Information
- Author Services
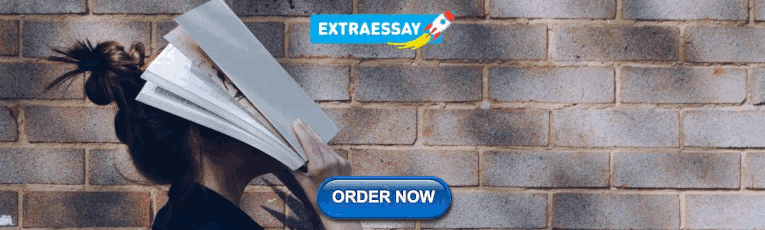
Initiatives
You are accessing a machine-readable page. In order to be human-readable, please install an RSS reader.
All articles published by MDPI are made immediately available worldwide under an open access license. No special permission is required to reuse all or part of the article published by MDPI, including figures and tables. For articles published under an open access Creative Common CC BY license, any part of the article may be reused without permission provided that the original article is clearly cited. For more information, please refer to https://www.mdpi.com/openaccess .
Feature papers represent the most advanced research with significant potential for high impact in the field. A Feature Paper should be a substantial original Article that involves several techniques or approaches, provides an outlook for future research directions and describes possible research applications.
Feature papers are submitted upon individual invitation or recommendation by the scientific editors and must receive positive feedback from the reviewers.
Editor’s Choice articles are based on recommendations by the scientific editors of MDPI journals from around the world. Editors select a small number of articles recently published in the journal that they believe will be particularly interesting to readers, or important in the respective research area. The aim is to provide a snapshot of some of the most exciting work published in the various research areas of the journal.
Original Submission Date Received: .
- Active Journals
- Find a Journal
- Proceedings Series
- For Authors
- For Reviewers
- For Editors
- For Librarians
- For Publishers
- For Societies
- For Conference Organizers
- Open Access Policy
- Institutional Open Access Program
- Special Issues Guidelines
- Editorial Process
- Research and Publication Ethics
- Article Processing Charges
- Testimonials
- Preprints.org
- SciProfiles
- Encyclopedia
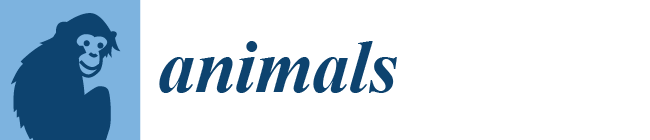
Article Menu
- Subscribe SciFeed
- Recommended Articles
- PubMed/Medline
- Google Scholar
- on Google Scholar
- Table of Contents
Find support for a specific problem in the support section of our website.
Please let us know what you think of our products and services.
Visit our dedicated information section to learn more about MDPI.
JSmol Viewer
Problem solving in animals: proposal for an ontogenetic perspective.
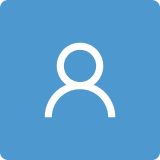
Simple Summary
1. introduction, 2. factors affecting the development of problem solving, 2.1. instrinic factors, 2.1.1. direct genetic effects, 2.1.2. indirect genetic effects, 2.1.3. neuroendocrine effects—brain morphology, 2.1.4. neuroendocrine effects—hormones, 2.2. extrinsic factors, 2.2.1. physical environmental factors, 2.2.2. social environmental factors, 3. interacting factors that influence the development of problem solving, 3.1. gene × environment interactions, 3.2. neuroendocrine × environment interactions, 3.3. age effects, 3.4. learning and experience, 3.5. behavioural flexibility and personality, 4. forgotten components limiting our understanding of problem solving and its development, 5. an individual-centric focus can be beneficial, 6. conclusions, author contributions, acknowledgments, conflicts of interest.
- Rymer, T.L.; Pillay, N.; Schradin, C. Extinction or survival? Behavioral flexibility in response to environmental change in the African striped mouse Rhabdomys . Sustainability 2013 , 5 , 163–186. [ Google Scholar ] [ CrossRef ] [ Green Version ]
- Benson-Amram, S.; Dantzer, B.; Stricker, G.; Swanson, E.M.; Holekamp, K.E. Brain size predicts problem-solving ability in mammalian carnivores. Proc. Natl. Acad. Sci. USA 2016 , 113 , 2532–2537. [ Google Scholar ] [ CrossRef ] [ Green Version ]
- Lambert, M.L.; Jacobs, I.; Osvath, M.; von Bayern, A.M. Birds of a feather? Parrot and corvid cognition compared. Behaviour 2019 , 156 , 505–594. [ Google Scholar ] [ CrossRef ]
- Taylor, A.H. Corvid cognition. Wiley Interdiscip. Rev. Cogn. Sci. 2014 , 5 , 361–372. [ Google Scholar ] [ CrossRef ]
- Szabo, B.; Noble, D.W.; Whiting, M.J. Learning in non-avian reptiles 40 years on: Advances and promising new directions. Biol. Rev. 2020 . [ Google Scholar ] [ CrossRef ] [ PubMed ]
- Munteanu, A.M.; Starnberger, I.; Pašukonis, A.; Bugnyar, T.; Hödl, W.; Fitch, W.T. Take the long way home: Behaviour of a neotropical frog, Allobates femoralis , in a detour task. Behav. Process. 2016 , 126 , 71–75. [ Google Scholar ] [ CrossRef ] [ Green Version ]
- Laland, K.N.; Reader, S.M. Foraging innovation in the guppy. Anim. Behav. 1999 , 57 , 331–340. [ Google Scholar ] [ CrossRef ] [ PubMed ] [ Green Version ]
- Dussutour, A.; Deneubourg, J.L.; Beshers, S.; Fourcassié, V. Individual and collective problem-solving in a foraging context in the leaf-cutting ant Atta colombica . Anim. Cogn. 2009 , 12 , 21. [ Google Scholar ] [ CrossRef ] [ PubMed ]
- Keagy, J.; Savard, J.-F.; Borgia, G. Male satin bowerbird problem-solving ability predicts mating success. Anim. Behav. 2009 , 78 , 809–817. [ Google Scholar ] [ CrossRef ]
- Cauchard, L.; Boogert, N.J.; Lefebvre, L.; Dubois, F.; Doligez, B. Problem-solving performance is correlated with reproductive success in a wild bird population. Anim. Behav. 2013 , 85 , 19–26. [ Google Scholar ] [ CrossRef ]
- Reader, S.M.; Laland, K.N. Animal Innovation ; Oxford University Press: Oxford, UK, 2003. [ Google Scholar ]
- Kummer, H.; Goodall, J. Conditions of innovative behaviour in primates. Philos. Trans. R. Soc. Lond. Ser. B 1985 , 308 , 203–214. [ Google Scholar ] [ CrossRef ]
- Dean, L.G.; Hoppitt, W.; Laland, K.N.; Kendal, R.L. Sex ratio affects sex-specific innovation and learning in captive ruffed lemurs ( Varecia variegata and Varecia rubra ). Am. J. Primatol. 2011 , 73 , 1210–1221. [ Google Scholar ] [ CrossRef ]
- Daniels, S.E.; Fanelli, R.E.; Gilbert, A.; Benson-Amram, S. Behavioral flexibility of a generalist carnivore. Anim. Cogn. 2019 , 22 , 387–396. [ Google Scholar ] [ CrossRef ] [ Green Version ]
- Epstein, R. Generativity theory. Encycl. Creat. 1999 , 1 , 759–766. [ Google Scholar ]
- Seed, A.M.; Call, J. Problem-solving in tool-using and non-tool-using animals. Encycl. Anim. Behav. 2010 , 2 , 778–785. [ Google Scholar ]
- Duncker, K.; Lees, L.S. On problem solving. Psychol. Monogr. 1945 , 58 , i-113. [ Google Scholar ] [ CrossRef ]
- Scheerer, M. Problem-solving. Sci. Am. 1963 , 208 , 118–131. [ Google Scholar ] [ CrossRef ]
- Anderson, B.F. The Complete Thinker: A Handbook of Techniques for Creative and Critical Problem Solving ; Prentice-Hall: Upper Saddle River, NJ, USA, 1980. [ Google Scholar ]
- Heppner, P.P.; Krauskopf, C.J. An information-processing approach to personal problem solving. Couns. Psychol. 1987 , 15 , 371–447. [ Google Scholar ] [ CrossRef ]
- Hauser, M.D.; Kralik, J.; Botto-Mahan, C. Problem solving and functional design features: Experiments on cotton-top tamarins, Saguinus oedipus oedipus . Anim. Behav. 1999 , 57 , 565–582. [ Google Scholar ] [ CrossRef ] [ PubMed ]
- Shimabukuro, C.; Putrino, N.; Helbling, J.; Tognetti, S.; Bentosela, M. Individual differences in social and non-social behaviors in domestic dogs ( Canis familiaris ) during the acquisition, extinction and reacquisition of a problem solving task. Behav. Process. 2015 , 113 , 179–186. [ Google Scholar ] [ CrossRef ] [ PubMed ]
- Chow, P.K.Y.; Lea, S.E.G.; Hempel de Ibarra, N.; Robert, T. How to stay perfect: The role of memory and behavioural traits in an experienced problem and a similar problem. Anim. Cogn. 2017 , 20 , 941–952. [ Google Scholar ] [ CrossRef ] [ Green Version ]
- Sol, D.; Duncan, R.P.; Blackburn, T.M.; Cassey, P.; Lefebvre, L. Big brains, enhanced cognition, and response of birds to novel environments. Proc. Natl. Acad. Sci. USA 2005 , 102 , 5460–5465. [ Google Scholar ] [ CrossRef ] [ PubMed ] [ Green Version ]
- Sol, D.; Lefebvre, L. Behavioural flexibility predicts invasion success in birds introduced to New Zealand. Oikos 2000 , 90 , 599–605. [ Google Scholar ] [ CrossRef ] [ Green Version ]
- Kozlovsky, D.Y.; Branch, C.L.; Pravosudov, V.V. Problem-solving ability and response to novelty in mountain chickadees ( Poecile gambeli ) from different elevations. Behav. Ecol. Sociobiol. 2015 , 69 , 635–643. [ Google Scholar ] [ CrossRef ]
- Thornton, A.; Samson, J. Innovative problem solving in wild meerkats. Anim. Behav. 2012 , 83 , 1459–1468. [ Google Scholar ] [ CrossRef ]
- Heinrich, B.; Bugnyar, T. Testing Problem Solving in Ravens: String-Pulling to Reach Food. Ethology 2005 , 111 , 962–976. [ Google Scholar ] [ CrossRef ]
- Webster, S.J.; Lefebvre, L. Problem solving and neophobia in a columbiform–passeriform assemblage in Barbados. Anim. Behav. 2001 , 62 , 23–32. [ Google Scholar ] [ CrossRef ] [ Green Version ]
- Ducatez, S.; Audet, J.; Rodriguez, J.R.; Kayello, L.; Lefebvre, L. Innovativeness and the effects of urbanization on risk-taking behaviors in wild Barbados birds. Anim. Cogn. 2017 , 20 , 33–42. [ Google Scholar ] [ CrossRef ]
- MacLean, E.V.; Matthews, L.J.; Hare, B.A.; Nunn, C.L.; Anderson, R.C.; Aureli, F.; Brannon, E.M.; Call, J.; Drea, C.M.; Emery, N.J.; et al. How does cognition evolve? Phylogenetic comparative psychology. Anim. Cogn. 2014 , 15 , 223–238. [ Google Scholar ] [ CrossRef ]
- Cook, M.O.; Weaver, M.J.; Hutton, P.; McGraw, K.J. The effects of urbanization and human disturbance on problem solving in juvenile house finches ( Haemorhous mexicanus ). Behav. Ecol. Sociobiol. 2017 , 71 , 85. [ Google Scholar ] [ CrossRef ]
- Holekamp, K.E.; Dantzer, B.; Stricker, G.; Yoshida, K.C.S.; Benson-Amram, S. Brains, brawn and sociality: A hyaena’s tale. Anim. Behav. 2015 , 103 , 237–248. [ Google Scholar ] [ CrossRef ] [ Green Version ]
- Stamps, J. Behavioural processes affecting development: Tinbergen’s fourth question comes of age. Anim. Behav. 2003 , 66 , 1–13. [ Google Scholar ] [ CrossRef ] [ Green Version ]
- Ryan, M.J.; Wilczynski, W. An Introduction to Animal Behaviour ; Cold Spring Harbor Laboratory Press: Cold Spring Harbor, NY, USA, 2011. [ Google Scholar ]
- Groothuis, T.G.G.; Trillmich, F. Unfolding Personalities: The Importance of Studying Ontogeny. Dev. Psychobiol. 2011 , 53 , 641–655. [ Google Scholar ] [ CrossRef ] [ PubMed ] [ Green Version ]
- Fraser, D.F.; Gilliam, J.F. Feeding under predation hazard: Response of the guppy and Hart’s rivulus from sites with contrasting predation hazard. Behav. Ecol. Sociobiol. 1987 , 21 , 203–209. [ Google Scholar ] [ CrossRef ]
- Guenther, A.; Finkemeier, M.-A.; Trillmich, F. The ontogeny of personality in the wild guinea pig. Anim. Behav. 2014 , 90 , 131–139. [ Google Scholar ] [ CrossRef ]
- Rymer, T.L.; Pillay, N. An integrated understanding of paternal care in mammals: Lessons from the rodents. J. Zool. 2018 , 306 , 69–76. [ Google Scholar ] [ CrossRef ] [ Green Version ]
- Cole, E.F.; Cram, D.L.; Quinn, J.L. Individual variation in spontaneous problem-solving performance among wild great tits. Anim. Behav. 2011 , 81 , 491–498. [ Google Scholar ] [ CrossRef ]
- Rowe, C.; Healy, S.D. Measuring variation in cognition. Behav. Ecol. 2014 , 25 , 1287–1292. [ Google Scholar ] [ CrossRef ] [ Green Version ]
- Kaplan, G. Bird Minds: Cognition and Behaviour of Australian Native Birds ; CSIRO Publishing: Clayton, Australia, 2015. [ Google Scholar ]
- Elliott, O.; Scott, J.P. The analysis of breed differences in maze performance in dogs. Anim. Behav. 1965 , 13 , 5–18. [ Google Scholar ] [ CrossRef ]
- Miller, C.A.; Sweatt, J.D. Covalent Modification of DNA Regulates Memory Formation. Neuron 2007 , 53 , 857–869. [ Google Scholar ] [ CrossRef ] [ PubMed ] [ Green Version ]
- Bókony, V.; Lendvai, A.Z.; Vagasi, C.I.; Patras, L.; Pap, P.L.; Nemeth, J.; Vincze, E.; Papp, S.; Preiszner, B.; Seress, G.; et al. Necessity or capacity? Physiological state predicts problem-solving performance in house sparrows. Behav. Ecol. 2014 , 25 , 124–135. [ Google Scholar ] [ CrossRef ]
- Shaw, R.C. Testing cognition in the wild: Factors affecting performance and individual consistency in two measures of avian cognition. Behav. Process. 2017 , 134 , 31–36. [ Google Scholar ] [ CrossRef ] [ PubMed ]
- Jenkins, B.R.; Vitousek, M.N.; Hubbard, J.K.; Safran, R.J. An experimental analysis of the heritability of variation in glucocorticoid concentrations in a wild avian population. Proc. Biol. Sci. 2014 , 281 , 20141302. [ Google Scholar ] [ CrossRef ]
- Rymer, T.; Pillay, N. Transmission of parental care behavior in African striped mice, Rhabdomys pumilio . J. Exp. Zool. 2011 , 315 , 631–638. [ Google Scholar ] [ CrossRef ] [ PubMed ]
- Dingemanse, N.J.; Both, C.; Drent, P.J.; van Oers, K.; van Noordwijk, A.J. Repeatability and heritability of exploratory behaviour in great tits from the wild. Anim. Behav. 2002 , 64 , 929–938. [ Google Scholar ] [ CrossRef ] [ Green Version ]
- Hopkins, W.D.; Russell, J.L.; Schaeffer, J. Chimpanzee intelligence is heritable. Curr. Biol. 2014 , 24 , 1649–1652. [ Google Scholar ] [ CrossRef ] [ Green Version ]
- Dudde, A.; Krause, E.T.; Matthews, L.R.; Schrader, L. More Than Eggs—Relationship Between Productivity and Learning in Laying Hens. Front. Psychol. 2018 , 9 , 2000. [ Google Scholar ] [ CrossRef ] [ PubMed ] [ Green Version ]
- Upchurch, M.; Wehner, J.M. Inheritance of spatial learning ability in inbred mice: A classical genetic analysis. Behav. Neurosci. 1989 , 103 , 1251–1258. [ Google Scholar ] [ CrossRef ]
- Laumer, I.B.; Bugnyar, T.; Reber, S.A.; Auersperg, A.M. Can hook-bending be let off the hook? Bending/unbending of pliant tools by cockatoos. Proc. R. Soc. B: Biol. Sci. 2017 , 284 , 20171026. [ Google Scholar ] [ CrossRef ] [ Green Version ]
- Audet, J.N.; Kayello, L.; Ducatez, S.; Perillo, S.; Cauchard, L.; Howard, J.T.; O’Connell, L.A.; Jarvis, E.D.; Lefebvre, L. Divergence in problem-solving skills is associated with differential expression of glutamate receptors in wild finches. Sci. Adv. 2018 , 4 , eaao6369. [ Google Scholar ] [ CrossRef ] [ Green Version ]
- Tolman, E.C. The Inheritance of Maze-Learning Ability in Rats. J. Comp. Psychol. 1924 , 4 , 1–18. [ Google Scholar ] [ CrossRef ] [ Green Version ]
- Heron, W.T. The inheritance of maze learning ability in rats. J. Comp. Psychol. 1935 , 19 , 77–89. [ Google Scholar ] [ CrossRef ] [ Green Version ]
- Lagzi, I.; Soh, S.; Wesson, P.J.; Browne, K.P.; Grzybowski, B.A. Maze Solving by Chemotactic Droplets. J. Am. Chem. Soc. 2010 , 132 , 1198–1199. [ Google Scholar ] [ CrossRef ]
- Quinn, J.L.; Cole, E.F.; Reed, T.E.; Morand-Ferron, J. Environmental and genetic determinants of innovativeness in a natural population of birds. Philos. Trans. R. Soc. B 2016 , 371 , 20150184. [ Google Scholar ] [ CrossRef ] [ PubMed ] [ Green Version ]
- Bókony, V.; Pipoly, I.; Szabó, K.; Preiszner, B.; Vincze, E.; Papp, S.; Seress, G.; Hammer, T.; Liker, A. Innovative females are more promiscuous in great tits ( Parus major ). Behav. Ecol. 2017 , 28 , 579–588. [ Google Scholar ] [ CrossRef ] [ Green Version ]
- Bonduriansky, R.; Day, T. Nongenetic Inheritance and Its Evolutionary Implications. Annu. Rev. Ecol. Evol. Syst. 2009 , 40 , 103–125. [ Google Scholar ] [ CrossRef ] [ Green Version ]
- Ma, Y.J.; Junier, M.P.; Costa, M.E.; Ojeda, S.R. Transforming growth factor-α gene expression in the hypothalamus is developmentally regulated and linked to sexual maturation. Neuron 1992 , 9 , 657–670. [ Google Scholar ] [ CrossRef ] [ PubMed ]
- Gräff, J.; Mansuy, I.M. Epigenetic codes in cognition and behaviour. Behav. Brain Res. 2008 , 192 , 70–87. [ Google Scholar ] [ CrossRef ]
- Forrest, D.; Hallbook’, F.; Persson, H.; Vennstrom, B. Distinct functions for thyroid hormone receptors alpha and beta in brain development indicated by differential expression of receptor genes. EMBO J. 1991 , 10 , 269–275. [ Google Scholar ] [ CrossRef ]
- Liu, D.; Diorio, J.; Day, J.C.; Francis, D.D.; Meaney, M.J. Maternal care, hippocampal synaptogenesis and cognitive development in rats. Nat. Neurosci. 2000 , 3 , 799–806. [ Google Scholar ] [ CrossRef ] [ PubMed ]
- Rowell, M.K.; Rymer, T.L. Memory enhances problem solving in the fawn-footed mosaic-tailed rat Melomys cervinipes . Anim. Cogn. in review.
- Champagne, F.A. Epigenetic mechanisms and the transgenerational effects of maternal care. Front. Neuroendocrinol. 2008 , 29 , 386–397. [ Google Scholar ] [ CrossRef ] [ Green Version ]
- Skinner, M.K.; Anway, M.D.; Savenkova, M.I.; Gore, A.C.; Crews, D. Transgenerational Epigenetic Programming of the Brain Transcriptome and Anxiety Behavior. PLoS ONE 2008 , 3 , e3745. [ Google Scholar ] [ CrossRef ]
- Li, S.; Tian, X.; Hartley, D.M.; Feig, L.A. The environment versus genetics in controlling the contribution of MAP kinases to synaptic plasticity. Curr. Biol. 2006 , 16 , 2303–2313. [ Google Scholar ] [ CrossRef ] [ Green Version ]
- Knudsen, E.I. Sensitive Periods in the Development of the Brain and Behavior. J. Cogn. Neurosci. 2004 , 16 , 1412–1425. [ Google Scholar ] [ CrossRef ]
- Medina, J.F.; Repa, J.C.; Mauk, M.D.; LeDoux, J.E. Parallels between cerebellum-and amygdala-dependent conditioning. Nat. Rev. Neurosci. 2002 , 3 , 122–131. [ Google Scholar ] [ CrossRef ]
- Malenka, R.C.; Nicoll, R.A. Long-term potentiation—A decade of progress? Science 1999 , 285 , 1870–1874. [ Google Scholar ] [ CrossRef ] [ Green Version ]
- Sherry, D.F.; Hoshooley, J.S. Seasonal hippocampal plasticity in food-storing birds. Philos. Trans. R. Soc. B: Biol. Sci. 2010 , 365 , 933–943. [ Google Scholar ] [ CrossRef ]
- Iwaniuk, A.A.; Lefebvre, L.; Wylie, D.R. The comparative approach and brain-behaviour relationships: A tool for understanding tool use. Can. J. Exp. Psychol. /Rev. Can. De Psychol. Exp. 2009 , 63 , 150–159. [ Google Scholar ] [ CrossRef ] [ Green Version ]
- Lefebvre, L.; Whittle, P.; Lascaris, E.; Finkelstein, A. Feeding innovations and forebrain size in birds. Anim. Behav. 1997 , 53 , 549–560. [ Google Scholar ] [ CrossRef ] [ Green Version ]
- Mehlhorn, J.; Hunt, G.R.; Gray, R.D.; Rehkämper, G.; Güntürkün, O. Tool-making New Caledonian crows have large associative brain areas. Brain Behav. Evol. 2010 , 75 , 63–70. [ Google Scholar ] [ CrossRef ] [ PubMed ]
- Ben Abdallah, N.M.-B.; Fuss, J.; Trusel, M.; Galsworthy, M.J.; Bobsin, K.; Colacicco, G.; Deacon, R.M.J.; Riva, M.A.; Kellendonk, C.; Sprengel, R.; et al. The puzzle box as a simple and efficient behavioral test for exploring impairments of general cognition and executive functions in mouse models of schizophrenia. Exp. Neurol. 2011 , 227 , 42–52. [ Google Scholar ] [ CrossRef ]
- Meethal, S.V.; Atwood, C.S. The role of hypothalamic-pituitary-gonadal hormones in the normal structure and functioning of the brain. Cell. Mol. Life Sci. 2005 , 62 , 257–270. [ Google Scholar ] [ CrossRef ]
- Kuiri-Hänninen, T.; Sankilampi, U.; Dunkel, L. Activation of the Hypothalamic-Pituitary-Gonadal Axis in Infancy: Minipuberty. Horm. Res. Paediatr. 2014 , 82 , 73–80. [ Google Scholar ] [ CrossRef ]
- Lincoln, E.A.; Zuber, T.J. Management of Precocious Puberty. Hosp. Pract. 1998 , 33 , 173–176. [ Google Scholar ] [ CrossRef ]
- Kimura, D.; Hampson, E. Cognitive pattern in men and women is influenced by fluctuations in sex hormones. Curr. Dir. Psychol. Sci. 1994 , 3 , 57–61. [ Google Scholar ] [ CrossRef ]
- Gibbs, R.B. Testosterone and estradiol produce different effects on cognitive performance in male rats. Horm. Behav. 2005 , 48 , 268–277. [ Google Scholar ] [ CrossRef ] [ Green Version ]
- Stewart, J.; Skvarenina, A.; Pottier, J. Effects of neonatal androgens on open-field behavior and maze learning in the prepubescent and adult rat. Physiol. Behav. 1975 , 14 , 291–295. [ Google Scholar ] [ CrossRef ]
- Belz, E.E.; Kennell, J.S.; Czambel, R.K.; Rubin, R.T.; Rhodes, M.E. Environmental enrichment lowers stress-responsive hormones in singly housed male and female rats. Pharmacol. Biochem. Behav. 2003 , 76 , 481–486. [ Google Scholar ] [ CrossRef ]
- Charmandari, E.; Tsigos, C.; Chrousos, G. Endocrinology of the stress response. Annu. Rev. Physiol. 2005 , 67 , 259–284. [ Google Scholar ] [ CrossRef ]
- Joëls, M.; Pu, Z.; Wiegert, O.; Oitzl, M.S.; Krugers, H.J. Learning under stress: How does it work? Trends Cogn. Sci. 2006 , 10 , 152–158. [ Google Scholar ] [ CrossRef ]
- Knapman, A.; Heinzmann, J.-M.; Hellweg, R.; Holsboer, F.; Landgraf, R.; Touma, C. Increased stress reactivity is associated with cognitive deficits and decreased hippocampal brain-derived neurotrophic factor in a mouse model of affective disorders. J. Psychiatr. Res. 2010 , 44 , 566–575. [ Google Scholar ] [ CrossRef ] [ PubMed ]
- Yu, T.; Guo, M.; Garza, J.; Rendon, S.; Sun, X.-L.; Zhang, W.; Lu, X.-Y. Cognitive and neural correlates of depression-like behaviour in socially defeated mice: An animal model of depression with cognitive dysfunction. Int. J. Neuropsychopharmacol. 2011 , 14 , 303–317. [ Google Scholar ] [ CrossRef ] [ PubMed ] [ Green Version ]
- McLay, R.N.; Freeman, S.M.; Zadina, J.E. Chronic Corticosterone Impairs Memory Performance in the Barnes Maze. Physiol. Behav. 1998 , 63 , 933–937. [ Google Scholar ] [ CrossRef ]
- Roozendaal, B. Stress and Memory: Opposing Effects of Glucocorticoids on Memory Consolidation and Memory Retrieval. Neurobiol. Learn. Mem. 2002 , 78 , 578–595. [ Google Scholar ] [ CrossRef ] [ Green Version ]
- Mashaly, M.M. Effect of exogenous corticosterone on chicken embryonic-development. Poult. Sci. 1991 , 70 , 371–374. [ Google Scholar ] [ CrossRef ] [ PubMed ]
- Eriksen, M.S.; Haug, A.; Torjesen, P.A.; Bakken, M. Prenatal exposure to corticosterone impairs embryonic development and increases fluctuating asymmetry in chickens ( Gallus gallus domesticus ). Br. Poult. Sci. 2003 , 44 , 690–697. [ Google Scholar ] [ CrossRef ] [ PubMed ]
- Alcaro, A.; Huber, R.; Panksepp, J. Behavioral Functions of the Mesolimbic Dopaminergic System: An Affective Neuroethological Perspective. Brain Res. Rev. 2007 , 56 , 283–321. [ Google Scholar ] [ CrossRef ] [ PubMed ] [ Green Version ]
- Brisch, R.; Saniotis, A.; Wolf, R.; Bielau, H.; Bernstein, H.; Steiner, J.; Bogerts, B.; Braun, K.; Jankowski, Z.; Kumaratilake, J.; et al. The role of dopamine in schizophrenia from a neurobiological and evolutionary perspective: Old fashioned, but still in vogue. Front. Psychiatry 2014 , 5 , 1–47. [ Google Scholar ] [ CrossRef ]
- Laszy, J.; Laszlovszky, I.; Gyertyán, I. Dopamine D3 receptor antagonists improve the learning performance in memory-impaired rats. Psychopharmacology 2005 , 179 , 567–575. [ Google Scholar ] [ CrossRef ]
- Griffin, A.S.; Guez, D. Innovation and problem solving: A review of common mechanisms. Behav. Process. 2014 , 109 , 121–134. [ Google Scholar ] [ CrossRef ] [ PubMed ]
- Papp, S.; Vincze, E.; Preiszner, B.; Liker, A.; Bókony, V. A comparison of problem-solving success between urban and rural house sparrows. Behav. Ecol. Sociobiol. 2015 , 69 , 471–480. [ Google Scholar ] [ CrossRef ]
- van Horik, J.O.; Madden, J.R. A problem with problem solving: Motivational traits, but not cognition, predict success on novel operant foraging tasks. Anim. Behav. 2016 , 114 , 189–198. [ Google Scholar ] [ CrossRef ] [ Green Version ]
- Lermite, F.; Peneaux, C.; Griffin, A.S. Personality and problem-solving in common mynas ( Acridotheres tristis ). Behav. Process. 2017 , 134 , 87–94. [ Google Scholar ] [ CrossRef ] [ PubMed ]
- Borrego, N.; Gaines, M. Social carnivores outperform asocial carnivores on an innovative problem. Anim. Behav. 2016 , 114 , 21–26. [ Google Scholar ] [ CrossRef ]
- Nieoullon, A. Dopamine and the regulation of cognition and attention. Prog. Neurobiol. 2002 , 67 , 53–83. [ Google Scholar ] [ CrossRef ]
- Ikemoto, S.; Panksepp, J. The role of nucleus accumbens dopamine in motivated behavior: A unifying interpretation with special reference to reward-seeking. Brain Res. Rev. 1999 , 31 , 6–41. [ Google Scholar ] [ CrossRef ]
- Taghzouti, K.; Louilot, A.; Herman, J.P.; le Moal, M.; Simon, H. Alternation behaviour, spatial discrimination, and reversal disturbances following 6-Hydroxydopamine lesions in the nucleus accumbens of the rat. Behav. Neural Biol. 1985 , 44 , 354–363. [ Google Scholar ] [ CrossRef ]
- Kabadayi, C.; Krasheninnikova, A.; O’neill, L.; van de Weijer, K.; Osvath, M.; von Bayern, A.M. Are parrots poor at motor self-regulation or is the cylinder task poor at measuring it? Anim. Cogn. 2017 , 20 , 1137–1146. [ Google Scholar ] [ CrossRef ]
- Broom, D.M. A History of Animal Welfare Science. Acta Biotheor. 2011 , 59 , 121–137. [ Google Scholar ] [ CrossRef ] [ PubMed ]
- Black, R.E.; Allen, L.H.; Bhutta, Z.A.; Caulfield, L.E.; De Onis, M.; Ezzati, M.; Mathers, C.; Rivera, J.; Maternal and Child Undernutrition Study Group. Maternal and child undernutrition: Global and regional exposures and health consequences. Lancet 2008 , 371 , 243–260. [ Google Scholar ] [ CrossRef ]
- Izquierdo, A.; Newman, T.K.; Higley, J.D.; Murray, E.A. Genetic modulation of cognitive flexibility and socioemotional behavior in rhesus monkeys. Proc. Natl. Acad. Sci. USA 2007 , 104 , 14128–14133. [ Google Scholar ] [ CrossRef ] [ Green Version ]
- Vallender, E.J.; Lynch, L.; Novak, M.A.; Miller, G.M. Polymorphisms in the 3 ′UTR of the serotonin transporter are associated with cognitive flexibility in rhesus macaques. Am. J. Med Genet. B Neuropsychiatr. Genet. 2009 , 150 , 467–475. [ Google Scholar ] [ CrossRef ] [ PubMed ] [ Green Version ]
- Fitzgerald, P.J. A neurochemical yin and yang: Does serotonin activate and norepinephrine deactivate the prefrontal cortex? Psychopharmacology 2011 , 213 , 171–182. [ Google Scholar ] [ CrossRef ] [ PubMed ]
- Delion, S.; Chalon, S.; Guillotaeu, D.; Besnard, J.-C.; Durand, G. α -Linolenic acid deficiency alters age-related changes of dopaminergic and serotonergic neurotransmission in the rat frontal cortex. J. Neurochem. 1996 , 66 , 1582–1591. [ Google Scholar ] [ CrossRef ]
- Zimmer, I.; Hembert, S.; Dward, G.; Breton, P.; Guilloteau, D.; Besnard, J.-C.; Chalon, S. Chronic n-3 polyunsaturated fatty acid diet-deficiency acts on dopamine metabolism in the rat frontal cortex: A microdialysis study. Neurosci. Lett. 1998 , 240 , 177–181. [ Google Scholar ] [ CrossRef ]
- Wainwright, P.C.; Osenberg, C.W.; Mittelbach, G.G. Trophic Polymorphism in the Pumpkinseed Sunfish ( Lepomis gibbosus Linnaeus ): Effects of Environment on Ontogeny. Funct. Ecol. 1991 , 5 , 40–55. [ Google Scholar ] [ CrossRef ]
- Wiens, J.A. Ecological heterogeneity: An ontogeny of concepts and approaches. In The Ecological Consequences of Heterogeneity ; Hutchin, M.J., John, E.A., Stewart, A.J.A., Eds.; Oxford: Berlin, Germany, 2000; pp. 9–31. [ Google Scholar ]
- Eccard, J.A.; Walther, R.B.; Milton, S.J. How livestock grazing affects vegetation structures and small mammal distribution in the semi-arid Karoo. J. Arid Environ. 2000 , 46 , 103–106. [ Google Scholar ] [ CrossRef ]
- Stamps, J.A.; Swaisgood, R.R. Someplace like home: Experience, habitat selection and conservation biology. Appl. Anim. Behav. Sci. 2007 , 102 , 392–409. [ Google Scholar ] [ CrossRef ]
- Madsen, T.; Shine, R. Seasonal migration of predators and prey—A study of pythons and rats in tropical Australia. Ecology 1996 , 77 , 149–156. [ Google Scholar ] [ CrossRef ]
- Sztainberg, Y.; Chen, A. An environmental enrichment model for mice. Nat. Protoc. 2010 , 5 , 1535–1539. [ Google Scholar ] [ CrossRef ]
- Husband, A.J.; Bryden, W.L. Nutrition, stress and immune activation. Proc. Nutr. Soc. Aust. 1996 , 20 , 60–70. [ Google Scholar ]
- Johnstone, C.P.; Lill, A.; Reina, R.D. Does habitat fragmentation cause stress in the agile antechinus? A haematological approach. J. Comp. Physiol. B 2012 , 182 , 139–155. [ Google Scholar ] [ CrossRef ]
- Genaro, G.; Schmidek, W.R. Exploratory activity of rats in three different environments. Ethology 2001 , 106 , 849–859. [ Google Scholar ] [ CrossRef ]
- Nilsson, M.; Perfilieva, E.; Johansson, U.; Orwar, O.; Eriksson, P.S. Enriched environment increases neurogenesis in the adult rat dentate gyrus and improves spatial memory. J. Neurobiol. 1999 , 39 , 569–578. [ Google Scholar ] [ CrossRef ]
- Liker, A.; Bókony, V. Larger groups are more successful in innovative problem solving in house sparrows. Proc. Natl. Acad. Sci. USA 2009 , 106 , 7893–7898. [ Google Scholar ] [ CrossRef ] [ Green Version ]
- Bergman, T.J.; Beehner, J.C. Measuring social complexity. Anim. Behav. 2015 , 103 , 203–209. [ Google Scholar ] [ CrossRef ]
- Gittleman, J.L.; Thompson, S.D. Energy allocation in mammalian reproduction. Am. Zool. 1988 , 28 , 863–875. [ Google Scholar ] [ CrossRef ]
- Goebelsmann, U.; Roberts, J.M.; Jaffe, R.B. Placental transfer of 3H-oestriol-3-sulphate-16-glucosidur-onate and 3H-oestriol-16-glucosiduronate-14C. Acta Endocrinol. 1972 , 70 , 132–142. [ Google Scholar ] [ CrossRef ]
- Schulz, K.M.; Pearson, J.N.; Neeley, E.W.; Berger, R.; Leonard, S.; Adams, C.E.; Stevens, K.E. Maternal stress during pregnancy causes sex-specific alterations in offspring memory performance, social interactions, indices of anxiety, and body mass. Physiol. Behav. 2011 , 104 , 340–347. [ Google Scholar ] [ CrossRef ] [ PubMed ] [ Green Version ]
- Pillay, N.; Rimbach, R.; Rymer, T. Pre-and postnatal dietary protein deficiency influences anxiety, memory and social behaviour in the African striped mouse Rhabdomys dilectus chakae . Physiol. Behav. 2016 , 161 , 38–46. [ Google Scholar ] [ CrossRef ] [ PubMed ]
- Cheney, D.L.; Seyfarth, R.M. Nonrandom dispersal in free-ranging vervet monkeys: Social and genetic consequences. Am. Nat. 1983 , 122 , 392–412. [ Google Scholar ] [ CrossRef ]
- Lynn, D.A.; Brown, G.R. The ontogeny of exploratory behavior in male and female adolescent rats ( Rattus norvegicus ). Dev. Psychobiol. 2009 , 51 , 513–520. [ Google Scholar ] [ CrossRef ] [ PubMed ] [ Green Version ]
- Boogert, N.J.; Reader, S.M.; Laland, K.N. The relation between social rank, neophobia and individual learning in starlings. Anim. Behav. 2006 , 72 , 1229–1239. [ Google Scholar ] [ CrossRef ]
- Young, J.K.; Touzot, L.; Brummer, S.P. Persistence and conspecific observations improve problem-solving abilities of coyotes. PLoS ONE 2019 , 14 , e0218778. [ Google Scholar ] [ CrossRef ] [ Green Version ]
- Drea, C.M.; Wallen, K. Low-status monkeys ‘play dumb’ when learning in mixed social groups. Proc. Natl. Acad. Sci. USA 1999 , 96 , 12965–12969. [ Google Scholar ] [ CrossRef ] [ Green Version ]
- Drea, C.M.; Carter, A.N. Cooperative problem solving in a social carnivore. Anim. Behav. 2009 , 78 , 967–977. [ Google Scholar ] [ CrossRef ]
- Aplin, L.M.; Sheldon, B.C.; Morand-Ferron, J. Milk bottles revisited: Social learning and individual variation in the blue tit, Cyanistes caeruleus . Anim. Behav. 2013 , 85 , 1225–1232. [ Google Scholar ] [ CrossRef ]
- Reader, S.M.; Laland, K.N. Primate Innovation: Sex, Age and Social Rank Differences. Int. J. Primatol. 2001 , 22 , 787–805. [ Google Scholar ] [ CrossRef ]
- Ashton, B.J.; Thornton, A.; Ridley, A.R. Larger group sizes facilitate the emergence and spread of innovations in a group-living bird. Anim. Behav. 2019 , 158 , 1–7. [ Google Scholar ] [ CrossRef ]
- Gleason, P.E.; Weber, P.G.; Weber, S.P. Effect of group size on avoidance learning in zebra fish, Brachydanio rerio (Pisces: Cyprinidae) . Anim. Learn. Behav. 1977 , 5 , 213–216. [ Google Scholar ] [ CrossRef ]
- Krasheninnikova, A.; Schneider, J.M. Testing problem-solving capacities: Differences between individual testing and social group setting. Anim. Cogn. 2014 , 1227–1232. [ Google Scholar ] [ CrossRef ] [ PubMed ]
- Manrod, J.D.; Hartdegen, R.; Burghardt, G.M. Rapid solving of a problem apparatus by juvenile black-throated monitor lizards ( Varanus albigularis albigularis ). Anim. Cogn. 2008 , 11 , 267–273. [ Google Scholar ] [ CrossRef ]
- Chow, P.K.Y.; Lea, S.E.G.; Leaver, L.A. How practice makes perfect: The role of persistence, flexibility and learning in problem-solving efficiency. Anim. Behav. 2016 , 112 , 273–283. [ Google Scholar ] [ CrossRef ] [ Green Version ]
- Tecwyn, E.C.; Thorpe, S.K.; Chappell, J. What cognitive strategies do orangutans ( Pongo pygmaeus ) use to solve a trial-unique puzzle-tube task incorporating multiple obstacles? Anim. Cogn. 2012 , 15 , 121–133. [ Google Scholar ] [ CrossRef ] [ PubMed ]
- Enoch, M.A.; Steer, C.D.; Newman, T.K.; Gibson, N.; Goldman, D. Early life stress, MAOA, and gene-environment interactions predict behavioral disinhibition in children. Genes Brain Behav. 2010 , 9 , 65–74. [ Google Scholar ] [ CrossRef ] [ Green Version ]
- Newman, T.K.; Syagailo, Y.V.; Barr, C.S.; Wendland, J.R.; Champoux, M.; Graessle, M.; Suomi, S.J.; Higley, D.; Lesch, K.-P. Monoamine Oxidase A Gene Promoter Variation and Rearing Experience Influences Aggressive Behavior in Rhesus Monkeys. Biol. Psychiatry 2005 , 57 , 167–172. [ Google Scholar ] [ CrossRef ] [ PubMed ]
- Fischer, A.; Sananbenesi, F.; Wang, X.; Dobbin, M.; Tsai, L.-H. Recovery of learning and memory is associated with chromatin remodelling. Nature 2007 , 447 , 178–182. [ Google Scholar ] [ CrossRef ]
- Wolf, J.B.; Brodie, E.D.I.I.I.; Cheverud, J.M.; Moore, A.J.; Wade, M.J. Evolutionary consequences of indirect genetic effects. Trends Ecol. Evol. 1998 , 13 , 64–69. [ Google Scholar ] [ CrossRef ]
- Strakovsky, R.S.; Zhang, X.; Zhou, D.; Pan, Y.X. Gestational high fat diet programs hepatic phosphoenolpyruvate carboxykinase gene expression and histone modification in neonatal offspring rats. J. Physiol. 2011 , 589 , 2707–2717. [ Google Scholar ] [ CrossRef ]
- Badyaev, A.V.; Uller, T. Parental effects in ecology and evolution: Mechanisms, processes and implications. Philos. Trans. R. Soc. Lond. Ser. BBiol. Sci. 2009 , 364 , 1169–1177. [ Google Scholar ] [ CrossRef ] [ Green Version ]
- Francis, D.; Diorio, J.; Liu, D.; Meaney, M.J. Nongenomic Transmission across Generations of Maternal Behavior and Stress Responses in the Rat. Sci. New Ser. 1999 , 286 , 1155–1158. [ Google Scholar ] [ CrossRef ] [ PubMed ] [ Green Version ]
- Fish, E.W.; Shahrokh, D.; Bagot, R.; Caldji, C.; Bredy, T.; Szyf, M.; Meaney, M.J. Epigenetic Programming of Stress Responses through Variations in Maternal Care. Ann. N. Y. Acad. Sci. 2004 , 1036 , 167–180. [ Google Scholar ] [ CrossRef ] [ Green Version ]
- Bredy, T.W.; Diorio, J.; Grant, R.; Meaney, M.J. Maternal care influences hippocampal neuron survival in the rat. Eur. J. Neurosci. 2003 , 18 , 2903–2909. [ Google Scholar ] [ CrossRef ]
- Liu, D.; Diorio, J.; Tannenbaum, B.; Caldji, C.; Francis, D.; Freedman, A.; Sharma, S.; Pearson, D.; Plotsky, P.M.; Meaney, M.J. Maternal Care, Hippocampal Glucocorticoid Receptors, and Hypothalamic-Pituitary-Adrenal Responses to Stress. Science 1997 , 277 , 1659–1662. [ Google Scholar ] [ CrossRef ] [ PubMed ] [ Green Version ]
- Caldji, C.; Tannenbaum, B.; Sharma, S.; Francis, D.; Plotsky, P.M.; Meaney, M.J. Maternal care during infancy regulates the development of neural systems mediating the expression of fearfulness in the rat. Proc. Natl. Acad. Sci. USA 1998 , 95 , 5335–5340. [ Google Scholar ] [ CrossRef ] [ Green Version ]
- Bredy, T.W.; Humpartzoomian, R.A.; Cain, D.P.; Meaney, M.J. The influence of maternal care and environmental enrichment on hippocampal development and function in the rat. Neuroscience 2003 , 118 , 571–576. [ Google Scholar ] [ CrossRef ]
- Herczeg, G.; Gonda, A.; Merilä, J. Predation mediated population divergence in complex behaviour of nine-spined stickleback (Pungitius pungitius) . J. Evol. Biol. 2009 , 22 , 544–552. [ Google Scholar ] [ CrossRef ] [ PubMed ]
- Gonda, A.; Herczeg, G.; Merilä, J. Habitat-Dependent and -Independent Plastic Responses to Social Environment in the Nine-Spined Stickleback ( Pungitius pungitius ) Brain. Proc. Biol. Sci. 2009 , 276 , 2085–2092. [ Google Scholar ] [ CrossRef ] [ Green Version ]
- Gonda, A.; Herczeg, G.; Merila, J. Adaptive brain size divergence in nine-spined sticklebacks ( Pungitius pungitius )? J. Evol. Biol. 2009 , 22 , 1721–1726. [ Google Scholar ] [ CrossRef ]
- Sallet, J.; Mars, R.B.; Noonan, M.P.; Andersson, J.L.; O’Reilly, J.X.; Jbabdi, S.; Croxson, P.L.; Jenkinson, M.; Miller, K.L.; Rushworth, M.F.S. Social Network Size Affects Neural Circuits in Macaques. Science 2011 , 334 , 697–700. [ Google Scholar ] [ CrossRef ] [ PubMed ] [ Green Version ]
- Hoffmann, L.C.; Schutte, S.R.M.; Koch, M.; Schwabe, K. Effect of “enriched environment” during development on adult rate behaviour and response to the dopamine receptor agonist apomorphine. Neuroscience 2009 , 158 , 1589–1598. [ Google Scholar ] [ CrossRef ] [ PubMed ]
- Berlucchi, G.; Buchtel, H.A. Neuronal plasticity: Historical roots and evolution of meaning. Exp. Brain Res. 2009 , 192 , 307–319. [ Google Scholar ] [ CrossRef ]
- Arai, J.A.; Feig, L.A. Long-lasting and transgenerational effects of an environmental enrichment on memory formation. Brain Res. Bull. 2011 , 85 , 30–35. [ Google Scholar ] [ CrossRef ] [ PubMed ] [ Green Version ]
- O’Connor, A.M.; Burton, T.J.; Leamey, C.A.; Sawatari, A. The Use of the Puzzle Box as a Means of Assessing the Efficacy of Environmental Enrichment. J. Vis. Exp. 2014 , 94 , e52225. [ Google Scholar ] [ CrossRef ] [ PubMed ] [ Green Version ]
- D’Aniello, B.; Scandurra, A. Ontogenetic effects on gazing behaviour: A case study of kennel dogs (Labrador Retrievers) in the impossible task paradigm. Anim. Cogn. 2016 , 19 , 565–570. [ Google Scholar ] [ CrossRef ] [ Green Version ]
- Shen, J.; Barnes, C.A.; McNaughton, B.L.; Skaggs, W.E.; Weaver, K.L. The effect of aging on experience-dependent plasticity of hippocampal place cells. J. Neurosci. 1997 , 17 , 6769–6782. [ Google Scholar ] [ CrossRef ] [ PubMed ] [ Green Version ]
- Smith, L.K.; White, C.W.; Villeda, S.A. The systemic environment: At the interface of aging and adult neurogenesis. Cell Tissue Res. 2018 , 371 , 105–113. [ Google Scholar ] [ CrossRef ] [ PubMed ] [ Green Version ]
- Milgram, N.W. Cognitive Experience and Its Effect on Age-Dependent Cognitive Decline in Beagle Dogs. Neurochem. Res. 2003 , 28 , 1677–1682. [ Google Scholar ] [ CrossRef ]
- Workel, J.O.; Oitzl, M.S.; Fluttert, M.; Lesscher, H.; Karssen, A.; de Kloet, E.R. Differential and Age-Dependent Effects of Maternal Deprivation on the Hypothalamic-Pituitary-Adrenal Axis of Brown Norway Rats from Youth to Senescence. J. Neuroendocrinol. 2001 , 13 , 569–580. [ Google Scholar ] [ CrossRef ] [ PubMed ]
- Frick, K.M.; Baxter, M.G.; Markowska, A.L.; Olton, D.S.; Price, D.L. Age-related spatial reference and working memory deficits assessed in the water maze. Neurobiol. Aging 1995 , 16 , 149–160. [ Google Scholar ] [ CrossRef ]
- Arakawa, H. Interaction between isolation rearing and social development on exploratory behavior in male rats. Behav. Process. 2005 , 70 , 223–234. [ Google Scholar ] [ CrossRef ]
- Biondi, L.M.; Bó, M.S.; Vassallo, A.I. Inter-individual and age differences in exploration, neophobia and problem-solving ability in a Neotropical raptor ( Milvago chimango ). Anim. Cogn. 2010 , 13 , 701–710. [ Google Scholar ] [ CrossRef ] [ PubMed ]
- Vince, M.A. “String-pulling” in birds. (2) Differences related to age in greenfinches, chaffinches and canaries. Anim. Behav. 1958 , 6 , 53–59. [ Google Scholar ] [ CrossRef ]
- Carter, A.J.; Marshall, H.H.; Heinsohn, R.; Cowlishaw, G. Personality predicts the propensity for social learning in a wild primate. PeerJ 2014 , 2 , e283. [ Google Scholar ] [ CrossRef ]
- Loepelt, J.; Shaw, R.C.; Burns, K.C. Can you teach an old parrot new tricks? Cognitive development in wild kaka ( Nestor meridionalis ). Proc. R. Soc. Lond. B 2016 , 283 , 20153056. [ Google Scholar ] [ CrossRef ] [ Green Version ]
- Pellis, S.M.; Pellis, V.C.; Bell, H.C. The function of play in the development of the social brain. Am. J. Play 2010 , 2 , 278–296. [ Google Scholar ]
- Griffin, A.S.; Diquelou, M.C. Innovative problem solving in birds: A cross-species comparison of two highly successful passerines. Anim. Behav. 2015 , 100 , 84–94. [ Google Scholar ] [ CrossRef ]
- Benson-Amram, S.; Holekamp, K.E. Innovative problem solving by wild spotted hyenas. Proc. Biol. Sci. 2012 , 279 , 4087–4095. [ Google Scholar ] [ CrossRef ] [ PubMed ] [ Green Version ]
- Prasher, S.; Evans, J.C.; Thompson, M.J.; Morand-Ferron, J. Characterizing innovators: Ecological and individual predictors of problem-solving performance. PLoS ONE 2019 , 14 , e0217464. [ Google Scholar ] [ CrossRef ] [ PubMed ]
- Brown, G.E. Learning about danger: Chemical alarm cues and local risk assessment in prey fishes. Fish Fish. 2003 , 4 , 227–234. [ Google Scholar ] [ CrossRef ]
- Lonnstedt, O.M.; McCormick, M.I.; Meekan, M.G.; Ferrari, M.C.O.; Chivers, D.P. Learn and live: Predator experience and feeding history determines prey behaviour and survival. Proc. R. Soc. B Biol. Sci. 2012 , 279 , 2091–2098. [ Google Scholar ] [ CrossRef ] [ PubMed ] [ Green Version ]
- Katz, M.; Lachlan, R.F. Social learning of food types in zebra finches ( Taenopygia guttata ) is directed by demonstrator sex and feeding activity. Anim. Cogn. 2003 , 6 , 11–16. [ Google Scholar ] [ CrossRef ]
- McCarty, R.; Kopin, I.J. Changes in Plasma Catecholamines and Behavior of Rats during the Anticipation of Footshock. Horm. Behav. 1978 , 11 , 248–257. [ Google Scholar ] [ CrossRef ]
- Dugatkin, L.A.; Godin, J.-G.J. Predator inspection, shoaling and foraging under predation hazards in the Trinidadian guppy, Poecilia reticulata . Environ. Biol. Fishes 1992 , 24 , 265–276. [ Google Scholar ] [ CrossRef ]
- Bird, C.D.; Emery, N.J. Insightful problem solving and creative tool modification by captive nontool-using rooks. Proc. Natl. Acad. Sci. USA 2009 , 106 , 10370–10375. [ Google Scholar ] [ CrossRef ] [ Green Version ]
- Range, F.; Huber, L. Attention in common marmosets: Implications for social-learning experiments. Anim. Behav. 2007 , 73 , 1033–1041. [ Google Scholar ] [ CrossRef ]
- Fragaszy, D.M.; Visalberghi, E. Social processes affecting the appearance of innovative behaviors in capuchin monkeys. Folia Primatol. 1990 , 54 , 155–165. [ Google Scholar ] [ CrossRef ]
- Heyes, C.M. Imitation, culture and cognition. Anim. Behav. 1993 , 46 , 999–1010. [ Google Scholar ] [ CrossRef ] [ Green Version ]
- Diatchenko, L.; Slade, G.D.; Nackley, A.G.; Sigurdsson, K.B.A.; Belfer, I.; Goldman, D.; Xu, K.; Shabalina, S.A.; Shagin, D.; Max, M.B.; et al. Genetic basis for individual variations in pain perception and the development of a chronic pain condition. Hum. Mol. Genet. 2005 , 14 , 135–143. [ Google Scholar ] [ CrossRef ] [ Green Version ]
- Tello-Ramos, M.C.; Branch, C.L.; Kozlovsky, D.Y.; Pitera, A.M.; Pravosudov, V.V. Spatial memory and cognitive flexibility trade-offs: To be or not to be flexible, that is the question. Anim. Behav. 2019 , 147 , 129–136. [ Google Scholar ] [ CrossRef ] [ Green Version ]
- Kappeler, P.; Kraus, C. Levels and mechanisms of behavioural variability. In Animal Behaviour: Evolution and Mechanisms ; Springer: Berlin/Heidelberg, Germany, 2010; pp. 655–684. [ Google Scholar ]
- Leal, M.; Powell, B.J. Behavioural flexibility and problem-solving in a tropical lizard. Biol. Lett. 2012 , 8 , 28–30. [ Google Scholar ] [ CrossRef ] [ PubMed ] [ Green Version ]
- Storks, L.; Leal, M. Thinking outside the box: Problem-solving in free-living lizards. Behav. Ecol. Sociobiol. 2020 , 74 , 75. [ Google Scholar ] [ CrossRef ]
- Auersperg, A.M.; Von Bayern, A.M.; Gajdon, G.K.; Huber, L.; Kacelnik, A. Flexibility in problem solving and tool use of kea and New Caledonian crows in a multi access box paradigm. PLoS ONE 2011 , 6 , e20231. [ Google Scholar ] [ CrossRef ] [ Green Version ]
- Bebus, S.E.; Small, T.W.; Jones, B.C.; Elderbrock, E.K.; Schoech, S.J. Associative learning is inversely related to reversal learning and varies with nestling corticosterone exposure. Anim. Behav. 2016 , 111 , 251–260. [ Google Scholar ] [ CrossRef ] [ Green Version ]
- Miller, R.; Laskowski, K.L.; Schiestl, M.; Bugnyar, T.; Schwab, C. Socially Driven Consistent Behavioural Differences during Development in Common Ravens and Carrion Crows. PLoS ONE 2016 , e0148822. [ Google Scholar ] [ CrossRef ] [ Green Version ]
- Sih, A.; Bell, A.M.; Johnson, J.C.; Ziemba, R.E. Behavioral syndromes: An integrative overview. Q. Rev. Biol. 2004 , 79 , 241–277. [ Google Scholar ] [ CrossRef ]
- Réale, D.; Reader, S.M.; Sol, D.; McDougall, P.T.; Dingemanse, N.J. Integrating animal temperament within ecology and evolution. Biol. Rev. 2007 , 82 , 291–318. [ Google Scholar ] [ CrossRef ] [ Green Version ]
- Couchoux, C.; Cresswell, W. Personality constraints versus flexible antipredation behaviors: How important is boldness in risk management of red shanks ( Tringa totanus ) foraging in a natural system? Behav. Ecol. 2012 , 23 , 290–301. [ Google Scholar ] [ CrossRef ] [ Green Version ]
- Koolhaas, J.; Korte, S.; De Boer, S.; Van Der Vegt, B.; Van Reenen, C.; Hopster, H.; De Jong, I.C.; Ruis, M.A.W.; Blokhuis, H. Coping styles in animals: Current status in behavior and stress-physiology. Neurosci. Biobehav. Rev. 1999 , 23 , 925–935. [ Google Scholar ] [ CrossRef ]
- Ariyomo, T.O.; Watt, P.J. Effect of hunger level and time of day on boldness and aggression in the zebrafish Danio rerio hunger level, time of day and behaviour. J. Fish Biol. 2015 , 86 , 1852–1859. [ Google Scholar ] [ CrossRef ]
- Relyea, R.A. Predators come and predators go: The reversibility of predator-induced traits. Ecology 2003 , 84 , 1840–1848. [ Google Scholar ] [ CrossRef ]
- Wat, K.K.; Banks, P.B.; McArthur, C. Linking animal personality to problem-solving performance in urban common brushtail possums. Anim. Behav. 2020 , 162 , 35–45. [ Google Scholar ] [ CrossRef ]
- Rowell, M.K.; Rymer, T.L. Personality influences problem solving in the fawn-footed mosaic-tailed rat ( Melomys cervinipes ). Ethology . in review.
