material, Figure S2A .
Differentiation Protocols
Myogenic differentiation of SM-MPC was induced by replacement of proliferation media with differentiation media: DMEM supplemented with 2% of horse serum. Cell cultures were taken for further analysis on seventh day after induction when myotubes were clearly visualized ( Supplementary Material, Figure S2B ).
RNA Isolation and Analysis
Total RNA was isolated with Trizol reagent (Sigma, USA) from SM-MPC as well as from the patient’s and the donor’s tissue biopsies. After isolation, RNA was quantified using Qubit 2.0 fluorometer (Life Technologies, Invitrogen division, Darmstadt, Germany) with Qubit RNA HS Assay Kit and Nanodrop 1000 spectrophotometer (Thermo Scientific, Wilmington, DE, USA). Additionally, total RNA was quantified using Bioanalyser 2100 (Agilent Technologies, Palo Alto, CA) RNA Nano kit; RINs for all samples were in the range >9. Libraries for RNA sequencing were prepared using TruSeq Stranded mRNA kit (Illumina, USA), according to the manufacturer’s recommendation. Single-read sequencing was performed on Illumina HiSeq 2500 instrument with TruSeq SR Cluster Kit v3 cBot, HS, and TruSeq SBS Kit v3 (50-cycles). Bcl files were processed to .fastq files using bcl2fastq Conversion Software v1.8.4 (Illumina). A quantitative evaluation of gene expression was performed with qPCR mix-HS SYBR+ROX (Evrogen, cat.no. PK156, Russia). Q-PCR data are presented as arbitrary units of mRNA expression normalized to GAPDH expression and to expression levels in reference sample. Sequences for Q-PCR primers can be found in Supplementary Material, Table S2 .
RNA-seq data were quantified using RSEM with GENCODE annotation ( Li and Dewey, 2011 ) and deposited in GEO repository ( {"type":"entrez-geo","attrs":{"text":"GSE119027","term_id":"119027"}} GSE119027 ). For pathways analysis, fgsea package was used (biorxiv, doi: https://doi.org/10.1101/060012 ) with MSigDB and Reactome collections; genes were ranked by log2-fold-change ( Liberzon et al., 2015 ; Fabregat et al., 2018 ). The inflammation expression pattern was confirmed by comparison to {"type":"entrez-geo","attrs":{"text":"GSE26852","term_id":"26852"}} GSE26852 dataset (Gene expression analysis of facioscapulohumeral muscular dystrophy muscle with different MRI pattern) ( Tasca et al., 2012 ).
Zebrafish Maintenance and Knockdown Studies
Adult zebrafish were maintained on a 14 h light/10 h dark cycle at the Karolinska Institute zebrafish core facility. Knockdown of endogenous myof was achieved by injecting 0.5-mM solution of a splice-modifying morpholino predicted to block intron 5 splicing and introduce a premature stop codon (5’ GCTGATCACACCGAAAAGTAAATGA 3’). Embryos expressing EGFP were positively selected at 24 hours post fertilization (hpf) and fixed at 48 hpf in 4% paraformaldehyde overnight at 4°C. Permeabilization was performed by incubation with 100% acetone for 20 min at −80°C, and immunolabelling was performed as previously described ( Kiselev et al., 2018 ). Antibodies used were anti-GFP (ab290, AbCam), anti-Dystrophin and anti-Dystroglycan (7A10 and 7D11, D.S.H.B)., and anti-Rabbit Alexa488 and anti-Mouse Alexa594 (Life Technologies). Nuclear staining was performed by incubation with DAPI (4′,6-diamidino-2-phenylindole) at a final concentration of 10 µg/ml. Imaging was performed in 7–12 embryos per staining and experimental condition from at least two separate rounds of injections. Electron microscopy was performed as previously published ( Ebarasi et al., 2009 ).
A female patient experienced a first episode of palpitation at 44 years of age and 5 years later noticed a weakness of the limb girdle muscle and inability to rise from a chair without the help of her hands. At the age of 53, she was diagnosed with sick sinus syndrome, sinoatrial block II, incessant focal atrial tachycardia, anterior left bundle branch block, and hemodynamically tolerated, sustained polymorphic ventricular tachycardia. An elevated serum CK level (863 U/L, normal range 29–200 U/L), as well as LDH (305 U/L, normal range 125–243 U/L) and myoglobin (233 ng/ml, normal range 0.0–106.0 ng/ml) was noticed. Biochemical level of Troponin I was within the normal range (0.37 ng/ml with reference interval 0–400 ng/ml), while CK-M level was slightly elevated (36.6 U/L with reference interval 0–24 U/L). Neurological examination confirmed reduced muscle strength, which in combination with morphological examination of muscle tissue resulted in the diagnosis of limb-girdle muscular dystrophy. Biochemical tests for anti-muscle antibodies and polymyositis-associated antibodies were negative. The cranial muscles, mimic, chewing, bulbar, and respiratory muscles were intact; muscle weakness was detected in the pelvic, proximal lower limb and upper girdle, and spine muscles. No muscle contracture, myotonia, or ataxia was observed; innervation of pelvic organs was intact and respiratory function assessment demonstrated intact respiratory muscles. Electromyography confirmed myopathic pattern and muscle CT of lower limbs detected symmetrical muscle wasting and decrease of muscle density due to fat substitution most prominent in the anterior and posterior hip muscles and gluteal muscles. Echocardiography demonstrated both left and right chambers enlargement, myocardial hypertrophy, hypokinetic interventricular septum, and basal segments of left ventricular posterior wall with preserved ejection fraction (55%). Notably, right ventricular and right atrial dimensions were increased in combination with thickening of the right ventricular myocardial wall ( Supplementary Material, Table S3 ). Cardiac MRI demonstrated late gadolinium enchantment in the left ventricle, enlarged atria (left atrium dimension 52×39 mm and right atrium dimension 58×64 mm), and moderately enlarged left ventricle (end-diastolic dimension—46 mm) with moderate decrease in contractility. No signs of ischemic heart disease, hypertension, or chronic lung disease were registered. The family history reported no cardiac nor skeletal muscle disorders, but parental DNA was not available for genetic analysis and the relatives were not available for a detailed clinical investigation and phenotyping.