- Overington, S.E.; Cauchard, L.; Côté, K.A.; Lefebvre, L. Innovative foraging behaviour in birds: What characterizes an innovator? Behav. Process. 2011 , 87 , 274–285. [ Google Scholar ] [ CrossRef ] [ PubMed ]
- Amici, F.; Caicoya, A.L.; Majolo, B.; Widdig, A. Innovation in wild Barbary macaques ( Macaca sylvanus ). Sci. Rep. 2020 , 10 , 1–12. [ Google Scholar ] [ CrossRef ] [ PubMed ]
- Krasheninnikova, A.; Bräger, S.; Wanker, R. Means-end comprehension in four parrot species: Explained by social complexity. Anim. Cogn. 2013 , 16 , 755–764. [ Google Scholar ] [ CrossRef ] [ PubMed ]
- Oritz, S.T.; Maxwell, A.; Krasheninnikova, A.; Wahlberg, M.; Larsen, O.N. Problem-solving capabilities of peach-fronted conures ( Eupsittula aurea ) studied with the string-pulling test. Behaviour 2019 , 156 , 815–846. [ Google Scholar ] [ CrossRef ]
- White, J.; Wilson, J.; Horskins, K. The role of adjacent habitats in rodent damage levels in Australian macadamia orchard systems. Crop Prot. 1997 , 16 , 727–732. [ Google Scholar ] [ CrossRef ]
- Hautier, L.; Lebrun, R.; Saksiri, S.; Michaux, J.; Vianey-Liaud, M.; Marivaux, L. Hystricognathy vs. sciurognathy in the rodent jaw: A new morphometric assessment of hystricognathy applied to living fossil Laonastes (Rodentia, Diatomyidae). PLoS ONE 2011 , 6 , e18698. [ Google Scholar ] [ CrossRef ]
- Cosmides, L.; Tooby, J. Beyond intuition and instinct blindness: Toward an evolutionarily rigorous cognitive science. Cognition 1994 , 50 , 41–77. [ Google Scholar ] [ CrossRef ]
- Tebbich, S.; Griffin, A.S.; Peschl, M.F.; Sterelny, K. From mechanisms to function: An integrated framework of animal innovation. Philos. Trans. R. Soc. B Biol. Sci. 2016 , 371 , 20150195. [ Google Scholar ] [ CrossRef ] [ PubMed ] [ Green Version ]
- Wainwright, P.E. Dietary essential fatty acids and brain function: A developmental perspective on mechanisms. Proc. Nutr. Soc. 2002 , 61 , 61–69. [ Google Scholar ] [ CrossRef ]
- Winkler, D.W.; Wrege, P.H.; Allen, P.E.; Kast, T.L.; Senesac, P.; Wasson, M.F.; Llambias, P.E.; Ferretti, V.; Sullivan, P.J. Breeding dispersal and philopatry in the tree swallow. Condor 2004 , 106 , 768–776. [ Google Scholar ] [ CrossRef ]
- Fone, K.C.F.; Porkess, M.V. Behavioural and neurochemical effects of post-weaning social isolation in rodents—Relevance to developmental neuropsychiatric disorders. Neurosci. Biobehav. Rev. 2008 , 32 , 1087–1102. [ Google Scholar ] [ CrossRef ] [ PubMed ]
- Chapman, K.M.; Weiss, D.J. Pulling to scale: Motor planning for sequences of repeated actions by cotton-top tamarins (Saguinus oedipus) . J. Exp. Psychol. Anim. Behav. Process. 2013 , 39 , 180–186. [ Google Scholar ] [ CrossRef ] [ Green Version ]
- Kuhn, H.G.; Dickinson-Anson, H.; Gage, F.H. Neurogenesis in the dentate gyrus of the adult rat: Age-related decrease of neuronal progenitor proliferation. J. Neurosci. 1996 , 16 , 2027–2033. [ Google Scholar ] [ CrossRef ] [ Green Version ]
- Wright, D.; Rimmer, L.B.; Pritchard, V.L.; Butlin, R.K.; Krause, J. Inter and intra-population variation in shoaling and boldness in the zebrafish ( Danio rerio ). J. Fish Biol. 2003 , 63 , 258–259. [ Google Scholar ] [ CrossRef ]
- Arnold, S.J.; Bennett, A.F. Behavioural variation in natural populations. III: Antipredator displays in the garter snake Thamnophis radix . Anim. Behav. 1984 , 32 , 1108–1118. [ Google Scholar ] [ CrossRef ]
- Stromswold, K. Why aren’t identical twins linguistically identical? Genetic, prenatal and postnatal factors. Cognition 2006 , 101 , 333–384. [ Google Scholar ] [ CrossRef ]
Click here to enlarge figure
Terminology | Drivers | Animal Properties | Definition | Reference |
---|---|---|---|---|
Innovation | Internal and External | Mechanical/Morphology and Cognitive | A new or modified learned behaviour not previously found in the population | [ ] |
Innovation | Internal and External | Mechanical/Morphology and Cognitive | The ability to invent new behaviours, or to use existing behaviours in new contexts A new or modified learned behaviour not previously found in the population A process that results in new or modified learned behaviour and that introduces novel behavioural variants into a population’s repertoire | [ ] |
Innovation | Internal and External | Mechanical/Morphology and Cognitive | The devising of new solutions | [ ] |
Innovation | Internal and External | Cognitive | An animal’s ability to apply previous knowledge to a novel problem or apply novel techniques to an old problem | [ ] |
Novel behaviour | Internal | Cognitive | The result of an orderly and dynamic competition among previously established behaviours, during which old behaviours blend or become interconnected in new ways | [ ] |
Physical problem solving | External | Mechanical/Morphology | Use of novel means to reach a goal when direct means are unavailable | [ ] |
Problem solving | Internal | Cognitive | Overcoming an obstacle that is preventing animals from achieving their goal immediately | [ ] |
Problem solving | External | Mechanical/Morphology and Cognitive | A problem exists when the goal that is sought is not directly attainable by the performance’ of a simple act available in the animal’s repertoire; the solution calls for either a novel action or a new integration of available actions | [ ] |
Problem solving | Internal | Cognitive | Any goal-directed sequence of cognitive operations | [ ] |
Problem solving | Internal and External | Mechanical/Morphology and Cognitive | A goal-directed sequence of cognitive and affective operations as well as behavioural responses for the purpose of adapting to internal or external demands or challenges | [ ] |
Problem solving | Internal | Cognitive | An analysis of means–end relationships | [ ] |
Problem solving | External | Mechanical/Morphology and Cognitive | A subset of instrumental responses that appear when an animal cannot achieve a goal using a direct action; the subject needs to perform a novel action or an innovative integration of available responses in order to solve the problem | [ ] |
Problem solving | Internal | Mechanical/Morphology | The ability to overcome obstacles and achieve a goal | [ ] |
MDPI stays neutral with regard to jurisdictional claims in published maps and institutional affiliations. |
Share and Cite
Rowell, M.K.; Pillay, N.; Rymer, T.L. Problem Solving in Animals: Proposal for an Ontogenetic Perspective. Animals 2021 , 11 , 866. https://doi.org/10.3390/ani11030866
Rowell MK, Pillay N, Rymer TL. Problem Solving in Animals: Proposal for an Ontogenetic Perspective. Animals . 2021; 11(3):866. https://doi.org/10.3390/ani11030866
Rowell, Misha K., Neville Pillay, and Tasmin L. Rymer. 2021. "Problem Solving in Animals: Proposal for an Ontogenetic Perspective" Animals 11, no. 3: 866. https://doi.org/10.3390/ani11030866
Article Metrics
Article access statistics, further information, mdpi initiatives, follow mdpi.
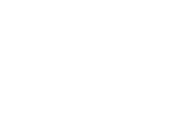
Subscribe to receive issue release notifications and newsletters from MDPI journals

An official website of the United States government
The .gov means it’s official. Federal government websites often end in .gov or .mil. Before sharing sensitive information, make sure you’re on a federal government site.
The site is secure. The https:// ensures that you are connecting to the official website and that any information you provide is encrypted and transmitted securely.
- Publications
- Account settings
Preview improvements coming to the PMC website in October 2024. Learn More or Try it out now .
- Advanced Search
- Journal List

Old and New Approaches to Animal Cognition: There Is Not “One Cognition”
Juliane bräuer.
1 Max Planck Institute for the Science of Human History, Department of Linguistic and Cultural Evolution, Kahlaische Strasse 10, 07745 Jena, Germany; ed.gpm.hhs@yarg (R.G.); moc.liamg@kcudart (N.U.)
2 Department of General Psychology, Friedrich-Schiller-University, Am Steiger 3, 07743 Jena, Germany
Daniel Hanus
3 Max Planck Institute for Evolutionary Anthropology, Department of Developmental and Comparative Psychology, Deutscher Platz 6, 04103 Leipzig, Germany; ed.gpm.ave@sunah
Simone Pika
4 Institute of Cognitive Science, Comparative BioCognition, University of Osnabrück, Artilleriestrasse 34, 49076 Osnabrück, Germany; [email protected]
Russell Gray
Natalie uomini.
Using the comparative approach, researchers draw inferences about the evolution of cognition. Psychologists have postulated several hypotheses to explain why certain species are cognitively more flexible than others, and these hypotheses assume that certain cognitive skills are linked together to create a generally “smart” species. However, empirical findings suggest that several animal species are highly specialized, showing exceptional skills in single cognitive domains while performing poorly in others. Although some cognitive skills may indeed overlap, we cannot a priori assume that they do across species. We argue that the term “cognition” has often been used by applying an anthropocentric viewpoint rather than a biocentric one. As a result, researchers tend to overrate cognitive skills that are human-like and assume that certain skills cluster together in other animals as they do in our own species. In this paper, we emphasize that specific physical and social environments create selection pressures that lead to the evolution of certain cognitive adaptations. Skills such as following the pointing gesture, tool-use, perspective-taking, or the ability to cooperate evolve independently from each other as a concrete result of specific selection pressures, and thus have appeared in distantly related species. Thus, there is not “one cognition”. Our argument is founded upon traditional Darwinian thinking, which—although always at the forefront of biology—has sometimes been neglected in animal cognition research. In accordance with the biocentric approach, we advocate a broader empirical perspective as we are convinced that to better understand animal minds, comparative researchers should focus much more on questions and experiments that are ecologically valid. We should investigate nonhuman cognition for its own sake, not only in comparison to the human model.
1. Current Hypotheses on Animal Cognition
Some of the most enduring questions in contemporary behavioral science concern which cognitive skills humans share with other animal species and which are uniquely human ( Premack and Woodruff 1978 ; Byrne 1996 ; Tomasello 2019 ). One prevalent approach to this question is the comparative approach, which pinpoints similarities and differences between human and nonhuman animals to then draw informed inferences about the evolution of human behavior and cognition ( Heyes and Huber 2000 ; Tennie et al. 2009 ; Borrego 2017 ). The term “cognition” (see Box 1 ) refers to “adaptive information processing in the broadest sense, from gathering information through the senses to making decisions and performing functionally appropriate actions, regardless of the complexity of any internal representational processes that behavior might imply” ( Shettleworth 2000, p. 43 ). According to this definition, animals receive inputs to their brain through, e.g., vision, hearing, touch, smell, taste, electric fields, air currents, or magnetic fields. The brain processes these inputs and controls behaviors. Behavior is the result of an animal’s decision to act on the inputs received. This definition of cognition encompasses all possible inputs and actions that are the result of mental processes. In this paper, we rely on this definition without distinguishing between more or less complex or sophisticated forms of cognition, as that would involve a judgment of what qualifies as “sophisticated”, which is what we want to avoid here, as we argue below. We are aware that animals may often use simple mechanisms to solve their social and physical problems, reserving the more complex mechanisms for situations in which the simpler mechanisms do not work. The important point for us is that individuals show flexible behavior and do not rely on innate or learned strategies only. In this paper, we show how this broad definition of cognition is at odds with narrow views that are still prevalent in viewing cognition as “one cognition”, in contrast to the biocentric perspective that focuses on each species in its own evolutionary history, which we support here.
Definitions used in this article.
Comparative Psychology : Investigation of similarities and differences in multiple animal species—including humans—using techniques that encompass everything from observational studies in nature to neurophysiological research in the laboratory ( Call et al. 2017 ; Tomasello and Herrmann 2010 ).
Cognition : Adaptive information processing in the broadest sense, from gathering information through the senses to making decisions and performing functionally appropriate actions, regardless of the complexity of any internal representational processes that behavior might imply ( Shettleworth 2000 ).
Physical Cognition : Knowledge of the physical world ( Tomasello and Call 1997 ).
Social Cognition : Knowledge of the social world ( Tomasello and Call 1997 ).
Animal Cognition : Describes the mental capacities of nonhuman animals and the study of those capacities. The field developed from comparative psychology, including the study of animal conditioning and learning ( Shettleworth 2000 .)
We now know that cognition comes in many forms across a huge diversity of nonhuman animal species (i.e., Shettleworth 1998 ; McMillan et al. 2015 ; Vonk 2016 ; Allen 2017 ; Call et al. 2017 ). The first studies on the complexity of animal minds were carried out by psychologists and traditionally centered on the phenomenon of learning ( Kamil 1987 ; Hanus 2016 ; Maestripieri 2003 ), for instance, in a few model species such as rats and pigeons ( Beach 1995 ; Hodos and Campbell 1969 ; Papini 2002 ). However, they were ignoring the biological context of behaviors, i.e., their potential adaptive implications (i.e., Kamil 1987 ). In a pioneering article entitled “A synthetic approach to the study of animal cognition”, Kamil ( 1987 ) argued for a broader approach to understanding animal minds and stressed two main points: (1) that the range of investigated skills needs to be expanded—i.e., to study phenomena besides learning, and (2) that researchers should consider ecological factors to situate their findings within a comparative evolutionary framework ( Kamil 1987 ; see also Shettleworth 1983 ; Bates and Byrne 2007 ; Bshary et al. 2011 ).
Since the publication of that article, researchers in the fields of comparative cognition, animal psychology, cognitive archaeology, and cognitive biology ( Shettleworth 2009 ; Fitch et al. 2010 ) have made considerable advances in tackling different cognitive and behavioral elements that form the layered system of cognition. Consequently, the range of skills and species studied has now significantly increased. In particular, in the last 20 years, there has been a growing interest in these fields (see Shettleworth 2009 for an overview), resulting in increasing citation rates from about 400 citations per year in 2000 to over 4000 citations in 2019 ( Table 1 ). The research in these fields focused on two major challenges animals have to deal with: foraging and maintaining social relationships ( Tomasello and Call 1997 ; Seyfarth and Cheney 2003 ; Seed et al. 2009 ). To date, the majority of the studies have examined distinct aspects of physical cognitive skills such as tool-use, memory, future planning, and numerosity, as well as social cognitive skills such as communication, cooperation, and social learning ( Call et al. 2017 ; Shettleworth 1998 ; Shettleworth 2010 ; De Waal and Tyack 2009 ; Reznikova 2007 ; Wasserman and Zentall 2006 ; Zentall and Wasserman 2012 ).
Citations of animal cognition papers from Web of Science, all databases 1894–2020 (accessed 25 April 2020), for the following search terms in the “topics” field: “animal cognition”, “animal psychology”, “cognitive ethology”, “comparative cognition”, “comparative psychology”.
Topic Search Term | Number of Publications | Total Citations | Total Excluding Self-Citations | Citations Per Year in 2000 | Citations Per Year in 2019 |
---|---|---|---|---|---|
animal cognition | 1202 | 18,550 | 17,760 | ||
animal psychology | 435 | 3329 | 3295 | ||
cognitive ethology | 216 | 3242 | 3051 | ||
comparative cognition | 642 | 8379 | 7822 | ||
comparative psychology | 1376 | 13,496 | 12,587 | ||
Kamil’s ( 1987 ) first suggestion was heeded. However, Kamil’s ( 1987 ) second suggestion, to adopt a biocentric view of cognition by considering ecological factors, which is quite natural for biologists, has been at times neglected by psychologists ( Sewall 2015 ; Macphail 1982 ; Eaton et al. 2018 ; Vasilieva 2019 ). We see two main problems that hinder current research in comparative psychology. Firstly, an anthropocentric approach dominates research ( Shettleworth 2010 ). Secondly and relatedly, cognition is viewed as a cluster of skills that evolve together, as in humans. We see that these positions are still prevalent in comparative psychology ( Vasilieva 2019 ).
In particular, psychologists have postulated several prominent hypotheses to explain why certain species are considered “intelligent”. These hypotheses assume that cognitive skills are linked together to create a broad (or universal) instantiation of intelligence, often termed cognitive flexibility (for an overview, see Allen 2017 ). For instance, the Social Intelligence Hypothesis —also termed the Machiavellian Intelligence Hypothesis or Social Brain Hypothesis ( Dunbar and Shultz 2007 ; Humphrey 1976 ; Jolly 1966 ; Byrne and Whiten 1988 )—seeks to explain the origins of primate intelligence in their sociality. It predicts that natural selection favored those individuals living in complex social environments, such as fission–fusion societies, for their ability to deal with the frequent unpredictable situations that occur in social interactions in such societies. Thus, intelligence is triggered by the demands and complexity of sociality. In a similar vein, the Cognitive Buffer Hypothesis predicts that large brains facilitate the construction of behavioral responses to unusual, novel, or complex socioecological challenges. This buffer effect should increase survival rates and favor a longer reproductive life, thereby compensating for the costs of delayed reproduction ( Sol 2009 ). The Domestication Hypothesis ( Hare and Tomasello 2005 ; Hare et al. 2012 ) proposes that selection for reduced aggression in some species, such as in domesticated species like dogs, but also in wild bonobos ( Pan paniscus ), caused a set of cognitive changes, including increases in levels of social tolerance, sensitivity to social cues, cooperation, risk aversion, occurrence of juvenile behaviors, and reduction of spatial memory. Similar to the Social Intelligence Hypothesis, the Domestication Hypothesis argues that a whole suite of cognitive skills was triggered by a single factor. The Cooperative Breeding Hypothesis ( Burkart et al. 2016 ; Burkart and van Schaik 2010 ; Burkart et al. 2009 ) considers the practice of cooperative breeding to have caused a “cascade” of effects on cognition such as changes in general intelligence, language, prosociality and social tolerance, teaching, and tool-making skill. It proposes that human cognitive abilities were amplified by our prosocial tendencies, again placing sociality at the center of a suite of changes. The Technical (or Physical ) Intelligence Hypothesis proposes that great apes’ complex food manipulations using tools caused the emergence of an ability for “flexible plan-building”, which involves a representational ability that could then be applied to living entities like conspecifics ( Byrne 1997 ). This ability allowed great ape ancestors to view other individuals as intentional agents, and thus to engage in social manipulations involving complex planning. Hence, the Technical Intelligence Hypothesis also predicts that tool-use and sociality are linked by a shared ability for flexible action planning.
This brief survey of selected major hypotheses exemplifies the problematic assumption that “intelligence” results from a cluster of cognitive skills that are linked together and are elicited by single evolutionary conditions and factors that create a set of selection pressures. All these hypotheses seek to explain why cognitive skills have evolved in particular species, but not in others—there is evidence for all of the hypotheses presented above, but they do not explain the whole picture. The data supporting these hypotheses have been discussed elsewhere (i.e., Burkart et al. 2016 ). However, cognitive arrays are the result of species-typical adaptions to their whole ecological and social environments ( Burkart et al. 2016 ).
Hence, the aim of the current paper is to counteract an overly simplistic reading of these hypotheses by emphasizing the cases that contradict them, showing that cognitive skills are often not linked together.
The related problem which arises from the “one cognition” assumption is that, implicitly or explicitly, the presented hypotheses consider human cognition as the maximum and standard capacity ( Vasilieva 2019 ). This idea is exemplified in cognitive niche construction theory, which considers human cognition to have evolved into the most flexible and adaptable form of intelligence due to a runaway feedback process of cumulative culture and developmental plasticity ( Sterelny 2003 ; Laland et al. 2000 ). Cognitive abilities of target species are subsequently compared and measured according to whether and how much they match the suite of human abilities. Historically, comparison against human standards was one of the original roles of animal cognition research derived from comparative psychology ( Beran et al. 2014 ; Shettleworth 1998 ; Kamil 1987 ; Box 1 ). As interest in other minds shifted from humans to nonhuman species, methods of human psychology were often transferred to other animals. However, this approach can only produce a restrictive, anthropocentric view of cognitive evolution that ignores the incredible diversity of cognitive skills present in the world ( Bates and Byrne 2007 ). On the contrary, considering each species in its own right—in accordance with traditional Darwinian thinking ( Darwin 1859 )—allows us to reveal the evolutionary, developmental, and environmental conditions that foster the growth of certain unique abilities in the young of a species, or the convergence of skills shared among species ( Griffiths and Stotz 2000 ; Shettleworth 2009 ; Shettleworth 2010 ). The core of this biocentric view is expressed in Darwin’s metaphor: “Nature [selects] only for that of the being which she tends. Every selected character is fully exercised by her, and the being is placed under well-suited conditions of life.” ( Darwin 1859, p. 83 ).
We believe the criticism raised by Shettleworth in 2010 is still valid today: “Although the extent of human–animal cognitive similarity is undoubtedly a key issue for comparative psychology, it sometimes seems the agenda is to support anthropomorphic interpretations” ( Shettleworth 2010, p. 2 ). One example is the investigation of the understanding of the human pointing gesture. There are a plethora of comparative studies in which animals are required to use human gestures—mainly the pointing gesture—to locate hidden food (reviewed by Krause et al. 2018 ; i.e., Bräuer et al. 2006 ). From a biocentric point of view, it does not make much sense to ask whether, for example, a nonhuman ape follows a human gesture as this task is not ecologically relevant to the ape. Firstly, humans are not relevant; secondly, although apes produce pointing gestures to communicate with humans in captivity ( Leavens et al. 1996 ; Halina et al. 2018 ), they rarely inform others about external events in the wild ( Burrows et al. 2013 ). Thirdly, due to their competitive social systems, nonhuman apes would not be expected to inform others about the location of hidden food ( Sterck et al. 1997 ; Wittig and Boesch 2003 ; Bräuer et al. 2006 ). Not surprisingly, for biologists, it turned out that apes and most of the animal species tested did not reliably follow the human pointing gesture to locate hidden food unless they were enculturated (i.e., had a lot of intense experience with humans) or belonged to a species that was domesticated. It is interesting that domesticated animals follow the pointing gesture, but it is not surprising that apes have problems doing so, given their social, ecological, and evolutionary backgrounds. Similarly, the studies of language-trained apes (reviewed in Gillespie-Lynch et al. 2014 ) give us some insight into what these animals are capable of with a large amount of training, although during evolution, there was no selection pressure to communicate with humans. Thus, these studies do not tell us much about apes, but rather about humans’ specially evolved skills such as language ( Morgan et al. 2015 ; Uomini and Meyer 2013 ; Uomini 2009 ; Uomini 2014 ; Uomini 2017 ; Uomini and Ruck 2018 ). Instead, these studies can tell us what these species are able to learn about situations they do not encounter naturally. For example, if some chimpanzee individuals can learn such “unnatural” skills as following the human pointing gesture or using a lexigram language, this shows us chimpanzees’ cognitive flexibility and can help us to understand the factors leading to innovation and their ability to learn and generalize. In contrast, the aim of a less anthropocentric approach should be to investigate their natural forms of communication and not to force them to use human-like communication.
In this paper, we discuss two related problems, namely, the assumption that cognition evolves as a cluster of skills as in humans, and the anthropocentric approach, following the criticisms raised by Shettleworth ( 1998 , 2009 , 2010 ). However, despite the subsequent progress noted by Shettleworth ( 2009 ), we still consider it necessary to advocate for a broader perspective on cognitive evolution. To be clear, we do not argue against comparative psychology as a valid discipline to gain insights into animal cognition; rather, we argue against how comparisons between humans and other animals are carried out by favoring the anthropocentric issues mentioned above. If we want to account for the fascinating variety of animal minds, comparative scientists should focus on skills that are ecologically relevant for a given species ( Section 2 ), as well as skills in which humans are outperformed by other animals ( Section 3 ). Moreover, the experimental operationalization of a research question should be ecologically valid, i.e., using naturalistic situations with relevant test settings that match naturally occurring contexts and—most importantly—the modality must be relevant for the tested species ( Section 4 ). By eschewing the traditional anthropocentric approach and turning our attention to skills that humans either do not excel in or do not possess, we are better positioned to advance the science of animal cognition ( Cantlon and Hayden 2017 ).
2. Performing Competently–Performing Poorly: Cognitive Skills Are Not Necessarily Linked Together
To illustrate the pitfalls of the anthropocentric approach, let us briefly consider human cognition as unique (i.e., MacLean 2016 ; Tomasello 2019 ; Sterelny 2003 ) and as the maximum capacity ( Pinker 2010 ; Suddendorf and Busby 2003 ; Jiang et al. 2018 ). In this case, we would assume that our closest living relatives, great apes, would show cognitive skills similar to humans, whereas less related species from other clades would underperform. However, numerous empirical findings of the past decades confirm that not only apes but also several other previously underestimated animal species demonstrate unexpected cognitive skills. Several bird species, for instance, show skills comparable to nonhuman primates in tasks concerning object permanence, delay of gratification, causal reasoning, theory of mind, and mental time travel (see Güntürkün and Bugnyar 2016 and McMillan et al. 2015 for reviews). As an example, western scrub jays (Aphelocoma californica ) hide food caches for future consumption, steal others’ caches, and engage in tactics to minimize the chance that their own caches will be stolen ( Dally et al. 2006 ). They also show spontaneous future planning behavior without reference to their current motivational state ( Raby et al. 2007 ). Similarly, two more recent studies revealed that ravens ( Corvus corax ) seem to plan for the future by saving tools for future use and tokens for future bartering ( Kabadayi and Osvath 2017 ; but see Redshaw et al. 2017 for an alternative interpretation). They also attribute visual access to unseen competitors ( Bugnyar et al. 2016 ). Moreover, in a study on the natural communication abilities of ravens in the wild, Pika and Bugnyar ( 2011 ) showed that ravens use an extremely rare form of attention-getters, a communicative ability previously confined to primates only. Furthermore, there is evidence for flexible cognitive skills in fish (see Patton and Braithwaite 2015 ; Bshary and Brown 2014 for reviews). Examples include the ability to use transitive inference—i.e., to conclude that if A > B and B > C then A > C—in cichlid fish ( Grosenick et al. 2007 ), numerical competence to track shoal size in shoaling fish ( Agrillo et al. 2012 ), updating rules to decide whether and from whom to learn about the location of food sources in nine-spined sticklebacks ( Pike and Laland 2010 ), and interspecific collaborative hunting in coral reef fishes ( Vail et al. 2013 ). Additionally, in reptiles ( Matsubara et al. 2017 ), insects ( Feinerman and Korman 2017 ; Webb 2012 ) and nonprimate mammals, there are new findings of unexpected cognitive skills such as social learning and face discrimination in domestic pigs ( Veit et al. 2017 ; Wondrak et al. 2018 ) or size and shape discrimination in horses ( Tomonaga et al. 2015 ). Data from social carnivores show that they are capable of “numerically assessing” the odds during aggressive encounters and only engage in aggression when the odds are favorable or the resource value is high ( McComb et al. 1994 ; Benson-Amram et al. 2011 ; see also Borrego 2017 ). Finally, elephants have extremely large-scale and long-lasting memories ( Hart et al. 2008 ; Polansky et al. 2015 ), and elephants show olfactory discrimination at least equal to dogs ( Arvidsson et al. 2012 ).
In addition to discoveries of surprising cognitive abilities in nonhumans, recent studies have also shown that animals that appear highly sophisticated in one cognitive domain often perform poorly in another. In the next paragraphs, we will illustrate this point by summarizing recent findings on three different, distantly related species on which we are experts ( Figure 1 ): chimpanzees ( Pan troglodytes ), domestic dogs ( Canis familiaris ), and New Caledonian crows ( Corvus moneduloides ). Chimpanzees are one of two closest living relatives to humans, and due to this shared phylogenetic trajectory, they are expected to share many cognitive skills with humans. Dogs have a long domestication history with humans, in which they have evolved some special skills. New Caledonian crows show sophisticated tool manufacturing skills and provide an example of convergent evolution of cognitive skills ( Hunt and Uomini 2016 ; see also below). These species are fairly well-studied, providing us with enough data to illustrate the main point of the present paper—that there is not always “one cognition”.

Clockwise from top left: chimpanzee, domestic dog, and New Caledonian crow participating in our research. Photographs by Simone Pika, Juliane Bräuer, and Natalie Uomini, respectively.
2.1. Chimpanzee Cognition
Chimpanzees have a very rich set of cognitive skills, and concerning the physical domain, often perform similarly to human children in captive settings (see, for example, Herrmann et al. 2007 ; Hanus et al. 2011 ). These findings renewed debates and theories about how human and chimpanzee cognitions differ (i.e., Tennie et al. 2009 ; Herrmann et al. 2007 ; Tomasello et al. 2005 ; Premack and Woodruff 1978 ; Kellogg and Kellogg 1933 ). Recent studies have shown that chimpanzees can also solve social problems using skills, such as mind-reading, that were previously thought to be uniquely human (i.e., Krachun et al. 2009 ; Call and Tomasello 2008 ). Chimpanzees seem to operate—at least on an implicit level—with an understanding of false beliefs, as they reliably look in anticipation of an agent acting on a location where an object is falsely believed to be hidden, even though the chimpanzees know that the object is no longer there ( Krupenye et al. 2017 , 2016 ; Kaminski et al. 2008 ). Moreover, chimpanzees are aware of others’ visual perspectives ( Figure 1 ) to target information toward ignorant group members ( Crockford et al. 2017 ; Crockford et al. 2012 ) and seem to plan for the future by building their night nests in the direction of their anticipated feeding tree the next morning ( Janmaat et al. 2014 ). They communicate in referential ways and show similarities to human conversational turn-taking ( Pika and Mitani 2006 ; Pika et al. 2018 ). In other social domains, chimpanzees also show remarkable behaviors comparable to humans; for example, they incur costs to watch the punishment of antisocial others ( Mendes et al. 2018 ).
Thus, the findings on chimpanzee cognition strongly support the Social Intelligence Hypothesis as this species lives in fission–fusion societies and faces selection pressure for general cognitive flexibility to deal with frequent unpredictable situations. However, although they show great flexibility in many distinct tasks, chimpanzees do not outperform other species from less complex societies in all cognitive domains. For instance, chimpanzees are only able to use pointing cues to locate hidden food in competitive, rather than cooperative, experimental tasks ( Bräuer et al. 2006 ; Herrmann and Tomasello 2006 ; Hare and Tomasello 2004 ). This limitation seems to be related to the fact that cooperative pointing does not play a dominant role in their social environment ( Bräuer et al. 2006 ; Hare and Tomasello 2004 ; see above) and is instead restricted to specific contexts and social settings (e.g., Pika and Mitani 2006 ; Pika 2014 ). Another study showed that captive chimpanzees are outperformed in inhibition tasks by orangutans ( Pongo abelii) ( Vlamings et al. 2010 ) that live semi-solitary. This difference may be due to chimpanzees being strongly attracted by the food, as they face much stronger food competition with group mates in their natural environments than do orangutans ( Vlamings et al. 2010 ; Pusey and Schroepfer-Walker 2013 ; Goodall 1986 ). Thus, although living in fission–fusion societies might have created selection pressures for the development of a number of cognitive skills in chimpanzees, other skills such as inhibition may have been less adaptive. Indeed, it is conceivable that the pressure to react quickly in social situations with high competition was more adaptive for chimpanzees than the development of inhibition skills. Future studies should focus more on socially and ecologically relevant needs for chimpanzees. Studies could, for example, address how the short reaction times of chimpanzees influence their behavior in rapidly-changing social interactions ( Inoue and Matsuzawa 2007 ).
2.2. Dog Cognition
The domestic dog was domesticated about 30,000 years ago ( Botigué et al. 2017 ; Thalmann et al. 2013 ) and shows outstanding skills in the social-cognitive domain (see Kaminski and Marshall-Pescini 2014 ; Miklosi 2007 ; Huber 2016 for reviews). These skills involve, in particular, the way dogs communicate with humans ( Figure 1 ; Kaminski et al. 2004a ; Kaminski et al. 2012 ; Kaminski et al. 2009a ), their sensitivity to human attention and perspectives ( Kaminski et al. 2017 ; Kaminski et al. 2009b ; Call et al. 2003 ; Bräuer et al. 2013b ), and their motivation to cooperate with humans (i.e., Bräuer et al. 2013a ; Bräuer 2015 ; Piotti and Kaminski 2016 ; but see also Quervel-Chaumette et al. 2016 ; Marshall-Pescini et al. 2016 ). In contrast, dogs do not show exceptional physical cognitive skills but perform similarly to other nonprimate mammals and birds ( Bräuer et al. 2006 ; Erdohegyi et al. 2007 ; Osthaus et al. 2005 ; Rooijakkers et al. 2009 ; Miletto Petrazzini and Wynne 2016 ). In some tasks, dogs are outperformed by wolves ( Canis lupus ), their closest living relatives, who are able to use causal cues to locate hidden food ( Lampe et al. 2017 ). These findings have been explained as ancestral dogs experiencing selection pressures for cognitive flexibility only in the social domain, as they have adapted to function effectively in human society ( Kaminski and Marshall-Pescini 2014 ). In comparison to wolves, dogs faced new challenges and thus may have acquired new social skills while losing those skills related to independent problem-solving and understanding their physical environment, skills that were critical for survival in the wild ( Lampe et al. 2017 ). However, even within the social domain, there is evidence for wolves outperforming dogs. For instance, a number of studies showed that dogs cooperate poorly with each other ( Bräuer et al. 2013c ; Dale et al. 2016 ; Marshall-Pescini et al. 2017 ), suggesting that dogs were selected to cooperate specifically with humans ( Bräuer et al. 2013a ; Bräuer 2015 ; Range et al. 2019 ). In other words, the specific social environment of the domestic dog created a specific selection pressure for a specific cognitive skill such as the ability to cooperate with humans , but not for cooperative ability in general. This should be investigated further.
In sum, areas in which dogs show outstanding cognitive skills and outperform all other species—such as the ability to communicate with humans—are not necessarily linked together with other cognitive skills. Although dogs are more socially tolerant, more cooperative, and more sensitive to social cues than wolves, as predicted by the Domestication Hypothesis, the abovementioned findings go against predictions of the Social Intelligence Hypothesis or the Cooperative Breeding Hypothesis. Consequently, it is more likely that these abovementioned skills evolved independently from each other.
2.3. New Caledonian Crow Cognition
A disconnection between cognitive abilities has also been shown in a member of the corvid family. New Caledonian crows are renowned for their technological abilities ( Weir et al. 2002 ; Taylor et al. 2007 ; Hunt and Gray 2004 ; Rutz et al. 2010 ; Hunt and Uomini 2016 ; Uomini and Hunt 2017 ). They not only use stick, stem, and grass tools in their natural environments ( Figure 1 ) but also manufacture pandanus tools following templates to produce specific tool shapes that vary between populations and between individuals ( Hunt and Gray 2004 ; Kenward et al. 2006 ; Taylor et al. 2012b ). According to the Technical Intelligence Hypothesis (see above; Byrne 1997 ), one would predict that the New Caledonian crow, as a tool-making species, should possess enhanced physical cognition and should outperform closely related species in physical problem-solving tasks. However, evidence for enhanced abilities beyond their exceptional technological skills is still uncertain (see Taylor and Gray 2014 for a review). In the tool-using woodpecker finch ( Cactospiza pallida ), there was no difference in physical cognition between tool-using and non-tool-using individuals, when tested on non-tool physical tasks such as a movable perch that caused a food reward to be released or a puzzle box with a lid ( Teschke et al. 2011 ). However, a follow-up study that tested woodpecker finches, New Caledonian crows, and related non-tool-using species from each clade found that non-tool-using small tree finches ( Camarhynchus parvulus ) performed equally to woodpecker finches. In contrast, New Caledonian crows outperformed carrion crows; the authors attribute these differing results to the more sophisticated tool skills of New Caledonian crows compared to woodpecker finches ( Teschke et al. 2013 ). Therefore, it is possible that very elaborated tool skills and the related enhanced physical cognition can be found in New Caledonian crows. In particular, to resolve this question, observational data from wild crows are needed, because all of the data currently available on New Caledonian crows are from experiments in captive settings using artificial (human-created) tasks. In future studies, increased attention to observational data on wild crows can help to greatly improve ecological validity for this species, as called for by Kamil ( 1987 ), and as we discuss in Section 4 .
Although New Caledonian crows may have a better understanding than related bird species of how to use metatools (i.e., the ability to use one tool on another tool; Taylor et al. 2007 ; Kenward et al. 2006 ; Gruber et al. 2019 ), they do not outperform them in other physical cognition tasks. So far, in captive setups, all corvid species tested have been found to show equal skills in the Aesop’s fable paradigm, a task designed to assess causal understanding of water displacement, in which subjects must discover how to drop stones into a water-filled container to raise the water level in order to obtain a floating reward ( Logan et al. 2014 ), as well as in hook manufacture ( Weir et al. 2002 ; Taylor et al. 2012b ; Laumer et al. 2017 ), and trap-tube tasks ( Taylor et al. 2009 ; Tebbich et al. 2007 ; Seed et al. 2006 ). In perceptual feedback studies, New Caledonian crows show the same understanding of the problem as keas ( Nestor notabilis ) and ravens, but they do not solve most string-pulling tasks as fully as keas and ravens, leading Taylor and colleagues (2010) to conclude that New Caledonian crows fail to perceive connectivity ( Taylor et al. 2012a ; Werdenich and Huber 2006 ; Taylor et al. 2010 ). The complex tool-using behaviors of New Caledonian crows also possibly do not enable them to make causal interventions, i.e., to learn a cause–effect relationship and then act to take advantage of that cause ( Taylor et al. 2014 ; but see Jacobs et al. 2015 for an opposite viewpoint). Finally, these crows—although they can learn to produce collaborative behaviors to obtain food rewards in experimentally trained settings—do not understand the causality of cooperation, leading Jelbert et al. ( 2015 ) to conclude that the flexible use of physical tools does not necessarily enable animals to grasp that a conspecific can be used as a social tool ( Jelbert et al. 2015 ). However, relatedness likely plays a role in the motivation to cooperate in this species, as New Caledonian crows are thought to spend most of their time in extended family groups ( Holzhaider et al. 2011 ). If the crows understand that a conspecific can be used as a social tool only after direct experience with that individual, we would predict that the crows should be more likely to cooperate with kin than non-kin, particularly with kin who are already collaboration partners. To determine what factors underlie cooperative performance, future studies would need to test pairs of individuals of known relatedness, as well as to document the range of their cooperative behaviors in the wild.
Regarding other social cognitive skills, experimental results on social learning are consistent with the spontaneous behaviors documented in the wild. Logan and colleagues (2016) tested social transmission of various methods to open puzzle boxes and found that New Caledonian crows of all ages learned socially by stimulus enhancement ( Logan et al. 2016 ). Similarly, long-term developmental observations in the wild showed that juvenile New Caledonian crows relied mostly on scaffolded individual learning—with templates as guidance to the final form of the tools—to develop their tool-making sequences; they produced the same tool types they saw being used and discarded by their parents, but they did not always produce the same variants as their parents ( Holzhaider et al. 2010a ; Holzhaider et al. 2010b ). More developmental studies are needed to establish the variability and consistency in the social learning of New Caledonian crows ( Uomini et al. 2020 ), but in comparison to other birds (i.e., Auersperg et al. 2014 ), so far they do not seem to perform especially well in social learning tasks.
In sum, the New Caledonian crow appears to be a species with excellent tool-related cognition but not outstanding social cognitive skills. However, it is not yet clear from the currently limited evidence if differences between New Caledonian crows and other species will become apparent when more nuanced tasks are used that more closely match the cognitive requirements of tool-use and tool-manufacture ( Taylor and Gray 2014 ). At present, it appears that the selection pressures leading to the outstanding tool behaviors of New Caledonian crows did not foster the emergence of enhanced social skills (regarding cooperation and social learning), again suggesting that cognitive skills evolve in a domain-specific manner, often independently from each other ( Macphail 1982 ).
3. When Animals Outperform Humans
As humans, our collective reluctance to acknowledge exceptional cognitive skills in nonhuman animals is at odds with the biological study of other species (i.e., Allen and Trestman 2017 ). It seems we are accustomed to accepting animal supremacy in anatomical features or physical performances: many mammalian species are bigger, stronger, or faster than humans, and we readily apply ecological explanations to determine such specific evolutionary adaptations. Interestingly, however, we do not appear willing to apply the same explanatory rigor in seeking specific selection pressures or species-typical ecological affordances when it comes to cognitive abilities. No physiologist would consider human respiration or digestion as a particularly useful reference point to describe or understand the variety and complexity of animal metabolism, but many psychologists still appear to mark human cognition as the pivotal point from which any comparison with nonhuman systems ought to start ( Shettleworth 2010 ; Premack 2007 ; Vonk 2016 ). It is therefore not surprising that the term “cognition” (and “intelligence”, respectively), even in its broadest definition, is traditionally closely connected to “human cognition” (or “human intelligence”). As a result, we tend to overrate those cognitive skills that are human-like (see also Vonk and Povinelli 2012 ) and—what turns out to be scientifically more fatal—we run the risk of overlooking cognitive skills that play only a minor role or no role in human psychology. Furthermore, it is no surprise that we expect (and find) more similar forms of cognition in phylogenetically closely related species (i.e., Balda et al. 1996 ) and assume human-like clustering of cognitive abilities in other species. To be clear, we do not argue that cognitive abilities do not cluster at all in other taxa or species, but rather we doubt the presumption that they always cluster the way they do in humans. In this section, we review the outstanding skills of animals that sometimes outperform humans. These examples illustrate our point that humans cannot be considered “superior” to other animals, but rather that each species has its own cognitive specialisms, which may be unique or exceptionally elaborated within the animal kingdom.
In the following paragraphs, we describe just a few of countless examples of animal cognition that seem astonishing in comparison with human skills, but only when human cognitive performance is considered to be the highest possible level. However, in reality, these examples simply reflect species-typical behavioral repertoires.
Adult humans have been described as exceptionally patient, especially in contrast to other primate species, which are traditionally described as more impulsive and present-oriented ( Tobin et al. 1996 ; Roberts 2002 ). However, recent work has shown that the assumed phylogenetic gap between human and nonhuman inhibitory skills is strongly influenced by specific parameters of the testing situation. Rosati and colleagues (2007), for instance, demonstrated that human patience drastically decreased when the relevant currency was food instead of money—as in the vast majority of previous human experiments—but also that chimpanzees and bonobos were much more patient than any other animal species tested so far. Chimpanzees also outperformed bonobos as well as human adults in a “food-waiting-paradigm” ( Rosati et al. 2007 ). The intra- Pan difference is especially interesting as it directly relates to different ecological affordances of those two closely related species. Whereas wild chimpanzees have to deal with rather unpredictable and unstable fruit and meat resources ( Wrangham et al. 1998 ), bonobos live in rather stable forest environments with comparatively stable and predictable food patches ( Boesch et al. 2002 ; Furuichi et al. 1998 ). In particular, hunting and extractive technologies (e.g., nut-cracking)—two behavioral peculiarities of chimpanzees with major nutritional benefits—are rare or nonexistent in bonobos ( Surbeck and Hohmann 2008 ; Boesch and Boesch-Achermann 2000 ; Mitani and Watts 2001 ). Habitat variation seems, therefore, a plausible explanation for the cognitive differences found between the two Pan species in the social and physical domains ( Rosati et al. 2007 ; Rosati 2017 ; Rosati and Hare 2011 ). In sum, human patience is not as exceptional as previously thought.
Humans are also thought to be the most rational of all primates. Contrary to what is predicted by traditional economic models, however, humans are far from being rational maximizers when it comes to resource distribution in a social scenario. Instead of pure self-interest, their decision-making seems to be strongly affected by fairness concerns, which lead, in certain situations, to (seemingly) less rational decisions. Using the experimental paradigm of the Ultimatum Game, robust findings from several laboratories and multiple human cultures confirm the assumption that adult humans are willing to pay a cost by rejecting offers that they consider unfair (e.g., Fehr and Gächter 2002 ). In contrast, confronted with an adapted ape-version of the task, chimpanzees appear more rational than humans by accepting any non-zero offer and therefore maximizing their benefits more efficiently ( Jensen et al. 2007 ). It is crucial to note that “rational” does not necessarily translate to “adaptive” here, even though it seems prima facie disadvantageous to prefer a zero outcome over a non-zero outcome just to punish a violation of a fairness principle. What appears rational and clever in the chimpanzee case is simply the most successful strategy in a social system that does not have strong other-regarding concerns of equality or fairness. In human societies, on the other hand, it might be highly adaptive to pay short-term costs to ensure fair future interactions. Such investment might pay off in the long run, given social systems with omnipresent implicit fairness expectations and explicit fairness norms.
Other than apes, several nonmammalian species show extraordinary cognitive performances that are comparable to those of human experts or even beyond. In order to navigate and communicate, birds have evolved considerable information-processing capabilities ( McMillan et al. 2015 ). For example, many raptor bird species (e.g., eagles, hawks) are equipped with remarkable visual perception and classification abilities. Experiments with pigeons demonstrated that they can recognize different letters of the alphabet, can classify images based on animal taxa criteria (e.g., cats vs. dogs) or physical features of inanimate objects (chairs vs. tables), and are able to distinguish between Monet and Picasso paintings after some period of training ( Watanabe 2001 ; Watanabe et al. 1995 ; Emery 2006 ). Results from a recent study even suggest that pigeons can be trained to “detect” cancer. The task consisted of classifying histopathological images as well as mammograms as either benign or malignant. Pigeons were able to generalize from training stimuli to new exemplars, and the performance level of the birds reached that of experienced human pathologists ( Levenson et al. 2015 ). These skills seem impressive to a human observer, even though they demonstrate nothing but ordinary pattern recognition skills—a rather specific adaptation of pigeons. Similarly, it was shown that pigeons rely on a more efficient process than humans to visually identify objects presented in various spatial orientations. This difference is presumably rooted in the differing ecological demands placed on the visual systems of flying birds compared to earth-bound humans ( Hollard and Delius 1982 ; McMillan et al. 2015 ).
Pigeons also outperform humans in other tasks. For instance, Herbranson ( 2012 ) compared pigeons and humans in a probability puzzle, i.e., the Monty Hall Dilemma. Subjects were given a choice from among three doors, one of which concealed a valuable prize. After an initial selection, one of the remaining, nonwinning doors was opened, and the participant was given a chance to switch to the other unopened door. The probability of winning is higher if the participant switches. Pigeons maximized their wins by switching on nearly all trials of a Monty Hall Dilemma analog, whereas humans utilized a suboptimal strategy involving probability matching ( Herbranson 2012 ). One possible reason why humans used probability matching is that they were searching for a strategy that would be correct 100% of the time, whether or not that level of accuracy can actually be attained. This human use of probability matching might reflect an active search that progresses even when there are no consistent patterns to be found ( Gaissmaier and Schooler 2008 ; Herbranson 2012 ). This idea is supported by another study in which rats and humans were trained in rule-based and information-integration category-learning tasks with visual stimuli. The generalization performance of rats and humans was equal in rule-based categorization, but rats outperformed humans on generalization in the information-integration task. While the performance of the rats was consistent with a nondimensional, similarity-based categorization strategy, humans again showed a bias toward rule-based strategies, which in that case impeded their performance on generalization tasks ( Vermaercke et al. 2014 ).
Humans are also outperformed in spatial memory tasks, in particular by specific bird species. The most impressive spatial memory has been demonstrated by members of food-storing bird families like parrots and corvids (see Shettleworth 1983 ; for overviews, see Clayton et al. 2006 ; Clayton and Emery 2002 ). Some of these bird species (e.g., Clark’s nutcracker Nucifraga columbiana and marsh tit Poecile palustris ) are able to remember over 100 cache sites after time delays of several months, by far exceeding the average human memory. Remarkably, not only can bird species that cache food for themselves (e.g., marsh tits) correctly remember the hiding locations they visited before, but so can species that are specialized in cache pilfering. Great tits, for example, are capable of memorizing caching locations just by “secretly” observing marsh tits caching ( Urhan et al. 2017 ; Balda and Kamil 1992 .)
What all these remarkable animal performances have in common is that they fascinate and puzzle human observers at the same time. The fascination comes from the fact that these demonstrated abilities are comparable or even superior to those of our own species within the same domains. They are puzzling because these are cognitive domains, and some aspects of cognition were traditionally thought to represent a unique characteristic of human minds that distinguishes us from other animals more than any other trait ( Premack 2007 ; Uomini 2008 ; Shettleworth 2012 ; Uomini 2014 ; MacLean 2016 ; Uomini and Ruck 2018 ; Uomini et al. 2020 ). From an anthropocentric perspective, such skills would need an explanation because they challenge human superiority, but from a genuine biological perspective, such skills are simply examples of the unique traits that each species has evolved due to specific situations and needs. Just as physical traits (e.g., an elephant’s trunk, life history, or a digestive system that can process poisonous leaves) are considered in their evolutionary context, cognitive traits should similarly be considered according to their species-specific context.
4. Ecology, Perception, and Crucial Limitations
In addition to the fact that research approaches in animal cognition are often anthropocentric and driven by our own cognitive skills, we see another problem with the current comparative character of the field. The usual approach is to present the same task to different species. This strategy is problematic when the experimental task is not equally relevant to each of the compared species ( Boesch 2007 ; Tomasello and Call 2008 ; MacLean et al. 2012 ; Roth et al. 2019 ). One classic example is the question of whether animals, chimpanzees in particular, can take the visual perspective of others. In a number of studies in which chimpanzees had to beg for food from humans, they did not show outstanding perspective-taking skills ( Povinelli and Eddy 1996 ; Kaminski et al. 2004b ). However, begging from humans is far from being a natural situation for a chimpanzee, which led Hare and colleagues to create a competitive situation in which two chimpanzees compete over a food resource. Given such a new and ecologically relevant situation, chimpanzees suddenly knew what others can see ( Hare et al. 2000 ; Bräuer et al. 2007 ) and even understood what others have seen (i.e., that seeing leads to knowing; Hare et al. 2001 ; Hare 2001 ). Kaminski and colleagues (2008) followed up the idea of a competitive and, therefore, relevant situation and tested pairs of chimpanzees in a setup in which they sat opposite to each other and competed over two pieces of food on a sliding table that was pushed back and forth. They found that chimpanzees understood what their rival knows, but not what the rival believes ( Kaminski et al. 2008 ). Thus, experiments do not have to be “natural”, but species-relevant, and subjects have to understand the test situation. Although subjects in these studies were not tested in a “natural” competitive situation in which chimpanzees physically compete over food, they showed very flexible perspective-taking skills. This finding suggests that it was crucial that chimpanzees were able to perceive the situation as competitive.
Certainly, one aspect of experiments that is often overlooked is how animals are able to perceive a situation. Shettleworth’s definition of cognition includes perception as it refers to adaptive information processing in the broadest sense. The modality in which a task is presented is absolutely crucial for detecting the cognitive potentials of a given species. For example, until now, nearly all studies about dog cognition have taken an anthropocentric view, mainly searching for skills and modalities that are important for humans, such as perspective-taking, cooperation, social learning, and visual or auditory communication. Most data on dogs’ understanding of their social and physical environment is based on performance in the visual or auditory modality ( Bräuer et al. 2013b ; Kaminski et al. 2004a ; Kundey et al. 2010 ), even though olfaction is the most relevant sense that dogs use to explore their environment ( Gazit and Terkel 2003 ; Horowitz et al. 2013 ). Therefore, dog cognition studies should, in fact, design tests based on olfaction, not on vision or hearing. Indeed, a recent study by Bräuer and Belger ( 2018 ) suggests that dogs have a flexible representation of what they smell. The fact that olfaction was neglected in dog cognition research until recently, when dogs were shown to excel at a task when it was reframed in the olfactory modality, illustrates how an anthropocentric approach can create the appearance of limitations in cognitive performance. On the contrary, apparent limitations can be due simply to the use of a perceptual modality that is disadvantageous to the animal being tested.
The modality also seems to be crucial in biological market tasks. In a study by Salwiczek and colleagues (2012), individuals of several fish and primate species had to make a choice between two actions in a foraging task. They could choose between two plates (differing in color and patterns to allow discrimination) with exactly the same food. However, one plate was ephemeral and the other one permanent. The food maximizing solution involved eating from the ephemeral food source first and only then from the permanent one that yielded an additional delayed reward. This task was ecologically relevant for the tested cleaner fish as it mimicked the simultaneous visit of a resident and a visitor to the cleaning station. Indeed, the cleaner fishes outperformed the tested chimpanzees, orangutans, and capuchin monkeys in that task ( Salwiczek et al. 2012 ). However, when the task was made more perceivable for primates, primates improved their performance: when the food was colored instead of the plates, and when the food reward was hidden, capuchin monkeys readily learned to solve the task ( Prétôt et al. 2016 ). Similarly, rats and pigeons could solve the task when there was a 20-second delay between the choice and its outcome ( Zentall and Case 2018 ). These examples reflect the prime importance of perceptual modality on reaching conclusions about a species’ cognitive abilities.
In addition to the importance of modality on how species perceive tasks, there are ecological restrictions to what a member of a certain species can learn ( Campbell et al. 2008 ). For example, cleaner fishes can only use generalized rule learning when the rule has ecological relevance. They can learn that predators are safe havens when chased by a punishing client while nonpredatory clients are not. However, they cannot learn to approach a nonpredatory client as a safe haven ( Wismer et al. 2016 ). Similarly, bees can associate color with food but apparently not with danger ( de Ibarra et al. 2014 ; Craig 1994 ). This difference between learnability in modalities might be comparable to the way humans who get food poisoning in a restaurant will develop an aversion to the food rather than to the person they went out with.
In sum, we emphasize again the importance of ecologically relevant experiments to uncover cognitive processes in nonhuman animals. As we illustrated above, many experiments that were designed anthropocentrically found negative results, which were then prematurely generalized to the species. The solution to this problem is that the tested skills and the experiments themselves should be ecologically valid. Ecological validity can be achieved by taking into account the importance of perception due to the modality of task presentation and the limitations for learning. One of the hardest tasks for animal cognition researchers in the coming years will be to design experiments that can detect the upper limits of animals’ abilities—a challenge that is especially difficult for us, as humans, in the case of nonhuman cognitive abilities that exceed anything we can imagine with our limited perception and cognition.
5. New Challenges in Animal Cognition
We began with a reminder of the fundamental but sometimes neglected call ( Kamil 1987 ) for comparative research to take a biocentric view of cognition and to avoid common anthropocentric viewpoints ( Allen 2017 ; Shettleworth 2010 ; Shettleworth 2012 ; Vonk 2016 ). We see three concrete weaknesses in current animal cognition research. Firstly, there is a widely shared conception that certain cognitive clusters found in humans, such as technical intelligence, are similarly organized in other animals, although there is no clear evidence for such similarities ( Ducatez et al. 2015 ; see Section 2 ). Secondly, skills that are on par with those of humans have sometimes been overrated in humans and underrated in other species (i.e., Boesch et al. 2017 ). Therefore, species-specific cognitive skills (i.e., Kaminski and Marshall-Pescini 2014 ; McMillan et al. 2015 ) and findings of species that outperform humans on distinct tasks were sometimes overlooked or not tested, as it is difficult to find the appropriate experimental design (see Section 3 ). Thirdly, another element that should be strengthened is the importance of ecologically relevant experimental designs that consider perception and the limitations for learning in the tested species ( Bates and Byrne 2007 ; De Waal 2016 ; see Section 4 ).
In addition to the concerns we have raised here, other researchers have highlighted the need to consider within-species variability ( Barrett 2016 ), phylogenetic factors (to control for effects of shared descent; Balda et al. 1996 ), and social characteristics (e.g., level of competition and tolerance; Hare 2001 ; Fröhlich et al. 2016 ), as well as the application of different methodologies across species ( Leavens et al. 2017 ). To remedy these issues, researchers interested in animal cognition should collaborate to test a wider variety of animal taxa rather than only the most common model species with presumably human-like cognitive abilities (i.e., Rowell 1999 ; Stanton et al. 2017 ). As animal cognition research is a truly interdisciplinary subject that appeals to researchers from distinct disciplines such as psychology, biology, anthropology, and neuroscience (e.g., Osiurak et al. 2020 ), we need to acknowledge the fact that they can and should complement each other. For example, it would be helpful for behavioral ecologists to include more cognitive research in their studies as they are experts on the ecology of a given species. On the other hand, psychologists who usually concentrate on the mechanism of a behavior could consider the ecological relevance and the phylogenetic history of their behavior of interest. Hence, observational investigations of the natural behavior of species and experimental studies should go hand in hand to enable detailed insights into the cognitive potential of a given species ( Janmaat 2019 ).
In summary, taking together all of the old and new criticisms that we have identified for the future of animal cognition, we advocate that
- Studies should be clear about which cognitive skill(s) they are testing and should not interpret evidence for one skill as automatically proving another, untested skill. Research should not assume that cognitive skills cluster the way they do in humans, but rather should start from the expectation of multiple cognitions until proven otherwise.
- Studies should be based on detailed knowledge of the natural behavior and the ecological environment of the test species, so that it is possible to generate precise hypotheses about the species’ performance on a specific cognitive task ( Bates and Byrne 2007 ).
- Experimental settings should take into account social structures, developmental constraints, and preferred modality of the species under study ( Bates and Byrne 2007 ; Roth et al. 2019 ).
- Studies with nonhuman animals should no longer target only typically human cognitive skills such as tool-use, self-control, or social cooperation, but should also test skills in which humans might be outperformed by other animals, such as visual and odor perception, working memory, and reaction time (i.e., De Waal 2016 ; Bräuer and Belger 2018 ; Inoue and Matsuzawa 2007 ).
- A holistic approach should be implemented to better integrate laboratory and fieldwork of behavioral ecologists, including the conducting of more rigorous observations and field experiments ( Janmaat 2019 ; Boesch 2010 ; Bueno-Guerra and Amici 2018 ).
- An even wider variety of animal taxa should be tested—starting with species that are as yet untested and under-represented in experiments—to gain a whole picture of cognition in the Animal kingdom ( Vonk 2016 ; Roth et al. 2019 ).
Once research turns to the study of each species’ cognitive skills for its own sake ( Allen 1998 ; Holekamp 2007 ; Borrego 2017 ), we will gain a more relevant perspective on animals’ cognitive skills that incorporates factors such as ecology, social environment, behavior, and development ( Uomini 2008 ; Sewall 2015 ; González-Forero and Gardner 2018 ; Uomini et al. 2020 ), overlain onto the recognition that unique single cognitive capacities in some species coexist with full-blown cognitive arrays in others. Hence, there is not “one cognition”.
Acknowledgments
We thank Alex Taylor, Redouan Bshary, Asif Ghazanfar, Blanca Vidal Orga, and three anonymous reviewers for helpful comments on previous drafts.
Author Contributions
The review was initiated and conceived by J.B. All authors contributed to the design, implementation, and writing of the article, (coordinated by J.B. and N.U.) J.B. and N.U. revised the article, with inputs from all authors. All authors have read and agreed to the published version of the manuscript.
We thank the Max Planck Society for supporting our research, the European Research Council (772000—TURNTAKING, SP), and the support of a grant from the Templeton World Charity Foundation ( https://www.templetonworldcharity.org/ ) #0271 (NU).
Conflicts of Interest
The opinions expressed in this publication are those of the authors and do not necessarily reflect the views of the Templeton World Charity Foundation, Inc.
- Agrillo Christian, Piffer Laura, Bisazza Angelo, Butterworth Brian. Evidence for two numerical systems that are similar in humans and guppies. PLoS ONE. 2012; 7 :e31923. doi: 10.1371/journal.pone.0031923. [ PMC free article ] [ PubMed ] [ CrossRef ] [ Google Scholar ]
- Allen Colin. Assessing animal cognition: Ethological and philosophical perspectives. Journal of Animal Science. 1998; 76 :42–47. doi: 10.2527/1998.76142x. [ PubMed ] [ CrossRef ] [ Google Scholar ]
- Allen Colin. On (not) defining cognition. Synthese. 2017; 194 :4233–49. doi: 10.1007/s11229-017-1454-4. [ CrossRef ] [ Google Scholar ]
- Allen Colin, Trestman Michael. The Blackwell Companion to Consciousness. John Wiley & Sons Inc.; Hoboken: 2017. Animal Consciousness; pp. 63–76. [ Google Scholar ]
- Arvidsson Josefin, Amundin Mats, Laska Matthias. Successful acquisition of an olfactory discrimination test by Asian elephants, Elephas maximus. Physiology Behaviour. 2012; 105 :809–14. doi: 10.1016/j.physbeh.2011.08.021. [ PubMed ] [ CrossRef ] [ Google Scholar ]
- Auersperg Alice M. I., von Bayern Auguste M. I., Weber S., Szabadvari A., Bugnyar Thomas, Kacelnik Alex. Social transmission of tool use and tool manufacture in Goffin cockatoos ( Cacatua goffini ) Proceedings of the Royal Society B: Biological Sciences. 2014; 281 :20140972. doi: 10.1098/rspb.2014.0972. [ PMC free article ] [ PubMed ] [ CrossRef ] [ Google Scholar ]
- Balda Russell P., Kamil Alan C. Long-term spatial memory in clark’s nutcracker, Nucifraga columbiana. Animal Behaviour. 1992; 44 :761–69. doi: 10.1016/S0003-3472(05)80302-1. [ CrossRef ] [ Google Scholar ]
- Balda Russell P., Kamil Alan C., Bednekoff Peter A. Predicting cognitive capacity from Natural History. In: Nolan Val, Ketterson Ellen D., editors. Current Ornithology. Springer US; Boston: 1996. pp. 33–66. [ Google Scholar ]
- Barrett Louise. Why brains are not computers, why behaviorism is not satanism, and why dolphins are not aquatic apes. The Behavior Analyst. 2016; 39 :9–23. doi: 10.1007/s40614-015-0047-0. [ PMC free article ] [ PubMed ] [ CrossRef ] [ Google Scholar ]
- Bates Lucy Anne, Byrne Richard W. Creative or created: Using anecdotes to investigate animal cognition. Methods. 2007; 42 :12–21. doi: 10.1016/j.ymeth.2006.11.006. [ PubMed ] [ CrossRef ] [ Google Scholar ]
- Beach Frank A. The snark was a boojum. The American Psychologist. 1995; 5 :115–24. doi: 10.1037/h0056510. [ CrossRef ] [ Google Scholar ]
- Benson-Amram Sarah, Heinen Virginia K., Dryer Sean L., Holekamp Kay E. Numerical assessment and individual call discrimination by wild spotted hyaenas, Crocuta crocuta. Animal Behaviour. 2011; 82 :743–52. doi: 10.1016/j.anbehav.2011.07.004. [ CrossRef ] [ Google Scholar ]
- Beran Michael J., Parrish Audrey E., Perdue Bonnie M., Washburn David A. Comparative cognition: Past, present, and future. International Journal of Comparative Psychology. 2014; 27 :3–30. [ PMC free article ] [ PubMed ] [ Google Scholar ]
- Boesch Christophe. What makes us human ( Homo sapiens )? The challenge of cognitive cross-species comparison. Journal of Comparative Psychology. 2007; 121 :227–40. doi: 10.1037/0735-7036.121.3.227. [ PubMed ] [ CrossRef ] [ Google Scholar ]
- Boesch Christophe. Listening to the appeal from the wild. Animal Behavior and Cognition. Animal Behavior and Cognition. 2010; 7 doi: 10.26451/abc.07.02.14.2020. [ CrossRef ] [ Google Scholar ]
- Boesch Christophe, Boesch-Achermann Hedwige. The Chimpanzees of the Tai Forest. Oxford University Press; Oxford: 2000. [ Google Scholar ]
- Boesch Christophe, Hohmann Gottfried, Marchant Linda F. Behavioural diversity in chimpanzees and bonobos. In: Boesch Christophe, Hohmann Gottfried, Marchant Linda F., editors. Behavioural Diversity in Chimpanzees and Bonobos. University Press; Cambridge: 2002. [ Google Scholar ]
- Boesch Christophe, Bombjaková Dasa, Boyette Adam, Meier Amelia. Technical intelligence and culture: Nut cracking in humans and chimpanzees. American Journal of Physical Anthropology. 2017; 163 :339–55. doi: 10.1002/ajpa.23211. [ PubMed ] [ CrossRef ] [ Google Scholar ]
- Borrego Natalia. Big cats as a model system for the study of the evolution of intelligence. Behavioral Processes. 2017; 141 doi: 10.1016/j.beproc.2017.03.010. [ PubMed ] [ CrossRef ] [ Google Scholar ]
- Botigué Laura R., Song Shiya, Scheu Amelie, Gopalan Shyamalika, Pendleton Amanda L., Oetjens Matthew, Taravella Angela M., Seregély Timo, Zeeb-Lanz Andrea, Arbogast Rose-Marie, et al. Ancient European dog genomes reveal continuity since the Early Neolithic. Nature Communications. 2017; 8 :16082. doi: 10.1038/ncomms16082. [ PMC free article ] [ PubMed ] [ CrossRef ] [ Google Scholar ]
- Bräuer Juliane. I do not understand but I care: The prosocial dog. Interaction Studies. 2015; 16 :341–60. [ Google Scholar ]
- Bräuer Juliane, Belger Julia. A ball is not a Kong: Odor representation and search behavior in domestic dogs ( Canis familiaris ) of different education. Journal of Comparative Psychology. 2018; 132 :189–99. doi: 10.1037/com0000115. [ PubMed ] [ CrossRef ] [ Google Scholar ]
- Bräuer Juliane, Kaminski Juliane, Riedel Julia, Call Josep, Tomasello Michael. Making inferences about the location of hidden food: Social dog, causal ape. Journal of Comparative Psychology. 2006; 120 :38–47. doi: 10.1037/0735-7036.120.1.38. [ PubMed ] [ CrossRef ] [ Google Scholar ]
- Bräuer Juliane, Call Josep, Tomasello Michael. Chimpanzees really know what others can see in a competitive situation. Animal Cognition. 2007; 10 :439–48. doi: 10.1007/s10071-007-0088-1. [ PubMed ] [ CrossRef ] [ Google Scholar ]
- Bräuer Juliane, Schönefeld Katja, Call Josep. When do dogs help humans? Applied Animal Behaviour Science. 2013a; 148 :138–49. doi: 10.1016/j.applanim.2013.07.009. [ CrossRef ] [ Google Scholar ]
- Bräuer Juliane, Keckeisen Magdalena, Pitsch Andrea, Kaminski Juliane, Call Josep, Tomasello Michael. Domestic dogs conceal auditory but not visual information from others. Animal Cognition. 2013b; 16 :351–59. doi: 10.1007/s10071-012-0576-9. [ PubMed ] [ CrossRef ] [ Google Scholar ]
- Bräuer Juliane, Bös Milena, Call Josep, Tomasello Michael. Domestic dogs (Canis familiaris) coordinate their actions in a problem-solving task. Animal Cognition. 2013c; 16 :273–85. doi: 10.1007/s10071-012-0571-1. [ PubMed ] [ CrossRef ] [ Google Scholar ]
- Bshary Redouan, Brown Culum. Fish cognition. Current Biology. 2014; 24 :R947–R50. doi: 10.1016/j.cub.2014.08.043. [ PubMed ] [ CrossRef ] [ Google Scholar ]
- Bshary Redouan, Lascio Felice, Pinto Ana, van de Waal Erica. How Intelligent Is Machiavellian Behavior? MIT Press; Cambridge: 2011. [ Google Scholar ]
- Bueno-Guerra Nereida, Amici Federica., editors. Field and Laboratory Methods in Animal Cognition: A Comparative Guide. Cambridge University Press; Cambridge: 2018. [ Google Scholar ]
- Bugnyar Thomas, Reber Stephan A., Buckner Cameron. Ravens attribute visual access to unseen competitors. Nature Communications. 2016; 7 :10506. doi: 10.1038/ncomms10506. [ PMC free article ] [ PubMed ] [ CrossRef ] [ Google Scholar ]
- Burkart Judith M., Van Schaik Carel P. Cognitive consequences of cooperative breeding in primates? Animal Cognition. 2010; 13 :1–19. doi: 10.1007/s10071-009-0263-7. [ PubMed ] [ CrossRef ] [ Google Scholar ]
- Burkart Judith M., Hrdy Sarah B., Van Schaik Carel P. Cooperative breeding and human cognitive evolution. Evolutionary Anthropology: Issues, News, and Reviews. 2009; 18 :175–86. doi: 10.1002/evan.20222. [ CrossRef ] [ Google Scholar ]
- Burkart Judith M., Schubiger Michele N., van Schaik Carel P. The evolution of general intelligence. Behavioral and Brain Sciences. 2016; 40 :e195. doi: 10.1017/S0140525X16000959. [ PubMed ] [ CrossRef ] [ Google Scholar ]
- Burrows Anne M., Waller Bridget M., Liebal Katja. Primate Communication: A Multimodal Approach. Cambridge University Press; Cambridge: 2013. [ PMC free article ] [ PubMed ] [ Google Scholar ]
- Byrne Richard W. Machiavellian Intelligence. Evolutionary Anthropology. 1996; 5 :172–80. doi: 10.1002/(SICI)1520-6505(1996)5:5<172::AID-EVAN6>3.0.CO;2-H. [ CrossRef ] [ Google Scholar ]
- Byrne Richard W. The Technical Intelligence hypothesis: An additional evolutionary stimulus to intelligence? In: Byrne Richard W., Whiten Andrew., editors. Machiavellian Intelligence II: Extensions and Evaluations. University Press; Cambridge: 1997. pp. 289–311. [ Google Scholar ]
- Byrne Richard W., Whiten Andrew. Machiavellian Intelligence: Social Expertise and the Evolution of Intellect in Monkeys, Apes and Humans. Clarendon Press; Oxford: 1988. [ Google Scholar ]
- Call Josep, Tomasello Michael. Does the chimpanzee have a Theory of Mind? 30 years later. Trends in Cognitive Sciences. 2008; 12 :187–92. doi: 10.1016/j.tics.2008.02.010. [ PubMed ] [ CrossRef ] [ Google Scholar ]
- Call Josep, Bräuer Juliane, Kaminski Juliane, Tomasello Michael. Domestic Dogs ( Canis familiaris ) Are Sensitive to the Attentional State of Humans. Journal of Comparative Psychology. 2003; 117 :257–63. doi: 10.1037/0735-7036.117.3.257. [ PubMed ] [ CrossRef ] [ Google Scholar ]
- Call Josep, Burghardt Gordon M., Pepperberg Irene M., Snowdon Charles T., Zentall Thomas. In: APA Handbook of Comparative Psychology. Call J., Burghardt G. M., Pepperberg I. M., Snowdon C. T., Zentall T., editors. American Psychological Association; Washington: 2017. (Volume 1: Basic Concepts, Methods, Neural Substrate, and Behavior, Volume 2: Perception, Learning, and Cognition. 2 vols. Part of the APA Handbooks in Psychology Series). [ Google Scholar ]
- Campbell Neil A., Reece Jane B., Urry Lisa A., Cain Michael L., Wasserman Steven A., Minorsky Peter V., Jackson Robert B. Biology. Pearson Benjamin Cummings; Berkeley: 2008. [ Google Scholar ]
- Cantlon Jessica F., Hayden Benjamin Y. Editorial overview: Comparative cognition. Current Opinion in Behavioral Sciences. 2017; 16 :iv–vi. doi: 10.1016/j.cobeha.2017.07.008. [ CrossRef ] [ Google Scholar ]
- Clayton Nicola S., Emery Nathan J. Corvid cognition. Current Biology. 2002; 15 :R80–R81. doi: 10.1016/j.cub.2005.01.020. [ PubMed ] [ CrossRef ] [ Google Scholar ]
- Clayton Nicola S., Emery Nathan J., Dickinson Anthony. The prospective cognition of food caching and recovery. Comparative Cognition and Behavior Reviews. 2006; 1 :1–11. doi: 10.3819/ccbr.2008.10001. [ CrossRef ] [ Google Scholar ]
- Craig Catherine L. Limits to learning: Effects of predator pattern and colour on perception and avoidance-learning by prey. Animal Behaviour. 1994; 47 :1087–99. doi: 10.1006/anbe.1994.1147. [ CrossRef ] [ Google Scholar ]
- Crockford Catherine, Wittig Roman, Mundry Roger, Zuberbühler Klaus. Wild chimpanzees inform ignorant group members of danger. Current Biology. 2012; 22 :142–46. doi: 10.1016/j.cub.2011.11.053. [ PubMed ] [ CrossRef ] [ Google Scholar ]
- Crockford Catherine, Wittig Roman M., Zuberbühler Klaus. Vocalizing in chimpanzees is influenced by social-cognitive processes. Science Advances. 2017; 3 :e1701742. doi: 10.1126/sciadv.1701742. [ PMC free article ] [ PubMed ] [ CrossRef ] [ Google Scholar ]
- Dale Rachel, Quervel-Chaumette Mylene, Huber Ludwig, Range Friederike, Marshall-Pescini Sarah. Task differences and prosociality: Investigating pet dogs’ prosocial preferences in a token choice paradigm. PLoS ONE. 2016; 11 :e0167750. doi: 10.1371/journal.pone.0167750. [ PMC free article ] [ PubMed ] [ CrossRef ] [ Google Scholar ]
- Dally Joanna M., Emery Nathan J., Clayton Nicola S. Food-Caching Western Scrub-Jays Keep Track of Who Was Watching When. Science. 2006; 310 :1662–65. doi: 10.1126/science.1126539. [ PubMed ] [ CrossRef ] [ Google Scholar ]
- Darwin Charles. On the Origin of Species by Means of Natural Selection. John Murray; London: 1859. [ Google Scholar ]
- De Waal Frans B. M. Are We Smart Enough to Know How Smart ANIMALS are? W.W. Norton et Company; New York: 2016. [ Google Scholar ]
- De Waal Frans B. M., Tyack Peter L., editors. Animal Social Complexity: Intelligence, Culture, and Individualized Societies. Harvard University Press; Cambridge: 2009. [ Google Scholar ]
- Ducatez Simon, Clavel Joanne, Lefebvre Louis. Ecological generalism and behavioural innovation in birds: Technical intelligence or the simple incorporation of new foods? Journal of Animal Ecology. 2015; 84 :79–89. doi: 10.1111/1365-2656.12255. [ PubMed ] [ CrossRef ] [ Google Scholar ]
- Dunbar Robin I. M., Shultz Susanne. Evolution in the social brain. Science. 2007; 317 :1344–47. doi: 10.1126/science.1145463. [ PubMed ] [ CrossRef ] [ Google Scholar ]
- Eaton Taryn, Hutton Robert, Leete Jessica, Lieb Jennifer, Robeson Audrey, Vonk Jennifer. Bottoms-up! Rejecting top-down human-centered approaches in comparative psychology. International Journal of Comparative Psychology. 2018; 31 :1–19. [ Google Scholar ]
- Emery Nathan J. Cognitive ornithology: The evolution of avian intelligence. Philosophical Transactions of the Royal Society B: Biological Sciences. 2006; 361 :23–43. doi: 10.1098/rstb.2005.1736. [ PMC free article ] [ PubMed ] [ CrossRef ] [ Google Scholar ]
- Erdohegyi Ágnes, Topál József, Virányi Zsófia, Miklósi Ádám. Dog-Logic: Inferential Reasoning in a Two-Way Choice Task and Its Restricted Use. Animal Behaviour. 2007; 74 :725–37. doi: 10.1016/j.anbehav.2007.03.004. [ CrossRef ] [ Google Scholar ]
- Fehr Ernst, Gächter Simon. Altruistic punishment in humans. Nature. 2002; 415 :137–40. doi: 10.1038/415137a. [ PubMed ] [ CrossRef ] [ Google Scholar ]
- Feinerman Ofer, Korman Amos. Individual versus collective cognition in social insects. The Journal of Experimental Biology. 2017; 220 :73–82. doi: 10.1242/jeb.143891. [ PMC free article ] [ PubMed ] [ CrossRef ] [ Google Scholar ]
- Fitch W. Tecumseh, Huber Ludwig, Bugnyar Thomas. Social cognition and the evolution of language: Constructing cognitive phylogenies. Neuron. 2010; 65 :795–814. doi: 10.1016/j.neuron.2010.03.011. [ PMC free article ] [ PubMed ] [ CrossRef ] [ Google Scholar ]
- Fröhlich Marlen, Kuchenbuch Paul, Müller Gudrun, Fruth Barbara, Furuichi Takeshi, Wittig Roman M., Pika Simone. Unpeeling the layers of language: Bonobos and chimpanzees engage in cooperative turn-taking sequences. Scientific Reports. 2016; 6 :25887. doi: 10.1038/srep25887. [ PMC free article ] [ PubMed ] [ CrossRef ] [ Google Scholar ]
- Furuichi Takeshi, Idani Genichi, Ihobe Hiroshi, Kuroda Suehisa, Kitamura Koji, Mori Akio, Enomoto Tomoo, Okayasu Naobi, Hashimoto Chie, Kano Takayoshi. “Population Dynamics of Wild Bonobos (Pan paniscus) at Wamba” International Journal of Primatology. 1998; 19 :1029–43. doi: 10.1023/A:1020326304074. [ CrossRef ] [ Google Scholar ]
- Gaissmaier Wolfgang, Schooler Lael J. The smart potential behind probability matching. Cognition. 2008; 109 :416–22. doi: 10.1016/j.cognition.2008.09.007. [ PubMed ] [ CrossRef ] [ Google Scholar ]
- Gazit Irit, Terkel Joseph. Domination of Olfaction over Vision in Explosives Detection by Dogs. Applied Animal Behaviour Science. 2003; 82 :65–73. doi: 10.1016/S0168-1591(03)00051-0. [ CrossRef ] [ Google Scholar ]
- Gillespie-Lynch Kristen, Greenfield Patricia M., Lyn Heidi, Savage-Rumbaugh Sue. Gestural and symbolic development among apes and humans: Support for a multimodal theory of language evolution. Frontiers in Psychology. 2014; 5 doi: 10.3389/fpsyg.2014.01228. [ PMC free article ] [ PubMed ] [ CrossRef ] [ Google Scholar ]
- González-Forero Mauricio, Gardner Andy. Inference of ecological and social drivers of human brain-size evolution. Nature. 2018; 557 :554–57. doi: 10.1038/s41586-018-0127-x. [ PubMed ] [ CrossRef ] [ Google Scholar ]
- Goodall Jane. The Chimpanzees of Gombe. Belknap Press; Cambridge: 1986. [ Google Scholar ]
- Griffiths Paul E., Stotz Karola. How the mind grows: A developmental perspective on the biology of cognition. Synthese. 2000; 122 :29–51. doi: 10.1023/A:1005215909498. [ CrossRef ] [ Google Scholar ]
- Grosenick Logan, Clement Tricia S., Fernald Russell D. Fish can infer social rank by observation alone. Nature. 2007; 445 :429. doi: 10.1038/nature05511. [ PubMed ] [ CrossRef ] [ Google Scholar ]
- Gruber Romana, Schiestl Martina, Boeckle Markus, Frohnwieser Anna, Miller Rachael, Gray Russell, Clayton Nicola, Taylor Alex. New Caledonian Crows Use Mental Representations to Solve Metatool Problems. Current Biology. 2019; 29 doi: 10.1016/j.cub.2019.01.008. [ PMC free article ] [ PubMed ] [ CrossRef ] [ Google Scholar ]
- Güntürkün Onur, Bugnyar Thomas. Cognition without cortex. Trends in Cognitive Sciences. 2016; 20 :291–303. doi: 10.1016/j.tics.2016.02.001. [ PubMed ] [ CrossRef ] [ Google Scholar ]
- Halina Marta, Liebal Katja, Tomasello Michael. The goal of ape pointing. PLoS ONE. 2018; 13 :e0195182. doi: 10.1371/journal.pone.0195182. [ PMC free article ] [ PubMed ] [ CrossRef ] [ Google Scholar ]
- Hanus Daniel. Causal reasoning versus associative learning: A useful dichotomy or a strawman battle in comparative psychology? Journal of Comparative Psychology. 2016; 130 :241–48. doi: 10.1037/a0040235. [ PubMed ] [ CrossRef ] [ Google Scholar ]
- Hanus Daniel, Mendes Natacha, Tennie Claudio, Call Josep. Comparing the performances of apes ( Gorilla gorilla, Pan troglodytes, Pongo pygmaeus ) and human children ( Homo sapiens ) in the Floating Peanut Task. PLoS ONE. 2011; 6 :e19555. doi: 10.1371/journal.pone.0019555. [ PMC free article ] [ PubMed ] [ CrossRef ] [ Google Scholar ]
- Hare Brian. Can competitive paradigms increase the validity of experiments on primate social cognition? Animal Cognition. 2001; 4 :269–80. doi: 10.1007/s100710100084. [ PubMed ] [ CrossRef ] [ Google Scholar ]
- Hare Brian, Tomasello Michael. Chimpanzees are more skilful in competitive than in cooperative cognitive tasks. Animal Behaviour. 2004; 68 :571–81. doi: 10.1016/j.anbehav.2003.11.011. [ CrossRef ] [ Google Scholar ]
- Hare Brian, Tomasello Michael. Human-like social skills in dogs? Trends in Cognitive Sciences. 2005; 9 :439–44. doi: 10.1016/j.tics.2005.07.003. [ PubMed ] [ CrossRef ] [ Google Scholar ]
- Hare Brian, Call Josep, Agnetta Bryan, Tomasello Michael. Chimpanzees Know What Conspecifics Do and Do Not See. Animal Behaviour. 2000; 59 :771–85. doi: 10.1006/anbe.1999.1377. [ PubMed ] [ CrossRef ] [ Google Scholar ]
- Hare Brian, Call Josep, Tomasello Michael. Do Chimpanzees Know What Conspecifics Know? Animal Behaviour. 2001; 61 :139–51. doi: 10.1006/anbe.2000.1518. [ PubMed ] [ CrossRef ] [ Google Scholar ]
- Hare Brian, Wobber Victoria, Wrangham Richard. The self-domestication hypothesis: Evolution of bonobo psychology is due to selection against aggression. Animal Behavior. 2012; 83 :573–85. doi: 10.1016/j.anbehav.2011.12.007. [ CrossRef ] [ Google Scholar ]
- Hart Benjamin L., Hart Lynette A., Pinter-Wollman Noa. Large brains and cognition: Where do elephants fit in? Neuroscience & Biobehavioral Reviews. 2008; 32 :86–98. doi: 10.1016/j.neubiorev.2007.05.012. [ PubMed ] [ CrossRef ] [ Google Scholar ]
- De Ibarra N. Hempel, Vorobyev Misha, Menzel Randolf. Mechanisms, functions and ecology of colour vision in the honeybee. Journal of Comparative Physiology A: Neuroethology, Sensory, Neural, and Behavioral Physiology. 2014; 200 :411–33. doi: 10.1007/s00359-014-0915-1. [ PMC free article ] [ PubMed ] [ CrossRef ] [ Google Scholar ]
- Herbranson Walter T. Pigeons, Humans, and the Monty Hall Dilemma. Current Directions in Psychological Science. 2012; 21 :297–301. doi: 10.1177/0963721412453585. [ CrossRef ] [ Google Scholar ]
- Herrmann Esther, Tomasello Michael. Apes’ and children’s understanding of cooperative and competitive motives in a communicative situation. Developmental Science. 2006; 9 :518–29. doi: 10.1111/j.1467-7687.2006.00519.x. [ PubMed ] [ CrossRef ] [ Google Scholar ]
- Herrmann Esther, Call Josep, Hernández-Lloreda María Victoria, Hare Brian, Tomasello Michael. Humans have evolved specialized skills of social cognition: The Cultural Intelligence Hypothesis. Science. 2007; 317 :1360–66. doi: 10.1126/science.1146282. [ PubMed ] [ CrossRef ] [ Google Scholar ]
- Heyes Cecilia, Huber Ludwig. The Evolution of Cognition. MIT Press; Cambridge: 2000. [ Google Scholar ]
- Hodos William, Campbell Colin Boyd G. Scala naturae: Why there is no theory in comparative psychology. Psychological Review. 1969; 76 :337–50. doi: 10.1037/h0027523. [ CrossRef ] [ Google Scholar ]
- Holekamp Kay E. Questioning the social intelligence hypothesis. Trends in Cognitive Sciences. 2007; 11 :65–69. doi: 10.1016/j.tics.2006.11.003. [ PubMed ] [ CrossRef ] [ Google Scholar ]
- Hollard Valerie D., Delius Juan D. Rotational invariance in visual pattern recognition by pigeons and humans. Science. 1982; 218 :804. doi: 10.1126/science.7134976. [ PubMed ] [ CrossRef ] [ Google Scholar ]
- Holzhaider Jennifer C., Hunt Gavin R., Gray Russell D. Social learning in New Caledonian crows. Learning and Behavior. 2010a; 38 :206–19. doi: 10.3758/LB.38.3.206. [ PubMed ] [ CrossRef ] [ Google Scholar ]
- Holzhaider Jennifer C., Hunt Gavin R., Gray Russell D. The development of pandanus tool manufacture in wild New Caledonian crows. Behaviour. 2010b; 147 :553–86. doi: 10.1163/000579510X12629536366284. [ CrossRef ] [ Google Scholar ]
- Holzhaider J. C., Sibley M. D., Taylor A. H., Singh P. H., Gray R. D., Hunt G. R. The social structure of New Caledonian crows. Animal Behaviour. 2011; 81 :83–92. doi: 10.1016/j.anbehav.2010.09.015. [ CrossRef ] [ Google Scholar ]
- Horowitz Alexandra, Hecht Julie, Dedrick Alexandra. Smelling more or less: Investigating the olfactory experience of the domestic dog. Learning and Motivation. 2013; 44 :207–17. doi: 10.1016/j.lmot.2013.02.002. [ CrossRef ] [ Google Scholar ]
- Huber Ludwig. How dogs perceive and understand us. Current Directions in Psychological Science. 2016; 25 :339–44. doi: 10.1177/0963721416656329. [ CrossRef ] [ Google Scholar ]
- Humphrey Nicholas K. The social function of intellect. In: Bateson Patrick P. G., Hinde Robert A., editors. Growing Points in Ethology. Cambridge University Press; Cambridge: 1976. pp. 303–17. [ Google Scholar ]