Primary genetic screening using a targeted panel of 108 cardiomyopathy-associated genes, including those associated with neuromuscular disorders, did not identify any likely disease-causing variants. Subsequently, WES was performed and after filtering, a list of non-synonymous and potentially affecting protein function variants was further analyzed ( Supplementary Material, Table S4 ). Data processing, variant filtering, and assessment are described in Supplementary Material (Figure S1 , Filtering Strategy). This analysis identified two neighboring cis variants in MYOF (MYOF chr10:95066186-95242074, GRCh37.p13) resulting in a frameshift mutation ( {"type":"entrez-nucleotide","attrs":{"text":"NM_133337","term_id":"1676439709","term_text":"NM_133337"}} NM_133337 :c.[2576delG; 2575G>C], p.G859QfsTer8) ( Figure 1A ). The variant is classified as likely pathogenic according to the 2018 American College of Medical Genetics and Genomics (ACMG) guidelines (criteria codes PS3, PM2, PM4).

Genetic and morphological data on MYOF loss of function variant. (A) A heterozygous variant p.G859QfsTer8 resulted from cis-change of 2-neighbor mutated nucleotides (aligned RAW-reads and allele cloning of PCR products). (B) Morphological examination performed on m. deltoideus , following hematoxylin-eosin staining. Variation in fiber size, nuclear centralization, and fat accumulation along with inflammatory cells infiltration are often observed. (C) Increased mRNA expression of MYOF, embryonic (MYH3), and developmental (MYH8) myosins in the patient’s skeletal muscle tissue compared to donor samples (n = 13). The patient’s sample is represented in red; donor samples in green and black. (D) Decrease by 65% of the MYOF level in the patient’s SM-MPC when compared with healthy control. The detected fragments correspond to full size MYOF transcript (234 kDa) and suspected cleavage products (74 and 160 kDa) [30]. (E) Immunostaining of SM-MPC: low intensity of MYOF staining in the patient’s cells, patchy aggregate distribution, and nuclear intensification of the signal compared to control cells (×100). Histogram demonstrates the distribution of larger aggregates in the patient’s cells. (F) Quantitative characterization of MYOF aggregates. Representative frequency distribution of aggregate area in the control and patient SM-MPC and comparison of the mean aggregate area values for the control and the patient. The graph is presented as Tukey box-plot.
Morphological examination of the patient’s skeletal muscle demonstrated fiber size variation, splitting, and nuclear centralization in more than 30% of fibers in combination with fat infiltration and fibrosis ( Figure 1B ). Signs of muscle degeneration/regeneration and fatty infiltration were observed. An upregulation of MYOF mRNA expression in the patient’s skeletal muscle tissue was detected compared to healthy donors. This was accompanied by increased expression in skeletal muscle biopsy of embryonic and developmental myosins MYH3 and MYH8 , while expression of adult myosin forms ( MYH1 , MYH4 , MYH7 ) was within the normal range ( Figure 1C ). In contrast, Western blot analysis performed on the patient’s SM-MPC confirmed a decreased expression (65%) of MYOF in the patient’s SM-MPC with almost complete absence of the low molecular weight isoform ( Figure 1D ). Staining of the patient’s SM-MPC with anti-MYOF antibody further confirmed a decreased staining intensity and formation of intracellular aggregates of larger diameter and greater number compared to control cells ( Figure 1E, F ). Notably, in a primary culture of the patient’s SM-MPC, MYOF was often localized in the nuclear area, which was not observed in control cells.
RNA sequencing was performed on the patient’s SM-MPC and differentiated myotubes in order to analyze the effect of the MYOF which is likely pathogenic variant on intracellular pathways. The analysis revealed dysregulation of multiple pathways including inflammation and mitochondrial metabolism. Myogenesis was activated in both patient and control samples after differentiation, as seen by MYH1 , MYH2 , MYL2 , TNNC2 , and CASQ2 expression levels ( Figure 2A, B ). Consistently with the Western blot analysis, following RNA sequencing, MYOF mRNA showed twofold down-regulation in patient undifferentiated cells compared to donor cells [56.1 transcripts per million (TPM) compared to 112.25 TPM, Supplementary Material, Table S5 ]. However, a comparison of the patient’s samples with the corresponding control samples after differentiation showed a down-regulation of the myogenesis pathway genes in the patient’s samples ( Figure 2C ). This was accompanied by activation of inflammatory and hypoxia-induced genes ( Figure 2D ). Additionally, mitochondrial metabolism pathways (e.g., SLC25A4 , ATP5D , NQO2 , NDUFB7 ) and several inflammation pathways including IFNγ response (e.g., CFB , IL6 , IFI30 , ICAM1 , IFI27 , CCL2 ) were up-regulated in the patient’s SM-MPC ( Figure 2E ).