- Hunt Gavin R., Gray Russell D. The crafting of hook tools by wild New Caledonian crows. Proceedings of the Royal Society of London B. 2004; 271 :S88–S90. doi: 10.1098/rsbl.2003.0085. [ PMC free article ] [ PubMed ] [ CrossRef ] [ Google Scholar ]
- Hunt Gavin R., Uomini Natalie. A complex adaptive system may be essential for cumulative modifications in tool design. Japanese Journal of Animal Psychology. 2016; 66 doi: 10.2502/janip.66.2.2. [ CrossRef ] [ Google Scholar ]
- Inoue Sana, Matsuzawa Tetsuro. Working memory of numerals in chimpanzees. Current Biology. 2007; 17 :R1004–R1005. doi: 10.1016/j.cub.2007.10.027. [ PubMed ] [ CrossRef ] [ Google Scholar ]
- Jacobs Ivo F., von Bayern Auguste, Martin-Ordas Gema, Rat-Fischer Lauriane, Osvath Mathias. Corvids create novel causal interventions after all. Proceedings of the Royal Society B: Biological Sciences. 2015; 282 :20142504. doi: 10.1098/rspb.2014.2504. [ PMC free article ] [ PubMed ] [ CrossRef ] [ Google Scholar ]
- Janmaat Karline R. L. What animals do not do or fail to find: A novel observational approach for studying cognition in the wild. Evolutionary Anthropology: Issues, News, and Reviews. 2019; 28 :303–20. doi: 10.1002/evan.21794. [ PMC free article ] [ PubMed ] [ CrossRef ] [ Google Scholar ]
- Janmaat Karline R. L., Polansky Leo, Ban Simone Dagui, Boesch Christophe. Wild chimpanzees plan their breakfast time, type, and location. Proceedings of the National Academy of Sciences. 2014; 111 :16343. doi: 10.1073/pnas.1407524111. [ PMC free article ] [ PubMed ] [ CrossRef ] [ Google Scholar ]
- Jelbert Sarah A., Singh Puja J., Gray Russell D., Taylor Alex H. New Caledonian Crows rapidly solve a collaborative problem without cooperative cognition. PLoS ONE. 2015; 10 :e0133253. doi: 10.1371/journal.pone.0133253. [ PMC free article ] [ PubMed ] [ CrossRef ] [ Google Scholar ]
- Jensen Keith, Call Josep, Tomasello Michael. Chimpanzees are rational maximizers in an Ultimatum Game. Science. 2007; 318 :107–9. doi: 10.1126/science.1145850. [ PubMed ] [ CrossRef ] [ Google Scholar ]
- Jiang Xinjian, Long Tenghai, Cao Weicong, Li Junru, Dehaene Stanislas, Wang Liping. Production of Supra-regular Spatial Sequences by Macaque Monkeys. Current Biology. 2018; 28 :1851–59. doi: 10.1016/j.cub.2018.04.047. [ PMC free article ] [ PubMed ] [ CrossRef ] [ Google Scholar ]
- Jolly Alison. Lemur social behavior and primate intelligence. Science. 1966; 153 :501–6. doi: 10.1126/science.153.3735.501. [ PubMed ] [ CrossRef ] [ Google Scholar ]
- Kabadayi Can, Osvath Mathias. Ravens parallel great apes in flexible planning for tool-use and bartering. Science. 2017; 357 :202. doi: 10.1126/science.aam8138. [ PubMed ] [ CrossRef ] [ Google Scholar ]
- Kamil Alan C. A synthetic approach to the study of animal intelligence. Papers in Behavior and Biological Sciences. 1987; 35 :257–308. [ PubMed ] [ Google Scholar ]
- Kaminski Juliane, Marshall-Pescini Sarah. The Social Dog: Behaviour and Cognition. Elsevier Publishers; San Diego, London, Waltham: 2014. [ Google Scholar ]
- Kaminski Juliane, Call Josep, Fischer Julia. Word learning in a domestic dog: Evidence for “Fast Mapping” Science. 2004a; 304 :1682–83. doi: 10.1126/science.1097859. [ PubMed ] [ CrossRef ] [ Google Scholar ]
- Kaminski Juliane, Call Josep, Tomasello Michael. Body Orientation and Face Orientation: Two Factors Controlling Apes’ Begging Behavior from Humans. Animal Cognition. 2004b; 7 :216–23. doi: 10.1007/s10071-004-0214-2. [ PubMed ] [ CrossRef ] [ Google Scholar ]
- Kaminski Juliane, Call Josep, Tomasello Michael. Chimpanzees know what others know, but not what they believe. Cognition. 2008; 109 :224–34. doi: 10.1016/j.cognition.2008.08.010. [ PubMed ] [ CrossRef ] [ Google Scholar ]
- Kaminski Juliane, Bräuer Juliane, Call Josep, Tomasello Michael. Domestic dogs are sensitive to a human’s perspective. Behaviour. 2009a; 146 :979–98. doi: 10.1163/156853908X395530. [ CrossRef ] [ Google Scholar ]
- Kaminski Juliane, Tempelmann Sebastian, Call Josep, Tomasello Michael. Domestic dogs comprehend human communication with iconic signs. Developmental Science. 2009b; 12 :831–37. doi: 10.1111/j.1467-7687.2009.00815.x. [ PubMed ] [ CrossRef ] [ Google Scholar ]
- Kaminski Juliane, Schulz Linda, Tomasello Michael. How dogs know when communication is intended for them. Developmental Science. 2012; 15 :222–32. doi: 10.1111/j.1467-7687.2011.01120.x. [ PubMed ] [ CrossRef ] [ Google Scholar ]
- Kaminski Juliane, Hynds Jennifer, Morris Paul, Waller Bridget M. Human attention affects facial expressions in domestic dogs. Scientific Reports. 2017; 7 :12914. doi: 10.1038/s41598-017-12781-x. [ PMC free article ] [ PubMed ] [ CrossRef ] [ Google Scholar ]
- Kellogg Winthrop N., Kellogg Luella Agger. The Ape and the Child: A Study of Early Environmental Influence Upon Early Behavior. McGraw-Hill; New York: 1933. [ Google Scholar ]
- Kenward Ben, Rutz Christian, Weir Alex A. S., Kacelnik Alex. Development of tool use in new caledonian crows: Inherited action patterns and social influences. Animal Behaviour. 2006; 72 :1329–43. doi: 10.1016/j.anbehav.2006.04.007. [ CrossRef ] [ Google Scholar ]
- Krachun Carla, Carpenter Malinda, Call Josep, Tomasello Michael. A competitive nonverbal false belief task for children and apes. Developmental Science. 2009; 12 :521–35. doi: 10.1111/j.1467-7687.2008.00793.x. [ PubMed ] [ CrossRef ] [ Google Scholar ]
- Krause Mark, Udell Monique, Leavens David, Skopos Lyra. Animal pointing: Changing trends and findings from 30 years of research (2018) Journal of Comparative Psychology. 2018; 132 doi: 10.1037/com0000125. [ PubMed ] [ CrossRef ] [ Google Scholar ]
- Krupenye Christopher, Kano Fumihiro, Hirata Satoshi, Call Josep, Tomasello Michael. Great apes anticipate that other individuals will act according to false beliefs. Science. 2016; 354 :110. doi: 10.1126/science.aaf8110. [ PubMed ] [ CrossRef ] [ Google Scholar ]
- Krupenye Christopher, Kano Fumihiro, Hirata Satoshi, Call Josep, Tomasello Michael. A test of the submentalizing hypothesis: Apes’ performance in a false belief task inanimate control. Communicative and Integrative Biology. 2017; 10 :e1343771. doi: 10.1080/19420889.2017.1343771. [ PMC free article ] [ PubMed ] [ CrossRef ] [ Google Scholar ]
- Kundey Shannon M. A., De Los Reyes Andres, Taglang Chelsea, Allen Rebecca, Molina Sabrina, Royer Erica, German Rebecca. Domesticated Dogs ( Canis familiaris ) React to What Others Can and Cannot Hear. Applied Animal Behaviour Science. 2010; 126 :45–50. doi: 10.1016/j.applanim.2010.06.002. [ CrossRef ] [ Google Scholar ]
- Laland Kevin N., Odling-Smee John, Feldman Marcus W. Niche construction, biological evolution, and cultural change. Behavioral Brain Sciences. 2000; 23 :131–46. doi: 10.1017/S0140525X00002417. [ PubMed ] [ CrossRef ] [ Google Scholar ]
- Lampe Michelle, Bräuer Juliane, Kaminski Juliane, Virányi Zsófia. The effects of domestication and ontogeny on cognition in dogs and wolves. Scientific Reports. 2017:11690. doi: 10.1038/s41598-017-12055-6. [ PMC free article ] [ PubMed ] [ CrossRef ] [ Google Scholar ]
- Laumer Isabelle B., Bugnyar Thomas, Reber Stephan A., Auersperg Alice M. I. Can hook-bending be let off the hook? Bending/unbending of pliant tools by cockatoos. Proceedings Biology Sciences. 2017; 284 :1862. doi: 10.1098/rspb.2017.1026. [ PMC free article ] [ PubMed ] [ CrossRef ] [ Google Scholar ]
- Leavens David A., Hopkins William D., Bard Kim A. Indexical and referential pointing in chimpanzees (Pan troglodytes) Journal of Comparative Psychology. 1996; 110 :346–53. doi: 10.1037/0735-7036.110.4.346. [ PMC free article ] [ PubMed ] [ CrossRef ] [ Google Scholar ]
- Leavens David A., Bard Kim A., Hopkins William D. The mismeasure of ape social cognition. Animal Cognition. 2017 doi: 10.1007/s10071-017-1119-1. [ PMC free article ] [ PubMed ] [ CrossRef ] [ Google Scholar ]
- Levenson Richard M., Krupinski Elizabeth A., Navarro Victor M., Wasserman Edward A. Pigeons ( Columba livia ) as trainable observers of pathology and radiology breast cancer images. PLoS ONE. 2015; 10 :e0141357. doi: 10.1371/journal.pone.0141357. [ PMC free article ] [ PubMed ] [ CrossRef ] [ Google Scholar ]
- Logan Corina, Jelbert Sarah, Breen Alexis, Gray Russell, Taylor Alex. Modifications to the Aesop’s Fable Paradigm Change New Caledonian Crow Performances. PLoS ONE. 2014; 9 :e103049. doi: 10.1371/journal.pone.0103049. [ PMC free article ] [ PubMed ] [ CrossRef ] [ Google Scholar ]
- Logan Corina J., Breen Alexis J., Taylor Alex H., Gray Russell D., Hoppitt William J. E. How New Caledonian crows solve novel foraging problems and what it means for cumulative culture. Learning and Behavior. 2016; 44 :18–28. doi: 10.3758/s13420-015-0194-x. [ PubMed ] [ CrossRef ] [ Google Scholar ]
- MacLean Evan L. Unraveling the evolution of uniquely human cognition. Proceedings of the National Academy of Sciences. 2016; 113 :6348. doi: 10.1073/pnas.1521270113. [ PMC free article ] [ PubMed ] [ CrossRef ] [ Google Scholar ]
- MacLean Evan L., Matthews Luke J., Hare Brian A., Nunn Charles L., Anderson Rindy C., Aureli Filippo, Brannon Elizabeth M., Call Josep, Drea Christine M., Emery Nathan J., et al. How does cognition evolve? Phylogenetic comparative psychology. Animal Cognition. 2012; 15 :223–38. doi: 10.1007/s10071-011-0448-8. [ PMC free article ] [ PubMed ] [ CrossRef ] [ Google Scholar ]
- Macphail Euan M. Brain and Intelligence in Vertebrates. Oxford University Press; Oxford: 1982. [ Google Scholar ]
- Maestripieri Dario. Primate Psychology. Harvard University Press; Cambridge: 2003. [ Google Scholar ]
- Marshall-Pescini Sarah, Dale Rachel, Quervel-Chaumette Mylene, Range Friederike. Critical issues in experimental studies of prosociality in non-human species. Animal Cognition. 2016; 19 :679–705. doi: 10.1007/s10071-016-0973-6. [ PMC free article ] [ PubMed ] [ CrossRef ] [ Google Scholar ]
- Marshall-Pescini Sarah, Schwarz Jonas F. L., Kostelnik Inga, Viranyi Zsofia, Range Friederike. Importance of a species’ socioecology: Wolves outperform dogs in a conspecific cooperation task. Proceedings of the National Academy of Sciences. 2017; 114 :11793–98. doi: 10.1073/pnas.1709027114. [ PMC free article ] [ PubMed ] [ CrossRef ] [ Google Scholar ]
- Matsubara Satoko, Deeming D. Charles, Wilkinson Anna. Cold-blooded cognition: New directions in reptile cognition. Current Opinion in Behavioral Sciences. 2017; 16 :126–30. doi: 10.1016/j.cobeha.2017.06.006. [ CrossRef ] [ Google Scholar ]
- McComb Karen, Packer Craig, Pusey Anne. Roaring and Numerical Assessment in Contests Between Groups of Female Lions, Panthera leo . Animal Behaviour. 1994; 47 :379–87. doi: 10.1006/anbe.1994.1052. [ CrossRef ] [ Google Scholar ]
- McMillan Neil, Hahn Allison H., Spetch Marcia L., Sturdy Christopher B. Avian cognition: Examples of sophisticated capabilities in space and song. Wiley Interdisciplinary Reviews: Cognitive Science. 2015; 6 :285–97. doi: 10.1002/wcs.1346. [ PubMed ] [ CrossRef ] [ Google Scholar ]
- Mendes Natacha, Steinbeis Nikolaus, Bueno-Guerra Nereida, Call Josep, Singer Tania. Preschool children and chimpanzees incur costs to watch punishment of antisocial others. Nature Human Behaviour. 2018; 2 :45–51. doi: 10.1038/s41562-017-0264-5. [ PubMed ] [ CrossRef ] [ Google Scholar ]
- Miklosi Adam. Dog Behaviour, Evolution, and Cognition. 1st ed. Oxford University Press; Oxford: 2007. [ Google Scholar ]
- Miletto Petrazzini Maria E., Wynne Clive D. What counts for dogs (Canis lupus familiaris) in a quantity discrimination task? Behavioural Processes. 2016; 122 :90–97. doi: 10.1016/j.beproc.2015.11.013. [ PubMed ] [ CrossRef ] [ Google Scholar ]
- Mitani John C., Watts David P. Why do chimpanzees hunt and share meat? Animal Behaviour. 2001; 61 :915–24. doi: 10.1006/anbe.2000.1681. [ CrossRef ] [ Google Scholar ]
- Morgan Thomas, Uomini Natalie T., Rendell Luke E., Chouinard-Thuly Laura, Street Sally E., Lewis Helen M., Cross Catherine P., Evans Cara, Kearney R., de la Torre Ignacio, et al. Experimental evidence for the co-evolution of hominin tool-making teaching and language. Nature Communications. 2015; 6 :6029. doi: 10.1038/ncomms7029. [ PMC free article ] [ PubMed ] [ CrossRef ] [ Google Scholar ]
- Osiurak François, Lesourd Mathieu, Navarro Jordan, Reynaud Emanuelle. Technition: When tools come out of the closet. Perspectives on Psychological Science. 2020 doi: 10.1177/1745691620902145. Online ahead of print. [ PubMed ] [ CrossRef ] [ Google Scholar ]
- Osthaus Britta, Lea Stephen E. G., Slater Alan M. Dogs (Canis lupus familiaris) fail to show understanding of means-end connections in a string-pulling task. Animal Cognition. 2005; 8 :37–47. doi: 10.1007/s10071-004-0230-2. [ PubMed ] [ CrossRef ] [ Google Scholar ]
- Papini Mauricio R. Pattern and process in the evolution of learning. Psychol Rev. 2002; 109 :186–201. doi: 10.1037/0033-295X.109.1.186. [ PubMed ] [ CrossRef ] [ Google Scholar ]
- Patton B. Wren, Braithwaite Victoria A. Changing tides: Ecological and historical perspectives on fish cognition. Wiley Interdisciplinary Reviews: Cognitive Science. 2015; 6 :159–76. doi: 10.1002/wcs.1337. [ PubMed ] [ CrossRef ] [ Google Scholar ]
- Pika S. Chimpanzee grooming gestures and sounds: What might they tell us about how language evolved? In: Dor Daniel, Knight Cris, Lewis Jerome., editors. The Social Origins of Language: Early Society, Communication and Polymodality. Oxford University Press; Oxford: 2014. pp. 129–40. [ Google Scholar ]
- Pika Simone, Bugnyar Thomas. The use of referential gestures in ravens (Corvus corax) in the wild. Nature Communications. 2011; 2 :560. doi: 10.1038/ncomms1567. [ PMC free article ] [ PubMed ] [ CrossRef ] [ Google Scholar ]
- Pika Simone, Mitani John. Referential gestural communication in wild chimpanzees (Pan troglodytes) Current Biology. 2006; 16 :R191–R192. doi: 10.1016/j.cub.2006.02.037. [ PubMed ] [ CrossRef ] [ Google Scholar ]
- Pika Simone, Wilkinson Ray, Kendrick Kobin H., Vernes Sonja C. Taking turns: Bridging the gap between human and animal communication. Proceedings of the Royal Society B: Biological Sciences. 2018; 285 :20180598. doi: 10.1098/rspb.2018.0598. [ PMC free article ] [ PubMed ] [ CrossRef ] [ Google Scholar ]
- Pike Thomas W., Laland Kevin N. Conformist learning in nine-spined sticklebacks’ foraging decisions. Biology Letters. 2010; 6 :466–68. doi: 10.1098/rsbl.2009.1014. [ PMC free article ] [ PubMed ] [ CrossRef ] [ Google Scholar ]
- Pinker Steven. The cognitive niche: Coevolution of intelligence, sociality, and language. Proceedings of the National Academy of Sciences. 2010; 107 :8993. doi: 10.1073/pnas.0914630107. [ PMC free article ] [ PubMed ] [ CrossRef ] [ Google Scholar ]
- Piotti Patrizia, Kaminski Juliane. Do Dogs Provide Information Helpfully? PLoS ONE. 2016; 11 :e0159797. doi: 10.1371/journal.pone.0159797. [ PMC free article ] [ PubMed ] [ CrossRef ] [ Google Scholar ]
- Polansky Leo, Kilian Werner, Wittemyer George. Elucidating the significance of spatial memory on movement decisions by African savannah elephants using state-space models. Proceedings. Biological sciences. 2015; 282 :20143042. doi: 10.1098/rspb.2014.3042. [ PMC free article ] [ PubMed ] [ CrossRef ] [ Google Scholar ]
- Povinelli Daniel J., Eddy Timothy J. What young chimpanzees know about seeing. Monographs of the Society for Research in Child Development. 1996; 61 :1–152. doi: 10.2307/1166159. [ PubMed ] [ CrossRef ] [ Google Scholar ]
- Premack David. Human and animal cognition: Continuity and discontinuity. Proceedings of the National Academy of Sciences. 2007; 104 :13861. doi: 10.1073/pnas.0706147104. [ PMC free article ] [ PubMed ] [ CrossRef ] [ Google Scholar ]
- Premack David, Woodruff Guy. Does the chimpanzee have a theory of mind? Behavioral and Brain Sciences. 1978; 1 :515–26. doi: 10.1017/S0140525X00076512. [ CrossRef ] [ Google Scholar ]
- Prétôt Laurent, Bshary Redouan, Brosnan Sarah. Factors influencing the different performance of fish and primates on a dichotomous choice task. Animal Behaviour. 2016; 119 doi: 10.1016/j.anbehav.2016.06.023. [ CrossRef ] [ Google Scholar ]
- Pusey Anne E., Schroepfer-Walker Kara. Female competition in chimpanzees. Philosophical Transactions of the Royal Society B: Biological Sciences. 2013; 368 :20130077. doi: 10.1098/rstb.2013.0077. [ PMC free article ] [ PubMed ] [ CrossRef ] [ Google Scholar ]
- Quervel-Chaumette Mylene, Mainix Gaëlle, Range Friederike, Marshall-Pescini Sarah. Dogs do not show pro-social preferences towards humans. Humans Frontier in Psycholy. 2016; 7 :1416. doi: 10.3389/fpsyg.2016.01416. [ PMC free article ] [ PubMed ] [ CrossRef ] [ Google Scholar ]
- Raby C. R., Alexis D. M., Dickinson Anthony, Clayton Nicola S. Planning for the Future by Western Scrub-Jays. Nature. 2007; 445 :919–21. doi: 10.1038/nature05575. [ PubMed ] [ CrossRef ] [ Google Scholar ]
- Range Friederike, Marshall-Pescini Sarah, Kratz Corinna, Virányi Zsófia. Wolves lead and dogs follow, but they both cooperate with humans. Scientific Reports. 2019; 9 :3796. doi: 10.1038/s41598-019-40468-y. [ PMC free article ] [ PubMed ] [ CrossRef ] [ Google Scholar ]
- Redshaw Jonathan, Taylor Alex H., Suddendorf Thomas. Flexible planning in ravens? Trends in Cognitive Sciences. 2017; 21 :821–22. doi: 10.1016/j.tics.2017.09.001. [ PubMed ] [ CrossRef ] [ Google Scholar ]
- Reznikova Z. Zhanna. Animal Intelligence: From Individual to Social Cognition. Cambridge University Press; Cambridge: 2007. [ Google Scholar ]
- Roberts William A. Are animals stuck in time? Psychological Bulletin. 2002; 128 :473–89. doi: 10.1037/0033-2909.128.3.473. [ PubMed ] [ CrossRef ] [ Google Scholar ]
- Rooijakkers Eveline, Kaminski Juliane, Call Josep. Comparing dogs and great apes in their ability to visually track object transpositions. Animal Cognition. 2009; 12 :789–96. doi: 10.1007/s10071-009-0238-8. [ PMC free article ] [ PubMed ] [ CrossRef ] [ Google Scholar ]
- Rosati Alexandra G. Foraging cognition: Reviving the Ecological Intelligence Hypothesis. Trends in Cognitive Sciences. 2017; 21 :691–702. doi: 10.1016/j.tics.2017.05.011. [ PubMed ] [ CrossRef ] [ Google Scholar ]
- Rosati Alexandra G., Hare Brian. Chimpanzees and bonobos distinguish between risk and ambiguity. Biology Letters. 2011; 7 :15–18. doi: 10.1098/rsbl.2010.0927. [ PMC free article ] [ PubMed ] [ CrossRef ] [ Google Scholar ]
- Rosati Alexandra G., Stevens Jeffrey R., Hare Brian, Hauser Marc D. The evolutionary origins of human patience: Temporal preferences in chimpanzees, bonobos, and human adults. Current Biology. 2007; 17 :1663–68. doi: 10.1016/j.cub.2007.08.033. [ PubMed ] [ CrossRef ] [ Google Scholar ]
- Roth Timothy C., Krochmal Aaaron R., LaDage Lara D. Reptilian cognition: A more complex picture via integration of neurological mechanisms, behavioral constraints, and evolutionary context. BioEssays. 2019; 41 :1900033. doi: 10.1002/bies.201900033. [ PubMed ] [ CrossRef ] [ Google Scholar ]
- Rowell Thelma. The myth of peculiar primates. In: Box Hilary O., Gibson Kathleen R., editors. Symposia of the Zoological Society of London; Mammalian Social Learning: Comparative and Ecological Perspectives. Cambridge University Press; Cambridge: 1999. [ Google Scholar ]
- Rutz Christian, Bluff Lucas A., Reed Nicola, Troscianko Jolyon, Newton Jason, Inger Richard, Kacelnik Alex, Bearhop Stuart. The ecological significance of tool use in New Caledonian Crows. Science. 2010; 329 :1523. doi: 10.1126/science.1192053. [ PubMed ] [ CrossRef ] [ Google Scholar ]
- Salwiczek Lucie H., Prétôt Laurent, Demarta Lanila, Proctor Darby, Essler Jennifer, Pinto Ana I., Wismer Sharon, Stoinski Tara, Brosnan Sarah F., Bshary Redouan. Adult Cleaner Wrasse Outperform Capuchin Monkeys, Chimpanzees and Orang-utans in a Complex Foraging Task Derived from Cleaner—Client Reef Fish Cooperation. PLoS ONE. 2012; 7 :e49068. doi: 10.1371/journal.pone.0049068. [ PMC free article ] [ PubMed ] [ CrossRef ] [ Google Scholar ]
- Seed Amanda M., Tebbich Sabine, Emery Nathan J., Clayton Nicola S. Investigating physical cognition in rooks, Corvus frugilegus . Current Biology. 2006; 16 :697–701. doi: 10.1016/j.cub.2006.02.066. [ PubMed ] [ CrossRef ] [ Google Scholar ]
- Seed Amanda, Emery Nathan, Clayton Nicola. Intelligence in corvids and apes: A case of convergent evolution? Ethology. 2009; 115 :401–20. doi: 10.1111/j.1439-0310.2009.01644.x. [ CrossRef ] [ Google Scholar ]
- Sewall Kendra B. Social complexity as a driver of communication and cognition. Integrative Comparative Biology. 2015; 55 :384–95. doi: 10.1093/icb/icv064. [ PubMed ] [ CrossRef ] [ Google Scholar ]
- Seyfarth Robert M., Cheney Dorothy L. The structure of social knowledge in monkeys. In: de Waal Frans B. M., Tyack Peter L., editors. Animal Social Complexity: Intelligence, Culture, and Individualized Societies. Harvard University Press; Cambridge: 2003. pp. 207–29. [ Google Scholar ]
- Shettleworth Sarah J. Memory in food-hoarding birds. Scientific American. 1983; 248 :102–11. doi: 10.1038/scientificamerican0383-102. [ CrossRef ] [ Google Scholar ]
- Shettleworth Sarah J. Cognition, Evolution, and Behavior. Oxford University Press; New York: 1998. [ Google Scholar ]
- Shettleworth Sarah J. Modularity and the evolution of cognition. In: Heyes Cecilia, Huber Ludwig., editors. The Evolution of Cognition. MIT Press; Cambridge: 2000. pp. 43–60. [ Google Scholar ]
- Shettleworth Sarah J. The evolution of comparative cognition: Is the snark still a boojum? Behavioural Processes. 2009; 80 :210–17. doi: 10.1016/j.beproc.2008.09.001. [ PubMed ] [ CrossRef ] [ Google Scholar ]
- Shettleworth Sarah J. Clever animals and killjoy explanations in comparative psychology. Trends in Cognitive Sciences. 2010; 14 :477–81. doi: 10.1016/j.tics.2010.07.002. [ PubMed ] [ CrossRef ] [ Google Scholar ]
- Shettleworth Sarah J. Modularity, comparative cognition and human uniqueness. Philosophical Transactions of the Royal Society B: Biological Sciences. 2012; 367 :2794–802. doi: 10.1098/rstb.2012.0211. [ PMC free article ] [ PubMed ] [ CrossRef ] [ Google Scholar ]
- Sol Daniel. Revisiting the cognitive buffer hypothesis for the evolution of large brains. Biology Letters. 2009; 5 :130–33. doi: 10.1098/rsbl.2008.0621. [ PMC free article ] [ PubMed ] [ CrossRef ] [ Google Scholar ]
- Stanton Lauren, Davis Emely, Johnson Shylo, Gilbert Amy, Benson-Amram Sarah. Adaptation of the Aesop’s Fable paradigm for use with raccoons ( Procyon lotor ): Considerations for future application in non-avian and non-primate species. Animal Cognition. 2017; 20 :1147–52. doi: 10.1007/s10071-017-1129-z. [ PubMed ] [ CrossRef ] [ Google Scholar ]
- Sterck Elisabeth H. M., Watts David P., van Schaik Carel P. The evolution of female social relationships in nonhuman primates. Behavioral Ecology and Sociobiology. 1997; 41 :291–309. doi: 10.1007/s002650050390. [ CrossRef ] [ Google Scholar ]
- Sterelny Kim. Thought in a Hostile World: The Evolution of Human Cognition. Malden; Blackwell: 2003. [ Google Scholar ]
- Suddendorf Thomas, Busby Janie. Mental Time Travel in Animals? Trends in Cognitive Sciences. 2003; 7 :391–96. doi: 10.1016/S1364-6613(03)00187-6. [ PubMed ] [ CrossRef ] [ Google Scholar ]
- Surbeck Martin, Hohmann Gottfried. Primate hunting by bonobos at LuiKotale, Salonga National Park. Current Biology. 2008; 18 :R906–R907. doi: 10.1016/j.cub.2008.08.040. [ PubMed ] [ CrossRef ] [ Google Scholar ]
- Taylor Alex H., Gray Russell D. Is there a link between the crafting of tools and the evolution of cognition? Wiley Interdisciplinary Reviews: Cognitive Science. 2014; 5 :693–703. doi: 10.1002/wcs.1322. [ PubMed ] [ CrossRef ] [ Google Scholar ]
- Taylor Alex H., Hunt Gavin R., Holzhaider Jennifer C., Gray Russell D. Spontaneous metatool use by new caledonian crows. Current Biology. 2007; 17 :1504–7. doi: 10.1016/j.cub.2007.07.057. [ PubMed ] [ CrossRef ] [ Google Scholar ]
- Taylor Alex H., Hunt Gavin R., Medina Felipe S., Gray Russell D. Do New Caledonian crows solve physical problems through causal reasoning? Proceedings of the Royal Society B: Biological Sciences. 2009; 276 :247–54. doi: 10.1098/rspb.2008.1107. [ PMC free article ] [ PubMed ] [ CrossRef ] [ Google Scholar ]
- Taylor Alex H., Medina Felipe S., Holzhaider Jennifer C., Hearne Lindsay J., Hunt Gavin R., Gray Russell D. An investigation into the cognition behind spontaneous string pulling in New Caledonian Crows. PLoS ONE. 2010; 5 :e9345. doi: 10.1371/journal.pone.0009345. [ PMC free article ] [ PubMed ] [ CrossRef ] [ Google Scholar ]
- Taylor Alex H., Knaebe Brenna, Gray Russell D. An end to insight? New Caledonian crows can spontaneously solve problems without planning their actions. Proceedings of the Royal Society B: Biological Sciences. 2012a; 279 :4977. doi: 10.1098/rspb.2012.1998. [ PMC free article ] [ PubMed ] [ CrossRef ] [ Google Scholar ]
- Taylor Alex H., Hunt Gavin R., Gray Russell D. Context-dependent tool use in New Caledonian crows. Biology Letters. 2012b; 8 :205. doi: 10.1098/rsbl.2011.0782. [ PMC free article ] [ PubMed ] [ CrossRef ] [ Google Scholar ]
- Taylor Alex H., Cheke Lucy G., Waismeyer Anna, Meltzoff Andrew N., Miller Rachael, Gopnik Alison, Clayton Nicola S., Gray Russell D. Of babies and birds: Complex tool behaviours are not sufficient for the evolution of the ability to create a novel causal intervention. Proceedings of the Royal Society B: Biological Sciences. 2014; 281 :20140837. doi: 10.1098/rspb.2014.0837. [ PMC free article ] [ PubMed ] [ CrossRef ] [ Google Scholar ]
- Tebbich Sabine, Seed Amanda M., Emery Nathan J., Clayton Nicola S. Non-tool-using rooks, Corvus frugilegus , solve the trap-tube problem. Animal Cognition. 2007; 10 :225–31. doi: 10.1007/s10071-006-0061-4. [ PubMed ] [ CrossRef ] [ Google Scholar ]
- Tennie Claudio, Call Josep, Tomasello Michael. Ratcheting up the ratchet: On the evolution of cumulative culture. Philosophical Transactions of the Royal Society of London B Biological Sciences. 2009; 364 :2405–15. doi: 10.1098/rstb.2009.0052. [ PMC free article ] [ PubMed ] [ CrossRef ] [ Google Scholar ]
- Teschke Irmgard, Cartmill E. A., Stankewitz S., Tebbich Sabine. Sometimes tool use is not the key: No evidence for cognitive adaptive specializations in tool-using woodpecker finches. Animal Behaviour. 2011; 82 :945–56. doi: 10.1016/j.anbehav.2011.07.032. [ CrossRef ] [ Google Scholar ]
- Teschke Irmgard, Wascher Claudia A. F., Scriba Madeleine, von Bayern Auguste M. P., Huml Jana Vanessa, Siemers B., Tebbich Sabine. Did tool-use evolve with enhanced physical cognitive abilities? Philosophical transactions of the Royal Society of London. Series B, Biological Sciences. 2013; 368 :20120418. doi: 10.1098/rstb.2012.0418. [ PMC free article ] [ PubMed ] [ CrossRef ] [ Google Scholar ]
- Thalmann Olaf, Shapiro B., Cui P., Schuenemann V. J., Sawyer S. K., Greenfield D. L., Germonpré M. B., Sablin M. V., López-Giráldez S., Domingo-Roura X., et al. Complete mitochondrial genomes of ancient canids suggest a european origin of domestic dogs. Science. 2013; 342 :871–74. doi: 10.1126/science.1243650. [ PubMed ] [ CrossRef ] [ Google Scholar ]
- Tobin Henry, Logue A. W., Chelonis John J., Ackerman Kimberly T., May Jack G. Self-control in the monkey Macaca fascicularis . Animal Learning and Behavior. 1996; 24 :168–74. doi: 10.3758/BF03198964. [ CrossRef ] [ Google Scholar ]
- Tomasello Michael. Becoming Human: A Theory of Ontogeny. Belknap Press; Cambridge: 2019. [ Google Scholar ]
- Tomasello Michael, Call Josep. Primate Cognition. Oxford University Press; New York: 1997. [ Google Scholar ]
- Tomasello Michael, Call Josep. Assessing the Validity of Ape-Human Comparisons: A Reply to Boesch (2007) Journal of Comparative Psychology. 2008; 122 :449–52. doi: 10.1037/0735-7036.122.4.449. [ PubMed ] [ CrossRef ] [ Google Scholar ]
- Tomasello Michael, Herrmann Esther. Ape and human cognition: What’s the difference? Current Directions in Psychological Science. 2010; 19 :3–8. doi: 10.1177/0963721409359300. [ CrossRef ] [ Google Scholar ]
- Tomasello Michael, Carpenter Malinda, Call Josep, Behne Tanya, Moll Henrike. Understanding and sharing intentions: The origins of cultural cognition. Behavioral and Brain Sciences. 2005; 28 :675–735. doi: 10.1017/S0140525X05000129. [ PubMed ] [ CrossRef ] [ Google Scholar ]
- Tomonaga Masaki, Kumazaki Kiyonori, Camus Florine, Nicod Sophie, Pereira Carlos, Matsuzawa Tetsuro. A horse’s eye view: Size and shape discrimination compared with other mammals. Biology Letters. 2015; 11 :20150701. doi: 10.1098/rsbl.2015.0701. [ PMC free article ] [ PubMed ] [ CrossRef ] [ Google Scholar ]
- Uomini Natalie. Cognition and culture: The potential for archaeology. Commentary on Haidle. Erwägen Wissen Ethik (Deliberation Knowledge Ethics) 2008; 19 :50–53. [ Google Scholar ]
- Uomini Natalie. Prehistoric left-handers and prehistoric language. In: de Beaune S. A., Coolidge F. L., editors. The Emergence of Cognitive Abilities: The Contribution of Neuropsychology to Archaeology. Cambridge University Press; Cambridge: 2009. pp. 37–55. [ Google Scholar ]
- Uomini Natalie. Paleoneurology and behaviour. In: Bruner Emiliano., editor. Human Paleoneurology. vol. 3. Springer; Cham: 2014. pp. 121–43. (Springer Series in Bio-/Neuroinformatics). [ Google Scholar ]
- Uomini Natalie. Neuroarchaeology: Language and tools in the brain. Nature Human Behaviour. 2017 doi: 10.1038/s41562-017-0114. [ CrossRef ] [ Google Scholar ]
- Uomini Natalie, Hunt Gavin. A new tool-using bird to crow about. Learning and Behaviour. 2017 doi: 10.3758/s13420-017-0262-5. [ PMC free article ] [ PubMed ] [ CrossRef ] [ Google Scholar ]
- Uomini Natalie, Meyer Georg. Shared cerebral blood flow lateralization patterns in language and stone tool production. PLoS ONE. 2013; 8 :e72693. doi: 10.1371/journal.pone.0072693. [ PMC free article ] [ PubMed ] [ CrossRef ] [ Google Scholar ]
- Uomini Natalie, Ruck Lana. Manual laterality and cognition through evolution: An archaeological perspective. Progress in Brain Research. 2018; 238 :295–323. [ PubMed ] [ Google Scholar ]
- Uomini Natalie, Fairlie Joanna, Gray Russell D., Griesser Michael. Extended parenting and the evolution of cognition. Philosophical Transactions of the Royal Society B. 2020 doi: 10.1098/rstb.2019.0495. [ PMC free article ] [ PubMed ] [ CrossRef ] [ Google Scholar ]
- Urhan A. Utku, Emilsson Ellen, Brodin Anders. Evidence against observational spatial memory for cache locations of conspecifics in marsh tits Poecile palustris. Behavioral Ecology and Sociobiology. 2017; 71 :34. doi: 10.1007/s00265-016-2264-2. [ PMC free article ] [ PubMed ] [ CrossRef ] [ Google Scholar ]
- Vail Alexander, Manica Andrea, Bshary Redouan. Referential gestures in fish collaborative hunting. Nature Communications. 2013; 4 :1765. doi: 10.1038/ncomms2781. [ PubMed ] [ CrossRef ] [ Google Scholar ]
- Vasilieva Olga. Beyond “uniqueness”: Habitual traits in the context of cognitive-communicative continuity. Theoria et Historia Scientiarum. 2019; 16 :129–50. doi: 10.12775/ths.2019.008. [ CrossRef ] [ Google Scholar ]
- Veit Ariane, Wondrak Marianne, Huber Ludwig. Object movement re-enactment in free-ranging Kune Kune piglets. Animal Behaviour. 2017; 132 :49–59. doi: 10.1016/j.anbehav.2017.08.004. [ CrossRef ] [ Google Scholar ]
- Vermaercke Ben, Cop Elsy, Willems Sam, D’Hooge Rudi, de Beeck Hans P. Op. More complex brains are not always better: Rats outperform humans in implicit category-based generalization by implementing a similarity-based strategy. Psychonomic Bulletin & Review. 2014; 21 :1080–6. doi: 10.3758/s13423-013-0579-9. [ PubMed ] [ CrossRef ] [ Google Scholar ]
- Vlamings Petra, Hare Brian, Call Josep. Reaching around barriers: The performance of the great apes and 3–5-year-old children. Animal Cognition. 2010; 13 :273–85. doi: 10.1007/s10071-009-0265-5. [ PMC free article ] [ PubMed ] [ CrossRef ] [ Google Scholar ]
- Vonk Jennifer. Advances in animal cognition. Behavioral Sciences. 2016; 6 :27. doi: 10.3390/bs6040027. [ PMC free article ] [ PubMed ] [ CrossRef ] [ Google Scholar ]
- Vonk Jennifer, Povinelli Daniel J. Similarity and difference in the conceptual systems of primates: The unobservability hypothesis. In: Zentall Thomas R., Wasserman Edward A., editors. The Oxford Handbook of Comparative Cognition. Oxford University Press; Oxford: 2012. pp. 552–75. [ Google Scholar ]
- Wasserman Edward A., Zentall Thomas R., editors. Comparative Cognition: Experimental Explorations of Animal Intelligence. Oxford University Press; Oxford: 2006. [ Google Scholar ]
- Watanabe Shigeru. Van Gogh, Chagall and pigeons: Picture discrimination in pigeons and humans. Animal Cognition. 2001; 4 :147–51. doi: 10.1007/s100710100112. [ PubMed ] [ CrossRef ] [ Google Scholar ]
- Watanabe Shigeru, Sakamoto Junko, Wakita Masumi. Pigeons’ discrimination of paintings by Monet and Picasso. Journal of the Experimental Analysis of Behavior. 1995; 63 :165–74. doi: 10.1901/jeab.1995.63-165. [ PMC free article ] [ PubMed ] [ CrossRef ] [ Google Scholar ]
- Webb Barbara A. Cognition in insects. Journal of the Philosophical Transactions of the Royal Society of London. Series B, Biological Sciences. 2012; 367 :2715–22. doi: 10.1098/rstb.2012.0218. [ PMC free article ] [ PubMed ] [ CrossRef ] [ Google Scholar ]
- Weir Alex A., Chappell Jackie, Kacelnik Alex. Shaping of hooks in new caledonian crows. Science. 2002; 297 :981. doi: 10.1126/science.1073433. [ PubMed ] [ CrossRef ] [ Google Scholar ]
- Werdenich Dagmar, Huber Ludwig. A case of quick problem solving in birds: String pulling in keas, Nestor notabilis. Animal Behaviour. 2006; 71 :855–63. doi: 10.1016/j.anbehav.2005.06.018. [ CrossRef ] [ Google Scholar ]
- Wismer Sharon, Grutter Alexandra, Bshary Redouan. Generalized rule application in bluestreak cleaner wrasse (Labroides dimidiatus): Using predator species as social tools to reduce punishment. Animal Cognition. 2016; 19 doi: 10.1007/s10071-016-0975-4. [ PubMed ] [ CrossRef ] [ Google Scholar ]
- Wittig Roman, Boesch Christophe. Food competition and linear dominance hierarchy among female chimpanzees of the Tai National Park. International Journal of Primatology. 2003; 24 :847–67. doi: 10.1023/A:1024632923180. [ CrossRef ] [ Google Scholar ]
- Wondrak Marianne, Conzelmann Elin, Veit Ariane, Huber Ludwig. Pigs (Sus scrofa domesticus) categorize pictures of human heads. Applied Animal Behaviour Science. 2018; 205 :19–27. doi: 10.1016/j.applanim.2018.05.009. [ CrossRef ] [ Google Scholar ]
- Wrangham Richard W., Conklin-Brittain Nancy L., Hunt Kevin D. Dietary response of chimpanzees and cercopithecines to seasonal variation in fruit abundance. International Journal of Primatology. 1998; 19 :949. doi: 10.1023/A:1020318102257. [ CrossRef ] [ Google Scholar ]
- Zentall Thomas R., Case Jacob P. The Ephemeral-Reward Task: Optimal Performance Depends on Reducing Impulsive Choice. Current Directions in Psychological Science. 2018; 27 :103–9. doi: 10.1177/0963721417735522. [ CrossRef ] [ Google Scholar ]
- Zentall Thomas R., Wasserman Edward A., editors. The Oxford Handbook of Comparative Cognition. Oxford University Press; Oxford: 2012. [ Google Scholar ]
Last updated 10th July 2024: Online ordering is currently unavailable due to technical issues. We apologise for any delays responding to customers while we resolve this. For further updates please visit our website https://www.cambridge.org/news-and-insights/technical-incident
We use cookies to distinguish you from other users and to provide you with a better experience on our websites. Close this message to accept cookies or find out how to manage your cookie settings .
Login Alert

- > The Cambridge Handbook of Animal Cognition
- > Innovation and Problem-Solving Overview
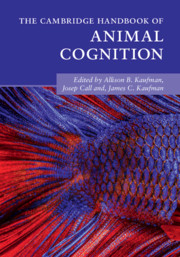
Book contents
- The Cambridge Handbook of Animal Cognition
- Copyright page
- Figures, Tables, and Boxes
- Contributors
- Acknowledgments
- Introduction
- Part I Communication and Language
- Part II Memory and Recall
- Part III Social Cognition
- Part IV Social Learning and Teaching
- Part V Numerical and Quantitative Abilities
- Part VI Innovation and Problem-Solving
- 28 Innovation and Problem-Solving Overview
- 29 General Intelligence ( g ) in Mice
- 30 Bowerbird Innovation and Problem-Solving
- 31 Parrot Innovation
- 32 Innovation in Marine Mammals
- 33 Innovation in Capuchin Monkeys
- 34 Innovation and Problem-Solving in Orangutans
- 35 Do Apes and Monkeys Know What They (Don’t) Know?
- 36 Decision Making in Animals
28 - Innovation and Problem-Solving Overview
from Part VI - Innovation and Problem-Solving
Published online by Cambridge University Press: 01 July 2021
- 29 General Intelligence (g) in Mice
Innovation – the process that generates novel learned behaviours – is a defining feature of intelligence, and has long attracted the interest of scientists for its implications in brain evolution, emergence of culture, and adaptation to environmental changes. Although most animals have the capacity to innovate, only a few excel in their innovative capacities. A salient feature of these animals is a highly encephalized brain, which provides the cognitive basis for complex behaviors. Highly innovative animals also tend to be ecological generalists, long-lived and sociable, features that are thought to enhance the payoff of innovation. The evolutionary origin of innovative abilities is unclear, however, because innovating implies coping with problems the animal has not experienced before. A possibility is to consider innovation as an emergent property that results from the combination of cognitive and noncognitive traits that have coevolved as part of a life-history syndrome to cope with environmental changes. The coevolution of innovation and social learning capacities is particularly relevant because it has facilitated the accumulation of the knowledge needed for more complex behaviours. The ability to socially transmit knowledge may thus be behind the exceptional variety and sophistication of human innovations.
Access options
Save book to kindle.
To save this book to your Kindle, first ensure [email protected] is added to your Approved Personal Document E-mail List under your Personal Document Settings on the Manage Your Content and Devices page of your Amazon account. Then enter the ‘name’ part of your Kindle email address below. Find out more about saving to your Kindle .
Note you can select to save to either the @free.kindle.com or @kindle.com variations. ‘@free.kindle.com’ emails are free but can only be saved to your device when it is connected to wi-fi. ‘@kindle.com’ emails can be delivered even when you are not connected to wi-fi, but note that service fees apply.
Find out more about the Kindle Personal Document Service .
- Innovation and Problem-Solving Overview
- By Daniel Sol
- Edited by Allison B. Kaufman , University of Connecticut , Josep Call , University of St Andrews, Scotland , James C. Kaufman , University of Connecticut
- Book: The Cambridge Handbook of Animal Cognition
- Online publication: 01 July 2021
- Chapter DOI: https://doi.org/10.1017/9781108564113.035
Save book to Dropbox
To save content items to your account, please confirm that you agree to abide by our usage policies. If this is the first time you use this feature, you will be asked to authorise Cambridge Core to connect with your account. Find out more about saving content to Dropbox .
Save book to Google Drive
To save content items to your account, please confirm that you agree to abide by our usage policies. If this is the first time you use this feature, you will be asked to authorise Cambridge Core to connect with your account. Find out more about saving content to Google Drive .
Thank you for visiting nature.com. You are using a browser version with limited support for CSS. To obtain the best experience, we recommend you use a more up to date browser (or turn off compatibility mode in Internet Explorer). In the meantime, to ensure continued support, we are displaying the site without styles and JavaScript.
- View all journals
- Explore content
- About the journal
- Publish with us
- Sign up for alerts
- Open access
- Published: 29 December 2022
Breed differences in social cognition, inhibitory control, and spatial problem-solving ability in the domestic dog ( Canis familiaris )
- Saara Junttila 1 ,
- Anna Valros 1 ,
- Katariina Mäki 2 ,
- Heli Väätäjä 3 ,
- Elisa Reunanen 4 &
- Katriina Tiira 5 , 6
Scientific Reports volume 12 , Article number: 22529 ( 2022 ) Cite this article
281k Accesses
9 Citations
1732 Altmetric
Metrics details
- Animal behaviour
- Learning and memory
- Social behaviour
This article has been updated
The extraordinary genetic and behavioural diversity of dog breeds provides a unique opportunity for investigating the heritability of cognitive traits, such as problem-solving ability, social cognition, inhibitory control, and memory. Previous studies have mainly investigated cognitive differences between breed groups, and information on individual dog breeds is scarce. As a result, findings are often contradictory and inconsistent. The aim of this study was to provide more clarity on between-breed differences of cognitive traits in dogs. We examined the performance of 13 dog breeds (N = 1002 dogs) in a standardized test battery. Significant breed differences were found for understanding of human communicative gestures, following a human’s misleading gesture, spatial problem-solving ability in a V-detour task, inhibitory control in a cylinder test, and persistence and human-directed behaviour during an unsolvable task. Breeds also differed significantly in their behaviour towards an unfamiliar person, activity level, and exploration of a novel environment. No significant differences were identified in tasks measuring memory or logical reasoning. Breed differences thus emerged mainly in tasks measuring social cognition, problem-solving, and inhibitory control. Our results suggest that these traits may have come under diversifying artificial selection in different breeds. These results provide a deeper understanding on breed-specific traits in dogs.
Similar content being viewed by others
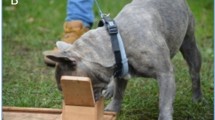
The difference between two brachycephalic and one mesocephalic dog breeds’ problem-solving performance suggests evidence for paedomorphism in behaviour
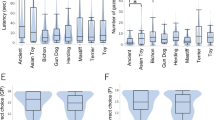
Identification of genes associated with human-canine communication in canine evolution
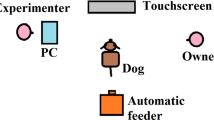
Individual Differences in Response to Ambiguous Stimuli in a Modified Go/No-Go Paradigm are Associated with Personality in Family Dogs
Introduction.
Cognitive abilities (such as learning, memory, inhibitory control, problem-solving, and social cognition) are important traits in almost all aspects of an animal’s life, from finding food to cooperating with conspecifics. Despite this, the heritability of cognitive traits in non-human animals is still a largely unknown topic 1 . The domestic dog ( Canis familiaris ), with its extraordinary genetic and phenotypic diversity, provides a unique opportunity for advancing our understanding of this subject. Behavioural variation between dog breeds is substantial 2 , and there is evidence that many of these breed differences are attributable to genetic factors 3 (but see Morrill et al. 4 ).
Cognitive differences between breeds have been investigated to some extent, but the results seem to be largely contradictory and inconsistent. For example, studies have demonstrated differences in breeds’ understanding of human referential cues, such as pointing or gazing 5 , 6 , 7 , 8 , and genetic relatedness between breeds seems to account for a substantial portion of variation in this trait 9 . Several other studies, however, have not been able to replicate these results 10 , 11 , 12 , 13 . Similarly, studies have failed to find any significant differences between breeds for logical reasoning ability 13 , inhibitory control 13 , or memory 12 , 13 , although Gnanadesikan et al. 9 demonstrated that genetic relatedness among breeds accounted for a significant proportion of variation in these traits.
The difficulty in interpreting previous results lies partly in the fact that most studies have grouped breeds together, either based on original function or genetic relatedness. What further complicates interpretation is that across studies, groups are often composed of different breeds, and the criteria for categorizing breeds differ greatly. Some authors have questioned the use of breed group classifications based on the breeds’ original purpose 14 , 15 , 16 , 17 , mainly because selection pressures may have changed drastically. For instance, Svartberg 17 found no associations between behavioural profiles of breeds and their original purpose; instead, breed-typical behaviour was correlated with the current use of the breed. Even grouping breeds based on their genetic relatedness is not without its faults. For example, both Svartberg 17 and Turcsán et al. 16 used cluster analysis to group breeds based on behavioural similarity, and found that these clusters corresponded poorly with the genetic categorization of breeds. These results suggest that when breeds are divided into groups, important differences between breeds may be missed.
Only a handful of studies have compared cognitive differences between individual breeds instead of breed groups 7 , 8 , 12 , 14 , 18 , 19 , 20 . Unfortunately, most of these have involved restricted sample sizes and a limited number of breeds. Moreover, very little empirical research has targeted non-social cognitive traits such as memory, inhibitory control, spatial problem-solving, and logical reasoning. The aim of our study was to provide a more complete picture of cognitive differences between dog breeds. We explored breed differences not only in dogs’ socio-cognitive abilities, but also in several cognitive traits not involving a social aspect. In addition, we compared breeds regarding their exploration in a novel space, greeting an unfamiliar person, and activity level, since these may also be linked to cognitive performance 21 , 22 , 23 , 24 . Our large sample size allowed us to look at differences between individual breeds rather than having to resort to breed group classifications. Our aim was to provide a more comprehensive understanding of how breeds vary cognitively and behaviourally, which improves our ability to predict how individual dogs are likely to behave. Our results may also further our understanding of the heritability and genetics of cognition, both in humans and in other animals.
A total of 2,352 adult dogs participated in a smartDOG™ test battery 25 between March 2016 and February 2022. Participating dogs were required to be interested in working for food, and to not be overly aggressive to people. We limited the analysis to dogs between the ages of 1 and 8 years, since cognitive traits may not have fully developed in younger dogs 26 , 27 , while older dogs may experience cognitive decline 28 . We included only dog breeds with a minimum of 40 individuals tested per breed. This resulted in a final sample size of 1,002 dogs representing 13 breeds, including one category consisting of mixed breeds. Any individual which had parents belonging to different breeds was classified as mixed breed, except for the Labradoodle. Details of participant dogs’ ages, sexes, and breeds are presented in Table 1 .
Participant dogs took part in a test battery involving multiple tests, but not all dogs had results for every test included in this study (see Supplementary Table S1 for number of participant dogs in each test section). Most of the results for each test came from the same dogs, with some results missing, mostly because 17% of the dogs took part in a shortened version of the test battery (see Supplementary Materials S1 for details).
Most participant dogs were privately owned pet dogs. We did not have information on the training history of most dogs, apart from 31 police dogs (see Suppl. Table S2 ). A large proportion of the pet dogs were actively used in various dog sports (e.g., agility, scent work, obedience, etc.), based on discussions the testers have had with participating owners. Most dogs (including the police dogs) lived inside the house with their owners.
Cognitive test battery
Dogs included in the study were participants in a commercial cognitive test battery (smartDOG™) 25 , which was developed by one of the authors (KT) based on previous scientific publications. Tests were performed by eight trained female smartDOG licence testers (including KT) at testing sites across Finland. We included 10 tests, seven of which measured cognitive traits and three of which measured behaviour. Descriptions of included tests are outlined in Table 2 .
All tests involved solving various problems with food rewards. The owners were asked to bring the dog’s favourite treats, which were then used as rewards. In some cases, a toy was also used, if the dog was more motivated by toys than food. The owner was advised not to feed their dog prior to the test to ensure food motivation during the test. Fresh drinking water was available throughout the test. Testing took place indoors, and the minimum size of the testing room was approximately 30 m 2 . Most often only the tester, owner, and dog were present during testing, but occasionally family members were present as observers. The dog was off the lead throughout the test battery. A short break was included in the COGNITION test battery, during which the owner was asked to walk the dog outside for a maximum of two minutes.
The order of tests was the same for all dogs to ensure consistency across subjects. Each test included several trials. At the beginning of each trial, the tester always drew the dog’s attention, if necessary, by showing them a treat and saying the dog’s name. A (Finnish) word, such as “ok” or similar, was used to indicate to the owner the beginning of each trial. The owner was advised to release the dog upon hearing this word during the test battery. After each trial, the owner called or moved the dog back to the starting position using the collar and held the dog in place before releasing the dog for the next trial. The owner was advised to remain quiet and neutral when the dog was focusing on a task, but they were allowed to praise the dog when the dog ate a treat. Only during the V-detour was the owner allowed to encourage the dog to continue if the dog continuously stared at the owner. Between test sections, the dog was free to walk around for about 1–2 min.
A short description of each test is provided below, and more detailed information can be found in the Supplementary Information S1 . An example of each cognitive test can also be found in the supplementary videos S1 .
When the owner and dog first entered the test room, the dog’s response to the tester (an unknown person) was rated. The tester faced the dog while talking in a friendly voice and allowed the dog to approach herself. If the dog was not fearful or aggressive, the tester bent down and attempted to stroke the dog. The tester then continued stroking the dog as long as the dog was willing. This test lasted between 1–2 min in total.
The tester rated the dog’s response according to a scale ranging from 1 to 7. For analysis purposes, these scores were condensed into four groups: ‘fearful’ (score 1–3), ‘indifferent’ (score 4–5), ‘friendly’ (score 6), and ‘overexcited’ (score 7). The largest category (‘friendly’) was used as the reference category.
Activity level
After the tester had greeted the dog, she attached a FitBark (FitBark Inc., Kansas City, MO, USA) activity monitor to the dog’s collar or harness. FitBark generates ‘BarkPoints’ (from here on referred to as ‘activity points’), which represent a proxy measure for the average activity level of the dog during the test battery. The monitor was kept on throughout testing and taken off when the test battery ended. Only dogs which had taken part in the COGNITION test battery were analysed.
Exploration
After the FitBark had been attached, the dog was released and allowed to freely explore the test room for approximately five minutes. The tester rated the dog’s behaviour on a scale of 1–5. These scores were condensed into four groups: ‘low investigation’ (score 1–2), ‘moderate investigation’ (score 3), active investigation, walking (score 4), and very active investigation, running (score 5). The aim of this test was to measure the extent to which the dogs were willing to investigate a novel environment, which is thought to be an indication of curiosity, boldness, and activity level, whereas remaining by the owner’s side was thought to be a possible indication of fear, anxiety, or neophobia.
The cylinder test
The cylinder test has been used extensively in animal cognition research to study impulsivity and inhibitory control 29 , more specifically the motor inhibitory response. Inhibitory control is a core executive function, which involves suppression of a prepotent but inefficient behaviour in favour of a more beneficial response. In this test, the dog is required to inhibit reaching directly for a visible food reward and instead detour around the transparent barrier to reach the reward.
The owner and dog were positioned 2–2.5 m away from the cylinder. During the training trials, the cylinder was opaque, and the dog was taught to access a food reward from either of the open sides. The experimenter stood directly behind the cylinder and placed a food reward inside while the dog was watching. The dog was then released and allowed to eat the treat (Supplementary Video S1 ). After the dog fulfilled the learning criteria (4 out of 5 trials without touching the outside of the cylinder), the test phase began. During the test phase the cylinder was transparent, and the dog was required to inhibit reaching for the now visible food directly, and to instead go around to the side of the cylinder to access the reward (Supplementary Video S2 ). Each trial during which the dog touched the outside of the cylinder was marked as an incorrect trial. If the dog ate the food without first touching the outside of the cylinder, this was marked as a correct trial. Percentage of correct responses (out of a total of 10 trials) was used as the response variable.
Human gestures
Dogs’ understanding of pointing and other human gestures is often used as a measure of social cognition 30 , 31 . The test battery included five different gestures. Before the test phase, the dog was familiarized with the test set-up over four training trials, during which the dog was simply taught that food is available in either of the two bowls. (See Supplementary Video S3 for the training phase procedure.).
During the test phase, dogs took part in six trials for each gesture (30 trials in total). (See Supplementary Video S4 for the test procedure for each of the five gestures.) The percentage of correct responses (out of 30 trials) was calculated from all the gesture tests combined. The order of rewarded bowls was the same for each dog, starting with the left side. Every other trial was rewarded to the right and every other to the left. In order to ensure dogs were not learning this pattern, the percentage of correct responses from the final 6 trials (gaze) were compared to the percentage of correct responses from the first 6 trials (dynamic distal pointing) using a two-tailed paired t-test.
The trial always started with the dog and owner facing the tester, standing 2–2.5 m away. The tester showed a piece of food to the dog and placed it inside one of the bowls which she held in her hands. The bowls were placed on the floor in front of the tester, 95 cm apart from each other. Making sure the dog was watching, the tester provided the gesture (these are described in more detail below). After this, the dog was released and allowed to make a choice.
The procedure for each gesture was the same as described above. Each dog received the gestures in the same order (6 trials each): (1) dynamic distal pointing: the tester pointed at the correct bowl with an extended arm and index finger after which the dog was released, and the tester kept her arm in the same position while the dog made their choice, (2) momentary distal pointing: the tester pointed at the correct bowl with an extended arm and index finger for a duration of 2 s, after which the tester lowered her arm and the dog was released while the tester’s arms were flush at her sides, (3) dynamic proximal foot pointing: the tester placed the tip of her foot on the ground directly behind the correct bowl, and the dog was released while the tester remained in this position, (4) dynamic cross-forward pointing: THE tester used her contralateral arm to point at the correct bowl while rotating her shoulders in the same direction, sustaining this position while the dog made their choice, (5) gaze: the tester alternated her gaze between the dog and the bowl three times, and the dog was released while the tester maintained her gaze on the correct bowl.
The V-detour has been used in canine cognitive research to investigate spatial problem-solving ability 29 . The dog has to detour around a transparent V-shaped fence to access a food reward which is placed on the other side (Supplementary Video S5 ). Since the dog is required to move away from the visible treat to access it, the task is also often considered to measure inhibitory control.
The V-shaped fence was made out of compost fence panels, which were attached at an angle of approximately 70°. The owner and dog waited about 40 cm away from the intersecting angle of the V-shaped fence. The tester showed the dog several treats (or a toy) and placed them inside the fence while standing outside the fence. The owner released the dog while the dog was looking at the food. The number of seconds taken to solve the task was measured using a stopwatch. If the dog was not able to solve the task within 3 min, the trial was terminated.
Unsolvable task
The unsolvable task has been used in canine cognitive research to assess persistence, problem-solving behaviour, human-directed communication, and social cognition 32 . In our version of this task, the dog was presented with four solvable trials, after which the task became impossible to solve (Supplementary Video S6 ).
The test involved a plastic or wooden box with a transparent lid, which had small holes to allow the dog to smell the food inside. During the four training trials, the dog was taught to access a treat placed inside the box by moving the plastic lid off. The difficulty of the trials increased gradually. Once the dog was successful in opening the lid, the test trial was begun. With the dog watching, the tester placed several treats inside the box. She then secured the lid in place so that it could not be opened, after which the owner released the dog. Both owner and experimenter remained quiet and still, looking only at the box during the subsequent 2-min period. The tester measured the time the dog spent on each behaviour: (a) independent problem-solving: attempting to solve the task independently, (b) human-directed behaviour: initiating social contact with either the tester or the owner, or (c) abandoning the task: not directing their behaviour toward the task or a person.
Three variables were used to measure the dogs’ behaviour during the unsolvable task. (1) Complete independence (comparing dogs which did not spend any time on human-directed behaviour to those which spent any amount of time on human-directed behaviour), (2) Percentage of time spent on human-directed behaviour, and (3) Abandoning task (comparing dogs which abandoned the task to those which never abandoned the task).
Logical reasoning
This test aimed to measure the dog’s ability to make inferences based on exclusion 33 . The dog could see that one of two bowls was empty, and it had to infer that the treat was hidden under the other bowl. The tester sat on a chair or on the floor, about 1 m away from the dog. Two opaque bowls were placed upside down in front of the tester at arm’s length, one on the left and one on the right side. In each trial, a piece of food was placed under one of the bowls. The order of baiting was the same for each dog; first the left-hand bowl was baited, after which every other trial was baited to the right and every other trial to the left. The dog first took part in a training phase consisting of four trials, the aim of which was to familiarize the dog with the set-up and learn that there is always food hidden under one of the bowls. (See Supplementary Video S7 for the procedure of the training phase.).
When the dog correctly performed all four training trials, the test phase was initiated. This consisted of six trials. The tester held a writing pad as a visual barrier in front of the left-hand bowl and placed a treat under the bowl. She then placed the writing pad in front of the right-hand bowl and sham-baited the bowl. The writing pad was then removed, and the tester lifted the empty bowl up about 30 cm above the floor, keeping it there for about 1–2 s while the dog was watching. At the same time, the tester held her other hand on top of the baited bowl. The bowl was then placed back down, the tester placed her hands on her lap, and the dog was released. If the dog approached the correct bowl, they were allowed to eat the treat. The trial was then repeated another five times, every other trial rewarded on the right and every other on the left. (See Supplementary Video S8 for the procedure of the test phase.).
Two variables were used to measure logical reasoning of dogs: (a) percentage of correct responses and (b) understanding of the task. For percentage of correct responses, dogs were divided into three groups: (1) 0–50% of trials correct, (2) 51–82% of trials correct, and (3) 83–100% of trials correct. The tester also evaluated whether the dog had understood the task and was successfully making inferences based on exclusion. The dog was considered to have understood the task if at least 4 out of 6 trials were correct or the final 2–3 trials were correct.
Memory vs gesture
Previous studies have shown that dogs are more likely to choose an empty bowl out of two choices if a human points towards it, even when they have seen that the other bowl has food in it 34 , 35 , 36 , 37 . Similar to the gesture tests, this test aims to measure social cognition. The procedure for this test was also similar to the gesture test, but instead of gesturing towards the baited bowl, the tester gestured towards the empty bowl. The tester used the gesture which the dog had been most successful with—this was often the dynamic distal point. The bowls remained on the floor throughout the test, and the treat was placed in one of the bowls while the dog was watching. The test consisted of two trials, with the first trial always baited to the left and the second trial baited to the right. (See Supplementary Video S9 for the procedure.) Dogs were divided into two groups: those which chose the baited container on both trials (relied on their memory), and those which chose the empty container on 1–2 trials (relied on the human’s gesture).
The aim of this test was to measure the duration of the dogs’ short-term memory 38 . Three identical opaque bowls were placed upside down on the floor in a straight line, about 1 m apart from each other. A piece of food (or a toy) was placed under one of the bowls in each trial. The owner sat on a chair 3 m away from the middle bowl with the dog in front of her. During 7 training trials the dog learned to find a food reward from under one of the three bowls. Only a very short delay between hiding the food and releasing the dog was in place during this phase.
Once the dog had passed the training phase, the four test trials began. The tester placed a treat under one bowl while the dog was watching, after which a visual barrier was placed in front of the dog. The tester waited an increasing duration of time behind the owner and the dog (1st trial: 1 min, 2nd trial: 1.5 min, 3rd trial: 2 min, 4th trial: 2.5 min). After the waiting period, the tester removed the barrier, and the dog was released and allowed to make a choice. The order of baiting the bowls was the same for each dog: trial 1: middle, trial 2: left, trial 3: right, and trial 4: middle. (See Supplementary Video S11 for the procedure of the test phase.) Number of correct trials (out of a total of 4) was calculated for each dog. Since a very small number of dogs had a score of 0, these dogs were combined into a group with dogs that had a score of 1.
Data analysis
All statistical analyses were performed using IBM SPSS Statistics Version 28. An alpha level of 0.05 was used for all statistical tests. Multiple and logistic regression analyses were used with the enter method to analyse differences between breeds for each variable. For ordinal variables, cumulative odds ordinal logistic regression with proportional odds was used. In each model, we included age and sex of the dogs as control variables, since previous research suggests these may affect various measures of cognition and behaviour 39 , 40 , 41 , 42 . Each model therefore included the predictors breed, age, and sex (apart from success in the V-detour, which only included breed as a predictor). The Labrador Retriever, one of the most popular breeds worldwide, was used as the reference breed, since it had the largest number of individuals tested out of all included pedigree breeds.
For the greeting variable, the data failed the assumption of proportional odds according to the full likelihood ratio test, and therefore a multinomial logistic regression was conducted instead of ordinal logistic regression. For success in the V-detour task, Fisher’s Exact test using the Monte Carlo method was run with 10,000 simulations, since an insufficient number of individuals failed to solve the task for a binary logistic regression to be reliable. For latency (s) to solve the V-detour task, the dependent variable was log-transformed to normalize its distribution. Since the cylinder test variable ‘percentage of correct responses’ was negatively skewed, a reflect and square root transformation was applied (i.e., each data point was subtracted from the maximum value plus 1, and a square root transformation was then applied to these scores). Due to the transformation, the variable was inverted, and therefore named ‘percentage of incorrect responses’ to aid with interpretability. For all transformed variables, the original values are included in the figures, whereas the transformed variables are reported in the tables and text.
Ethical statement
We confirm that the procedures comply with national and EU legislation. Research was performed in accordance with the Declaration of Helsinki. The study was approved by the University of Helsinki Viikki Campus Research Ethics Committee (Statement 12/2021, accepted on 18/05/2021). Before participating in the cognitive test battery, each dog owner gave informed written consent for using their dogs’ test results in research. Reporting of results follows the recommendations of the ARRIVE guidelines. Informed consent was given by each subject for publication of identifying images/videos in an online-access publication.
A total of 934 dogs had results for the greeting test. Out of these, 12.8% were fearful when meeting the unknown person, 28.2% were indifferent, 41.1% responded in a friendly manner, and 11.1% of dogs had an overexcited greeting.
The multinomial regression model significantly predicted greeting scores over and above the intercept-only model (χ 2 (42) = 174.1, p < 0.001). The model explained 18.8% (Nagelkerke R 2 ) of the variance in greeting behaviour. Sex did not have a significant effect on the prediction of greeting (χ 2 (3) = 2.65, p = 0.45), but age did (χ 2 (3) = 20.77, p < 0.001). An increase in age was associated with a decrease in the odds of having an overexcited greeting compared to a friendly greeting (which was used as the reference group) (χ 2 (1) = 9.94, p = 0.002). Breed had a significant effect on the prediction of greeting score (χ 2 (36) = 152.86, p < 0.001). See Fig. 1 a for the proportions of greeting scores for each breed, and Supplementary Table S3 for parameter estimates for each greeting score.
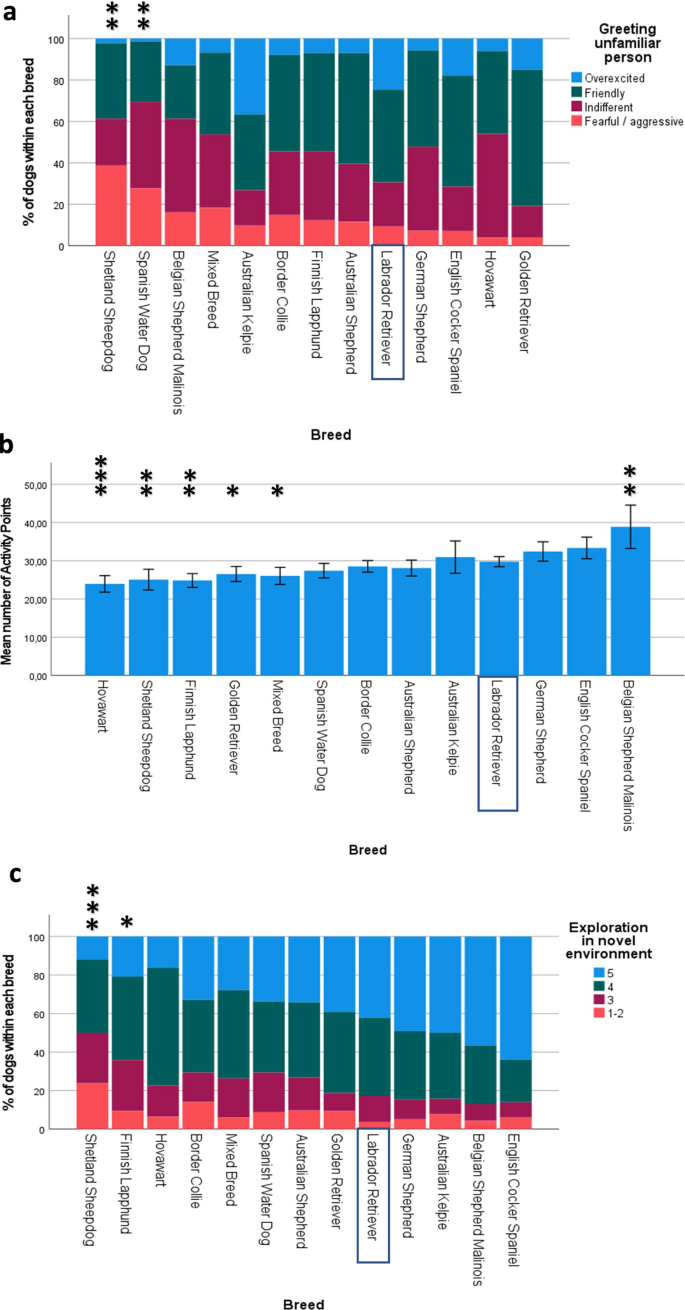
Breed differences of greeting unfamiliar person, activity level, and exploration in novel environment. Significant P-values (Bonferroni-corrected) are indicated with asterisks: ***p ≤ 0.001, **p ≤ 0.01, *p ≤ 0.05. The Labrador Retriever was used as the reference breed. ( a ) Percentage of dogs within each breed receiving each of the four greeting scores (n = 934). Breeds have been ordered based on odds ratios, from most fearful (highest odds ratio for score 1–3) on the left to least fearful (lowest odds ratio for score 1–3) on the right. P-values have been indicated for fearful response using asterisks. ( b ) Mean activity level scores (measured in FitBark activity points) for each breed (N = 759). Error bars represent 95% confidence intervals. Breeds are ordered based on B-values, with lowest activity levels on the left and highest activity levels on the right. ( c ) Percentage of dogs within each breed with each exploration score (N = 820). Breeds are ordered based on B-values, with lowest exploration on the left and highest exploration on the right. Score 1–2: low investigation (stays close to owner). Score 3: moderate investigation. Score 4: active investigation (walking). Score 5: very active investigation (running).
A total of 759 dogs had activity level results available. Dogs received a mean of 29.4 FitBark activity points, ranging from 9.9 to 93.4 activity points. The multiple regression model significantly predicted activity levels of dogs (F (14) = 7.91, p < 0.001, adj. R 2 = 0.12). There were 10 outliers but none of these were influential according to Cook’s Distance. Age and sex did not significantly predict activity level in dogs aged 1–8 years (age β = − 0.04, p = 0.23, sex β = − 0.03, p = 0.42). Six breeds differed significantly from the Labrador Retriever (which was used as the reference breed for all analyses). See Fig. 1 b for mean activity points for each breed, and Supplementary Table S4 for parameter estimates.
A total of 820 dogs had results for exploration in a novel environment. Out of these, 8.3% showed low investigation, 15.8% showed moderate investigation, 39.7% showed active investigation (walking), and 36.3% showed very active investigation (running).
The ordinal regression model significantly predicted exploration scores over and above the intercept-only model (χ 2 (14) = 61.98, p < 0.001). The model explained 8% (Nagelkerke R 2 ) of variance in exploratory behaviour. Age and sex did not have a significant effect on the prediction of exploration in dogs aged 1–8 years (age χ 2 (1) = 1.9, p = 0.17; sex χ 2 (1) = 0.05, p = 0.82), but breed did (χ 2 (12) = 57.68, p < 0.001). See Fig. 1 c for percentage of each exploration score for each breed, and Supplementary Table S5 for parameter estimates.
Cylinder test
A total of 992 dogs took part in the cylinder test. The median success rate of dogs was 80% (IQR = 30%). The multiple regression model significantly predicted the percentage of incorrect trials in the cylinder test (F (14, 961) = 6.96, p < 0.001, adj. R 2 = 0.08). No outliers were detected. Age was a significant predictor of performance in the cylinder test, β = 0.13, p < 0.001, with increasing age associated with an increasing percentage of incorrect trials (in dogs aged 1–8-years old). Sex was also a significant predictor of cylinder test performance, β = − 0.09, p = 0.004, with females making fewer mistakes than males. Five breeds had significantly lower percentages of incorrect trials compared to the Labrador Retriever. See Fig. 2 for the mean percentage of correct trials for each breed, and Supplementary Table S6 for parameter estimates.
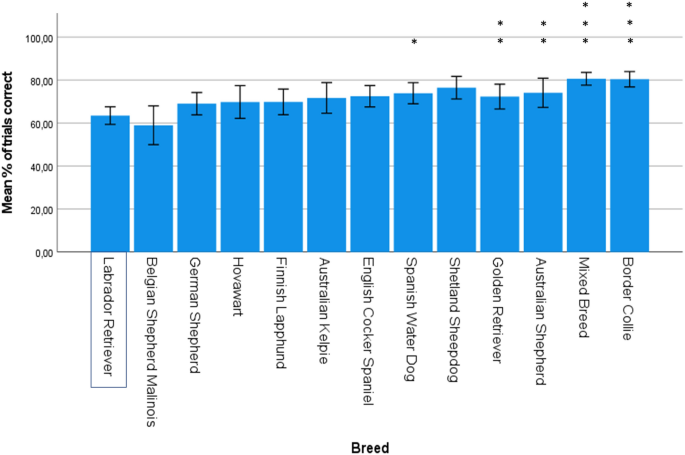
Mean percentage of correct trials for each breed in the cylinder test are presented, using the untransformed, original data (n = 992). Error bars represent 95% confidence intervals. Breeds are ordered based on B-values, with lowest success (low inhibitory control) on the left and highest success (high inhibitory control) on the right. The Labrador Retriever was used as the reference breed. Significant P-values (Bonferroni-corrected) are indicated with asterisks: ***p ≤ 0.001, **p ≤ 0.01, *p ≤ 0.05.
A total of 831 dogs took part in each of the five gesture tests. The mean percentage of correct trials for these dogs was 79% (SD = 12.9%). A two-tailed paired samples t-test showed that there was no significant change from the first 6 object choice trials to the last 6 trials, with a mean decrease of 0.84% in correct responses (95% CI [− 2.65, 0.96], t(831) = − 0.92, p = 0.36, d = -0.03). Therefore, it is unlikely that dogs were learning the pattern for baiting the bowls.
The multiple regression model significantly predicted the percentage of correct trials for the gesture tests (F (14, 804) = 3.41, p < 0.001, adj. R 2 = 0.04). There were two outliers, but these were not influential according to Cook’s Distance. Age and sex did not significantly predict performance in dogs aged 1–8 years (age β = 0.03, p = 0.45; sex β = − 0.06, p = 0.12). Three breeds had significantly lower scores than the Labrador Retriever. See Fig. 3 for the mean percentage of correct trials for each breed, and Supplementary Table S7 for parameter estimates.
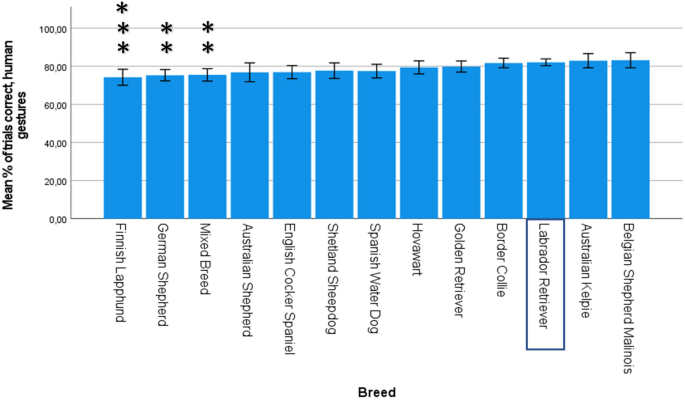
Mean percentage of correct trials in the gesture tests within each breed (n = 831). Error bars represent 95% confidence intervals. Breeds have been ordered based on B-values, with lowest success on the left and highest success on the right. The Labrador Retriever was used as the reference breed. Significant P-values (Bonferroni-corrected) are indicated with asterisks: ***p ≤ 0.001, **p ≤ 0.01, *p ≤ 0.05.
A total of 993 dogs took part in the V-detour test. Out of these, 88.5% successfully solved the V-detour within 3 min. There was no significant difference in the proportions of dogs within each breed succeeding compared to those failing (p = 0.13, 99% CI [0.12, 0.14]). See Fig. 4 a for the proportion of successful dogs in each breed.
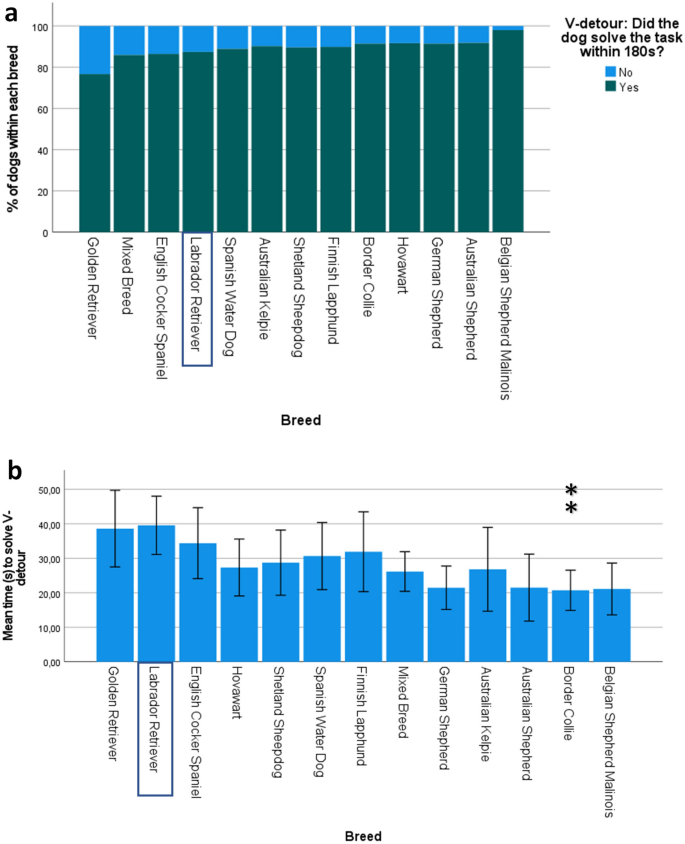
Performance of dogs during the V-detour task. The Labrador Retriever was used as the reference breed. Significant P-values (Bonferroni-corrected) are indicated with asterisks: ***p ≤ 0.001, **p ≤ 0.01, *p ≤ 0.05. ( a ) Percentage of dogs within each breed succeeding vs failing in the V-detour task (n = 993). Breeds have been ordered based on B-values, with lowest succeeding breeds on the left and highest succeeding breeds on the right. ( b ) Mean latency (s) to solve the V-detour for each breed are presented using the untransformed, original data (n = 863). Error bars represent 95% confidence intervals. Breeds are ordered based on B-values, with lowest succeeding breeds (took a long time to solve the task) on the left and highest succeeding breeds (solved the task quickly) on the right.
The median time for solving the V-detour task (when unsuccessful dogs were removed from the analysis) was 12 s (IQR = 29 s). The multiple regression model significantly predicted the time taken to solve the task (n = 863, F (14, 848) = 2.52, p = 0.002, adj. R 2 = 0.02). No outliers were detected. Age and sex did not significantly predict performance in the V-detour in dogs aged 1–8 years (age β = 0.05, p = 0.14; sex β = − 0.05, p = 0.12). The Border Collie had a significantly lower predicted mean log score compared to the Labrador Retriever (β = − 0.14, p = 0.01). See Fig. 4 b for mean time taken to solve the task for each breed, and Supplementary Table S8 for parameter estimates.
A total of 969 dogs took part in the unsolvable task. Only 57 (5.7%) of these were completely independent during the unsolvable task (i.e., they spent 0% of their time on human-directed behaviour). When comparing these dogs to those which spent over 0% of their time on human-directed behaviour, the binomial logistic regression model was statistically significant (χ 2 (14) = 34.28, p = 0.002). The model explained 9.7% (Nagelkerke R 2 ) of the variance. Age was not a significant predictor of independence in dogs aged 1–8 years (χ 2 (1) = 0.59, p = 0.44), but females had lower odds of being completely independent compared to males (χ 2 (1) = 5.22, p = 0.02). Breed was also a significant predictor of independence (χ 2 (12) = 23.13, p = 0.03), but no breed was significantly different from the Labrador Retriever. See Fig. 5 a for the percentage of completely independent dogs within each breed, and Supplementary Table S9 for parameter estimates.
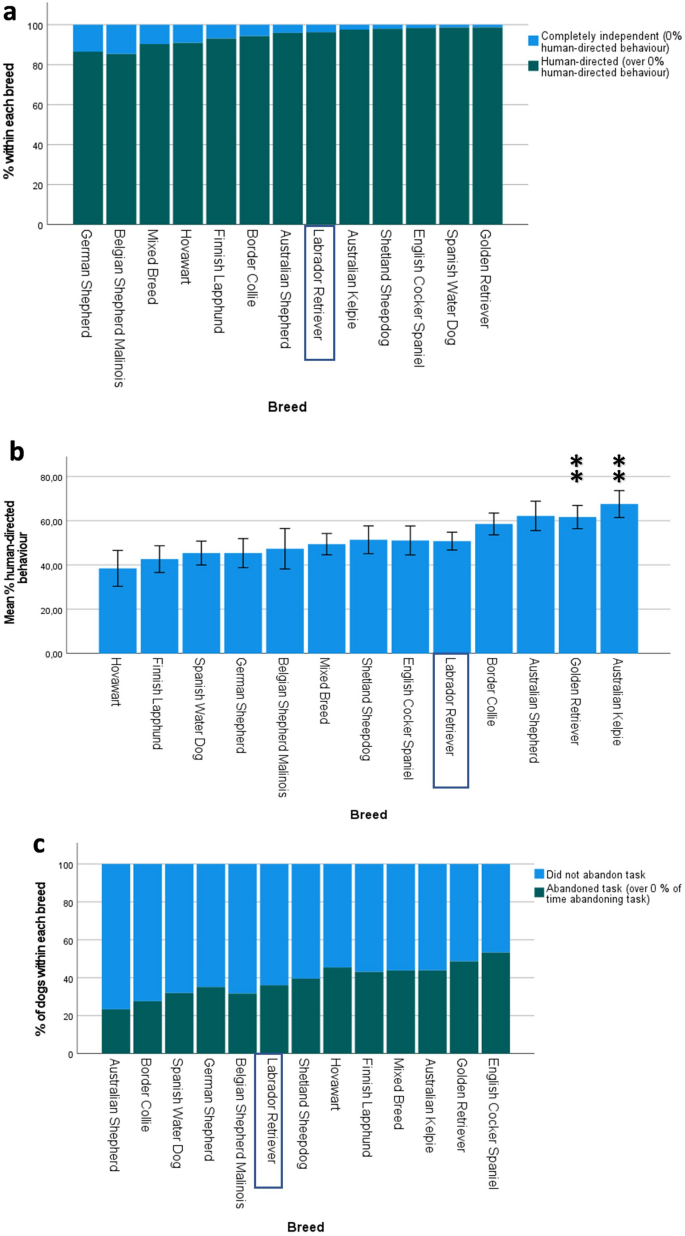
Performance of breeds in the unsolvable task (n = 969). The Labrador Retriever was used as the reference breed. Significant P-values (Bonferroni-corrected) are indicated with asterisks: ***p ≤ 0.001, **p ≤ 0.01, *p ≤ 0.05. ( a ) Percentage of dogs within each breed belonging to the human-directed compared to the completely independent groups during the Unsolvable Task. Breeds have been ordered based on odds ratios, with most independent breeds (most likely to spend 0% of their time on human-directed behaviour) on the left and least independent breeds (least likely to spend 0% of their time on human-directed behaviour) on the right. ( b ) Mean percentage of time (out of a total of 120 s) spent on human-directed behaviour during the unsolvable task for each breed. Error bars represent 95% confidence intervals. Breeds are ordered based on B-values, with lowest amount of time spent on human-directed behaviour on the left and highest amount of time on the right. ( c ) Percentage of dogs within each breed abandoning the task (over 0% of time spent on abandoning task) vs persisting (0% of time spent on abandoning task) with the unsolvable task. Breeds are ordered based on odds ratios, with the most persistent breeds (least likely to abandon task) on the left and the least persistent breeds (most likely to abandon task) on the right.
When completely independent dogs had been removed from the analysis, the median amount of time spent on human-directed behaviour was 54.2% (65 s out of a total of 120 s) (IQR = 40%). The multiple regression model significantly predicted the percentage of time spent on human-directed behaviour (n = 912, F (14, 885) = 5.7, p < 0.001, adj. R 2 = 0.07). No outliers were detected. Age significantly predicted time spent on human-directed behaviour (β = 0.09, p = 0.009), with older dogs spending a larger proportion of their time on human-directed behaviour. Sex did not significantly predict time spent on human-directed behaviour (β = 0.002, p = 0.95). The Golden Retriever and Australian Kelpie had significantly higher scores than the Labrador Retriever. See Fig. 5 b for the percentage of time spent on human-directed behaviour for each breed, and Supplementary Table S10 for parameter estimates.
A total of 37.2% of dogs spent over 0% of their time abandoning the task. When comparing these dogs to those which persisted with the task (0% of time spent on abandoning the task), the binomial logistic regression model was statistically significant (χ 2 (14) = 40.89, p < 0.001). The model explained 5.7% (Nagelkerke R 2 ) of the variance. Age was a significant predictor (χ 2 (1) = 16.34, p < 0.001), with older dogs being less likely to abandon the task. Breed was a significant predictor of abandoning the task, χ 2 (12) = 24.16, p = 0.02, but sex was not (χ 2 (1) = 0.32, p = 0.57). No breed significantly differed from the Labrador Retriever. See Fig. 5 c for the percentage of dogs within each breed abandoning the task, and Supplementary Table S11 for parameter estimates.
A total of 826 dogs took part in the logical reasoning task. Out of these, 33.4% were rated as having understood the logical reasoning task. When comparing dogs which understood the task to dogs which failed to understand the task, the binomial logistic regression model was not statistically significant (χ 2 (14) = 20.95, p = 0.1). See Supplementary Fig. S5 a for the percentage of dogs within each breed which understood the task.
For percentage of correct trials, 60.9% of dogs were correct on 0–50% of trials, 22.6% of dogs were correct on 51–82% of trials, and 16.5% of dogs were correct on 83–100% of trials. The ordinal regression model did not significantly predict success in the logical reasoning task above the intercept-only model, χ 2 (14) = 20.03, p = 0.13. See Supplementary Fig. S5 b for the percentage of dogs within each breed with different rates of success.
Gesture vs memory
A total of 823 dogs took part in the gesture vs memory test. Out of these, 40.5% followed the human gesture to the baited container in at least one trial. The binomial logistic regression model for trusting the human gesture was statistically significant (χ 2 (14) = 29.23, p = 0.01). The model explained 4.8% (Nagelkerke R 2 ) of the variance in the gesture vs memory test. Age and sex were not significant predictors in dogs aged 1–8 years, but breed was a significant predictor of trusting the gesture, χ 2 (12) = 25.42, p = 0.01. See Fig. 6 for percentage of dogs within each breed which trusted the human gesture vs their own memory, and Supplementary Table S12 for parameter estimates.
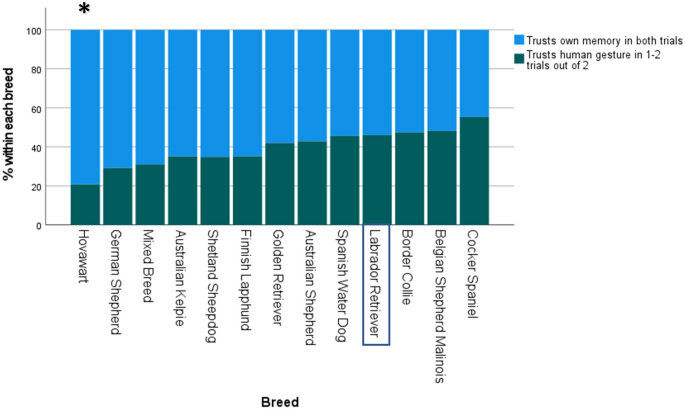
Percentage of dogs within each breed which trusted the human gesture compared to those which trusted their own memory (n = 823). Breeds have been ordered based on odds ratios, with breeds which are most likely to trust their own memory on the left and breeds which are most likely to trust the human’s gesture on the right. Significant P-values (Bonferroni-corrected) are indicated with asterisks: ***p ≤ 0.001, **p ≤ 0.01, *p ≤ 0.05. The Labrador Retriever was used as the reference breed.
A total of 822 dogs took part in the memory test. Out of these, 14.1% were correct on 0–1 trials, 30.4% were correct on two out of four trials, 35.3% were correct on three trials, and 20.2% were correct on all four trials. The ordinal logistic regression model did not significantly predict the number of correct trials in the spatial memory task above the intercept-only model (χ 2 (14) = 19.26, p = 0.16). See Supplementary Fig. S6 for the percentage of dogs within each breed with different success rates.
We found significant differences between individual dog breeds for five of the seven cognitive tests included in the test battery. Breed differences were found for measures of social cognition, persistence, inhibitory control, and spatial problem-solving ability. Differences were also evident for activity level, greeting of an unfamiliar person, and exploration of a novel environment. In contrast, no breed differences were found for short-term memory or logical reasoning.
Both inhibitory control and social cognition are likely to be especially important traits during artificial selection of dog breeds, both historically and in the present day. For example, inhibitory control may be a valued trait in herding dogs, which are required to inhibit their predatory responses. The Border Collie and Australian Shepherd were among the highest-scoring breeds in the cylinder test, indicating high inhibitory control. In contrast, the Malinois and German Shepherd were some of the lowest-scoring breeds. These breeds are often used in working roles requiring high responsiveness, which is often associated with low inhibitory control and high impulsivity 43 , 44 . Human-directed behaviour and socio-cognitive abilities may be highly valued in pet dogs and breeds required to work closely with people, such as herding dogs and retrievers 45 , 46 . In line with this, the Kelpie, Golden Retriever, Australian Shepherd, and Border Collie spent the largest proportion of their time on human-directed behaviour during the unsolvable task. In contrast, the ability to work independently may be important for various working dogs, such as detection dogs 47 , 48 . In our study, the two breeds which were most likely to be completely independent during the unsolvable task (spending 0% of their time on human-directed behaviour) were the German Shepherd and Malinois.
In conclusion, many of our results seem to reflect the breeds’ original or current function, but several breed differences could not be easily explained by breed function alone. For example, many of the breeds in our study belonged to the herding group, but there was often large variation in their results in several cognitive tests. The Finnish Lapphund received the lowest score in the gesture tests, whereas the Kelpie and Malinois had the highest scores for this test, despite all three being herding breeds. Similarly, during the unsolvable task, the Australian Shepherd was the least likely breed to abandon the task, whereas the Kelpie was among the breeds most likely to abandon the task. It seems evident that breeds can vary behaviourally from each other even within their breed groups, since different traits may have been (both intentionally and unintentionally) selected for in different breeds, despite the breed group they belong to.
Our results replicate the findings of various studies which have investigated differences between individual breeds in the V-detour task 19 , understanding of human gestures 7 , 8 , and the unsolvable task 8 , 18 . In contrast, most studies which have failed to find breed differences have compared breed groups 10 , 11 , 13 , 19 , 49 , 50 . It is possible that potential differences between breeds may have been missed in these studies, since (as shown by our current study) behavioural variation within breed groups can be substantial. For example, the Golden Retriever differed significantly from the Labrador Retriever in the unsolvable task and the gesture test, even though both belong to the retriever group. Our findings therefore highlight the importance of investigating behavioural differences between individual breeds rather than only relying on breed group categorizations.
We found no significant breed differences for logical reasoning or memory, and these results seem to be in line with previous studies 12 , 13 . However, it is important to note that even though we did not find differences between these specific breeds, this does not mean they do not exist between other breeds. For example, we did not include ancient breeds, hounds, or terriers in our analysis. Therefore, more research on individual breed differences on these cognitive traits is warranted.
It is important to note that the population used in this study is not representative of the entire dog population, since findings may differ across countries and cultures. In addition, since we used a commercial test battery, only certain types of owners and dogs were inevitably self-selected. The breeds included in our study were mostly breeds used in dog sports, and most owners were active in various dog sports or competitions. Even though this limits the generalization of our results, it is noteworthy that significant breed differences emerged despite the similarity of participant dogs and their training histories.
There is a possibility that the differences seen in our study were not based on genetic differences between breeds but rather due to variation in life experiences or training, since these have also been found to influence behaviour in cognitive tests 8 , 13 , 49 , 51 , 52 . Unfortunately, we were not able to control for the possible effects of training, environment, life experiences, or background of the dogs, since this information was not available to us. Therefore, the extent of their possible effects on breed differences in our study is not known; however, this is something we will investigate next. Previous studies suggest that environmental effects are unlikely to be the only explanation for breed differences. Genetic relatedness between dog breeds has been shown to account for a substantial portion of variation in understanding human gestures and inhibitory control 9 . In addition, when training history of participating dogs has been controlled for, significant breed differences have still been found in the unsolvable task 18 , 27 , 53 , problem-solving tasks 20 , 49 , inhibitory control 9 , and point-following ability 9 , 54 . However, this topic warrants further investigation to determine the extent of heritable differences between breeds as opposed to the effect of life experiences.
It could be argued that the large proportion of police dogs within the Malinois breed (45%) could have biased breed differences in our study. However, our sample also consisted of other working dogs as well as several pet dogs which participated extensively in training and competitions, but we did not have information about these individuals. Therefore, it would not have made much sense to exclude police dogs. We nevertheless analysed the cylinder test, V-detour, and unsolvable task (which were the only tests which police dogs took part in) with police dogs excluded. As a result, breed differences in the proportions of completely independent dogs in the unsolvable task became non-significant, and the proportion of completely independent Malinois dropped from 14.6 to 7.7%. For all other variables breed differences were still significant and the scores of Malinois changed only slightly (results not shown). Therefore, it seems that the inclusion of police dogs only affected complete independence during the unsolvable task but did not influence other results to a great extent.
Another factor which could affect breed differences is the brain size and skull shape of the breeds 13 , 54 , 55 , 56 . However, the breeds included in our study all had similar skull shapes, and there were no extremes in body size. The Shetland Sheepdog and Cocker Spaniel were the only breeds which markedly differed in size from the others, but they did not seem to consistently differ from the larger breeds in their results.
In conclusion, we found significant breed differences for various behavioural and cognitive traits in dogs. This is one of the few studies investigating individual breed differences in dogs, especially in non-social cognitive traits which are rarely studied in this context. Our results provide a more complete picture of breed-typical behaviour in dogs.
Data availability
The datasets analysed during the current study are not publicly available due to privacy restrictions but are available from the corresponding author on reasonable request.
Change history
29 january 2023.
The original online version of this Article was revised: In the original version of this Article the Supplementary Information file contained tracked changes, these have now been removed.
Croston, R., Branch, C. L., Kozlovsky, D. Y., Dukas, R. & Pravosudov, V. V. Heritability and the evolution of cognitive traits. Behav. Ecol. 26 , 1447–1459. https://doi.org/10.1093/beheco/arv088 (2015).
Article Google Scholar
Mehrkam, L. R. & Wynne, C. D. L. Behavioral differences among breeds of domestic dogs (Canis lupus familiaris): Current status of the science. Appl. Anim. Behav. Sci. 155 , 12–27. https://doi.org/10.1016/j.applanim.2014.03.005 (2014).
MacLean, E. L., Snyder-Mackler, N., vonHoldt, B. M. & Serpell, J. A. Highly heritable and functionally relevant breed differences in dog behaviour. Proc. Biol. Sci. 286 , 20190716. https://doi.org/10.1098/rspb.2019.0716 (2019).
Article CAS Google Scholar
Morrill, K. et al. Ancestry-inclusive dog genomics challenges popular breed stereotypes. Science 376 , eabk0639. https://doi.org/10.1126/science.abk0639 (2022).
Gacsi, M., Kara, E., Belenyi, B., Topal, J. & Miklosi, A. The effect of development and individual differences in pointing comprehension of dogs. Anim. Cogn. 12 , 471–479. https://doi.org/10.1007/s10071-008-0208-6 (2009).
Wobber, V., Hare, B., Koler-Matznick, J., Wrangham, R. & Tomasello, M. Breed differences in domestic dogs’ (Canis familiaris) comprehension of human communicative signals. Interact. Stud. Soc. Behav. Commun. Biol. Artif. Syst. 10 , 206–224. https://doi.org/10.1075/is.10.2.06wob (2009).
Udell, M. A. R., Ewald, M., Dorey, N. R. & Wynne, C. D. L. Exploring breed differences in dogs (Canis familiaris): does exaggeration or inhibition of predatory response predict performance on human-guided tasks?. Anim. Behav. 89 , 99–105. https://doi.org/10.1016/j.anbehav.2013.12.012 (2014).
Sundman, A.-S. et al. Understanding of human referential gestures is not correlated to human-directed social behaviour in Labrador retrievers and German shepherd dogs. Appl. Anim. Behav. Sci. 201 , 46–53. https://doi.org/10.1016/j.applanim.2017.12.017 (2018).
Gnanadesikan, G. E., Hare, B., Snyder-Mackler, N. & MacLean, E. L. Estimating the heritability of cognitive traits across dog breeds reveals highly heritable inhibitory control and communication factors. Anim. Cogn. 23 , 953–964. https://doi.org/10.1007/s10071-020-01400-4 (2020).
Riedel, J., Schumann, K., Kaminski, J., Call, J. & Tomasello, M. The early ontogeny of human–dog communication. Anim. Behav. 75 , 1003–1014. https://doi.org/10.1016/j.anbehav.2007.08.010 (2008).
Dorey, N. R., Udell, M. A. & Wynne, C. D. Breed differences in dogs sensitivity to human points: A meta-analysis. Behav. Processes 81 , 409–415. https://doi.org/10.1016/j.beproc.2009.03.011 (2009).
Szabo, D., Mills, D. S., Range, F., Viranyi, Z. & Miklosi, A. Is a local sample internationally representative? Reproducibility of four cognitive tests in family dogs across testing sites and breeds. Anim. Cogn. 20 , 1019–1033. https://doi.org/10.1007/s10071-017-1133-3 (2017).
Horschler, D. J. et al. Absolute brain size predicts dog breed differences in executive function. Anim. Cogn. 22 , 187–198. https://doi.org/10.1007/s10071-018-01234-1 (2019).
Clarke, T., Mills, D. & Cooper, J. Exploring the utility of traditional breed group classification as an explanation of problem-solving behavior of the domestic dog (Canis familiaris). J. Vet. Behav. 33 , 103–107. https://doi.org/10.1016/j.jveb.2019.06.004 (2019).