Gene set enrichment analysis of donor-derived and patient-derived SM-MPC. (A, B) Differentiated cells are highly enriched in hallmark myogenesis pathway both in the donor (A) and the patient’s (B) cells. (C) Myogenesis differentiation is decreased in the patient’s cells compared to donor cells. (D) Pathways from MSigDB hallmark database showing significant enrichment in donor versus patient comparison. Pathways are ordered according to normalized enrichment score (NES). Positive NES values correspond to pathways upregulated in the patient’s cells and negative values correspond to downregulated pathways. (E) Individual enrichment plots for interferon gamma response and oxidative phosphorylation pathways.
To further elucidate the role of MYOF in myogenesis, we performed knockdown experiments in the zebrafish embryo using a splice-target morpholino ( myof -MO). This MO binds to the intron 5–exon 6 boundary and is predicted to block the splicing of the intron ( Figure 3A ) and target the mRNA for degradation by non-sense mediated decay ( Lykke-Andersen and Jensen, 2015 ). RT-PCR analysis of myof -MO injected embryos showed a decrease in the WT myof mRNA when compared with uninjected siblings ( Figure 3A ), suggesting retention of intron 5. Loss of Myof in zebrafish resulted in a myopathy-like phenotype, including wavy fibers and disrupted Myosin patterning (in red, Figure 3B ). Other members of the Ferlin family of proteins are localized at the Z-disc and myosepta, with knockdown of dysferlin reported to result in myosepta disruption ( Kawahara et al., 2011 ). Since the myosepta of myof -MO injected embryos appeared to be wider than in control siblings, we immunolabelled for proteins of the myosepta, Dystrophin and Dystroglycan, and found a wider and less dense distribution of these proteins, suggesting myosepta disruption (in green, Figure 3B ). Analysis of the myofibers using electron microscopy showed a general disruption of the myofibrils ( Figure 3C4; arrows, C5 and C6 ), with abnormally organized and misshaped mitochondria (arrowheads, Figure 3C5 ), Z-disc streaming (arrowheads, Figure 3C6 ), and widening of triads.

Loss of Myof affects the skeletal muscle of zebrafish embryos. (A) myof knockdown was achieved by injecting a splice-target morpholino ( myof -MO). (B) Loss of Myof results in wavy and abnormally patterned myofibers (Myosin, red), and disruption of the myosepta, seen by a decreased intensity in the staining and increased width (Dystrophin and Dystroglycan, green). (C) Analysis of the myofiber ultrastructure by electron microscopy shows disruption of myofiber structure (C4) and their sarcomeres (arrows, C5, C6), abnormally shaped and distributed mitochondria (arrowheads, C5), when compared to control mitochondria (arrowheads, C1), and Z-disc thickening (arrowheads, C6).
Here we have presented a clinical case of cardiomyopathy associated with limb-girdle type muscular dystrophy where WES strategy allowed the identification of a truncating variant in myoferlin ( MYOF ), c.[2576delG; 2575G>C], p.G859QfsTer8. The clinical phenotype in combination with in vitro and in vivo studies allowed us to classify the detected MYOF variant as likely pathogenic and to link for the first time MYOF with a human genetic disease.
MYOF is mainly expressed in heart and skeletal muscle where, similar to DYSF , it participates in membrane repair and muscle regeneration after injury through the activation of the NFAT-dependent promoter ( Demonbreun et al., 2010a ; Han, 2011 ). It is also involved in myoblast and satellite cell fusion into mature myofibers during embryo development and postnatally ( Doherty et al., 2005 ). Moreover, MYOF modulates inflammatory and growth response by participation in IGF1R, EGFR, and VEGFR2 trafficking and recycling ( Demonbreun et al., 2010b ; Turtoi et al., 2013 ). The recent implication in exosome maturation made it an important protein for cell–cell communication both in myogenesis and tumorigenesis ( Forterre et al., 2014 ; Blomme et al., 2016 ). In spite of their structural similarity and tissue-specific expression, MYOF and DYSF do not present with overlapping functions. In fact, they have distinct time-dependent expression profiles as well as slightly distinct subcellular localization and Ca 2+ -dependent cleavage sites ( Piper et al., 2017 ). In contrast to DYSF, Ca 2+ -dependent MYOF cleavage occurs in resting cells leading to constant basal release of MYOF cleavage products ( Redpath et al., 2014 ; Piper et al., 2017 ). The absence of overlapping function between MYOF and DYSF is further supported by the fact that MYOF can partly compensate for DYSF loss in vitro , while having a limited ability to compensate DYSF loss in vivo ( Inoue et al., 2006 ; Lostal et al., 2012 ).
The described clinical phenotype in combination with morphological data and CK elevation allowed us to suggest MYOF as a strong candidate for being a disease-causing gene. A combination of in vitro and in vivo studies allowed us to classify the identified variant as likely pathogenic and for the first time link MYOF to human inherited disease. Of note, we observed a much stronger MYOF staining in the nucleus of patient-derived muscle stromal cells compared to control cells—a phenomenon previously reported by Davis et al. (2000) . This, in part, may be attributed to the role of MYOF in chaperoning phosphorylated STAT3 into the nucleus under IL-6 induced signaling ( Yadav et al., 2017 ), and supported by the activation of the IFNγ-proinflammatory pathway and IL-6 signaling in MYOF -mutant SM-MPC during differentiation. Together, the RNA signature of proinflammatory pathway activation and downregulation of myogenesis in MYOF -mutant cells confirms the role of MYOF in myogenesis and modulation of inflammatory signaling. The inflammation expression pattern was confirmed by comparison to {"type":"entrez-geo","attrs":{"text":"GSE26852","term_id":"26852"}} GSE26852 dataset (gene expression analysis of facioscapulohumeral muscular dystrophy muscle with different MRI pattern) ( Tasca et al., 2012 ). There, genes upregulated in T2-short tau inversion recovery positive samples corresponding to more inflammation muscle phenotype (T2-STIR+) significantly overlap with genes upregulated in the patient’s samples (p-value of GSEA test < 1e−5). The increase in embryonic and developmental regeneration markers such as MYH3 and MYH8 in the patient’s muscle tissue supports the ongoing inflammation-regeneration process. This, in part, can explain the activation of MYOF expression in the adult patient’s tissue—a phenomenon not observed in healthy donor skeletal muscle due to very low MYOF expression in adult matured myofibers ( Schiaffino et al., 2015 ). However, on a single cell level in vitro MYOF expression in the patient’s SM-MPC was decreased compared to control cells suggesting that the p.G859QfsTer8 variant causes loss of MYOF function.
Loss of Myof in zebrafish results not only in a myopathy-like phenotype and myosepta disruption, but also abnormal sarcomeric organization, disrupted myosin patterning, mitochondria abnormalities, and Z-disc streaming. Other members of the Ferlin family are localized at the Z-disc and myosepta, with knockdown of dysferlin reported to result in myosepta disruption ( Kawahara et al., 2011 ). Given the lack of a working antibody against Myof in zebrafish, we were not able to verify the localization in the zebrafish skeletal muscle. Nevertheless, we suggest that Myof may present the same distribution as its family members, supported by the resulting phenotype when myof is knocked down. This is the first report on ultrastructural sarcomeric and Z-line abnormalities induced by MYOF loss, however, well in line with the previous observations on the role of MYOF in T-tubular system organization and remodeling ( Demonbreun et al., 2014 ). Disorganization of T-tubular system and triads can, in part, contribute to proarrhythmic phenotypes observed in the patient described here. Previous reports have showed a conservation of the DysF domains in the Ferlin family, and their importance for normal protein function and implication in disease ( Therrien et al., 2006 ; Patel et al., 2008 ). We therefore suggest that both loss and truncation of MYOF may result in similar phenotypes, which is shown here in the in vitro experiments. It is, however, not entirely clear whether disease-causing variants in MYOF act as gain-of-function or haploinsufficient variants.
A disease-causative role of the identified MYOF loss of function variant is supported by analyses of the patient’s satellite cells, RNA sequencing data, morphological and immunohistochemical studies, as well as recapitulation of the muscle phenotype in zebrafish experiments. The clinical presentation resembles that observed when dysferlin is knocked down ( Kawahara et al., 2011 ). In aggregate, our study provides the first evidence of MYOF gene as being associated with human disease phenotype and broadens the field for further research of the Ferlin protein family in connection to disorders of cardiac and skeletal muscle. The identification of singe allele damage leading to approximately 40% reduction in RNA and protein level in association with a late-onset clinical phenotype suggests an autosomal dominant inheritance pattern. However, as for many other neuromuscular disorder-associated genes, such as LMNA , DYSF , TTN , MYH7 , and COL6A2 , both dominant and recessive mechanisms are possible, depending on the number of alleles damaged and the effects on protein levels ( Bonne et al., 2017 ). Analysis of parental DNA and detailed phenotyping of close relatives would shed more light on the specific mechanism involved in the patient reported here, and the unavailability of these data represents a limitation of the current study.
Our data indicate that the identified MYOF variant acts as a loss of function allele and, thus, can potentially lead to deleterious functional effects due to decreased RNA and protein levels. However, more than 70 loss of function MYOF variants have been reported in public databases such as ExAC and gnomAD. Some of these affect alternative non-coding transcripts and no phenotype information is provided. Notably, only about 30% of the individuals in those datasets are expected to be older than the patient presented here. Even so, incomplete or low penetrance of MYOF associated muscle disease remains a possibility, and further studies are necessary to determine the functional and clinical effects of loss of MYOF .
Of note, we observed a stronger MYOF staining in the nucleus of the patient-derived SM-MPC to control cells previously reported by Davis et al. (2000) . This may, in part, be attributed to the role of MYOF in chaperoning phosphorylated STAT3 into the nucleus under IL-6 induced signaling ( Yadav et al., 2017 ), which was supported even further by our finding of activation of the IFNγ-proinflammatory pathway and IL-6 signaling in MYOF -mutant cells during differentiation. Together, the RNA signature of proinflammatory pathway activation and downregulation of myogenesis in MYOF -mutant cells are in line with previously proposed roles of MYOF in myogenesis and modulation of inflammation signaling ( Demonbreun et al., 2010 ; Turtoi et al., 2013 ; Forterre et al., 2014 ).
In summary, we present a first report of a loss of function MYOF variant in a patient with cardiomyopathy and limb-girdle type muscular dystrophy phenotype. The disease-causative role of the identified variant is supported by morphological and molecular studies, RNA sequencing data, as well as recapitulation of muscle phenotype in a zebrafish model. Our study provides the first evidence of the MYOF gene being associated with a human disease phenotype and broadens the field for further research on Ferlin protein family in connection with disorders of cardiac and skeletal muscles.
Ethics Statement
The study was performed according to the Declaration of Helsinki, and approval was obtained from the Ethical Review Boards of Karolinska Institute and Almazov National Medical Research Centre, approval number 2016/54. Written informed consent was obtained from both the study subject and healthy donors prior to the investigation, including a consent for publication. All procedures with zebrafish were performed in accordance with standard operating procedures approved by the Stockholm Ethical Board for Animal Experiments (permit number 13063-2017).