Bradshaw, J., Goodwin, D., Lea, A. & Whitehead, S. A survey of the behavioural characteristics of pure-bred dogs in the United Kingdom. Vet. Rec. 138 , 465–468 (1996).
Turcsán, B., Kubinyi, E. & Miklósi, Á. Trainability and boldness traits differ between dog breed clusters based on conventional breed categories and genetic relatedness. Appl. Anim. Behav. Sci. 132 , 61–70. https://doi.org/10.1016/j.applanim.2011.03.006 (2011).
Svartberg, K. Breed-typical behaviour in dogs—Historical remnants or recent constructs?. Appl. Anim. Behav. Sci. 96 , 293–313. https://doi.org/10.1016/j.applanim.2005.06.014 (2006).
Maglieri, V., Prato-Previde, E., Tommasi, E. & Palagi, E. Wolf-like or dog-like? A comparison of gazing behaviour across three dog breeds tested in their familiar environments. R Soc. Open Sci. 6 , 190946. https://doi.org/10.1098/rsos.190946 (2019).
Pongrácz, P., Miklósi, Á., Vida, V. & Csányi, V. The pet dogs ability for learning from a human demonstrator in a detour task is independent from the breed and age. Appl. Anim. Behav. Sci. 90 , 309–323. https://doi.org/10.1016/j.applanim.2004.08.004 (2005).
Shimabukuro, C., Putrino, N., Helbling, J., Tognetti, S. & Bentosela, M. Individual differences in social and non-social behaviors in domestic dogs (Canis familiaris) during the acquisition, extinction and reacquisition of a problem solving task. Behav. Processes 113 , 179–186. https://doi.org/10.1016/j.beproc.2015.02.002 (2015).
Pongrácz, P., Rieger, G. & Vékony, K. Grumpy dogs are smart learners—the association between dog-owner relationship and dogs’ performance in a social learning task. Animals 11 , 961 (2021).
Overall, K. L., Dunham, A. E., Scheifele, P. & Sonstrom Malowski, K. Fear of noises affects canine problem solving behavior and locomotion in standardized cognitive tests. Appl. Anim. Behav. Sci. 221 . https://doi.org/10.1016/j.applanim.2019.104863 (2019).
Passalacqua, C., Marshall-Pescini, S., Merola, I., Palestrini, C. & Previde, E. P. Different problem-solving strategies in dogs diagnosed with anxiety-related disorders and control dogs in an unsolvable task paradigm. Appl. Anim. Behav. Sci. 147 , 139–148. https://doi.org/10.1016/j.applanim.2013.05.003 (2013).
Bray, E. E., Sammel, M. D., Seyfarth, R. M., Serpell, J. A. & Cheney, D. L. Temperament and problem solving in a population of adolescent guide dogs. Anim. Cogn. 20 , 923–939. https://doi.org/10.1007/s10071-017-1112-8 (2017).
Tiira, K. smartDOG , http://smartdog.fi/english (2016).
Lazarowski, L., Krichbaum, S., Waggoner, L. P. & Katz, J. S. The development of problem-solving abilities in a population of candidate detection dogs (Canis familiaris). Anim. Cogn. 23 , 755–768. https://doi.org/10.1007/s10071-020-01387-y (2020).
Passalacqua, C. et al. Human-directed gazing behaviour in puppies and adult dogs, Canis lupus familiaris. Anim. Behav. 82 , 1043–1050. https://doi.org/10.1016/j.anbehav.2011.07.039 (2011).
Chapagain, D., Range, F., Huber, L. & Viranyi, Z. Cognitive aging in dogs. Gerontology 64 , 165–171. https://doi.org/10.1159/000481621 (2018).
Kabadayi, C., Bobrowicz, K. & Osvath, M. The detour paradigm in animal cognition. Anim. Cogn. 21 , 21–35 (2018).
Elgier, A. M., Jakovcevic, A., Mustaca, A. E. & Bentosela, M. Pointing following in dogs: Are simple or complex cognitive mechanisms involved?. Anim. Cogn. 15 , 1111–1119 (2012).
Kaminski, J. & Nitzschner, M. Do dogs get the point? A review of dog–human communication ability. Learn. Motiv. 44 , 294–302 (2013).
Mendes, J. W. W., Resende, B. & Savalli, C. A review of the unsolvable task in dog communication and cognition: comparing different methodologies. Anim. Cogn. 24 , 907–922 (2021).
Erdőhegyi, Á., Topál, J., Virányi, Z. & Miklósi, Á. Dog-logic: Inferential reasoning in a two-way choice task and its restricted use. Anim. Behav. 74 , 725–737. https://doi.org/10.1016/j.anbehav.2007.03.004 (2007).
Szetei, V., Miklósi, Á., Topál, J. & Csányi, V. When dogs seem to lose their nose: An investigation on the use of visual and olfactory cues in communicative context between dog and owner. Appl. Anim. Behav. Sci. 83 , 141–152. https://doi.org/10.1016/s0168-1591(03)00114-x (2003).
Petter, M., Musolino, E., Roberts, W. A. & Cole, M. Can dogs (Canis familiaris) detect human deception?. Behav. Processes 82 , 109–118. https://doi.org/10.1016/j.beproc.2009.07.002 (2009).
Dwyer, C. & Cole, M. R. Domesticated dogs (Canis familiaris) tend to follow repeated deceptive human cues even when food is visible. Learn Behav. 46 , 442–448. https://doi.org/10.3758/s13420-018-0356-8 (2018).
Bhattacharjee, D. & Bhadra, A. Adjustment in the point-following behaviour of free-ranging dogs—roles of social petting and informative-deceptive nature of cues. Anim. Cogn. https://doi.org/10.1007/s10071-021-01573-6 (2021).
Fiset, S., Beaulieu, C. & Landry, F. Duration of dogs’ (Canis familiaris) working memory in search for disappearing objects. Anim. Cogn. 6 , 1–10. https://doi.org/10.1007/s10071-002-0157-4 (2003).
Hori, Y., Kishi, H., Inoue-Murayama, M. & Fujita, K. Dopamine receptor D4 gene (DRD4) is associated with gazing toward humans in domestic dogs ( Canis familiaris ). Open J. Anim. Sci. 03 , 54–58. https://doi.org/10.4236/ojas.2013.31008 (2013).
Persson, M. E., Roth, L. S., Johnsson, M., Wright, D. & Jensen, P. Human-directed social behaviour in dogs shows significant heritability. Genes Brain Behav. 14 , 337–344. https://doi.org/10.1111/gbb.12194 (2015).
Scandurra, A., Alterisio, A., Di Cosmo, A. & D'Aniello, B. Behavioral and perceptual differences between sexes in dogs: An overview. Animals (Basel) 8 . https://doi.org/10.3390/ani8090151 (2018).
Watowich, M. M. et al. Age influences domestic dog cognitive performance independent of average breed lifespan. Anim. Cogn. 23 , 795–805. https://doi.org/10.1007/s10071-020-01385-0 (2020).
Brady, K., Cracknell, N., Zulch, H. & Mills, D. S. Factors associated with long-term success in working police dogs. Appl. Anim. Behav. Sci. 207 , 67–72. https://doi.org/10.1016/j.applanim.2018.07.003 (2018).
Piotti, P., Satchell, L. P. & Lockhart, T. S. Impulsivity and behaviour problems in dogs: A reinforcement sensitivity theory perspective. Behav. Proc. 151 , 104–110 (2018).
Van Poucke, E., Hoglin, A., Jensen, P. & Roth, L. S. V. Breed group differences in the unsolvable problem task: herding dogs prefer their owner, while solitary hunting dogs seek stranger proximity. Anim. Cogn. https://doi.org/10.1007/s10071-021-01582-5 (2021).
Bognar, Z., Szabo, D., Dees, A. & Kubinyi, E. Shorter headed dogs, visually cooperative breeds, younger and playful dogs form eye contact faster with an unfamiliar human. Sci. Rep. 11 , 9293. https://doi.org/10.1038/s41598-021-88702-w (2021).
Article ADS CAS Google Scholar
Lazarowski, L. et al. Comparing pet and detection dogs (Canis familiaris) on two aspects of social cognition. Learn. Behav. 48 , 432–443. https://doi.org/10.3758/s13420-020-00431-8 (2020).
Lazarowski, L., Rogers, B., Waggoner, L. P. & Katz, J. S. When the nose knows: ontogenetic changes in detection dogs’ (Canis familiaris) responsiveness to social and olfactory cues. Anim. Behav. 153 , 61–68. https://doi.org/10.1016/j.anbehav.2019.05.002 (2019).
Marshall-Pescini, S., Frazzi, C. & Valsecchi, P. The effect of training and breed group on problem-solving behaviours in dogs. Anim. Cogn. 19 , 571–579. https://doi.org/10.1007/s10071-016-0960-y (2016).
Hirschi, A., Mazzini, A. & Riemer, S. Disentangling help-seeking and giving up: differential human-directed gazing by dogs in a modified unsolvable task paradigm. Anim. Cogn. https://doi.org/10.1007/s10071-021-01595-0 (2022).
McKinley, J. & Sambrook, T. D. Use of human-given cues by domestic dogs (Canis familiaris) and horses (Equus caballus). Anim. Cogn. 3 , 13–22 (2000).
Hoglin, A. et al. Long-term stress in dogs is related to the human-dog relationship and personality traits. Sci. Rep. 11 , 8612. https://doi.org/10.1038/s41598-021-88201-y (2021).
Konno, A., Romero, T., Inoue-Murayama, M., Saito, A. & Hasegawa, T. Dog breed differences in visual communication with humans. PLOS ONE 11 , e0164760. https://doi.org/10.1371/journal.pone.0164760 (2016).
Gacsi, M., McGreevy, P., Kara, E. & Miklosi, A. Effects of selection for cooperation and attention in dogs. Behav. Brain Funct. 5 , 31. https://doi.org/10.1186/1744-9081-5-31 (2009).
Helton, W. S. & Helton, N. D. Physical size matters in the domestic dog’s (Canis lupus familiaris) ability to use human pointing cues. Behav. Processes 85 , 77–79. https://doi.org/10.1016/j.beproc.2010.05.008 (2010).
Hecht, E. E. et al. Significant neuroanatomical variation among domestic dog breeds. J. Neurosci. 39 , 7748–7758. https://doi.org/10.1523/JNEUROSCI.0303-19.2019 (2019).
FitBark , https://fitbark.com .
Download references
Acknowledgements
We thank all the owners and their dogs who participated in this research, as well as the smartDOG licence testers: Katja Kontu, Liisa Tikka, Sanni Somppi, Kati Vierimaa, and Ulla-Marja Ruistola. We would also like to thank the University of Helsinki and the Finnish Foundation of Veterinary Research for funding this study. Open access funded by the Helsinki University Library.
Author information
Authors and affiliations.
Department of Production Animal Medicine, University of Helsinki, 00014, Helsinki, Finland
Saara Junttila & Anna Valros
International Partnership for Dogs, Helsinki, Finland
Katariina Mäki
Digital Solutions, Lapland University of Applied Sciences, Jokiväylä 11C, 96300, Rovaniemi, Finland
Heli Väätäjä
Department of Finnish and Finno-Ugric Languages, University of Turku, 20014, Turku, Finland
Elisa Reunanen
smartDOG Ltd, 05800, Hyvinkää, Finland
Katriina Tiira
Department of Equine and Small Animal Medicine, University of Helsinki, 00014, Helsinki, Finland
You can also search for this author in PubMed Google Scholar
Contributions
K.T., H.V., and E.R. collected the data. A.V., K.M., and K.T. supervised the project. S.J. designed and performed the analysis. S.J. wrote the manuscript. All authors discussed and reviewed the manuscript and contributed to the final manuscript.
Corresponding author
Correspondence to Saara Junttila .
Ethics declarations
Competing interests.
KT is the founder and owner of smartDOG Ltd, and HV and ER are licence testers of the company. KM, SJ, and AV have no affiliation with smartDOG. SJ’s work has been funded by the the Finnish Foundation of Veterinary Research. There are no other conflicts of interest.
Additional information
Publisher's note.
Springer Nature remains neutral with regard to jurisdictional claims in published maps and institutional affiliations.
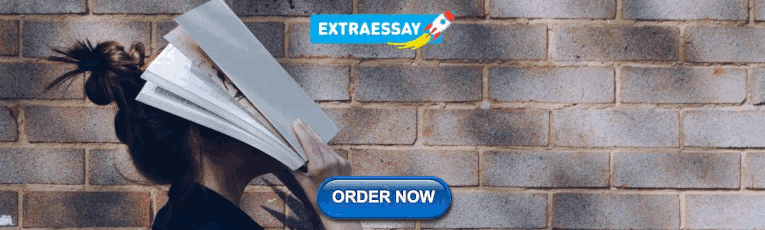
Supplementary Information
Supplementary Video 1.
Supplementary Video 2.
Supplementary Video 3.
Supplementary Video 4.
Supplementary Video 5.
Supplementary Video 6.
Supplementary Video 7.
Supplementary Video 8.
Supplementary Video 9.
Supplementary Video 10.
Supplementary Video 11.
Supplementary Information 1.
Rights and permissions.
Open Access This article is licensed under a Creative Commons Attribution 4.0 International License, which permits use, sharing, adaptation, distribution and reproduction in any medium or format, as long as you give appropriate credit to the original author(s) and the source, provide a link to the Creative Commons licence, and indicate if changes were made. The images or other third party material in this article are included in the article's Creative Commons licence, unless indicated otherwise in a credit line to the material. If material is not included in the article's Creative Commons licence and your intended use is not permitted by statutory regulation or exceeds the permitted use, you will need to obtain permission directly from the copyright holder. To view a copy of this licence, visit http://creativecommons.org/licenses/by/4.0/ .
Reprints and permissions
About this article
Cite this article.
Junttila, S., Valros, A., Mäki, K. et al. Breed differences in social cognition, inhibitory control, and spatial problem-solving ability in the domestic dog ( Canis familiaris ). Sci Rep 12 , 22529 (2022). https://doi.org/10.1038/s41598-022-26991-5
Download citation
Received : 20 September 2022
Accepted : 22 December 2022
Published : 29 December 2022
DOI : https://doi.org/10.1038/s41598-022-26991-5
Share this article
Anyone you share the following link with will be able to read this content:
Sorry, a shareable link is not currently available for this article.
Provided by the Springer Nature SharedIt content-sharing initiative
This article is cited by
Modulation of dog–owner relationship and dog social and cognitive behavior by owner temperament and dog breed group.
- Miiamaaria V. Kujala
- Noora Imponen
- Noona Kiuru
Scientific Reports (2023)
Comparative neurogenetics of dog behavior complements efforts towards human neuropsychiatric genetics
- Kathleen Morrill
- Frances Chen
- Elinor Karlsson
Human Genetics (2023)
By submitting a comment you agree to abide by our Terms and Community Guidelines . If you find something abusive or that does not comply with our terms or guidelines please flag it as inappropriate.
Quick links
- Explore articles by subject
- Guide to authors
- Editorial policies
Sign up for the Nature Briefing newsletter — what matters in science, free to your inbox daily.

Advertisement
Animal Creativity as a Function of Behavioral Innovation and Behavior Flexibility in Problem-solving Situations
- Regular Article
- Published: 17 March 2021
- Volume 56 , pages 218–233, ( 2022 )
Cite this article
- Luiz Henrique Santana ORCID: orcid.org/0000-0001-9931-9422 1 &
- Miriam Garcia-Mijares 1
1060 Accesses
5 Citations
Explore all metrics
A natural approach of animal creativity through insightful problem-solving may offer a panel of how physiological, contextual, cultural and developmental variables related to each other to produce new behaviors. The spontaneous interconnection of acquire behaviors is an Insightful Problem-Solving model based on the new combination and/or chaining of behaviors that were previously and independently trained. This model seems to offer an integrative alternative for the studies of Innovation and Behavioral Flexibility because it allows the research on innovation in a scenario in which the response that solves the problem situation is not available by trial-and-error. Measuring task-appropriateness by behavior flexibility and novelty by behavior innovation under insightful problem-solving paradigm can contribute for the integration of decades of evidence in Cognitive Psychology, Neuro-ethology, Behavior Analysis and Behavioral Neurosciences. The Insightful Problem-Solving allows the independent test of behavioral innovation and behavioral flexibility as it measures the behavioral innovation inside insightful test and tests if the BF depends on variables arranged in the problem-situation and/or on the previous training (e.g. familiarity with access to appetitive stimulus in the pre-test, the number of distinct behaviors trained, and contingency changes in the post-test).
This is a preview of subscription content, log in via an institution to check access.
Access this article
Subscribe and save.
- Get 10 units per month
- Download Article/Chapter or eBook
- 1 Unit = 1 Article or 1 Chapter
- Cancel anytime
Price excludes VAT (USA) Tax calculation will be finalised during checkout.
Instant access to the full article PDF.
Rent this article via DeepDyve
Institutional subscriptions
Similar content being viewed by others
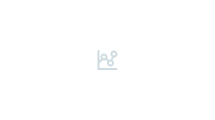
Creating Creativity: A Behavior Analytic Approach
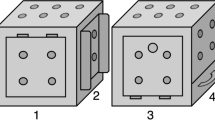
Innovative problem-solving in wild hyenas is reliable across time and contexts
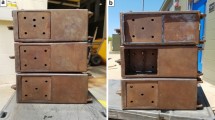
Persistence is key: investigating innovative problem solving by Asian elephants using a novel multi-access box
Arnold, M. A., & Christopher Newland, M. (2018). Variable behavior and repeated learning in two mouse strains: Developmental and genetic contributions. Behavioural Processes , 157, 509–518
Audet, J., & Lefebvre, L. (2017). What’s flexible in behavioral flexibility. Behavioral Ecology, 28 (4), 943–947. https://doi.org/10.1093/beheco/arx007 .
Article Google Scholar
Bandini, E., & Harrison, R. A. (2020). Innovation in chimpanzees. Biological Reviews, 95 (5), 1167–1197. https://doi.org/10.1111/brv.12604 .
Article PubMed Google Scholar
Barrett, L. P., Stanton, L., & Benson-Amram, S. (2018). The Cognition of ‘nuisance’ species. Animal Behavior , Special Issue Cognitive Ecology, 1–11. https://doi.org/10.1016/j.anbehav.2018.05.005 .
Barsalou, L. W. (2008). Grounded cognition. Annual Review in Psychology, 59, 617–645. https://doi.org/10.1146/annurev-psych-113011-143840
Beghetto, R. A. (2007a). Does creativity have a place in classroom discussions? prospective teachers’ response preferences. Thinking Skills and Creativity, 2 (1), 1–9. https://doi.org/10.1016/j.tsc.2006.09.002 .
Beghetto, R. A. (2007b). Ideational code-switching: Walking the talk about supporting student creativity in the classroom. Roeper Review: A Journal on Gifted Education, 29 (4), 265–270. https://doi.org/10.1080/02783190709554421 .
Beghetto, R. A. (2013). Killing ideas softly? The promise and perils of creativity in the classroom . Charlotte: IAP Information Age Publishing.
Google Scholar
Beghetto, R. A. (2014a). Creative mortification: An initial exploration. Psychology of Aesthetics, Creativity, and the Arts, 8 (3), 266–276. https://doi.org/10.1037/a0036618 .
Beghetto, R. A. (2014b). Creativity: Development and enhancement. In J. A. Plucker & C. M. Callahan (Eds.), Critical issues and practices in gifted education: What the research says (2nd ed., pp. 183–196). Waco: Prufrock Press.
Beghetto, R. A., & Kaufman, J. C. (2007a). The genesis of creative greatness: Mini-c and the expert performance approach. High Ability Studies, 18 (1), 59–61. https://doi.org/10.1080/13598130701350668 .
Beghetto, R. A., & Kaufman, J. C. (2007b). Toward a broader conception of creativity: A case for ‘mini-c’ creativity. Psychology of Aesthetics, Creativity, and the Arts, 1 (2), 73–79. https://doi.org/10.1037/1931-3896.1.2.73 .
Birch, H. G. (1945). The relation of previous experience to insightful problem-solving. Journal of Comparative Psychology, 38 (6), 367–383.
PubMed Google Scholar
Boden, M. (2004). The creative mind: Myths and mechanisms (2nd ed.). New York: Routledge.
Brady, A. M., & Floresco, S. B. (2015). operant procedures for assessing behavioral flexibility in rats. Journal of Visual Experimentation, 96 . https://doi.org/10.3791/52387 .
Brubaker, L., Dasgupta, S., Bhattacharjee, D., Bhadra, A., & Udell, A. R. (2017). Differences in problem-solving between canid populations: Do domestication and lifetime experience affect persistence. Animal Cognition, 20 (4), 717–723. https://doi.org/10.1007/s10071-017-1093-7 .
Buitenweg, J., van de Ven, R. M., Prinssen, S., Murre, J., & Ridderinkhof, K. R. (2017). Cognitive flexibility training: a large-scale multimodal adaptive active-control intervention study in healthy older adults. Frontiers in Human Neuroscience, 11, 529. https://doi.org/10.3389/fnhum.2017.00529
Article PubMed PubMed Central Google Scholar
Byrne, R. M. J., & Murray, M. A. (2005). Attention and working memory in insight problem-solving. Proceedings of the Annual Meeting of the Cognitive Science Society, 27, 1571–1575.
Canestrari, C., Branchini, E., Bianchi, I., Savardi, U., & Burro, R. (2018). Pleasures of the mind: what makes jokes and insight problems enjoyable. Frontiers in Psychology, 8, 2297. https://doi.org/10.3389/fpsyg.2017.02297
Catani, A. C., Ono, K., Souza, D. G. (2000). Sources of Novel Behavior: Stimulus Control Arranged for Different Response Dimensions. European Journal of Behavior Analysis, 1 (1), 23–32. https://doi.org/10.1080/15021149.2000.11434153 .
Cook, R. G., & Fowler, C. (2014). “Insight” in pigeons: absence of means–end processing in displacement tests. Animal Cognition , 17(2), 207–220
Cristofori, I., Salvi, C., Beeman, M., & Grafman, J. (2018). The effects of expected reward on creative problem-solving. Cognitive, Affective, & Behavioral Neuroscience . https://doi.org/10.3758/s13415-018-0613-5
Davidson, J. E., & Sternberg, R. J. (2003). The psychology of problem solving . New York: Cambridge University Press.
Delage, P. E. G. A. (2011). Transferência de aprendizagem no uso de ferramentas por Macacos-Prego (Cebus apella). (Tese de Doutorado). Universidade Federal do Pará . Pará: Belém.
Delage, P. E. G. A., & Carvalho-Neto, M. B. (2010). Um modo alternativo de construir um operant: a aprendizagem recombinativa. Psicologia em Pesquisa, 4 (1), 50–56.
Dicezare, R. H. (2017). Recombinação de comportamentos em ratos Wistar ( Rattusnorvegicus ) em um novo procedimento de deslocamento de caixa . Dissertação de Mestrado defendida junto ao Programa de Pós-Graduação em Psicologia Experimental da Universidade de São Paulo.
Dicezare, R. H., & Garcia-Mijares, M. (2019) Recombination of behaviors in Wistar rats (Rattus norvegicus) in a new box displacement procedure. Manuscript submitted for publication.
Dietrich, A. (2018). Types of creativity. Psychonomic Bulletin & Review . https://doi.org/10.3758/s13423-018-1517-7
Dostál, J. (2015). Theory of problem solving. Procedi: Social and Behavioral Sciences, 174, 2798–2805.
Ellis, J., Glaholt, M. G., & Reingold, E. M. (2011). Eye movements reveal solution knowledge prior to insight. Consciousness and Cognition, 20 (3), 768–776. https://doi.org/10.1016/j.concog.2010.12.007 .
Epstein, R. (1985). The spontaneous interconnection of three behaviors. The Psychological Record, 35 , 131–141.
Epstein, R. (1987). The spontaneous interconnection of four behaviors of behavior in a pigeon ( Columba livia ). Journal of Comparative Psychology, 101 (2), 197–201.
Epstein, R. (1991). Skinner, creativity, and the problem of spontaneous behavior. Psychological Science . https://doi.org/10.1111/j.1467-9280.1991.tb00168.x .
Epstein, R. (2014). On the orderliness of behavioral variability: Insights from generativity theory. Journal of Contextual Behavioral Science . https://doi.org/10.1016/j.jcbs.2014.08.004 .
Epstein, R. (2015). On the rediscovery of the principle of resurgence. Mexican Journal of Behavior Analysis , 41 .
Epstein, R., Kirshnit, C. E., Lanza, R. P., & Rubin, L. C. (1984). ‘Insight’ in the Pigeon: antecedents and determinants of an intelligent performance. Nature, 308 , 61–62.
Epstein, R., & Medalie, S. D. (1983). The Spontaneous Use of a Tool by a Pigeon. Behavior Analysis Letters, 3 , 241–247.
Felden, A., Paris, C. I., Chapple, D. G., Haywood, J., Suarez, A. V., Tsutsui, N. D., Lester, P. J., Gruber, M. A. M. (2018). Behavioral variation and plasticity along an invasive ant introduction pathway. Journal of Animal Ecology . https://doi.org/10.1111/1365-2656.12886 .
Ferster, C. B., & Skinner, B. F. (1997). Schedules of Reinforcement . Cambridge: B. F. Skinner Foundation. First Published at 1957.
Fleck, J. I. (2008). Working memory demands in insight versus analytic problem solving. European Journal of Cognitive Psychology, 20 (1), 139–176.
Fleck, J. I., & Weisberg, R. W. (2013). Insight versus analysis: Evidence for Diverse Methods in Problem-Solving. Journal of Cognitive Psychology, 25 (4), 436–463.
Foerder, P., Galloway, M., Barthel, T., Moore, D. E., & Reiss, D. (2011). Insightful Problem Solving in an Asian Elephant. PLoS ONE, 6 (8), e23251. https://doi.org/10.1371/journal.pone.0023251 .
Gilhooly, K. J. (2016). Incubation and Intuition in Creative Problem Solving. Frontiers in Psychology, 7 , 1076.
PubMed PubMed Central Google Scholar
Glaveanu, V. P. (2011). Creativity as cultural participation. Journal for the Theory of Social Behavior, 41 (1), 48–67. https://doi.org/10.1111/j.1468-5914.2010.00445.x .
Glaveanu, V. P. (2013). Rewriting the language of creativity: The five A’s framework. Review of General Psychology, 17 (1), 69–81. https://doi.org/10.1037/a0029528 .
Griffin, A. S. (2016). Innovativeness as an emergent property: a new alignment of comparative and experimental research on animal innovation. Phil. Trans. R. Soc. B, 371. https://doi.org/10.1098/rstb.2015.0544 .
Griffin, A. S., & Guez, D. (2014). Innovation and Problem Solving: A review of common mechanisms. Behavioral Processes, 109 , 121–134.
Griffin, A. S., Guez, D., Lermite, F., & Patience, M. (2013). Tracking Changing Environments: Innovators Are Fast, but Not Flexible Learners. PLoS ONE, 8(12). https://doi.org/10.1371/journal.pone.0084907 .
Guilford, J. P. (1950). Creativity. American Psychologist, 5 , 444–454.
Heinrich, B. (1995). An Experimental Investigation of Insight in Common Ravens (Corvuscorax). The Auk, 112 (4), 994–1003.
Helfand, M., Kaufman, J. C., & Beghetto, R. A. (2017). The Four C Model of Creativity: Culture and context. In V. P. Glăveanu (Ed.), Palgrave handbook of creativity and culture research (pp. 15–360). New York: Palgrave.
Hennessey, B. A., & Amabalie, T. M. (2010). Creativity. Annual Review of Psychology, 61 , 569–598. https://doi.org/10.1146/annurev.psych.093008.100416 .
Holekamp, K. E., & Benson-Amram, S. (2017). The evolution of intelligence in mammalian carnivores. Interface Focus, 7. https://doi.org/10.1098/rsfs.2016.0108 .
Huebner, F., & Fichtel, C. (2015). Innovation and behavioral flexibility in wild redfronted lemurs (Eulemurrufifrons). Animal Cognition, 18 (3), 777–787. https://doi.org/10.1007/s10071-015-0844-6 .
Kaufman, J. C., & Beghetto, R. A. (2009). Beyond big and little: the four c model of creativity. Review of General Psychology, 13 (1), 1–12. https://doi.org/10.1037/a0013688 .
Kaufman, J. C., & Beghetto, R. A. (2013). Do people recognize the Four Cs? Examining layperson conceptions of creativity. Psychology of Aesthetics, Creativity, and the Arts, 7 , 229–236.
Kaufman, J. C. (2015). Creativity is life: a commentary on the special issue. The Journal of Creative Behavior , 49(3), 233–237. https://doi.org/10.1002/jocb.128 .
Keane, M. (1989). Modelling problem solving in Gestalt “insight” problems. Irish Journal of Psychology, 10 , 201–215.
Kizilirmak, J. M., Thuerich, H., Folta-Schoofs, K., Schott, B. H., & Richardson-Klavehn, A. (2016). Neural Correlates of Learning from Induced Insight: A Case for Reward-Based Episodic Encoding. Frontiers in Psychology, 1 (7), 1693.
Knoblich, G., Ohlsson, S., & Raney, G. E. (2001). An eye movement study of insight problem solving. Memory & Cognition, 29 (7), 1000–1009. https://doi.org/10.3758/BF03195762 .
Köhler, W. (1952). The Mentality of Apes . London: Routledge & Kegan Paul. First Published in 1917.
Köhler, W. (1959). Gestalt Psychology Today. American Psychologist, 14 , 727–734.
Koops, K. (2020). Chimpanzee termite fishing etiquette. Nature Human Behaviour, 4 (9), 887–888. https://doi.org/10.1038/s41562-020-0895-9 .
Kounios, J., & Beeman, M. (2009). The Aha! Moment: the Cognitive Neuroscience. Current Directions in Psychological Science, 18 (4), 210–216.
Kounios, J., & Beeman, M. (2014). The Cognitive Neuroscience of Insight. The Annual Review of Psychology, 65 , 71–93.
Kubina, R. M., Morrison, R. S., Lee, D. L. (2006). Behavior Analytic Contributions to the Study of Creativity. Journal of Creative Behavior, 40 (4), 223–242. https://doi.org/10.1002/j.2162-6057.2006.tb01275.x .
Lassig, C. J. (2013). Approaches to creativity: How adolescents engage in the creative process. Thinking Skills and Creativity, 10 , 3–12. https://doi.org/10.1016/j.tsc.2013.05.002 .
Lattal, K. A. (1995). Contingency and Behavior Analysis. Mexican Journal of Behavior Analysis, 21 , 47–73.
Lea, S. E. G., Chow, P. K. Y., Leaver, L. A., & McLaren, I. P. L. (2020). Behavioral flexibility: A review, a model, and some exploratory tests. Learning & Behavior . https://doi.org/10.3758/s13420-020-00421-w .
Lee, C. S., Therriault, D. J., & Linderholm, T. (2012). On the cognitive benefits of cultural experience: Exploring the relationship between studying abroad and creative thinking. Applied Cognitive Psychology, 26 (5), 768–778. https://doi.org/10.1002/acp.2857 .
Lefebvre, L. (2013). Brains, innovations, tools and cultural transmission in birds, non-human primates, and fossil hominins. Frontiers in Human Neuroscience, 7 , 245. https://doi.org/10.3389/fnhum.2013.00245 .
Leung, A. K., & Chiu, C. (2010). Multicultural experience, idea receptiveness, and creativity. Journal of Cross-Cultural Psychology, 41 (5–6), 723–741. https://doi.org/10.1177/0022022110361707 .
Leung, A. K., Maddux, W. W., Galinsky, A. D., & Chiu, C. (2008). Multicultural experience enhances creativity: The when and how. American Psychologist, 63 (3), 169–181. https://doi.org/10.1037/0003-066X.63.3.169 .
Lind, J., Lönnberg, S., Persson, T., & Enquist, M. (2017). Time Does Not Help Orangutans Pongo abelii Solve Physical Problems. Frontiers in Psychology, 8 , 161–167. https://doi.org/10.3389/fpsyg.2017.00161 .
Logan, C. J. (2016a). Behavioral flexibility and problem solving in an invasive bird. PeerJ, 4 , e1975. https://doi.org/10.7717/peerj.1975 .
Logan, C. J. (2016b). How far will a behaviorallyflexible invasive bird go to innovate? Royal Society Open Science, 3. https://doi.org/10.1098/rsos.160247 .
Logan, C. J. (2016c). Behavioral flexibility in an invasive bird is independent of other behaviors. PeerJ, 12 (4), e2215. https://doi.org/10.7717/peerj.2215 .
Logan, C. J., Jelbert, S. A., Breen, A. J., Gray, R. D., & Taylor, A. H. (2014). Modifications to the Aesop’s Fable Paradigm Change New Caledonian Crow Performances. PLoS ONE, 9(7). https://doi.org/10.1371/journal.pone.0103049 .
Lu, J. G., Akinola, M., & Mason, M. F. (2017). “Switching on” creativity: Task switching can increase creativity by reducing cognitive fixation. Organizational Behavior and Human Decision Processes, 139 , 63–75.
Maier, N. R. F. (1940). The behavioral mechanisms concerned with problem solving. Psychological Review, 47 , 43–58.
Mednick, S. A. (1962). The Associative Basis of the Creative Process. Psychology Review, 69 , 220–232. https://doi.org/10.1037/h0048850 .
Morgan, D. L. (2010). Schedules of Reinforcement at 50: A Retrospective Appreciation. The Psychological Record, 60 , 151–158.
Neves-Filho, H., Dicezare, R. H. F., Martins-Filho, A. M., & Garcia-Mijares, M. (2016b). Efeitos de treinos sucessivo e concomitante sobre a recombinação de repertórios de cavar e escalar em Rattus norvegicus. Perspectivas em Análise do Comportamento, 7(2), 243–255.
Neves-Filho, H. B. (2010). Efeitos de Diferentes Histórias de Treino sobre a Ocorrência de “Insight” em Macacos-Prego (Cebus spp.) . Belém: Dissertação de Mestrado. Programa de Pós-Graduação em Teoria e Pesquisa do Comportamento.
Neves-Filho, H. B., Carvalho-Neto, M. B., Barros, R. S., & Costa, J. R. (2014). Insight em Macacos-Prego com diferentes contextos de treinos de habilidades pré-requisito. Interação em Psicologia, 18 (3), 333–350.
Neves-Filho, H. B., Carvalho-Neto, M. B., Taybelbaum, G. P. T., Malheiros, R. S., & Knaus, Y. C. (2016a). Effects of different training histories upon manufacturing a tool to solve a problem: insight in capuchin apes (Sapajus spp.). Animal Cognition, 1. 10.1007/s10071-016-1022-1.
Neves-Filho, H. B., Stella, L. D., Dicezare, R. H., & Garcia-Mijares, M. (2015). Insight in the white rat: spontaneous interconnection of two behaviors in Rattus norvegicus . European Journal of Behavior Analysis, 16(2). https://doi.org/10.1080/15021149.2015.1083283 .
Orita, R., & Hattori, M. (2018). Positive and negative affects facilitate insight problem-solving in different ways: a study with implicit hints. Japanese Psychological Research , 61(2), 94–106
Öllinger, M., & Müller, A. (2017). Search and Coherence-Building in Intuition and Insight Problem Solving. Frontiers in Psychology, 8. https://doi.org/10.3389/fpsyg.2017.00827 .
Pepperberg, I. M. (2015). Creativity and Innovation in the Grey Parrot (Psittacus erithacus). In A. B. Kaufman & J. C. Kaufman (Eds.), Animal Creativity and Innovation (pp. 3–25). London: Elsevier Academic Press.
Preiszner, B., Papp, S., Pipoly, I., Seress, G., Vincze, E., Liker, A., & Bókony, V. (2016). Problem-solving performance and reproductive success of great tits in urban and forest habitats. Animal Cognition, 20 (1), 53–63. https://doi.org/10.1007/s10071-016-1008-z .
Quinn, J. L., Cole, E. F., Reed, T. E., & Morand-Ferron, J. (2016). Environmental and genetic determinants of innovativeness in a natural population of birds. Philosophical Transactions Royal Society B, 371. https://doi.org/10.1098/rstb.2015.0184 .
Ramsey, G., Bastian, M. L., & Schaik, C. (2007). Animal Innovation defined and operationalized. Behavioral and Brain Sciences, 30 , 393–437.
Reader, S. M., Morand-Ferron, J., & Flynn, E. (2016). Animal and human innovation: novel problems and novel solutions. Philosophical Transactions of the Royal Society B: Biological Sciences , 371(1690), 20150182. https://doi.org/10.1098/rstb.2015.0182 .
Renner, E., Abramo, A. M., Hambright, K., & Phillips, K. A. (2017). Insightful problem solving and emulation in brown capuchin apes. Animal Cognition . https://doi.org/10.1007/s10071-017-1080-z .
Ruprecht, C. M., Taylor, C. D., Wolf, J. E., & Leising, K. J. (2014). Task complexity modifies the search strategy of rats. Behavioral and Brain Research, 258 , 208–217.
Rymer, T. L., Pillay, N., & Schradin, C. (2013). Extinction or Survival? Behavioral Flexibility in Response to Environmental Change in the African Striped Mouse Rhabdomys. Sustainability, 5 , 163–186.
Sandkühler, S., & Bhattacharya, J. (2008). Deconstructing Insight: EEG Correlates of Insightful Problem Solving. PLoS ONE, 3 (1), e1459. https://doi.org/10.1371/journal.pone.0001459 .
Santos, D. G. (2017). Efeitos da Topografia da Resposta sobre a Resolução de Problemas do Tipo Insight em Ratos . Belém: Dissertação de Mestrado. Programa de Pós-Graduação em Teoria e Pesquisa do Comportamento.
Schooler, J. W., Ohlsson, S., & Brooks, K. (1993). Thoughts beyond words: When language overshadows insight. Journal of Experimental Psychology: General, 122 , 166–183.
Schunn, C. D., & Dunbar, K. (1996). Priming, analogy, and awareness in complex reasoning. Memory & Cognition, 24 (3), 271–284.
Shettleworth, S. J. (2012). Do Animals Have Insight, and What Is Insight Anyway? Canadian Journal of Experimental Psychology, 66 (4), 217–226.
Sio, U. N., & Ormerod, T. C. (2009). Does incubation enhance problem solving? A meta-analytic review. Psychological Bulletin, 135 (1), 94–120.
Skinner, B. F. (1938). The Behavior of organisms: An experimental analysis . New York: Apple Century-Crofts.
Skinner, B. F. (1969). Contingencies of Reinforcement: A Theoretical Analysis . Upper Saddle River: Prentice Hall.
Skinner, B. F. (1984). An operant analysis of problem solving. Behavioral and Brain Sciences, 7 (4), 583–591. https://doi.org/10.1017/S0140525X00027412 .
Sterelny, K. (2016). Adaptable individuals and innovative lineages. Phil. Trans. R. Soc. B, 371. https://doi.org/10.1098/rstb.2015.0196 .
Sternberg, R. J., & Lubart, T. I. (1996). Investing in creativity. American Psychologist , 51(7), 677–688
Strickland, B. R. (1989). Internal-External Control Expectancies: From Contingency to Creativity. American Psychologist, 44 , 1–12.
Taylor, A. H., Medina, F. S., Holzhaider, J. C., Hearne, L. J., Hunt, G. R., Gray, R. D., & Allen, C. (2010). An Investigation into the Cognition Behind Spontaneous String Pulling in New Caledonian Crows. PLoS ONE , 5(2), e9345
Thorndike, E. L. (1898). Animal intelligence: An experimental study of the associative processes in animals. The Psychological Review: Monograph Supplements , 2(4), i–109. https://doi.org/10.1037/h0092987 .
Thorpe, W. H. (1956). Learning and instinct in animals . London.: Methuen.
Timberlake, W. (2003). Is the Operant Contingency Enough for a Science. Behavior and Philosophy, 31 , 1–33.
Vartanian, O. (2009). Variable attention facilitates creative problem solving. Psychology of Aesthetics, Creativity, and the Arts, 3 (1), 57–59.
Webb, M. E., Little, D. R., & Cropper, S. J. (2016). Insight is not in the problem: investigating insight in problem solving across task types. Frontiers in Psychology , 7
Wild, S., Hoppitt, W. J. E., Allen, S. J., & Krutzen, M. (2020). Integrating Genetic, Environmental, and Social Networks to Reveal Transmission Pathways of a Dolphin Foraging Innovation. Current Biology, 30 (15), 3024–3030. https://doi.org/10.1016/j.cub.2020.05.069 .
Wong, B. B. M., & Candolin, U. (2015). Behavioral responses to changing. Behavioral Ecology (2015), 26 (3), 665–673. https://doi.org/10.1093/beheco/aru183 .
Wood, W., & Rünger, D. (2016). Psychology of Habit. Annual Review of Psychology, 67 , 1–26.
Wright, T. F., Eberhard, J. R., Hobson, E. A., Avery, M. L., & Russello, M. A. (2010). Behavioral flexibility and species invasions: the adaptive flexibility hypothesis. Ethology, Ecology & Evolution, 22 , 394–404.
Zandberg, L., Quinn, J. L., Naguib, M., & Oers, K. (2017). Personality-dependent differences in problem-solving performance in a social context reflect foraging strategies. Behavioral Processes, 134 , 95–102.
Download references
This research was partially funded by the National Council for Scientific and Technological Develoment (CNPq-Brazil, number 142215/2016-3), and Coordination for the Improvement of Personal of Higher Education (CAPES-Brazil, 88887.469044/2019-00).
Author information
Authors and affiliations.
Institute of Psychology, University of São Paulo, Avenida Professor Mello de Moraes, 1721. Programa de Pós-Graduação em Psicologia Experimental, Instituto de Psicologia, Bloco A, sala A-6, 05508-030, São Paulo, Brazil
Luiz Henrique Santana & Miriam Garcia-Mijares
You can also search for this author in PubMed Google Scholar
Corresponding author
Correspondence to Luiz Henrique Santana .
Ethics declarations
Disclosure of potential conflicts of interest.
There are no conflicts of interest.
Research Involving Human Participants and/or animals
Not applicable.
Informed Consent
Conflict of interest.
The first author declares that he has no conflict of interest. The second author declares that she has no conflict of interest.
Additional information
Publisher’s note.
Springer Nature remains neutral with regard to jurisdictional claims in published maps and institutional affiliations.
Rights and permissions
Reprints and permissions
About this article
Santana, L.H., Garcia-Mijares, M. Animal Creativity as a Function of Behavioral Innovation and Behavior Flexibility in Problem-solving Situations. Integr. psych. behav. 56 , 218–233 (2022). https://doi.org/10.1007/s12124-020-09586-5
Download citation
Accepted : 26 October 2020
Published : 17 March 2021
Issue Date : March 2022
DOI : https://doi.org/10.1007/s12124-020-09586-5
Share this article
Anyone you share the following link with will be able to read this content:
Sorry, a shareable link is not currently available for this article.
Provided by the Springer Nature SharedIt content-sharing initiative
- Behavior innovation
- Behavioral flexibility
- Problem-solving
- Find a journal
- Publish with us
- Track your research
- The Open University
- Accessibility hub
- Guest user / Sign out
- Study with The Open University
My OpenLearn Profile
Personalise your OpenLearn profile, save your favourite content and get recognition for your learning
About this free course
Become an ou student, download this course, share this free course.

Start this free course now. Just create an account and sign in. Enrol and complete the course for a free statement of participation or digital badge if available.
5.1 Animal problem-solving: using tools
From the earliest, most primitive stick or piece of rock, to the most sophisticated supercomputer or jet aircraft of modern times, humans have been using tools to solve problems since prehistoric times.
Given the advantages of using tools, it is perhaps surprising that it's not more common for animals to use them. There are examples of tool use by other species: some otters use stones to break open shellfish; some monkeys do the same to break open nuts; and some chimpanzees ‘fish’ for termites with sticks (Emery and Clayton, 2009). But it appears to be a general pattern that all humans use tools and most other species do not. Is this because animal minds do not have the capability to use tools? Tool use does, after all, involve a number of aspects of executive function, including: working out what a tool can be used for; planning how to use it; and remembering what the tool has managed to do (and failed to do) before.
While other species may not have the same degree of neocortical development and executive function as humans, are they able to use tools to solve problems to some extent?
There is evidence that the nearest evolutionary neighbours of humans, the other great apes (gorillas, chimpanzees, bonobos and orangutans), are able to solve problems using tools. A typical laboratory experiment involves putting food into an apparatus where the animal cannot reach it using their bodies alone, e.g. if testing chimpanzees, the apparatus will prevent the chimpanzees from reaching the food with their fingers. Tools, such as sticks of varying lengths or shapes, are left near the apparatus that will, if used correctly, allow the animal to access the food. Visalberghi and colleagues (1995) showed that a variety of primate species could solve such problems, but great apes were better than other primates (monkeys) at selecting the best tools, and adapting tools to the needs of the task.
But possibly the best non-human tool users are, perhaps surprisingly, to be found in species without a neocortex: birds. Emery and Clayton (2009) and Seed and Byrne (2010) give examples of a number of bird species with impressive tool-using and problem-solving abilities, including crows, jays and finches. One of the star species, though, is the New Zealand kea (Figure 8).
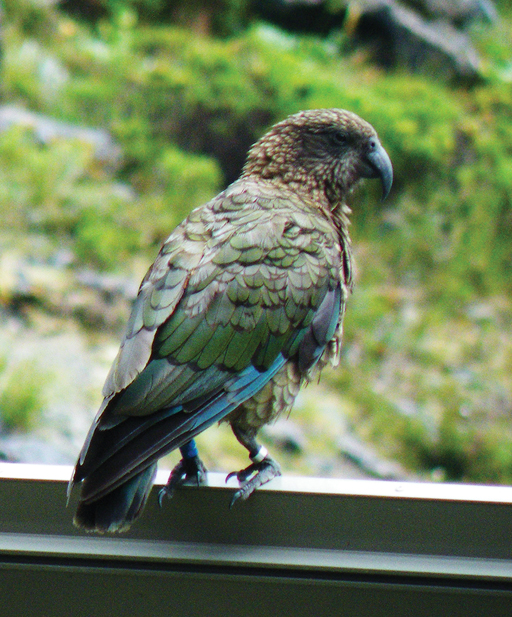
This is a photograph of a kea − a type of parrot from New Zealand that has impressive problem-solving abilities. The kea has green and blue plumage and is perched on a window frame.
Keas have been shown to solve a fairly simple problem (where food is obtained by hauling up a string) on the first attempt − suggesting they had mentally worked out the solution before starting the task, rather than by trial and error (Werdenich and Huber, 2006). They have also been shown to solve ‘second-order’ tool-use tasks, where one tool must be used to acquire or adapt another, in order to then complete the task (Auersperg et al., 2010), and there is evidence that they can learn from observing other keas performing a problem-solving task (Huber et al., 2001). As well as being able to solve problems as individuals, keas have been shown to collaborate to solve problems too (Tebbich et al., 1996).
Problem-solving ability: a link between cognition and conservation?
- Ridley, Amanda R.
- Speechley, Elizabeth M.
Traditionally, conservation and cognition have been disparate research disciplines. However, Audet et al.'s recent research contributes to an increasing body of evidence that innovative behaviours may determine the ability of species to respond to rapid environmental change, identifying an opportunity for cognition research to directly contribute to conservation outcomes.
- animal cognition;
- problem-solving;
- innovation;
- conservation

Animals With Larger Brains Are the Best Problem Solvers
Why did some species, such as humans and dolphins, evolve large brains relative to the size of their bodies? Why did others, such as blue whales and hippos, evolve to have brains that, compared to their bodies, are relatively puny?
It has long been thought that species with brains that are large relative to their body are more intelligent. Despite decades of research, the idea that relative brain size predicts cognitive abilities remains highly controversial, because there is still little experimental evidence to support it. However, a paper released today describes a massive experiment that supports the theory.
Sarah Benson-Amram, an assistant professor in the Department of Zoology and Physiology at the University of Wyoming, is the lead author on a new paper, titled “Brain size predicts problem-solving ability in mammalian carnivores.” It shows that carnivore species with larger brains relative to their body size are better at solving a novel problem-solving task. The paper appears in the Proceedings of the National Academy of Sciences , one of the world’s most prestigious scientific journals.
Other authors of the study include Kay Holekamp, a University Distinguished Professor at Michigan State University; Ben Dantzer, an assistant professor at the University of Michigan; Eli Swanson, a postdoctoral researcher at the University of Minnesota; and Greg Stricker, also from Michigan State University.
The authors traveled around the country to nine different zoos and presented 140 animals from 39 different mammalian carnivore species with a novel problem-solving task. The study included polar bears, arctic foxes, tigers, river otters, wolves, spotted hyenas and some rare, exotic species such as binturongs, snow leopards and wolverines. Each animal was given 30 minutes to try to extract food from a closed metal box. To access the food, an animal had to slide a bolt latch, which would allow a door to open. The box was baited with the favorite food of the study animal, so red pandas received bamboo and snow leopards got steak.
The main result is that species with larger brains relative to their body size were more successful than species with relatively smaller brains.