Author Contributions
AKi, RV, and AKn contributed to the conception and design of the study, analysis, and interpretation of the data and drafting of the manuscript. TS and AL made contributions to the conception and design of the study and revision of the manuscript critically. AKo contributed to the study concept and research design and wrote the manuscript. GS, DR, LM, and EM took part in the analysis and interpretation of the data and have been involved in revising the manuscript critically. AS, AP, and JJ performed bioinformatics analysis. AKh, RV, YF, RD, KS, and NS conducted the experiments and performed the analysis and interpretation of the data. All authors have read and approved the final version of the manuscript.
This work was supported by Russian Scientific Foundation (14-15-00745-П) for study design, data collection and analysis, sequencing and morphological studies, cell culturing, and expression analysis; Swedish Society for Medical Research and Swedish Research Council (2013-2603; 2017-02936), ALF funding (20140240, 20170831), Stiftelsen Frimurare Foundation, Promobilia for zebrafish model design and analysis and confocal imaging, Government of Russian Federation (08-08) for bioinformatics and statistical analysis.
Conflict of Interest Statement
The authors declare that the research was conducted in the absence of any commercial or financial relationships that could be construed as a potential conflict of interest.
Abbreviations
LGMD2B, limb-girdle muscle dystrophy 2B; WES, whole exome sequencing; FCS, fetal calf serum; DMEM, Dulbecco medium Eagle’s modified; MRI, magnetic resonance investigation; CK, creatine kinase; LDH, lactate dehydrogenase; SM-MPC, skeletal muscle mesenchymal progenitor cells; MO, morpholino; TPM, transcripts per million; T2-STIR+, T2-short tau inversion recovery positive samples; ExAC, The Exome Aggregation Consortium; gnomAD, The Genome Aggregation Database.
Acknowledgments
We thank the investigators for A4.1025 (H. M. Blau) and 7A10 and 7D11 (G. E. Morris), obtained from the Developmental Studies Hybridoma Bank (developed under the auspices of the NICHD and maintained by the University of Iowa, Department of Biology, Iowa City, IA). Sequencing was performed at Resource Centre «Biobank» and Research Resource Center of Molecular and Cell Technologies of St. Petersburg State University. We thank the Zebrafish Core Facility at Karolinska Institutet for zebrafish maintenance.
Supplementary Material
The Supplementary Material for this article can be found online at: https://www.frontiersin.org/articles/10.3389/fgene.2019.00608/full#supplementary-material
- Awaya T., Kato T., Mizuno Y., Chang H., Niwa A., Umeda K., et al. (2012). Selective development of myogenic mesenchymal cells from human embryonic and induced pluripotent stem cells . PLoS One 7 , e51638. 10.1371/journal.pone.0051638 [ PMC free article ] [ PubMed ] [ CrossRef ] [ Google Scholar ]
- Blomme A., Fahmy K., Peulen O., Costanza B., Fontaine M., Struman I., et al. (2016). Myoferlin is a novel exosomal protein and functional regulator of cancer-derived exosomes . Oncotarget 7 , 83669–83683. 10.18632/oncotarget.13276 [ PMC free article ] [ PubMed ] [ CrossRef ] [ Google Scholar ]
- Bondue A., Arbustini E., Bianco A., Ciccarelli M., Dawson D., De Rosa M., et al. (2018). Complex roads from genotype to phenotype in dilated cardiomyopathy: scientific update from the Working Group of Myocardial Function of the European Society of Cardiology . Cardiovasc. Res. 114 , 1287–1303. 10.1093/cvr/cvy122 [ PMC free article ] [ PubMed ] [ CrossRef ] [ Google Scholar ]
- Bonne G., Rivier F., Hamroun D. (2017). The 2018 version of the gene table of monogenic neuromuscular disorders (nuclear genome) . Neuromuscul. Disord. 27 , 1152–1183. 10.1016/j.nmd.2017.10.005 [ PubMed ] [ CrossRef ] [ Google Scholar ]
- Choi E. R., Park S. J., Choe Y. H., Ryu D. R., Chang S. A., Choi J. O., et al. (2010). Early detection of cardiac involvement in Miyoshi myopathy: 2D strain echocardiography and late gadolinium enhancement cardiovascular magnetic resonance . J. Cardiovasc. Magn. Reson. 12 , 31. 10.1186/1532-429X-12-31 [ PMC free article ] [ PubMed ] [ CrossRef ] [ Google Scholar ]
- Danoviz M. E., Yablonka-Reuveni Z. (2012). Skeletal muscle satellite cells: background and methods for isolation and analysis in a primary culture system . Methods Mol. Biol. 798 , 21–52. 10.1007/978-1-61779-343-1_2 [ PMC free article ] [ PubMed ] [ CrossRef ] [ Google Scholar ]
- Davis D. B., Delmonte A. J., Ly C. T., McNally E. M. (2000). Myoferlin, a candidate gene and potential modifier of muscular dystrophy . Hum. Mol. Genet. 9 , 217–226. 10.1093/hmg/9.2.217 [ PubMed ] [ CrossRef ] [ Google Scholar ]
- Demonbreun A. R., Lapidos K. A., Heretis K., Levin S., Dale R., Pytel P., et al. (2010. a). Myoferlin regulation by NFAT in muscle injury, regeneration and repair . J. Cell. Sci. 123 , 2413–2422. 10.1242/jcs.065375 [ PMC free article ] [ PubMed ] [ CrossRef ] [ Google Scholar ]
- Demonbreun A. R., Posey A. D., Heretis K., Swaggart K. A., Earley J. U., Pytel P., et al. (2010. b). Myoferlin is required for insulin-like growth factor response and muscle growth . FASEB J. 24 , 1284–1295. 10.1096/fj.09-136309 [ PMC free article ] [ PubMed ] [ CrossRef ] [ Google Scholar ]
- Demonbreun A. R., Rossi A. E., Alvarez M. G., Swanson K. E., Deveaux H. K., Earley J. U., et al. (2014). Dysferlin and myoferlin regulate transverse tubule formation and glycerol sensitivity . Am. J. Pathol. 184 , 248–259. 10.1016/j.ajpath.2013.09.009 [ PMC free article ] [ PubMed ] [ CrossRef ] [ Google Scholar ]
- Doherty K. R., Cave A., Davis D. B., Delmonte A. J., Posey A., Earley J. U., et al. (2005). Normal myoblast fusion requires myoferlin . Development 132 , 5565–5575. 10.1242/dev.02155 [ PMC free article ] [ PubMed ] [ CrossRef ] [ Google Scholar ]
- Ebarasi L., He L., Hultenby K., Takemoto M., Betsholtz C., Tryggvason K., et al. (2009). Reverse genetic screen in the zebrafish identifies crb2b as a regulator of the glomerular filtration barrier . Dev. Biol. 334 , 1–9. 10.1016/j.ydbio.2009.04.017 [ PubMed ] [ CrossRef ] [ Google Scholar ]
- Fabregat A., Jupe S., Matthews L., Sidiropoulos K., Gillespie M., Garapati P., et al. (2018). The Reactome pathway knowledgebase . Nucleic Acids Res. 46 , D649–D655. 10.1093/nar/gkt1102 [ PMC free article ] [ PubMed ] [ CrossRef ] [ Google Scholar ]
- Forterre A., Jalabert A., Berger E., Baudet M., Chikh K., Errazuriz E., et al. (2014). Proteomic analysis of C2C12 myoblast and myotube exosome-like vesicles: a new paradigm for myoblast-myotube cross talk ? PLoS One 9 , e84153. 10.1371/journal.pone.0084153 [ PMC free article ] [ PubMed ] [ CrossRef ] [ Google Scholar ]
- Han R., Bansal D., Miyake K., Muniz V. P., Weiss R. M., McNeil P. L., et al. (2007). Dysferlin-mediated membrane repair protects the heart from stress-induced left ventricular injury . J. Clin. Invest. 117 , 1805–1813. 10.1172/JCI30848 [ PMC free article ] [ PubMed ] [ CrossRef ] [ Google Scholar ]
- Han R. (2011). Muscle membrane repair and inflammatory attack in dysferlinopathy . Skelet. Muscle 1 , 10. 10.1186/2044-5040-1-10 [ PMC free article ] [ PubMed ] [ CrossRef ] [ Google Scholar ]
- Inoue M., Wakayama Y., Kojima H., Shibuya S., Jimi T., Oniki H., et al. (2006). Expression of myoferlin in skeletal muscles of patients with dysferlinopathy . Tohoku J. Exp. Med. 209 , 109–116. 10.1620/tjem.209.109 [ PubMed ] [ CrossRef ] [ Google Scholar ]
- Kawahara G., Serafini P. R., Myers J. A., Alexander M. S., Kunkel L. M. (2011). Characterization of zebrafish dysferlin by morpholino knockdown . Biochem. Biophys. Res. Commun. 413 , 358–363. 10.1016/j.bbrc.2011.08.105 [ PMC free article ] [ PubMed ] [ CrossRef ] [ Google Scholar ]
- Kiselev A., Vaz R., Knyazeva A., Khudiakov A., Tarnovskaya S., Liu J., et al. (2018). De novo mutations in FLNC leading to early-onset restrictive cardiomyopathy and congenital myopathy . Hum. Mutat. 39 , 1161–1172. 10.1002/humu.23559 [ PubMed ] [ CrossRef ] [ Google Scholar ]
- Kostareva A., Kiselev A., Gudkova A., Frishman G., Ruepp A., Frishman D., et al. (2016). Genetic spectrum of idiopathic restrictive cardiomyopathy uncovered by next-generation sequencing . PLoS One 11 , e0163362. 10.1371/journal.pone.0163362 [ PMC free article ] [ PubMed ] [ CrossRef ] [ Google Scholar ]
- Lapan A. D., Gussoni E. (2012). Isolation and characterization of human fetal myoblasts . Methods Mol. Biol. 798 , 3–19. 10.1007/978-1-61779-343-1_1 [ PMC free article ] [ PubMed ] [ CrossRef ] [ Google Scholar ]
- Li B., Dewey C. N. (2011). RSEM: accurate transcript quantification from RNA-Seq data with or without a reference genome . BMC Bioinformatics 12 , 323. 10.1186/1471-2105-12-323 [ PMC free article ] [ PubMed ] [ CrossRef ] [ Google Scholar ]
- Liberzon A., Birger C., Thorvaldsdottir H., Ghandi M., Mesirov J. P., Tamayo P. (2015). The Molecular signatures database (MSigDB) hallmark gene set collection . Cell Syst. 1 , 417–425. 10.1016/j.cels.2015.12.004 [ PMC free article ] [ PubMed ] [ CrossRef ] [ Google Scholar ]
- Lostal W., Bartoli M., Roudaut C., Bourg N., Krahn M., Pryadkina M., et al. (2012). Lack of correlation between outcomes of membrane repair assay and correction of dystrophic changes in experimental therapeutic strategy in dysferlinopathy . PLoS One 7 , e38036. 10.1371/journal.pone.0038036 [ PMC free article ] [ PubMed ] [ CrossRef ] [ Google Scholar ]
- Lykke-Andersen S., Jensen T. H. (2015). Nonsense-mediated mRNA decay: an intricate machinery that shapes transcriptomes . Nat. Rev. Mol. Cell Biol. 16 , 665–677. 10.1038/nrm4063 [ PubMed ] [ CrossRef ] [ Google Scholar ]