“This study offers a rare look at problem solving in carnivores, and the results provide important support for the claim that brain size reflects an animal’s problem-solving abilities — and enhance our understanding of why larger brains evolved in some species,” Benson-Amram says.
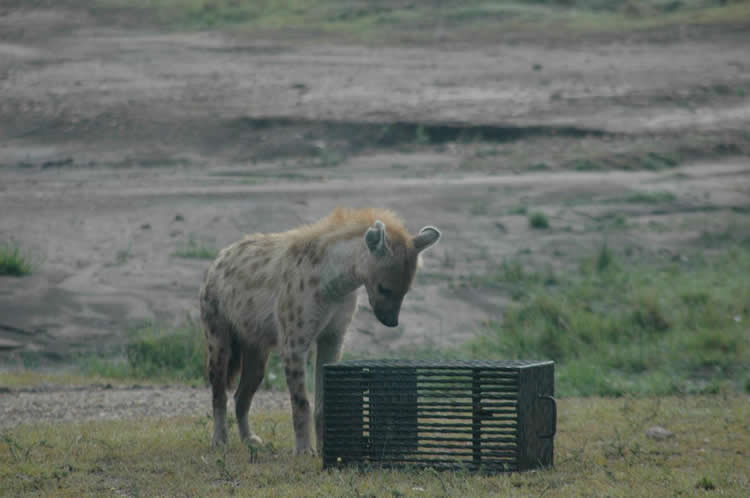
Dantzer explains that, “Overall, 35 percent of animals (49 individuals from 23 species) were successful in solving the problem. The bears were the most successful, solving the problem almost 70 percent of the time. Meerkats and mongooses were the least successful, with no individuals from their species solving the problem.”
Interestingly, larger animals were less successful overall than smaller-bodied animals. The paper also reports that manual dexterity did not affect problem-solving success.
In addition to examining the influence of brain size on problem-solving abilities, the authors also asked whether species that live in larger average group sizes are more successful problem solvers.
Holekamp explains, “A hypothesis that has garnered much support in primate studies is ‘the social brain hypothesis,’ which proposes that larger brains evolved to deal with challenges in the social domain. This hypothesis posits that intelligence evolved to enable animals to anticipate, respond to and, perhaps, even manipulate the actions of others in their social groups. If the social brain hypothesis is correct, then we would expect that species that live in larger social groups would be more intelligent. However, we did not find any support for the social brain hypothesis in this study. There was no indication that social group size influenced problem-solving abilities.”
Funding: Funding provided by National Science Foundation.
Source: Sarah Benson-Amram – University of Wyoming Image Credit: The image is credited to Sarah Benson-Amram Video Source: Video credited to Sarah Benson-Amram Original Research: Abstract for “Brain size predicts problem-solving ability in mammalian carnivores” by Sarah Benson-Amram, Ben Dantzer, Gregory Stricker, Eli M. Swanson, and Kay E. Holekamp in PNAS . Published online January 25 2016 doi:10.1073/pnas.1505913113
Brain size predicts problem-solving ability in mammalian carnivores
Despite considerable interest in the forces shaping the relationship between brain size and cognitive abilities, it remains controversial whether larger-brained animals are, indeed, better problem-solvers. Recently, several comparative studies have revealed correlations between brain size and traits thought to require advanced cognitive abilities, such as innovation, behavioral flexibility, invasion success, and self-control. However, the general assumption that animals with larger brains have superior cognitive abilities has been heavily criticized, primarily because of the lack of experimental support for it. Here, we designed an experiment to inquire whether specific neuroanatomical or socioecological measures predict success at solving a novel technical problem among species in the mammalian order Carnivora. We presented puzzle boxes, baited with food and scaled to accommodate body size, to members of 39 carnivore species from nine families housed in multiple North American zoos. We found that species with larger brains relative to their body mass were more successful at opening the boxes. In a subset of species, we also used virtual brain endocasts to measure volumes of four gross brain regions and show that some of these regions improve model prediction of success at opening the boxes when included with total brain size and body mass. Socioecological variables, including measures of social complexity and manual dexterity, failed to predict success at opening the boxes. Our results, thus, fail to support the social brain hypothesis but provide important empirical support for the relationship between relative brain size and the ability to solve this novel technical problem.
“Brain size predicts problem-solving ability in mammalian carnivores” by Sarah Benson-Amram, Ben Dantzer, Gregory Stricker, Eli M. Swanson, and Kay E. Holekamp in PNAS . Published online January 25 2016 doi:10.1073/pnas.1505913113
I did an animal psychology module in my undergraduate course but we didn’t focus on the physiological size of the brain itself. This is really interesting and it’s great to see some research supporting the theory that large brain relative to size = greater intelligence.
I wonder if the results would be different had there been a counterbalancing, or some measure of equating, done between the size – weight of the problem box, friction of the sliding latch/lever/rod and the size / ultra-structural form of the organisms involved. And considering different species specific behaviors pre-designed to search and capture food, the repertoire of solution behaviors would be easier or constrained as function of the behavioral phenotype per se and not the brain size. The Breland and Breland paper, titled “Misbehavior of Organisms” that was so widely sited during the “cognitive revolution” is relevant to this problem.
..now I know why adults sometimes told me: leave it to the horse-he has bigger head!.. carnivore or not. Researchers also missed opportunity to speed up solution timing by stimulation, i.e. having the food on spring loaded moving tray to give some urgency..
Comments are closed.

Key Neurons for Maternal Bonding Identified
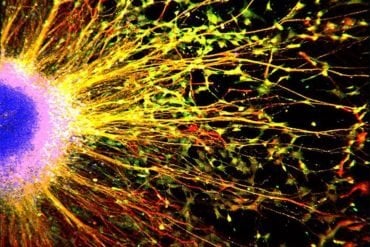
Cancer Drug Shows Promise for Autism Cognitive Function
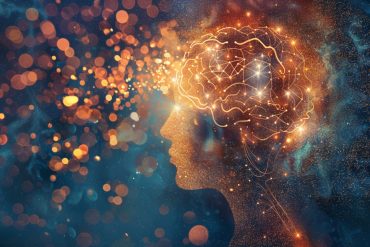
“Eureka Moments” Shed Light on Consciousness
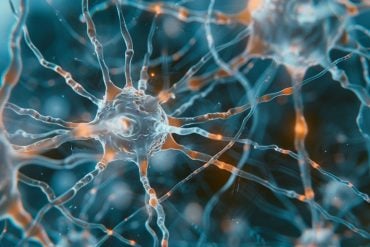
Neural Vulnerabilities and Resilience in Alzheimer’s Explored
September 1, 2018
11 min read
2 Mental Abilities Separate Humans from Animals
Two key features created the human mind
By Thomas Suddendorf
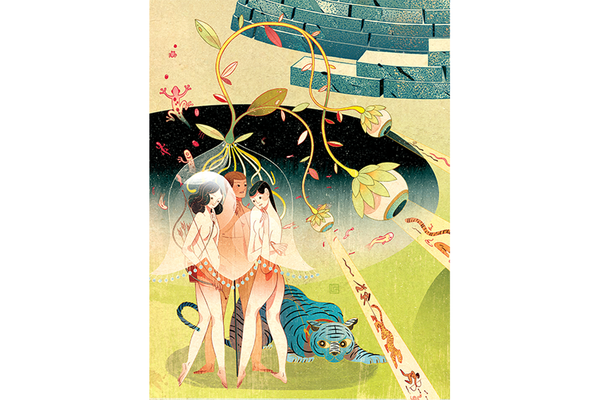
Why are we, and not the gorillas, running the zoos?
Other primates live inconspicuously in dwindling habitats, but humans have expanded and changed our surroundings to an astounding degree. Our dominance is obviously not the result of our physical ability; other animals are stronger and faster and have more acute senses. It is because of our mental abilities. Yet determining the cognitive traits that make us so special has turned out to be a devilishly complicated question to answer—one made more confusing by the frequent arrival of new studies that seem to show that animals from birds to chimpanzees can match many human cognitive skills.
Last year, to name just one example, a study published in Science boldly claimed that ravens can plan for the future just like humans do. Five birds learned to pick a stone and drop it into a box to get a reward. Subsequently, these ravens picked the rock from among distracting items minutes or even hours before the box was available to them. The researchers concluded from this achievement, along with a similar task in which the birds could exchange bottle tops for rewards, that the ravens were “thinking ahead” in flexible ways, an ability that is a key to human brainpower.
On supporting science journalism
If you're enjoying this article, consider supporting our award-winning journalism by subscribing . By purchasing a subscription you are helping to ensure the future of impactful stories about the discoveries and ideas shaping our world today.
Yet the achievements of the ravens, as well as cognitive feats of apes in other studies, can be explained in simpler ways. It also turns out that animal and human cognition, though similar in many respects, differ in two profound dimensions. One is the ability to form nested scenarios, an inner theater of the mind that allows us to envision and mentally manipulate many possible situations and anticipate different outcomes. The second is our drive to exchange our thoughts with others. Taken together, the emergence of these two characteristics transformed the human mind and set us on a world-changing path.
Bird Brains
Let us begin by taking a harder look at that raven experiment. Even before the tests started, the birds had learned, over several trials, to recognize that the target item, the stone, led to rewards and that distractor items did not. So it is not really surprising that when the actual trials began, the ravens selected what had already been reinforced.
This is a good reason why scientists, before they jump to conclusions about “rich” animal capacities, need to carefully rule out more straightforward, or “lean,” alternative explanations. They also need to conduct independent replications. In my laboratory, we have tried to do this by conducting studies with children that carefully limit the possibility of mistaking behavior actually driven by lean mechanisms for the products of rich cognition. We used single trials with novel tasks on our subjects to avoid giving them the learning opportunities that occur through repeated exposure. We also changed up the timing and spatial contexts of the tests to avoid cueing the children about the solution, and we concocted problems that involved the use of different skills to mitigate the effects of behavior that may result from a narrow innate predisposition.
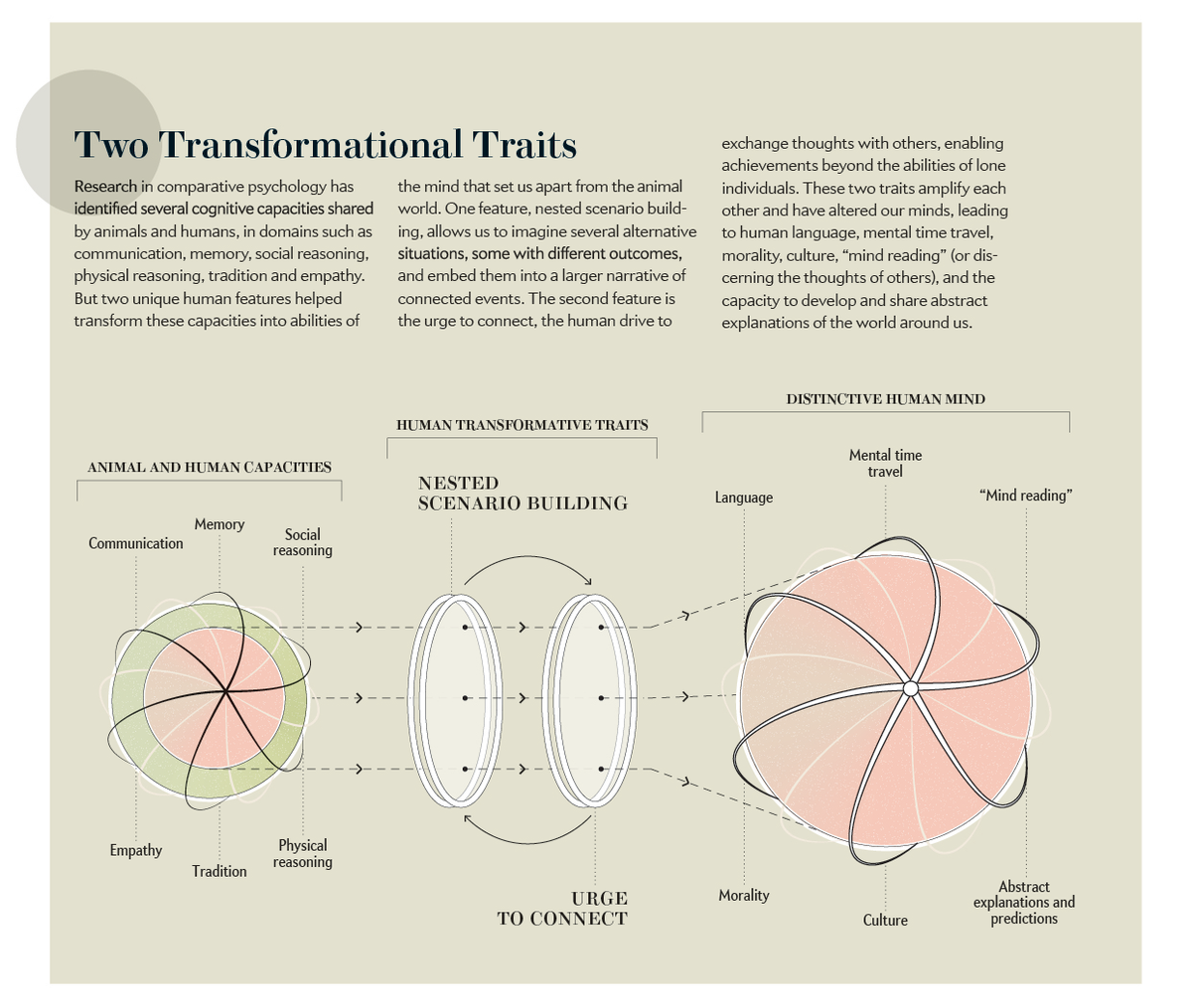
Credit: Federica Fragapane
For example, we showed the youngsters a puzzle box in one room before taking them to another room in which they were distracted with unrelated tasks. After 15 minutes, they were given the opportunity to pick one of several novel objects to take back to the first room. The three-year-olds picked randomly, but the four-year-olds tended to select the object that could later help solve the puzzle they were initially given. We have used this basic paradigm to assess the capacity for deliberate practice, which is the rehearsal of actions aimed at improving future performance [see “ An Evolved Uniqueness ”]. For instance, the children had the opportunity to practice catching a ball on a string with a cup in preparation for a return to the first room, where they could get a reward for success in a similar task. We found that the children could intentionally shape their own future abilities—they would practice the relevant skill in room two—after around age four or five but not before.
These tasks are designed to show basic capacities in areas such as foresight, and they do not map the upper limits of those abilities. When my son was four years old, for instance, we gave him a version of this task, and he succeeded. Later that day, when we were sitting on the bed back home, he put his hand on my thigh and said, “Papa, I don’t want you to die.” When I asked why he thought of that, he said that he would grow up, and I would become a granddad, and then I would die. He had a sophisticated capacity for envisioning the future that produced this unwelcome existential realization. Our study merely demonstrated that he had mental foresight and ruled out the leaner explanations.
The raven research and other animal studies have not met similar stringent criteria for establishing foresight, nor have they demonstrated deliberate practice. Does this mean we should conclude that animals do not have the relevant capacities at all? That would be premature. Absence of evidence is not evidence of absence, as the saying goes. Establishing competence in animals is difficult; establishing the absence of competence is even harder.
Consider the following study, in which my colleague Jon Redshaw of the University of Queensland in Australia and I tried to assess one of the most fundamental aspects of thinking about the future: the recognition that it is largely uncertain. When one realizes that events may unfold in more than one way, it makes sense to prepare for various possibilities and to make contingency plans. Human hunters demonstrate this when they lay a trap in front of all their prey’s potential escape routes rather than just in front of one. Our simple test of this capacity was to show a group of chimpanzees and orangutans a vertical tube and drop a reward at the top so they could catch it at the bottom. We compared the apes’ performance with that of a group of human children aged two to four doing the same thing. Both groups readily anticipated that the reward would reappear at the bottom of the tube: they placed their hand under the exit to prepare for the catch.
Next, however, we made events a little harder to predict. The straight tube was replaced by an upside-down Y-shaped tube that had two exits. In preparation for the drop, the apes and the two-year-old children alike tended to cover only one of the potential exits and thus ended up catching the reward in only half of the trials. But four-year-olds immediately and consistently covered both exits with their hands, thus demonstrating the capacity to prepare for at least two mutually exclusive versions of an imminent future event. Between ages two and four, we could see this contingency planning increase in frequency. We saw no such ability among the apes.
This experiment does not prove, however, that apes and two-year-old humans have no understanding that the future can unfold in distinct ways. As I mentioned, there is a fundamental problem when it comes to showing the absence of a capacity. Perhaps the animals were not motivated, did not understand the basic task or could not coordinate two hands. Or maybe we simply tested the wrong individuals, and more competent animals might be able to pass.
To truly prove this ability is absent, a scientist would have to test all animals, at all times, on some fool-proof task. Clearly, that is not practical. All we can do is give individuals the chance to demonstrate competence. If they consistently fail, we can become more confident that they really do not have the capacity in question, but even then, future work may prove that wrong. The debates between rich and lean interpretations of animal behavior, coupled with this fundamental problem of proving that an ability is always missing, have made it difficult to establish what does and does not set humans apart.
Mind the Gap
Difficult but not impossible. In my book The Gap: The Science of What Separates Us from Other Animals , I surveyed the evidence for cognitive capacities most frequently assumed to be distinctly human and found that animals are smarter than widely thought. For instance, chimpanzees can solve problems through insight, console others in distress and maintain social traditions. Nevertheless, there is something profoundly distinct about human language, foresight, intelligence, culture and morality, and the ability to imagine the thoughts of another individual (we commonly speak about putting yourself in someone else’s shoes). And in each of these domains, two underlying characteristics kept reemerging as making the critical human-animal difference. One is what I call “nested scenario building,” which is our ability to imagine alternative situations, reflect on them and embed them into larger narratives of related events. The other is the “urge to connect,” which is our deep-seated drive and capacity to exchange our thoughts with others, when we put our minds together to create something greater than what one individual can do alone.
Nested scenario building enables us to imagine other people’s situations, moral conundrums or entirely fictional stories. In the context of thinking ahead, it allows us to picture potential future events, reflect on possibilities and embed them into larger stories of unfolding events. This, in turn, enables us to plan and prepare for opportunities and threats before they materialize.
Other animals, even bacteria, are attuned to long-term regularities such as day-night rhythms, and many can adjust to local patterns as well. Through associative learning, animals can predict that a reward or punishment is coming after a specific event. But people can mentally entertain situations, even entirely novel scenarios without external triggers, by combining and recombining in our mind basic elements, such as actors, actions and objects, and we can draw prudent conclusions from these mental exercises. A simple example: you can picture playing blindman’s bluff on a busy street and figure out that it is a dangerous proposition even if you have never been in that situation. Nested scenario building depends on a host of sophisticated abilities working in concert, including imagination, memory, reflection and executive decision making.
Think of creating nested scenarios as an internal theater in which we can bring situations to life. Like a play, scenario building depends on certain components that have to come together. There is a “stage” to imagine events that are not actually occurring at that moment. Those events involve “actors” and their “set”: individuals and objects that are linked in a narrative. We also employ capacities akin to a “director” who evaluates and manages the scenes and an “executive producer” who makes the final decisions about what to pursue. These components map onto psychological constructs such as working memory, recursive thought and executive function, features that develop at different rates during human childhood. As a result, competence at foresight emerges slowly as we mature. And as adults, we still frequently fail to anticipate future situations accurately—I most certainly do. We are not clairvoyants.
Thus, because nested scenario building is a risky way to reach decisions, humans need to pair it with that second characteristic: connecting our minds. Psychologist Michael Tomasello has described this ability as shared intentionality [see “ The Origins of Morality ”]. After all, the best way to find out about the future is to ask someone who has already been there, as it were.
If you really want to know what a holiday in New Zealand is like or what a career in psychology entails, you can envision all the scenarios you want, but your best bet is to ask someone who has been to that country or has pursued such a career. Human language is ideally suited for such exchanges; most of our conversations are about events displaced in time. In this way, we can learn from one another’s experiences, reflections and plans. We ask questions and give advice, and we build deep bonds in the process. What is more, we can also shape the future in more deliberate ways by coordinating our actions in the pursuit of shared goals. We often do this by commenting on a companion’s strategy, reviewing progress and then guiding the person to the next step.
Most of our extraordinary powers, when you think about it, derive from our collective wit. Consider that we all benefit from the tools and technologies other people invented. Many animals use tools, and some even make them, but to turn them into an innovation, one has to recognize that it will be useful again in the future. After that realization, one has a reason to retain the tool, to refine it further and to share it with others.
We can see this evolution in our inventions of increasingly effective ways to cause harm at a distance. This was probably a vital capacity for our early ancestors, who shared the land with dangerous saber-toothed cats. At first, our progenitors may have thrown rocks to drive away predators, but eventually they armed themselves with spears, then invented spear throwers, and then bows and arrows. New tools are only an advance, however, if one can use them effectively, which brings us back to deliberate practice. Chimpanzees in Senegal have been reported to make rudimentary spears that they thrust into tree hollows to kill bush babies. But there is as yet no observation that they practice thrusting, let alone throwing. Unlike humans, they could not benefit from the invention of a spear thrower. You can safely give them one of ours; they would not use it as we do.
The earliest evidence of deliberate practice is more than a million years old. The Acheulean stone tools of Homo erectus some 1.8 million years ago already suggest considerable foresight, as they appeared to have been carried from one place to another for repeated use. Crafting these tools requires considerable knowledge about rocks and how to work them. At some sites, such as Olorgesailie in Kenya, the ground is still littered with shaped stones, raising the question of why our ancestors kept making more tools when there were plenty lying around. The answer is that they were probably practicing how to manufacture those tools. Once they were proficient, they could wander the plains knowing they could make a new tool if the old one broke. These ancestors were armed and ready to reload.
Most animal species can be categorized as either specialists or generalists, but humans are both: we are capable of quickly adapting to local demands, even to anticipated demands, by acquiring relevant expertise. Moreover, through cooperation and division of labor, we can benefit from complementary skills, thereby enabling us to dominate most diverse habitats. We can keep even the fiercest predators in our zoos because we can foresee what they need and what they can and cannot do. So far there is no obvious evidence of other species engaging in such mental time travel nor in exchanging plots for a coordinated escape from the zoo when the conditions are right next summer.
With nested scenario building and the urge to wire their minds together, our ancestors eventually spawned civilizations and technologies that have changed the face of the earth. Science is the disciplined use of our collective wit, and we can deploy it to better understand the origin of our place in nature. We can further use it to model the future systematically and ever more clearly. By foreseeing the consequences of our actions, we are also confronted with moral choices between different options. We can predict the consequences of continuing pollution or destruction of animal habitats, inform others about them and, as the Paris climate agreement dramatically demonstrates, negotiate globally coordinated actions aimed at more desirable outcomes.
None of this is an excuse for arrogance. It is, in fact, a call for care. We are the only creatures on this planet with these abilities. As Spider-Man’s Uncle Ben declared, communicating complex ideas in an urge to connect with his superhero nephew, “With great power comes great responsibility.”
Thomas Suddendorf is a professor of psychology at the University of Queensland in Australia. He studies the development of mental capacities in young children and nonhuman primates to answer fundamental questions about the nature and evolution of the human mind.
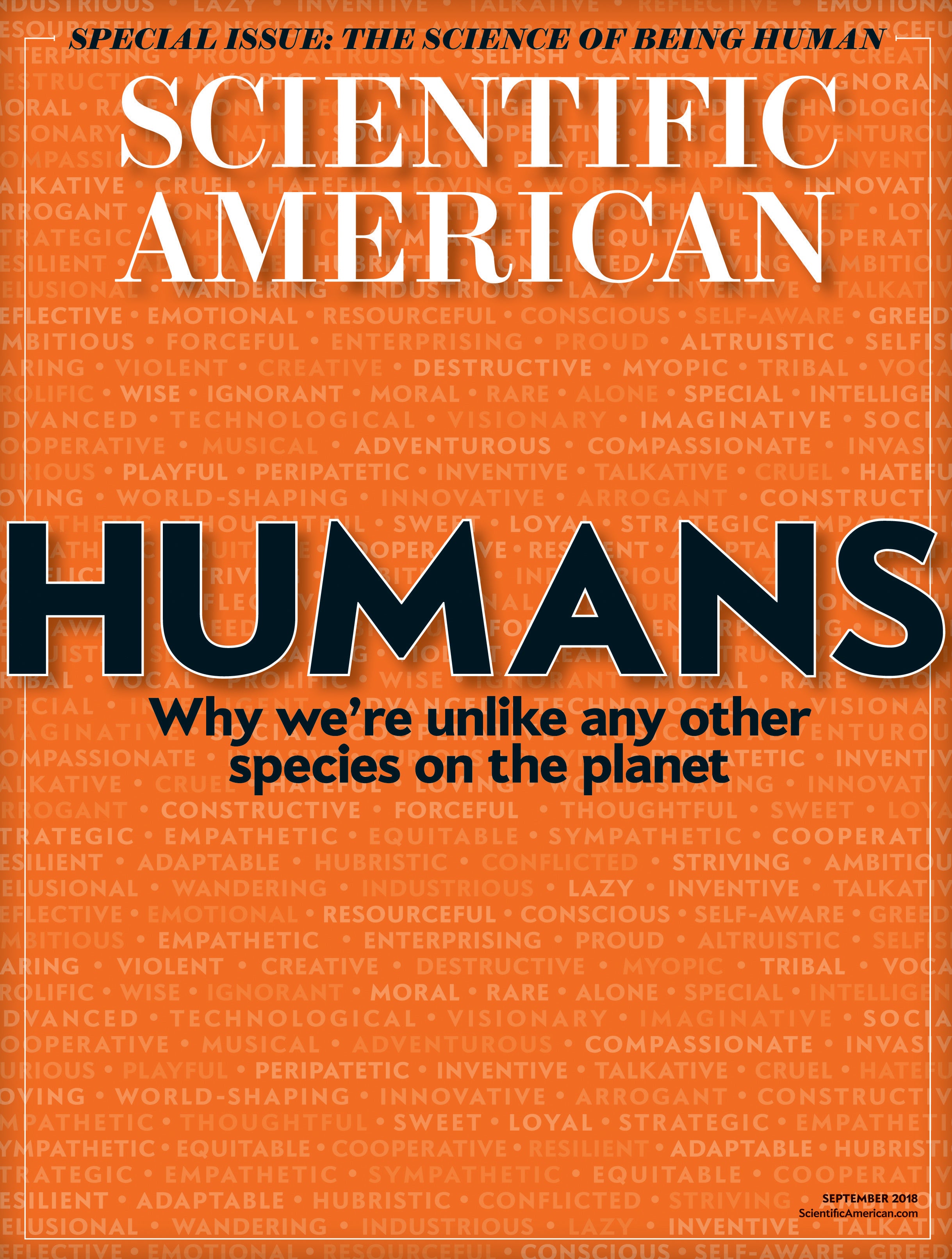
HYPOTHESIS AND THEORY article
Insights into conscious cognitive information processing.
- 1 Department of Behavioral and Clinical Neuroscience, Ruhr-University Bochum, Bochum, Germany
- 2 Unité de Formation et de Recherche des Sciences de la Vie (UFR 927), Institut de Biologie Paris-Seine, Sorbonne Université, Paris, France
For over a century, the neuro- and pathophysiological, behavioral, and cognitive correlates of consciousness have been an active field of theoretical considerations and empirical research in a wide range of modern disciplines. Conscious cognitive processing of information cannot be observed directly, but might be inferred from step-like discontinuities in learning performance or sudden insight-based improvements in problem solving behavior. It is assumed that a sudden step of knowledge associated with insight requires a creative reorganization of mental representations of task- or problem-relevant information and the restructuration of the task, respectively problem to overcome an cognitive dead-end or impasse. Discontinuities in learning performance or problem solving after an insight event can be used as time-tags to capture the time window in which conscious cognitive information processing must have taken place. According to the platform theory of conscious cognitive information processing, the reorganization and restructuration processes, require the maintenance of task- or problem-relevant information in working memory for the operation of executive functions on these mental representations. Electrophysiological evidence suggests that the reorganization and restructuration processes in working memory, that precede insight-based problem solutions are accompanied by an increase in the power of gamma oscillations in cortical areas including the prefrontal cortex. Empirical evidence and theoretical assumptions argue for an involvement of gap junction channels and connexin hemichannels in cortical gamma-oscillations and working memory processes. Discontinuities in learning or problem solving performance might be used as time-tags to investigate the implication of gap junction channels and hemichannels in conscious cognitive processing.
Conceptual issues with consciousness
Detailed information on the neurophysiological and molecular mechanisms, as well as regarding the behavioral correlates of consciousness is still scarce ( Dere et al., 2021 ; Zlomuzica and Dere, 2022 ). This gap of knowledge is even more astonishing in that the keyword “consciousness” entered into the online scientific publication database PubMed returns more than 59.000 hits. Nevertheless, there is no general definition in sight that would be unanimously accepted by all the different disciplines ( Dere et al., 2021 ; Zlomuzica and Dere, 2022 ). Owed to this conceptual vacuum, the measurement of cognitive, behavioral and neurophysiological correlates of consciousness in animals and humans has been an extremely challenging task. This an untenable situation, if one considers that altered consciousness (e.g., lack of insight into illness, tunnel vision, altered attention, perception and biased processing of disease-relevant stimuli) is a frequent symptom (and sometimes obstacle for successful treatment) among mental, neurological, and psychiatric diseases ( Bob et al., 2016 ; Dere et al., 2021 ; Zlomuzica et al., 2022 ; Zlomuzica and Dere, 2022 ; Martin, 2023 ; Stefanelli, 2023 ). However, due to the conceptual difficulty indicated above, alterations in consciousness as a clinical symptom is usually neglected (except in severe cases subsumed under the term disorders of consciousness) where the patient is no longer responsive or oriented in terms of time, location and personal information ( Edlow et al., 2021 ).
Conscious cognitive information processing
In order to resolve the definition issue and to pave the way to an empiric approach to this phenomenon, one should focus on the adaptive function of consciousness in everyday life and ask, which situations would probably induce a conscious cognitive information processing in the brain. It is reasonable to assume that people engage in conscious cognitive information processing when they are confronted with novel situations in which they cannot apply learned behavior, habits or scripts to master the situation. Such situations can occur, when a problem emerges (for example when environmental reinforcement contingencies suddenly change) and there is no masterplan at hand to cope with such an irregularity.
In contrast, one does not necessarily need conscious cognitive information processing to drive a car, prepare a meal or take a shower. Here, a mental autopilot driven by scripts, habits, reflexive and genetically predetermined behavior, and automatic motor programs would be sufficient to maintain such “unconscious” behavior. However, when something unexpected happens, a new problem is posed, conscious cognitive information processing is required to resolve the situation. Another situation that is likely to require conscious cognitive information processing is mental time travel ( Breeden et al., 2016 ; Dere et al., 2019 ). People engage in mental time travel to plan for the future, anticipate difficulties and problems and prepare effective coping strategies for such anticipated or imagined problems. Mental time travel into an anticipated or imagined future cannot be successfully implemented without conscious cognitive information processing.
An operational definition of conscious cognitive information processing has been proposed by Dere et al. (2021) . The central statement of this definition is that conscious cognitive information processing is initiated, whenever novel situations or problems are encountered that need to be resolved, or when mental time travel is performed to reconstruct past experiences that can be exploited to anticipate, imagine and prepare for future events in order to maximize the probability to experience rewarding situations and to minimize the probability of experiencing aversive situations ( Dere et al., 2021 ).
During conscious cognitive information processing, representations of perceived interoceptive and/or exteroceptive stimuli, as well as related semantic concepts, memories, and experiences are effortfully maintained in working memory to be actively manipulated (e.g., reorganized or restructured) in order to generate a novel creative output. According to this definition conscious cognitive information processing critically depends on the complexity of the situation with which the brain is dealing with and the complexity of the information that is actively manipulated on the working memory workbench ( Dere et al., 2021 ).
Platform theory of conscious cognitive information processing
The above definition is part of a larger theoretical framework, designed as the platform theory of conscious cognitive information processing, that attempts to explain how conscious cognitive information processing guides and controls flexible, respectively, adaptive behavior in humans and probably animals ( Dere et al., 2019 , 2021 ; Zlomuzica and Dere, 2022 ). The platform theory of conscious cognitive information processing proposes a hierarchical model of perception, memory, cognition and conscious cognitive information processing that is composed of a central executive/online processing platform that serves as a conscious cognitive operation control center that organizes, monitors and orchestrates subordinate operation and storage units called platforms (see Figure 1 for a summary of the platform functions). Conscious cognitive information processing requires the maintenance of mental representations of internal and external stimuli as well as related semantic concepts, memories, and experiences on a working memory platform that is endowed with a multitude of sophisticated executive functions ( Breeden et al., 2016 ; Dere et al., 2019 , 2021 ; Zlomuzica and Dere, 2022 ). The subordinate platforms serve, for example, as storage media for semantic and episodic knowledge or planned actions, activities, and the working-off of daily agendas.
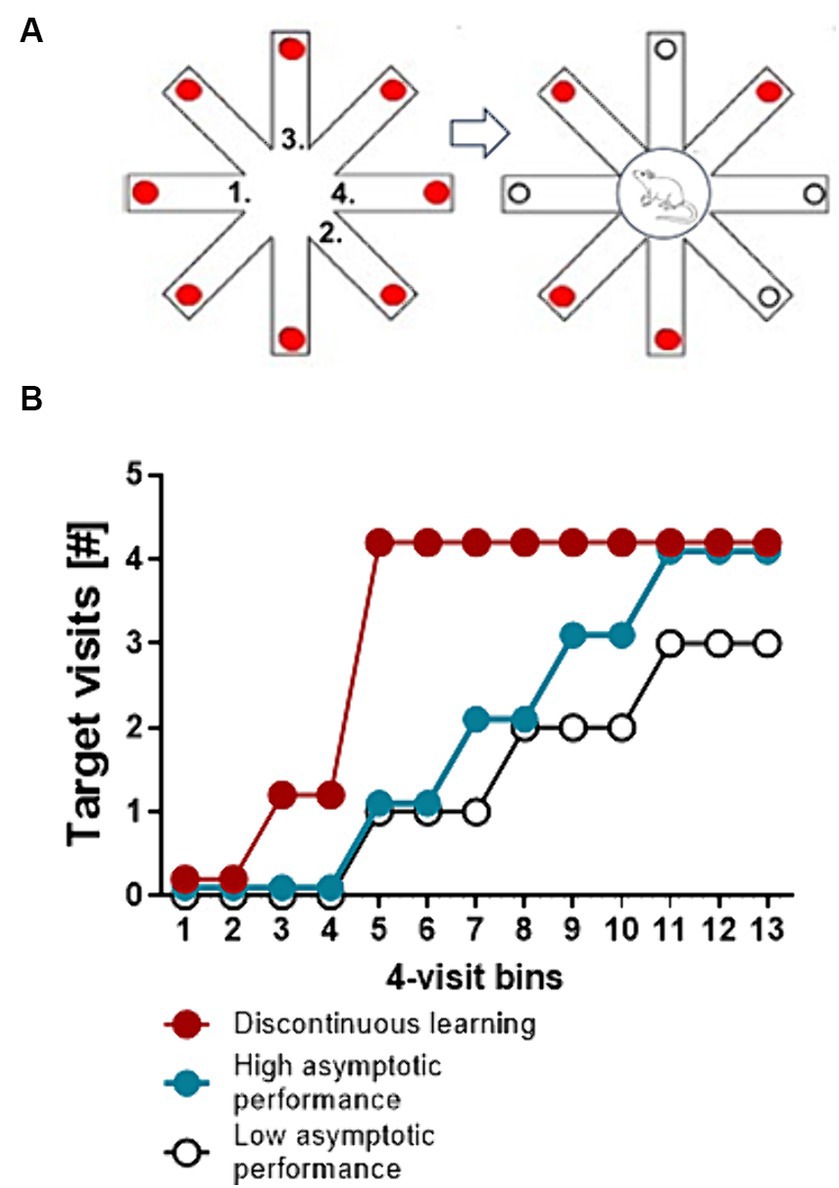
Figure 1 . Scheme of the different components of the platform theory of conscious cognitive information processing. The central executive/online platform is a consciousness control center that organizes and monitors conscious mental operations by the orchestration of subordinated operation and storage units called “platforms.” The main function of the central executive/online platform is the generation of a daily schedule or agenda with goals to archive and the allocation of different grades of consciousness to mental representations or contents that are required to accomplish these tasks. The central executive/online platform selects mental representations (internal or external stimuli, stored content from the off-line memory platform) to be maintained, used, manipulated, or to be placed on the steady-state or stand-by platforms. The steady-state, stand-by, and off-line memory platforms do not execute cognitive operations, but rather provide information and content that is important to accomplish tasks or solve problems. The contents of declarative and non-declarative memory including episodic, semantic, and procedural information are stored in the off-line memory platform and are accessible to the central executive/online and mental time travel platforms. The central executive/online platform controls and presets attentional stimuli filters and receives input from sensory systems, as well as from motivation, emotion, vegetative, and motor systems. Intense stimuli can by-pass the attentional filters and directly access the central executive/online platform. The central executive/online platform recruits the mental time travel platform to re-construct past experiences, anticipate future events, or simulate and calculate the outcomes of different actions in imagined scenarios by feeding this platform with information from external and internal perceptions, as well as stored content from the off-line memory platform ( Breeden et al., 2016 ; Dere et al., 2019 , 2021 ; Zlomuzica and Dere, 2022 ).
It has been proposed that the stream of consciousness including conscious perception, conscious processing of information and metacognition relies on the synchronization of neuronal network activity across distant cortical regions ( Alkire et al., 2008 ; Koch et al., 2016 ; Tian et al., 2020 ; Valencia and Froese, 2020 ). These far-reaching synchronization processes are always neuronal mass phenomena with large amplitudes (otherwise the signal could not be filtered out from the background noise), that only vary by the oscillation frequency ( Meador et al., 2002 ; Northoff, 2017 ). It is difficult to believe that such mass phenomena can represent specific content or cognitive processes. It is more reasonable to assume that they represent more global network synchronization states that enable specific conscious cognitive information processing in local neuronal circuits which in contrast are likely to have an neuronal activity pattern that is sequential, reverberatory, and asynchronous to the global neuronal network synchronization in which these local neuronal circuits are embedded ( Singer, 1998 ; Dere et al., 2021 ; Zlomuzica and Dere, 2022 ; Zlomuzica et al., 2023 ). The activity of local neuronal circuits as singular phenomena cannot be detected with electrophysiological methods such as the EEG and MEG or neuroimaging approaches and require intracerebral and intracellular recordings.
According to the platform theory of conscious cognitive information processing, conscious cognitive information processing and adaptive behavior is supported by local neuronal circuit and neuronal network oscillations shaped by gap junction channels and connexin hemichannels ( Dere et al., 2021 ; Zlomuzica et al., 2022 , 2023 ; Zlomuzica and Dere, 2022 ). The platform theory of conscious cognitive information processing also proposes a molecular mechanism for conscious cognitive operations in everyday life, including the execution of planned actions, and the accomplishment of behavioral goals and agendas. Specifically, it is proposed that pacemaker cells or neural circuit pulsars embedded into local neuronal circuits and more far-reaching networks ( Le Bon-Jego and Yuste, 2007 ; Wittner and Miles, 2007 ; Kocsis et al., 2022 ), connexin hemichannels, and gap junctions jointly generate local neural circuit (and network) oscillations ( Draguhn et al., 1998 ; Coulon and Landisman, 2017 ; Mäkinen et al., 2018 ; Traub et al., 2018 ), which are the basis for the maintenance of mental representations in working memory ( Allen et al., 2011 ), to enable the performance of conscious cognitive operations on these mental representations, and the initiation of behavioral changes, as consequences of these conscious cognitive operations ( Dere et al., 2021 ).
What is the “added value” of the platform theory of conscious cognitive information processing compared to other empirical and theoretical approaches to consciousness?
There is a great deal of research that attempts to correlate very general “states” of consciousness with corresponding “levels” of consciousness. These states include coma, vegetative state (unresponsive wakefulness syndrome), minimally conscious state, sleep and the awake state ( Laureys et al., 2010 ). However, since coma and vegetative state are usually the consequence of a severe disease, injury or poisoning, they are essentially manifestations of brain dysfunction or breakdown, rather than states of consciousness. The inability to maintain consciousness after brain damage or the absence of consciousness in a diseased brain should not be considered as a state of consciousness or a condition that should be investigated to understand the nature of consciousness in an intact and operational brain. If one follows such a rationale until its end, death could be also regarded as a state of consciousness that is associated with an “absolute zero” level of consciousness.
Attempts have also been made to determine the neural basis of consciousness through imaging and electrophysiological measurements during the regaining or recovery of consciousness, for example after anesthesia or upon awakening ( Edlow et al., 2021 ). The regaining of consciousness has been related to the “re-boot” of resting state networks including the default mode network, fronto-parietal network, and the salience network ( Li et al., 2023 ). At most, these measurements can be used to distinguish between a waking receptive state (and the brain networks required) and a non-receptive state, but they do not say much about brain processes that are associated with conscious cognitive information processing (see also Chenot et al., 2024 ). To use a metaphor, it’s like measuring the lowering of a rolled up ceiling recessed projector screen to project content onto it. It does not give much information about the projector or the content that is being projected.
Yet, another line of research probes the awake brain to tease apart conscious and unconscious processing. This seems to be a more convincing and promising approach which is also followed by the platform theory of conscious cognitive information processing. According to this line of research, conditions, respectively, experimental situations have to be created, in which conscious cognitive information processing is likely to be initiated and therefore can be measured. In other words, the “brain” can be actively put in a situation which “inevitably” initiates conscious cognitive information processing and can be “observed” or “measured” during conscious cognitive information processing. In the context of the platform theory of conscious cognitive information processing, consciousness is treated as an observable variable and it is proposed that conscious cognitive information processing is initiated in situations where the owner of the brain is confronted with a novel problem for which there is no prefabricated solution available. Consequently, it is assumed that, at the moment of an insight-based solution, the brain was engaged with conscious cognitive information processing, which is different from unconscious information processing. In other words, the changes in the electro- and neurochemical activity of different areas of the brain that accompany this special moment of insight will certainly tell us much more about the neurophysiological mechanisms of conscious cognitive information processing than the comparison of functional network activity during pathological brain states including the unresponsive wakefulness syndrome and minimally conscious state ( Panda et al., 2022 ).
Furthermore, there are reductionist approaches that attempt to identify electrophysiological correlates for the conscious subjective perception of visual stimuli (in contrast to basic activity that is evoked by mere sensory stimulation) in order to gain insight into neurophysiological mechanisms and principles which might also help to understand more complex phenomena, including conscious cognitive information processing. Animal research in this area has suggested that visual awareness is reflected by power modulation of high-frequency local field potentials (in the gamma oscillation range) in the lateral prefrontal cortex, temporal and parietal cortex, where spiking activity is found to be perceptually modulated ( Panagiotaropoulos et al., 2012 ; Tseng et al., 2016 ). The gamma oscillations in this brain network might be related to the maintenance of “conscious visual perceptions” in working memory (or online platform) for further conscious cognitive information processing in order to generate a novel creative output. In this regard these visual consciousness approaches emphasize the importance of working memory for the generation and maintenance of conscious cognitive information processing, in a similar way to the platform theory of conscious cognitive information processing ( Dere et al., 2021 ).
One of the most influential theories of consciousness is Bernhard J. Baars’ Global Workspace theory (updated in Baars et al., 2021 ). It attempts to explain how a serial, integrated and very limited stream of consciousness emerges from a system of specialized “receiving processes” that are “unconsciously” working in parallel. The most peripheral “receiving processes” transmit information from primarily sensory pathways to downstream “receiving processes” that process and interpret these sensory information. The content entering the global workspace is then projected to secondary cortical sensory association areas. It is proposed that only the most significant information is allowed to enter the global workspace, which in turn can be regarded as a fleeting memory area with a limited storage capacity (in the range of seconds). It is assumed that only information that is passively stored (for a few seconds only) within the global workspace is conscious. “Consciousness” is regarded as a passive response (the information in the global workspace is not subject to cognitive operations) to a significant and intense sensorial stimulation. Formulated pointedly, the global workspace theory merely describes a sensory gripping reflex without specifying the neuronal or molecular mechanisms that underlie this function ( Zlomuzica and Dere, 2022 ). The global workspace is therefore not well suited to maintain a larger amount of information which might be required to solve complex problems. It is therefore not clear how this model can explain conscious cognitive information processing, complex problem solving or mental time travel into the past and future in any way better that the platform theory of conscious cognitive information processing (see also Zlomuzica and Dere, 2022 ).
Another popular theory of consciousness is the integrated information theory, since it provides a mathematical model to actually measure the level of consciousness in any neuronal network or other system that is potentially capable to generate consciousness ( Tononi et al., 2016 ). In the framework of this theory it is assumed that consciousness is a subjective, immediate, direct, and unified process. It further attempts to define the basic properties of a physical system capable of generating consciousness. According to this theory, consciousness necessities an interconnected set of elements with reentrant feed-back loops, in which the single elements have a mutual physical cause-effect powers on each other leading to the integration of information. The integrated information theory equates integrated information with consciousness, suggests that the degree of consciousness (in both quantity and quality) is measurable by determining the amount of intrinsic cause-effect power via phi metrics and extends its claims beyond human consciousness to animal and artificial consciousness. The integrated information theory attempts to define the necessities and operation mode of physical substrates of consciousness in the process of generation of integrated information and system-immanent intrinsic modulation. Testing the compatibility of this theory with the platform theory of conscious cognitive information processing would require measuring the postulated cause-effect system-immanent intrinsic-modulation during the execution of a conscious cognitive information processing or insight event. It is currently difficult to imagine what such a proof-of-principle experiment should look like. In a first step, one could try to detect corresponding cause-effect activity patterns or self-modulating systems in an organic model system such as brain slice preparations from animals or brain organoids. As promising and intuitively plausible as this theory may seem at first glance, the essential assumptions of the theory are essentially claims that have no empirical basis and are hardly amenable to experimental verification in a healthy, intact and awake brain.
Conscious cognitive information processing in animals
Despite the importance of conscious cognitive processing for the mental life and adaptive behavior of humans (and with limitations in animals), and in view of the devastating consequences of impaired conscious cognitive processing in patients with mental disorders and neuropsychiatric diseases, there is no valid animal model of conscious cognitive information processing available, that could be exploited for psychopharmacological, comparative and translational research ( Zlomuzica et al., 2022 , 2023 ; Dere and Zlomuzica, 2023 ). Animal models of conscious cognitive information processing have to deal with important issues of construct, face and predictive validity ( Dere et al., 2021 ; Zlomuzica and Dere, 2022 ). In a recent review, the few available paradigms to measure animal consciousness have been reviewed and the conclusion was reached, that all available tests have major shortcomings and are not well suited to serve as routine tests for neurobiological and pre-clinical research ( Zlomuzica and Dere, 2022 ). Instead the combination of a number of sophisticated cognitive tests in a behavioral test battery and the calculation of individual composite performance scores might be a better approximation to the measurement of conscious cognitive information processing. However, the proposed test battery is very time consuming, requires considerable equipment, training, and expertise with behavioral testing. These factors will probably hinder the application this test battery on a larger scale. There might be an “cheaper” and more direct path to conscious cognitive information processing in animals and humans.
Classic and contemporary definitions of the insight phenomenon by cognitive psychologists conceptualize insight as a sudden comprehension, realization, or creative problem solution that is based on a reorganization of mental representations of relevant information that is opposed to incremental trial-and-error learning ( Hebb, 1949 ; Thorpe, 1956 ; Kounios and Beeman, 2014 ; but see Bowden et al., 2005 for a different view). A sudden insight into the nature of a problem that leads to an instantaneous step of knowledge is likely to be accompanied by a sudden and strong emotional arousal ( Shen et al., 2017 ) resulting in the formation of an episodic memory ( Dere et al., 2006 , 2010 ; Kinugawa et al., 2013 ; Pause et al., 2013 ), which probably from this point on is the mnemonic basis of the persisting improvement in task performance.
In Wolfgang Köhler’s insight learning theory ( Köhler, 1917 , 1925 ), insight is defined as an instantaneous type of understanding of relations and reinforcement contingencies that can emerge without prior trial-and-error learning and that leads to a solution to a current problem. Learning success based on insight has been proposed to be conceptually incompatible with learning based on operant conditioning or reinforcement learning which is associated with a gradual buildup of a reward value or reinforcement signal for the correct response or sequence of responses accumulated through experiences ( Thorndike, 1911 ; Schultz et al., 1997 ).
Insight and conscious cognitive information processing
Event-related potential studies suggest that complex perceptions and cognitive processes can occur in the range of milliseconds. It has been proposed that a sudden step of knowledge might also be the result of a latent process that that runs unconsciously in the background and suddenly reaches consciousness while escaping metacognitive monitoring up to this point of time (see Tolman, 1948 ; Qiu et al., 2008 ).