- Nishikawa A., Mori-Yoshimura M., Segawa K., Hayashi Y. K., Takahashi T., Saito Y., et al. (2016). Respiratory and cardiac function in Japanese patients with dysferlinopathy . Muscle Nerve 53 , 394–401. 10.1002/mus.24741 [ PubMed ] [ CrossRef ] [ Google Scholar ]
- Patel P., Harris R., Geddes S. M., Strehle E. M., Watson J. D., Bashir R., et al. (2008). Solution structure inner DysF domain of myoferlin and implications forlimb girdle musculardystrophy type 2b . J. Mol. Biol. 379 , 981–990. 10.1016/j.jmb.2008.04.046 [ PubMed ] [ CrossRef ] [ Google Scholar ]
- Piper A. K., Ross S. E., Redpath G. M., Lemckert F. A., Woolger N., Bournazos A., et al. (2017). Enzymatic cleavage of myoferlin releases a dual C2-domain module linked to ERK signalling . Cell. Signal. 33 , 30–40. 10.1016/j.cellsig.2017.02.009 [ PMC free article ] [ PubMed ] [ CrossRef ] [ Google Scholar ]
- Posey A. D., Jr., Demonbreun A., McNally E. M. (2011). Ferlin proteins in myoblast fusion and muscle growth . Curr. Top. Dev. Biol. 96 , 203–230. 10.1016/B978-0-12-385940-2.00008-5 [ PMC free article ] [ PubMed ] [ CrossRef ] [ Google Scholar ]
- Redpath G. M., Woolger N., Piper A. K., Lemckert F. A., Lek A., Greer P. A., et al. (2014). Calpain cleavage within dysferlin exon 40a releases a synaptotagmin-like module for membrane repair . Mol. Biol. Cell 25 , 3037–3048. 10.1091/mbc.e14-04-0947 [ PMC free article ] [ PubMed ] [ CrossRef ] [ Google Scholar ]
- Richards S., Aziz N., Bale S., Bick D., Das S., Gastier-Foster J., et al. (2015). Standards and guidelines for the interpretation of sequence variants: a joint consensus recommendation of the American College of Medical Genetics and Genomics and the Association for Molecular Pathology . Genet. Med. 17 , 405–424. 10.1038/gim.2015.30 [ PMC free article ] [ PubMed ] [ CrossRef ] [ Google Scholar ]
- Schiaffino S., Rossi A. C., Smerdu V., Leinwand L. A., Reggiani C. (2015). Developmental myosins: expression patterns and functional significance . Skelet. Muscle 5 , 22. 10.1186/s13395-015-0046-6 [ PMC free article ] [ PubMed ] [ CrossRef ] [ Google Scholar ]
- Smolina N., Kostareva A., Bruton J., Karpushev A., Sjoberg G., Sejersen T. (2015). Primary murine myotubes as a model for investigating muscular dystrophy . Biomed Res. Int. 2015 , 594751. 10.1155/2015/594751 [ PMC free article ] [ PubMed ] [ CrossRef ] [ Google Scholar ]
- Tasca G., Pescatori M., Monforte M., Mirabella M., Iannaccone E., Frusciante R., et al. (2012). Different molecular signatures in magnetic resonance imaging-staged facioscapulohumeral muscular dystrophy muscles . PLoS One 7 , e38779. 10.1371/journal.pone.0038779 [ PMC free article ] [ PubMed ] [ CrossRef ] [ Google Scholar ]
- Therrien C., Dodig D., Karpati G., Sinnreich M. (2006). Mutation impact on dysferlin inferred from database analysis and computer based structural predictions . J. Neurol. Sci. 250 , 71–78. 10.1016/j.jns.2006.07.004 [ PubMed ] [ CrossRef ] [ Google Scholar ]
- Turtoi A., Blomme A., Bellahcene A., Gilles C., Hennequiere V., Peixoto P., et al. (2013). Myoferlin is a key regulator of EGFR activity in breast cancer . Cancer Res. 73 , 5438–5448. 10.1158/0008-5472.CAN-13-1142 [ PubMed ] [ CrossRef ] [ Google Scholar ]
- Wenzel K., Geier C., Qadri F., Hubner N., Schulz H., Erdmann B., et al. (2007). Dysfunction of dysferlin-deficient hearts . J. Mol. Med. (Berl.) 85 , 1203–1214. 10.1007/s00109-007-0253-7 [ PubMed ] [ CrossRef ] [ Google Scholar ]
- Wang C., Wong J., Fung G., Shi J., Deng H., Zhang J., et al. (2015). Dysferlin deficiency confers increased susceptibility to coxsackievirus-induced cardiomyopathy . Cell Microbiol. 17 , 1423–1430. 10.1111/cmi.12473 [ PubMed ] [ CrossRef ] [ Google Scholar ]
- Yadav A., Kumar B., Lang J. C., Teknos T. N., Kumar P. (2017). A muscle-specific protein ‘myoferlin’ modulates IL-6/STAT3 signaling by chaperoning activated STAT3 to nucleus . Oncogene 36 , 6374–6382. 10.1038/onc.2017.245 [ PMC free article ] [ PubMed ] [ CrossRef ] [ Google Scholar ]
- Zhang T., Li J., He Y., Yang F., Hao Y., Jin W., et al. (2018). A small molecule targeting myoferlin exerts promising anti-tumor effects on breast cancer . Nat. Commun. 9 , 3726. 10.1038/s41467-018-06179-0 [ PMC free article ] [ PubMed ] [ CrossRef ] [ Google Scholar ]
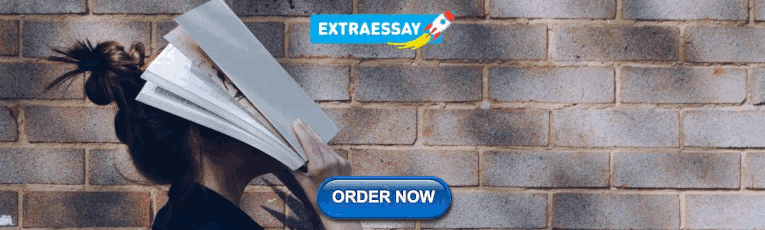
IMAGES
VIDEO
COMMENTS
The most commonly accepted method of evaluating muscle strength is the Medical Research Council Manual Muscle Testing scale. This method involves testing key muscles from the upper and lower extremities against the examiner's resistance and grading the patient's strength on a 0 to 5 scale accordingly:
The muscle scale grades muscle power on a scale of 0 to 5 in relation to the maximum expected for that muscle. In a recent comparison to an analogue scale the MRC scale is more reliable and accurate for clinical assessment in weak muscles (grades 0-3) while an analogue scale is more reliable and accurate for the assessment of stronger muscles (grades 4 and 5).
The Medical Research Council grading system has served through decades for the evaluation of muscle strength and has been recognized as a cardinal feature of daily neurological, rehabilitation and general medicine examination of patients, despite being ...
The muscle scale grades muscle power on a scale of 0 to 5 in relation to the maximum expected for that muscle. The patient's effort is graded on a scale of 0-5: Grade 5: Muscle contracts normally against full resistance. Grade 4: Muscle strength is reduced but muscle contraction can still move joint against resistance.
The Medical Research Council of Great Britain (MRC) system is the best known and most commonly used muscle strength grading system for manual muscle testing (MMT) worldwide. Dyck et al1 trace the development of the MRC system to the treatment of war injuries and poliomyelitis. S.W. Mitchell, a Civil War surgeon, recognized nerve damage as a cause of muscle weakness in 1872. Mitchell and M.J ...
The assessment of muscle power is a key part of a neurological examination of the upper or lower limbs. As a result, it is important to familiarise yourself with the Medical Research Council's scale (MRC scale) of muscle power. The MRC scale of muscle strength uses a score of 0 to 5 to grade the power of a particular muscle group in relation to the movement of a single joint.
The Medical Research Council grading system has served through decades for the evaluation of muscle strength and has been recognized as a cardinal feature of daily neurological, rehabilitation and general medicine examination of patients, despite being respectfully criticized due to the unequal width of its response options.
MRC stands for Medical Research Council and is a taxonomy standard to measure muscle strength by resisted isometrics on a scale from 0 to 5.
Medical research council grading system for muscle strength This is a commonly used grading system in neurosurgical practice to assess patient's muscle strength.
The Medical Research Council of Great Britain (MRC) system is known and most commonly used muscle strength grading system for manual muscle testing (MMT) worldwide. The MRC system was intended to ...
Use of the Medical Research Council muscle strength grading system in the upper extremity.
Medical Research Council and modified Medical Research Council scales are measurements with substantial inter-rater and intra-rater reliability in evaluating forearm muscles.
Two MRC scoring criteria were used to score muscle strength using MMSt in 40 muscle groups of the upper and lower limbs in patients with neuromuscular disorders. Three investigators performed the evaluations; the order of the MMSt and the use of the scales were performed according to the preferences of the investigators.
Scale To proceed to voluntary muscle strength assessment, the neurologic en hemodynamic
The Medical Research Council grading system has served through decades for the evaluation of muscle strength and has been recognized as a cardinal feature of daily neurological, rehabilitation and ...
Learn how to assess muscle strength in critically ill patients using manual muscle testing, a reliable and valid method, from cieslaetal2011.
Use of the Medical Research Council muscle strength grading system in the upper extremity. - Post - Medbullets Step 2/3 Hypertensive Urgency And Emergency
The Medical Research Council grading system has served through decades for the evaluation of muscle strength and has been recognized as a cardinal feature of daily neurological, rehabilitation and general medicine examination of patients, despite being respectfully criticized due to the unequal width of its response options.
Simple tests with potentially greater clinical applicability have been proposed. A measure of global peripheral muscle strength, the Medical Research Council sum score (MRC-SS), which ranges from 0 (complete paralysis) to 60 (normal strength) [ 16 ], has been widely used, with scores less than 48 providing the basis for diagnosing ICU-AW [ 14 ].
Below is the list of 17 best universities for Medicine in Saint Petersburg ranked based on their research performance: a graph of 271K citations received by 35.9K academic papers made by these universities was used to calculate ratings and create the top.
Grading System for Saint Petersburg State University Russia GPA calculator
Using the Medical Research Council (MRC) scale, muscle strength in the flexors of the lower legs was reduced by 4 grades, by 5 grades in the extensors, and 5 grades in the distal sections.
Neurological examination confirmed reduced muscle strength, which in combination with morphological examination of muscle tissue resulted in the diagnosis of limb-girdle muscular dystrophy.