There is an ongoing debate on whether insight is the result of conscious information processing (in the form of progress monitoring) after it has been realized that conventional solution attempts will not create the goal configuration or attenuate the problem space, e.g., in the 9-dot problem ( MacGregor et al., 2001 ) or an unconscious process in which self-imposed constraints on a problem or misleading presuppositions are discarded, and chunked items in the problem are decomposed and redistributed ( Knoblich et al., 2001 ).
There are also many anecdotical stories of sudden “inspirations” of how great researchers and scholars suddenly had an idea which immediately solved a problem that they had been trying to solve for a long time (think of the apple falling on Newton’s head or Archimedes idea to calculate material density through water displacement). The truth content of these romanticized stories of great scientists and inventors might be questionable. Nevertheless, many people report similar experiences of “inspiration” defined as a non-religious or mystical thought or idea that suddenly arises, is recognized as a solution to a problem, and seems to be detached from the actual context and current stream of thoughts or thinking.
It is not plausible to assume that an inspiration comes out of “nothing,” i.e., without preparatory cognitions. It seems for example more plausible to assume that conscious cognitive processing of information took place in advance, but the end product of this reasoning has not been recognized as the solution (and therefore has been “put aside”). It is also possible that in the course of reasoning, initially a small fragment of the solution was missing, but has been added at a later point in time. Phenomenologically, these processes might be felt as a flash of inspiration propelled by an metacognitive illusion ( Dere et al., 2021 ). Therefore, it seems to be more reasonable to assume that an “inspiration” is probably the intrusion of an insight-based solution (or promising part of the solution after a period of “incubation”), that has not been acknowledged as such by the time it was generated. For the remainder of this article insight is defined as the end product of an ongoing conscious cognitive information process, which should not be equated with the term “inspiration.”
Examples for insight-based problem solving in humans, non-human primates, and laboratory rats and mice
Robert W. Weisberg proposed in his integrated theory of insight in problem solving, that insight depends on conscious cognitive operations that aim to restructure problem-relevant information, in a way that the new information structure (comparable to individual puzzle pieces that have been put together), enables a direct solution to the problem ( Weisberg, 2015 ). Insight can be regarded as the endpoint or manifestation of a hidden problem solving mechanism and has been studied, for example, with ill-structured innovation tasks, including the hook bending paradigm or the tower of Hanoi. These tasks have in common that they require a multi-step solution, whereby the solution path is unknown and only information about the goal or target configuration is provided. Children who were at least 7 years old usually arrived suddenly at the solution to the hook bending problem, suggesting an insight-like problem solving mechanism ( Defeyter and German, 2003 ; Cutting et al., 2014 ). The hook bending problem has also been posed to non-human primates and large brained birds, which both showed task performance (e.g., very few failures after the task has been solved for the first time) that was interpreted in the sense of tool innovation that was made possible through sudden insight into the problem ( Weir, 2002 ; Bird and Emery, 2009 ; Laumer et al., 2018 ).
The Gestalt- and comparative psychologist Wolfgang Köhler investigated the phenomenon of insight in nonhuman primates ( Köhler, 1917 , 1925 ). The food-deprived chimpanzee Sultan had to realize that two small sticks in the cage could be inserted into each other to be able to pull a banana within reach that had been placed outside the cage. After several unsuccessful attempts to reach the banana with one of the two sticks, Sultan managed to put the two small sticks together by accident. Sultan immediately took the stick and pulled the banana towards him. However, this behavior cannot be clearly interpreted as insight because the solution was found more or less by chance. Nevertheless, Sultan understood that he needed a longer stick and when he saw it, he considered the problem solved and went straight to implementing it ( Köhler, 1917 ). However, it must be noted that the experiment was probably not designed in an ecologically valid way. In the wild, respectively, nature there are usually no sticks that can be stuck together, just like there are no magic wands. Therefore, Sultan would possibly not have been able to find the solution right away through prior conscious cognitive processing of all the information available. However, it is quite possible that Sultan, as a result of conscious cognitive processing of information, came to the conclusion that only a stick twice as long would make it possible to pull the banana.
As previously mentioned, insight can emerge from reorganizing or restructuring information ( MacGregor et al., 2001 ; Weisberg, 2015 ). However, this cognitive process can be impaired if a so-called functional fixation is present ( McCaffrey, 2012 ). This means, for example, that a tool is very strongly associated with a certain type of use or activity, so that a potential unconventional use of it to solve a problem is masked. Consequently, it has been shown that great apes have difficulties to find a solution to a new problem, when the available tools have been strongly associated with a different type of use or the solution of another problem ( Ebel et al., 2020 ). Again task design seems to be highly critical for the usefulness or sensitivity of a task or paradigm to detect problem solving based on insight.
The first study into the question of whether lower mammals such as rats are able to solve problems through insight was published by Helson (1927) . In the first phase of an elegant discrimination learning experiment, Helson trained one pair of rats (first pair) to prefer a food-rewarded light (60 W illumination) over a non-rewarded dark compartment (15 W illumination) and another pair of rats (second pair) to show the opposite preference. In the second phase of the experiment the illumination intensity of the two compartments was changed to 150 W and 60 W for the first pair of rats and to 15 W and 1 cd for the second pair of rats, while the reward contingency or task rule was not changed. The first pair would receive a reward for choosing the lighter compartment, while the second pair would receive a reward for the darker compartment. After the change of the intensity values of the stimuli presented the rats continued to prefer the light, respectively, dark compartment, suggesting that their decision was guided by structure–function relationships rather than simple stimulus–reward associations. The latter would require the rats to stay with the initially rewarded stimulus rather than to switch immediately to a novel stimulus that has not been paired with a reward. Helson concluded that the adaptive behavior of the rats was based on insight into the structure or general rule of the task ( Helson, 1927 ).
Behavioral correlates of insight as a manifestation of conscious cognitive information processing
Insight is a singular phenomenon that cannot be reliably reproduced over multiple teaching sessions and it can only be examined at the level of an individual and not in groups (but see Zlomuzica and Dere, 2022 ). Conscious cognitive processing of information cannot be observed directly but can perhaps be inferred from discontinuities in learning or problem solving behavior. As indicated above conscious cognitive information processing is initiated whenever a novel problem is posed that cannot be solved through learned, instinctive, or reflective behavior ( Dere et al., 2021 ; Zlomuzica and Dere, 2022 ) and it is realized that there is no ready-made solution available.
A new problem might be initially addressed through trial-and-error learning ( Thorndike, 1911 ). This type of problem solving strategy depends on randomly generated actions or sequences of actions. One of which happens to be correct and brings about the desired solution to the problem. Trial-and-error learning is generally characterized by gradual, incremental or continuous learning ( Thorndike, 1911 ). Even after a correct action or sequence of actions has been executed and the problem has been temporarily solved, it is possible that the correct response to the problem is not remembered shortly after. Just imagine that you incidentally managed to solve a “computer or software problem,” there is no guarantee that you will remember the sequence of actions that you have performed the next time when you are confronted with the same or a similar problem. The trial-and-error learning process may thus be more tedious than a quicker solution based on insight. The latter by definition is characterized by discontinuous or step-like learning and a sudden “step of knowledge” which stamps in permanently the correct response to the problem. The main point of this review is the hypothesis that learning and associated abrupt changes in performance through insight are likely to be the consequence of previous conscious cognitive information processing and that sudden and persistent changes in learning performance (discontinuities in learning performance) can be used as time tags indicating the time windows in which conscious cognitive information processing must have taken place. Equally, it can be assumed that an individual who exhibits merely continuous learning without abrupt increases in performance has not initiated conscious cognitive information processing to solve the problem.
Translated into an experimental learning paradigm this sudden insight would be reflected by an equally sudden and stable improvement in performance ( Gallistel et al., 2004 ). The analysis of the performance dynamics of individual participants or experimental animals during the acquisition of a task can help to identify the time point of this discontinuity in performance and thus the time point when conscious cognitive processing has been initiated. On the other hand the analysis of performance dynamics can also differentiate between slow and fast incremental continuous learning, that is the distinction between superior and inferior learners that do not engage in conscious cognitive processing and thus do not show discontinuities in learning performance ( Figure 2 ).
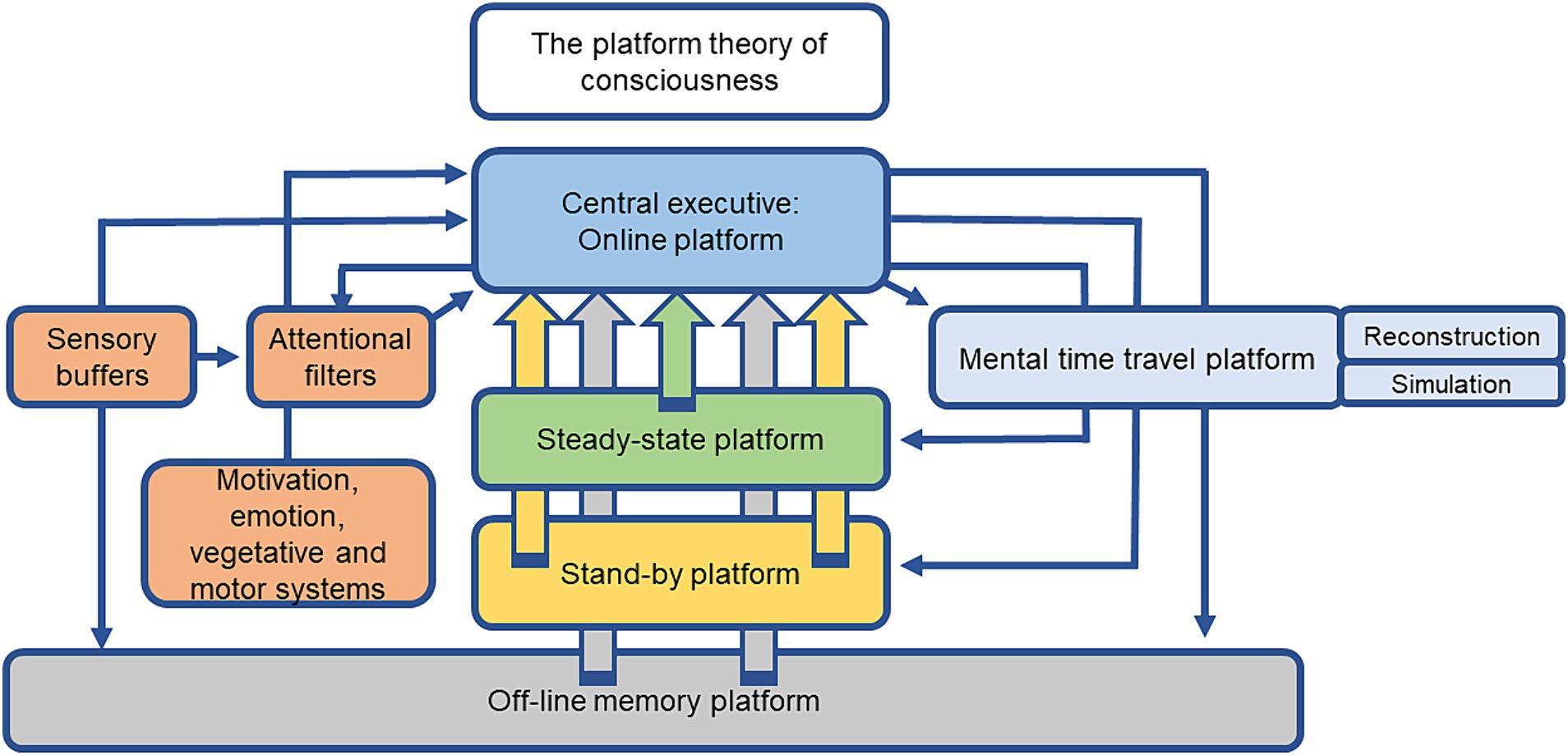
Figure 2 . Discontinuous and continuous learning performance. (A) Schematic drawing of a simple spatial working memory test to identify continuous and discontinuous learning performance. Depicted is a food-rewarded radial eight-arm maze. Red circles represent food rewards. The test starts with a free choice trial, in which a food deprived animal is allowed to make 4 choices. An example of a sequence of choices is indicated by the numbers. After the 4 th choice the animal is trapped at the central platform for a retention interval (e.g., 10 s). Thereafter all doors are unblocked and the animal is allowed to make 4 more choices. The number of correct choices in 4-choice bins (target choices of arms which are still baited) is recorded. (B) Line and scatter plots represent idealized learning curves (discontinuous learning, fast and slow incremental learning) of individual mice. Discontinuous learning is characterized by a sudden and stable improvement in the number of correct target choices within 4-choice or corner visit bins. Continuous learning is characterized by gradual incremental improvements in performance. The speed of learning equals the slope of the curve while a learning impairment is indicated by low asymptotic performance.
In a recent experiment by Rosenberg and colleagues, mice that had to solve a complex labyrinth navigation problem showed “sudden insight” that is a sharp discontinuity during learning, when the mice figured out to take a long but direct path with more than 6 correct binary choices to the goal. This sudden improvement in task performance persisted for the remainder of the experiment ( Rosenberg et al., 2021 ). Rosenberg et al. (2021) developed a “sudden insight statistics” procedure with which the slope of an individual learning curve can be analyzed in a way that enables the distinction between individuals that show a discontinuous learning curve from those that show continuous or incremental learning. This work demonstrates that the insight phenomenon can be quantified at the level of individual behavior and statistically analyzed (see also Reddy, 2022 ).
In conclusion, insight and associated discontinuities in learning or problem solving behavior can be regarded as the successful endpoint, respectively, outcome of conscious cognitive information processing, that might be used to time tag conscious cognitive processing and to investigate the underlying neurophysiological processes and substrates. This is especially relevant for experiments where learning behavior and electrophysiological readouts are measured simultaneously and continuously. It should be noted that has also been suggested that discontinuities in learning behavior do not necessarily reflect insight-based problem solving, but might be the consequence of other cognitive processes ( Van Steenburgh et al., 2012 ). However, this uncertainty might be eliminated by demonstrating the specificity of a putative neuro- or electrophysiological readout or correlate of discontinuous learning. For example, in the context of electrophysiological measurements, one could identify neuronal oscillations of certain frequency bands and power that correlate temporally with jumps in performance and that are not found in a comparable form or intensity during continuous learning. Future research will determine whether the neurobiological basis of conscious cognitive processing can be tackled by the simultaneous measurement of insight-like discontinuities in learning performance and in-vivo electrophysiological recordings and/or optogenetic manipulations with high temporal resolution.
The neurophysiological basis of discontinuous learning based on sudden insight
In a visual face and place recognition experiment with non-human primates, it has been found that discontinuous learning performance or sudden steps of knowledge coincides with a transient peak in neuronal network synchronization between the reward-sensitive areas of the prefrontal cortex and inferotemporal cortex known for the processing of images ( Csorba et al., 2022 ). However, the authors also reported that the amplitude of cross-region synchronization increased gradually across task performance, so that the maximal synchronization was build up slowly and not suddenly. These findings suggest that discontinuous or step-like learning performance based on insight might require the synchronization of neuronal activity in the brain areas that are involved in the processing of task-relevant information. Given that the synchronization of cross-regional neuronal activity was build up gradually, it is tempting to speculate whether conscious cognitive information processing that eventually leads to an sudden improvement in task performance is reflected by a gradual and cumulative increase in the synchronization of neuronal activity in the brain regions involved in the mental representation, maintenance and processing of task-relevant information.
Further evidence for the involvement of the prefrontal cortex in discontinuous learning performance that might be based on an insight-like decision process comes from a rule-shifting task with rats ( Durstewitz et al., 2010 ). In this experiment, rats were first trained to acquire a cue-based response strategy to obtain rewards in an operant chamber. Thereafter, the rats experienced a change in the reinforcement contingencies that required them to abandon the old strategy and gradually acquire a different egocentric response strategy by gathering evidence through trial-and-error. The authors suggested that novel rule learning can be regarded as an evidence-based decision process, that might be accompanied by moments of sudden insight at the instant, when there is enough information gathered that undoubtedly indicates that the reinforcement contingencies in the learning situation have changed permanently (a similar interpretation can be found in Bowden et al., 2005 ). It was found that transitions in behavioral performance of rats during rule learning in a set-shifting task were temporally correlated with abrupt transitions in the firing activity of “rule-selective” neural ensembles in the prefrontal cortex ( Durstewitz et al., 2010 ). This study suggests that behavioral and neural dynamics can be correlated in gradual or incremental learning situations, where an inefficient response gradually decreases, while an efficient response gradually increases. However, it remains to be determined whether the detected correlation between behavioral and neural dynamics in this experiment indeed reflects an insight-like cognitive process, or whether it is the manifestation of the execution of different responses, respectively the active inhibition of the inefficient response. It should be also considered that, one important criterion for the appreciation that a change in behavior occurred after insight, is that the performance leap is permanent, illustrated by a step-like learning curve (without a transition phase), which was probably not the case in the study by Durstewitz et al. (2010) .
A combined scalp electroencephalogram and functional magnetic resonance imaging study by Jung-Beeman and colleagues searched for the neural correlates of insight-like verbal problem solving. In this experiment, participants had to state whether the correct solutions were reached by insight or not. Insight-based solutions were found to be preceded (0.3 s prior to insight solutions) by a sudden burst of neural oscillations in the gamma-frequency range in the anterior superior temporal gyrus of the right hemisphere. Insight solutions also associated with increased neuronal activation in the anterior superior temporal gyrus as compared to non-insight solutions ( Jung-Beeman et al., 2004 ).
Further support for the implication of gamma-frequency neuronal oscillations in insight-like problem solving was provided by an electroencephalogram study by Rosen and Reiner (2017) . Here, participants were asked to solve a spatial puzzle (which can be solved either incrementally or by insight), and had to indicate whether they found the solution by sudden insight or in another way. Participants who have reported solutions based on insight showed an increase in gamma and beta frequency activity in frontal areas and with respect to alpha frequency activity in right temporal areas as compared to participants who reported an solution based on incremental learning ( Rosen and Reiner, 2017 ). Interestingly, the incremental group exhibited a decrease in gamma and beta activity during the task performance as compared to baseline recordings, suggesting that different solution strategies are mediated by different neuronal network operations rather than on the basis of a gradual difference. The latter alternative would mean that both an insight-based solution and a solution based on incremental learning would require gamma activity, but an insightful solution would be of example only possible if a certain intensity or power threshold is exceeded. In conclusion, these findings suggest that the assumed reorganization of information and the restructuration of the spatial puzzle problem prior to the insight-based solution was associated with gamma frequency neural oscillations in the right frontal cortex.
Another electroencephalographic study searched for neural activity that might be specific for different phases of insight-based problem solving, including a mental impasse (when it is realized that routine solutions are useless), the reorganization of the relevant information to have a different access to the problem, and, finally the sudden insight into the problem and its solution based on subjective ratings ( Sandkühler and Bhattacharya, 2008 ). According to the authors the states of mental impasse or sudden insight were correlated with the power of gamma-frequency activity at parietal-occipital regions, while the reorganization of task-relevant information seemed to be associated with a decreased power in the alpha-frequency range in the right prefrontal area ( Sandkühler and Bhattacharya, 2008 ).
In the studies reported above, the categorization of insight vs. non-insight solutions has been made by subjective or introspective reports requiring metacognition (hereinafter referred to as insight-like problem solving), rather than on the basis of more objective criterions, such as discontinuous learning performance as suggested above. Therefore, it remains to be determined whether the findings reported above can be replicated when a behavioral criterion for insight-like problem solving is used as a specifier for problem solving based on insight.
The studies discussed so far suggest an involvement of cortical brain regions in insight-like problem solving (prefrontal cortex, anterior superior temporal gyrus, parieto-occipital regions showing neural activity in the gamma-frequency range). However, there is also evidence for a subcortical contribution to insight-like problem solving. In an functional magnetic resonance experiment, participants signaled correct solutions in a remote associates task (inferring the commonality in word triplets) with a button press, which has been used as a time-tag for sudden insight-like solutions ( Tik et al., 2018 ). Insight-like solutions were not only accompanied by neuronal activation in the left anterior middle temporal gyrus, but also by bilateral activations in the thalamus, hippocampus, ventral tegmental area, nucleus accumbens, and caudate nucleus. The authors conclude that the subjective feeling of relief and the emotional arousal induced after the sudden emergence of the solution is accompanied by a reward signal in the mesolimbic dopamine system ( Tik et al., 2018 ). It would be interesting to know, whether insight-based solutions of more complex problem configurations (e.g., after conscious cognitive information processing) would likewise be accompanied by a subcortical dopaminergic reward signal. Such a reward signal is normally associated with strong emotional arousal, that is known to be required to trigger episodic memory formation for the preservation of the correct solution ( Dere et al., 2006 , 2008 , 2010 ; Pause et al., 2013 ). This episodic memory for the problem solving event is very likely the mnemonic basis for the persistent improvement in task performance that follows after problem solving based on insight.
The role of gap junction channels and hemichannels for discontinuous learning based on insight
Electrophysiological evidence suggests that the reorganization and restructuration processes in working memory, that precede insight-based problem solutions are accompanied by an increase in the power of gamma oscillations in cortical areas including the prefrontal cortex. In the following, I will review evidence for the involvement of gap junction channels and connexin hemichannels in cortical gamma-oscillations ( Cunningham et al., 2004 ; Traub and Whittington, 2010 , 2022 ; Traub et al., 2020 ) and working memory processes.
Gap junction channels composed of connexin proteins allow an intercellular coupling between neighboring cells. The connexins Cx36 and Cx45 are expressed in neurons, while Cx43 and Cx30 are expressed in astrocytes (see Dere, 2013 for an overview). Intercellular electrotonic and metabolic coupling allows the bidirectional diffusion of ions, cations, metabolites, second messengers, cyclic nucleotides, oligonucleotides, and small molecules with a molecular mass up to 1–2 kDa ( Dere, 2013 ). Coupling and uncoupling of adjacent cells via gap junctions is activity-dependent, shows plasticity similar to the modulation of synaptic strength, and is not restricted to cells of the same type ( Yang et al., 1990 ; Landisman et al., 2002 ; Alev et al., 2008 ). In this way, neuronal depolarization can theoretically directly propagate across neurons and generate action potentials. Intercellular communication and signal transmission via gap junctions operates almost without a temporal delay and is therefore much faster as compared to synaptic neurotransmission that operates at least in the order of milliseconds ( Dere and Zlomuzica, 2012 ; Dere, 2013 ).
Connexin hemichannels and gap junctions have been shown to shape neuronal network oscillations throughout the brain ( Coulon and Landisman, 2017 ). These network oscillations appear to be critically involved in working memory, cognition, and behavior ( Allen et al., 2011 ; Maciunas et al., 2016 ; Walrave et al., 2016 ; Tao et al., 2021 ; Linsambarth et al., 2022 ). There is evidence that theta and gamma oscillations in hippocampal neuronal networks are mediated by gap junction channels composed of Cx36, Cx45, or both ( Hormuzdi et al., 2001 ; Schmitz et al., 2001 ; Meier et al., 2002 ; Buhl et al., 2003 ; Zlomuzica et al., 2010 ). Electrophysiological studies with Cx36 deficient mice revealed slower hippocampal theta oscillations ( Allen et al., 2011 ), reduced overall power, and synchrony of hippocampal gamma oscillations in vitro ( Hormuzdi et al., 2001 ), and in vivo ( Buhl et al., 2003 ). Furthermore, the administration of gap junction blockers induced a complete suppression of hippocampal gamma oscillations in Cx36-deficient mice ( Buhl et al., 2003 ). Furthermore, Cx45-deficent mice showed changes in kainite-induced gamma oscillation in the CA1 and CA3 region together with impaired performance in an object recognition task ( Zlomuzica et al., 2010 ).
The behavioral phenotyping mCx36 deficient mice in our lab revealed changes in motor coordination and balancing performance, increased locomotion and running speed in a novel environment, changes in Y-maze exploration, increased anxiety-related behavior; changes in novel object exploration and impaired delay-dependent object and object-place recognition ( Frisch et al., 2005 ; Zlomuzica et al., 2012 ). The behavioral phenotypes of Cx36 and Cx45 deficient mice suggest altered learning and memory performance (including behavioral and emotional habituation to normal environments in Cx36-deficient mice) that might be related to changes in theta and gamma oscillations and conscious cognitive information processing.
Given that intercellular communication via gap junctions shows activity-dependent plasticity and might contribute to contribute to the formation of functional cell assemblies ( Traub et al., 2020 ), it would be extremely interesting to find a way that would allow the monitoring of the cellular coupling/uncoupling status of cells in the prefrontal cortex, before and after behavioral discontinuities during learning performance and problem solving. It is tempting to speculate that the reorganization of information and restructuration of the problem faced, might be associated with the sudden coupling or uncoupling of neuronal circuits and/or networks via gap junctions that ultimately leads out of an impasse or cognitive dead end and towards a sudden insight.
The platform theory of conscious cognitive information processing holds that the conscious experience of a mental representation requires working memory. The neuronal mechanism underlying working memory is thought to be a sustained reverberatory neuronal activity in the neural circuit that contains the mental representation. This maintained mental representation can then be used and manipulated by the central executive/online platform ( Dere et al., 2021 ). The platform theory of conscious cognitive information processing further proposes that the reverberation in the neuronal circuits could be maintained by the help of gap junction channels between neurons, astrocytes, as well as undocked connexin hemichannels ( Rash et al., 2001 ; Nagy et al., 2004 ; Dere et al., 2021 ). Successive neurons in the neural circuit can be either directly coupled via gap junctions or might be both coupled to the same astrocyte. Continuous reverberation and sustained excitability in neuronal circuits depends on the fast redistribution of ions and metabolites between the cytoplasm and extracellular space. These adjustments might be ensured by connexin hemichannels ( Dere et al., 2021 ). Connexin hemichannels are undocked connexin channels in the plasma membrane that are involved in paracrine communication. They provide a conduit between the intracellular and extracellular space, allowing the passage and exchange of ions and metabolites between the cytosol and extracellular milieu ( Dere and Zlomuzica, 2012 ; Dere, 2013 ). Therefore, gap junctions and connexin hemichannels have biophysical characteristics that are well suited to support working memory through fast and continuous propagation of neuronal depolarization between neurons in the neuronal circuit (probably mediated via gap junction channels with astrocytes) and via the preservation of excitability of the individual neurons for prolonged periods through the regulation of intra and extracellular ion homeostasis (ensured by the unopposed hemichannels; Dere et al., 2021 ).
The empirical evidence and theories presented so far can be summarized into 10 basic assumptions which might be helpful for future research into the neurophysiological mechanisms of conscious cognitive information processing.
1. Insight is the endpoint of conscious cognitive information processing, and, at the behavioral level, leads to a sudden step-like discontinuity in learning or problem solving performance.
2. Insight is based on a creative reorganization of mental representations of task-relevant information and the restructuration of the problem to overcome an impasse.
3. The reorganization and restructuration process requires the maintenance of task-relevant information in working memory and the operation of executive functions on these mental representations (ensured the central executive on the online platform).
4. The reorganization and restructuration processes, which precede sudden insight are correlated with an increase in the power of gamma oscillations in the prefrontal cortex, anterior superior temporal gyrus, parieto-occipital regions.
5. Gap junctions and connexin hemichannels have been implicated in cortical network oscillations and might be involved in the oscillation changes that are associated with conscious cognitive processing and insight.
6. On a subjective level, insight is perceived as a sudden comprehension, realization, or creative problem solution.
7. The subjective feeling of relief and the emotional arousal experienced by the sudden emergence of the solution might be accompanied by a reward signal in the mesolimbic dopamine system.
8. Insight is associated with strong emotional arousal that triggers episodic memory formation creating a memory for the insight event that includes context and content (solution path) information.
9. The generated insight memory is the basis of the persistent improvement in task or problem performance that is recollected whenever the same or similar problems are encountered.
10. Discontinuities in learning or problem solving performance might be used as time-tags to investigate the implication of gap junction channels and hemichannels in conscious cognitive processing.
Data availability statement
The original contributions presented in the study are included in the article/supplementary material, further inquiries can be directed to the corresponding author.
Author contributions
ED: Conceptualization, Methodology, Writing – original draft, Writing – review & editing.
The author(s) declare that no financial support was received for the research, authorship, and/or publication of this article.
Conflict of interest
The author declares that the research was conducted in the absence of any commercial or financial relationships that could be construed as a potential conflict of interest.
The author(s) declared that they were an editorial board member of Frontiers, at the time of submission. This had no impact on the peer review process and the final decision.
Publisher’s note
All claims expressed in this article are solely those of the authors and do not necessarily represent those of their affiliated organizations, or those of the publisher, the editors and the reviewers. Any product that may be evaluated in this article, or claim that may be made by its manufacturer, is not guaranteed or endorsed by the publisher.
Alev, C., Urschel, S., Sonntag, S., Zoidl, G., Fort, A. G., Höher, T., et al. (2008). The neuronal connexin-36 interacts with and is phosphorylated by CaMKII in a way similar to CaMKII interaction with glutamate receptors. Proc. Natl. Acad. Sci. USA 105, 20964–20969. doi: 10.1073/pnas.0805408105
PubMed Abstract | Crossref Full Text | Google Scholar
Alkire, M. T., Hudetz, A. G., and Tononi, G. (2008). Consciousness and anesthesia. Science 322, 876–880. doi: 10.1126/science.1149213
Allen, K., Fuchs, E. C., Jaschonek, H., Bannerman, D. M., and Monyer, H. (2011). Gap junctions between interneurons are required for normal spatial coding in the hippocampus and short-term spatial memory. J. Neurosci. 31, 6542–6552. doi: 10.1523/JNEUROSCI.6512-10.2011
Baars, B. J., Geld, N., and Kozma, R. (2021). Global workspace theory (GWT) and prefrontal cortex: recent developments. Front. Psychol. 12:749868. doi: 10.3389/fpsyg.2021.749868
Crossref Full Text | Google Scholar
Bird, C. D., and Emery, N. J. (2009). Insightful problem solving and creative tool modification by captive nontool-using rooks. Proc. Natl. Acad. Sci. USA 106, 10370–10375. doi: 10.1073/pnas.0901008106
Bob, P., Pec, O., Mishara, A. L., Touskova, T., and Lysaker, P. H. (2016). Conscious brain, metacognition and schizophrenia. Int. J. Psychophysiol. 105, 1–8. doi: 10.1016/j.ijpsycho.2016.05.003
Bowden, E. M., Jung-Beeman, M., Fleck, J., and Kounios, J. (2005). New approaches to demystifying insight. Trends Cogn. Sci. 9, 322–328. doi: 10.1016/j.tics.2005.05.012
Breeden, P., Dere, D., Zlomuzica, A., and Dere, E. (2016). The mental time travel continuum: on the architecture, capacity, versatility and extension of the mental bridge into the past and future. Rev. Neurosci. 27, 421–434. doi: 10.1515/revneuro-2015-0053
Buhl, D. L., Harris, K. D., Hormuzdi, S. G., Monyer, H., and Buzsáki, G. (2003). Selective impairment of hippocampal gamma oscillations in connexin-36 knock-out mouse in vivo. J. Neurosci. 23, 1013–1018. doi: 10.1523/JNEUROSCI.23-03-01013.2003
Chenot, Q., Hamery, C., Truninger, M., Langer, N., De Boissezon, X., and Scannella, S. (2024). Investigating the relationship between resting-state EEG microstates and executive functions: a null finding. Cortex 178, 1–17. doi: 10.1016/j.cortex.2024.05.019
Coulon, P., and Landisman, C. E. (2017). The potential role of gap junctional plasticity in the regulation of state. Neuron 93, 1275–1295. doi: 10.1016/j.neuron.2017.02.041
Csorba, B. A., Krause, M. R., Zanos, T. P., and Pack, C. C. (2022). Long-range cortical synchronization supports abrupt visual learning. Curr. Biol. 32, 2467–2479.e4. doi: 10.1016/j.cub.2022.04.029
Cunningham, M. O., Whittington, M. A., Bibbig, A., Roopun, A., LeBeau, F. E. N., Vogt, A., et al. (2004). A role for fast rhythmic bursting neurons in cortical gamma oscillations in vitro. Proc. Natl. Acad. Sci. USA 101, 7152–7157. doi: 10.1073/pnas.0402060101
Cutting, N., Apperly, I. A., Chappell, J., and Beck, S. R. (2014). The puzzling difficulty of tool innovation: why can’t children piece their knowledge together? J. Exp. Child Psychol. 125, 110–117. doi: 10.1016/j.jecp.2013.11.010
Defeyter, M. A., and German, T. P. (2003). Acquiring an understanding of design: evidence from children's insight problem solving. Cognition 89, 133–155. doi: 10.1016/S0010-0277(03)00098-2
Dere, E. (2013). Gap junctions in the brain: physiological and pathological roles . San Diego, CA: Academic Press.
Google Scholar
Dere, E., Kart-Teke, E., Huston, J. P., and De Souza Silva, M. A. (2006). The case for episodic memory in animals. Neurosci. Biobehav. Rev. 30, 1206–1224. doi: 10.1016/j.neubiorev.2006.09.005
Dere, E., Pause, B., and Pietrowsky, R. (2010). Emotion and episodic memory in neuropsychiatric disorders. Behav. Brain Res. 215, 162–171. doi: 10.1016/j.bbr.2010.03.017
Dere, E., and Zlomuzica, A. (2012). The role of gap junctions in the brain in health and disease. Neurosci. Biobehav. Rev. 36, 206–217. doi: 10.1016/j.neubiorev.2011.05.015
Dere, E., and Zlomuzica, A. (2023). Editorial: special issue on the neuroscience of consciousness. Behav. Brain Res. 437:114166. doi: 10.1016/j.bbr.2022.114166
Dere, D., Zlomuzica, A., and Dere, E. (2019). Fellow travellers in cognitive evolution: co-evolution of working memory and mental time travel? Neurosci. Biobehav. Rev. 105, 94–105. doi: 10.1016/j.neubiorev.2019.07.016
Dere, D., Zlomuzica, A., and Dere, E. (2021). Channels to consciousness: a possible role of gap junctions in consciousness. Rev. Neurosci. 32, 101–129. doi: 10.1515/revneuro-2020-0012
Dere, E., Zlomuzica, A., Huston, J. P., and De Souza Silva, M. A. (2008). “Chapter 2.2: animal episodic memory” in Handbook of episodic memory . eds. E. Dere, A. Easton, L. Nadel, and J. P. Huston, vol. 18 (Amsterdam: Elsevier Science), 155–184.
Draguhn, A., Traub, R. D., Schmitz, D., and Jefferys, J. G. (1998). Electrical coupling underlies high-frequency oscillations in the hippocampus in vitro. Nature 394, 189–192. doi: 10.1038/28184
Durstewitz, D., Vittoz, N. M., Floresco, S. B., and Seamans, J. K. (2010). Abrupt transitions between prefrontal neural ensemble states accompany behavioral transitions during rule learning. Neuron 66, 438–448. doi: 10.1016/j.neuron.2010.03.029
Ebel, S., Völter, C., and Call, J. (2020). Prior experience mediates the usage of food items as tools in great apes (pan paniscus, pan troglodytes, Gorilla gorilla, and Pongo abelii ). J. Comp. Psychol. 135, 64–73. doi: 10.1037/com0000236
Edlow, B. L., Claassen, J., Schiff, N. D., and Greer, D. M. (2021). Recovery from disorders of consciousness: mechanisms, prognosis and emerging therapies. Nat. Rev. Neurol. 17, 135–156. doi: 10.1038/s41582-020-00428-x
Frisch, C., De Souza-Silva, M. A., Söhl, G., Güldenagel, M., Willecke, K., Huston, J. P., et al. (2005). Stimulus complexity dependent memory impairment and changes in motor performance after deletion of the neuronal gap junction protein connexin36 in mice. Behav. Brain Res. 157, 177–185. doi: 10.1016/j.bbr.2004.06.023
Gallistel, C. R., Fairhurst, S., and Balsam, P. (2004). The learning curve: implications of a quantitative analysis. Proc. Natl. Acad. Sci. USA 101, 13124–13131. doi: 10.1073/pnas.0404965101
Hebb, D. O. (1949). The organization of behaviour: A neuropsychological theory . New York: Wiley.
Helson, H. (1927). Insight in the white rat. J. Exp. Psychol. 10, 378–396. doi: 10.1037/h0070577
Hormuzdi, S. G., Pais, I., LeBeau, F. E., Towers, S. K., Rozov, A., Buhl, E. H., et al. (2001). Impaired electrical signaling disrupts gamma frequency oscillations in connexin 36-deficient mice. Neuron 31, 487–495. doi: 10.1016/S0896-6273(01)00387-7
Jung-Beeman, M., Bowden, E. M., Haberman, J., Frymiare, J. L., Arambel-Liu, S., Greenblatt, R., et al. (2004). Neural activity when people solve verbal problems with insight. PLoS Biol. 2:E97. doi: 10.1371/journal.pbio.0020097
Kinugawa, K., Schumm, S., Pollina, M., Depre, M., Jungbluth, C., Doulazmi, M., et al. (2013). Aging-related episodic memory decline: are emotions the key? Front. Behav. Neurosci. 7:2. doi: 10.3389/fnbeh.2013.00002
Knoblich, G., Ohlsson, S., and Raney, G. E. (2001). An eye movement study of insight problem solving. Mem. Cogn. 29, 1000–1009. doi: 10.3758/BF03195762
Koch, C., Massimini, M., Boly, M., and Tononi, G. (2016). Neural correlates of consciousness: progress and problems. Nat. Rev. Neurosci. 17, 307–321. doi: 10.1038/nrn.2016.22
Kocsis, B., Martínez-Bellver, S., Fiáth, R., Domonkos, A., Sviatkó, K., Schlingloff, D., et al. (2022). Huygens synchronization of medial septal pacemaker neurons generates hippocampal theta oscillation. Cell Rep. 40:111149. doi: 10.1016/j.celrep.2022.111149
Köhler, W. (1917). Intelligenzprüfungen an Anthropoiden . Berlin: Royal Prussian Society of Sciences.
Köhler, W. (1925). The mentality of apes . Oxford, England: Harcourt, Brace.
Kounios, J., and Beeman, M. (2014). The cognitive neuroscience of insight. Annu. Rev. Psychol. 65, 71–93. doi: 10.1146/annurev-psych-010213-115154
Landisman, C. E., Long, M. A., Beierlein, M., Deans, M. R., Paul, D. L., and Connors, B. W. (2002). Electrical synapses in the thalamic reticular nucleus. J. Neurosci. 22, 1002–1009. doi: 10.1523/JNEUROSCI.22-03-01002.2002
Laumer, I. B., Call, J., Bugnyar, T., and Auersperg, A. M. I. (2018). Spontaneous innovation of hook-bending and unbending in orangutans ( Pongo abelii ). Sci. Rep. 8:16518. doi: 10.1038/s41598-018-34607-0
Laureys, S., Celesia, G. G., Cohadon, F., Lavrijsen, J., León-Carrión, J., Sannita, W. G., et al. (2010). European task force on disorders of consciousness. Unresponsive wakefulness syndrome: a new name for the vegetative state or apallic syndrome. BMC Med. 8:68. doi: 10.1186/1741-7015-8-68
Le Bon-Jego, M., and Yuste, R. (2007). Persistently active, pacemaker-like neurons in neocortex. Front. Neurosci. 1, 123–129. doi: 10.3389/neuro.01.1.1.009.2007
Li, H., Zhang, X., Sun, X., Dong, L., Lu, H., Yue, S., et al. (2023). Functional networks in prolonged disorders of consciousness. Front. Neurosci. 17:1113695. doi: 10.3389/fnins.2023.1113695
Linsambarth, S., Carvajal, F. J., Moraga-Amaro, R., Mendez, L., Tamburini, G., Jimenez, I., et al. (2022). Astroglial gliotransmitters released via Cx43 hemichannels regulate NMDAR-dependent transmission and short-term fear memory in the basolateral amygdala. FASEB J. 36:e22134. doi: 10.1096/fj.202100798RR
MacGregor, J. N., Ormerod, T. C., and Chronicle, E. P. (2001). Information processing and insight: a process model of performance on the nine-dot and related problems. J. Exp. Psychol. Learn. Mem. Cogn. 27, 176–201. doi: 10.1037/0278-7393.27.1.176
Maciunas, K., Snipas, M., Paulauskas, N., and Bukauskas, F. F. (2016). Reverberation of excitation in neuronal networks interconnected through voltage-gated gap junction channels. J. Gen. Physiol. 147, 273–288. doi: 10.1085/jgp.201511488
Mäkinen, M. E., Ylä-Outinen, L., and Narkilahti, S. (2018). GABA and gap junctions in the development of synchronized activity in human pluripotent stem cell-derived neural networks. Front. Cell. Neurosci. 12:56. doi: 10.3389/fncel.2018.00056
Martin, S. (2023). Why using “consciousness” in psychotherapy? Insight, metacognition and self-consciousness. New Ideas Psychol. 70:101015. doi: 10.1016/j.newideapsych.2023.101015
McCaffrey, T. (2012). Innovation relies on the obscure: a key to overcoming the classic problem of functional fixedness. Psychol. Sci. 23, 215–218. doi: 10.1177/0956797611429580
Meador, K. J., Ray, P. G., Echauz, J. R., Loring, D. W., and Vachtsevanos, G. J. (2002). Gamma coherence and conscious perception. Neurology 59, 847–854. doi: 10.1212/WNL.59.6.847
Meier, C., Petrasch-Parwez, E., Habbes, H. W., Teubner, B., Güldenagel, M., Degen, J., et al. (2002). Immunohistochemical detection of the neuronal connexin36 in the mouse central nervous system in comparison to connexin36-deficient tissues. Histochem. Cell Biol. 117, 461–471. doi: 10.1007/s00418-002-0417-z
Nagy, J. I., Dudek, F. E., and Rash, J. E. (2004). Update on connexins and gap junctions in neurons and glia in the mammalian nervous system. Brain Res. Brain Res. Rev. 47, 191–215. doi: 10.1016/j.brainresrev.2004.05.005
Northoff, G. (2017). "paradox of slow frequencies"—are slow frequencies in upper cortical layers a neural predisposition of the level/state of consciousness (NPC)? Conscious. Cogn. 54, 20–35. doi: 10.1016/j.concog.2017.03.006
Panagiotaropoulos, T. I., Deco, G., Kapoor, V., and Logothetis, N. K. (2012). Neuronal discharges and gamma oscillations explicitly reflect visual consciousness in the lateral prefrontal cortex. Neuron 74, 924–935. doi: 10.1016/j.neuron.2012.04.013
Panda, R., Thibaut, A., Lopez-Gonzalez, A., Escrichs, A., Bahri, M. A., Hillebrand, A., et al. (2022). Disruption in structural-functional network repertoire and time-resolved subcortical fronto-temporoparietal connectivity in disorders of consciousness. eLife 11:e77462. doi: 10.7554/eLife.77462
Pause, B. M., Zlomuzica, A., Kinugawa, K., Mariani, J., Pietrowsky, R., and Dere, E. (2013). Perspectives on episodic-like and episodic memory. Front. Behav. Neurosci. 7:33. doi: 10.3389/fnbeh.2013.00033
Qiu, J., Li, H., Yang, D., Luo, Y., Li, Y., Wu, Z., et al. (2008). The neural basis of insight problem solving: an event-related potential study. Brain Cogn. 68, 100–106. doi: 10.1016/j.bandc.2008.03.004
Rash, J. E., Yasumura, T., Dudek, F. E., and Nagy, J. I. (2001). Cell-specific expression of connexins and evidence of restricted gap junctional coupling between glial cells and between neurons. J. Neurosci. 21, 1983–2000. doi: 10.1523/JNEUROSCI.21-06-01983.2001
Reddy, G. (2022). A reinforcement-based mechanism for discontinuous learning. Proc. Natl. Acad. Sci. USA 119:e2215352119. doi: 10.1073/pnas.2215352119
Rosen, A., and Reiner, M. (2017). Right frontal gamma and beta band enhancement while solving a spatial puzzle with insight. Int. J. Psychophysiol. 122, 50–55. doi: 10.1016/j.ijpsycho.2016.09.008
Rosenberg, M., Zhang, T., Perona, P., and Meister, M. (2021). Mice in a labyrinth show rapid learning, sudden insight, and efficient exploration. eLife 10:e66175. doi: 10.7554/eLife.66175
Sandkühler, S., and Bhattacharya, J. (2008). Deconstructing insight: EEG correlates of insightful problem solving. PLoS One 3:e1459. doi: 10.1371/journal.pone.0001459
Schmitz, D., Schuchmann, S., Fisahn, A., Draguhn, A., Buhl, E. H., Petrasch-Parwez, E., et al. (2001). Axo-axonal coupling. A novel mechanism for ultrafast neuronal communication. Neuron 31, 831–840. doi: 10.1016/S0896-6273(01)00410-X
Schultz, W., Dayan, P., and Montague, P. R. (1997). A neural substrate of prediction and reward. Science 275, 1593–1599. doi: 10.1126/science.275.5306.1593
Shen, W., Tong, Y., Yuan, Y., Zhan, H., Liu, C., Luo, J., et al. (2017). Feeling the insight: uncovering somatic markers of the “aha” experience. Appl. Psychophysiol. Biofeedback 43, 13–21. doi: 10.1007/s10484-017-9381-1
Singer, W. (1998). Consciousness and the structure of neuronal representations. Philos. Trans. R. Soc. Lond. Ser. B Biol. Sci. 353, 1829–1840. doi: 10.1098/rstb.1998.0335
Stefanelli, R. (2023). Theories of consciousness and psychiatric disorders—a comparative analysis. Neurosci. Biobehav. Rev. 152:105204. doi: 10.1016/j.neubiorev.2023.105204
Tao, X. D., Liu, Z. R., Zhang, Y. Q., and Zhang, X. H. (2021). Connexin43 hemichannels contribute to working memory and excitatory synaptic transmission of pyramidal neurons in the prefrontal cortex of rats. Life Sci. 286:120049. doi: 10.1016/j.lfs.2021.120049
Thorndike, E. L. (1911). Animal Intelligence: Experimental Studies . Lewiston, NY, United States: Macmillan Press.
Thorpe, W. H. (1956). Learning and instinct in animals . Cambridge, MA: Harvard University Press.
Tian, Y., Margulies, D. S., Breakspear, M., and Zalesky, A. (2020). Topographic organization of the human subcortex unveiled with functional connectivity gradients. Nat. Neurosci. 23, 1421–1432. doi: 10.1038/s41593-020-00711-6
Tik, M., Sladky, R., Luft, C. D. B., Willinger, D., Hoffmann, A., Banissy, M. J., et al. (2018). Ultra-high-field fMRI insights on insight: neural correlates of the Aha!-moment. Hum. Brain Mapp. 39, 3241–3252. doi: 10.1002/hbm.24073
Tolman, E. C. (1948). Cognitive maps in rats and men. Psychol. Rev. 55, 189–208. doi: 10.1037/h0061626
Tononi, G., Boly, M., Massimini, M., and Koch, C. (2016). Integrated information theory: from consciousness to its physical substrate. Nat. Rev. Neurosci. 17, 450–461. doi: 10.1038/nrn.2016.44
Traub, R. D., and Whittington, M. A. (2010). Cortical oscillations in health and disease . New York: Oxford Univ. Press.
Traub, R. D., and Whittington, M. A. (2022). Processing of cell assemblies in the lateral entorhinal cortex. Rev. Neurosci. 33, 829–847. doi: 10.1515/revneuro-2022-0011
Traub, R. D., Whittington, M. A., Gutiérrez, R., and Draguhn, A. (2018). Electrical coupling between hippocampal neurons: contrasting roles of principal cell gap junctions and interneuron gap junctions. Cell Tissue Res. 373, 671–691. doi: 10.1007/s00441-018-2881-3
Traub, R. D., Whittington, M. A., Maier, N., Schmitz, D., and Nagy, J. I. (2020). Could electrical coupling contribute to the formation of cell assemblies? Rev. Neurosci. 31, 121–141. doi: 10.1515/revneuro-2019-0059
Tseng, P., Chang, Y. T., Chang, C. F., Liang, W. K., and Juan, C. H. (2016). The critical role of phase difference in gamma oscillation within the temporoparietal network for binding visual working memory. Sci. Rep. 6:32138. doi: 10.1038/srep32138
Valencia, A. L., and Froese, T. (2020). What binds us? Inter-brain neural synchronization and its implications for theories of human consciousness. Neurosci. Conscious 2020:niaa010. doi: 10.1093/nc/niaa010
Van Steenburgh, J. J., Fleck, J. I., Beeman, M., and Kounios, J. (2012). Insight. The Oxford handbook of thinking and reasoning . United Kingdom: Oxford University Press.
Walrave, L., Vinken, M., Albertini, G., De Bundel, D., Leybaert, L., and Smolders, I. J. (2016). Inhibition of Connexin43 Hemichannels impairs spatial short-term memory without affecting spatial working memory. Front. Cell. Neurosci. 10:288. doi: 10.3389/fncel.2016.00288
Weir, A. A. S. (2002). Shaping of hooks in new Caledonian crows. Science 297:981. doi: 10.1126/science.1073433
Weisberg, R. W. (2015). Toward an integrated theory of insight in problem solving. Think. Reason. 21, 5–39. doi: 10.1080/13546783.2014.886625
Wittner, L., and Miles, R. (2007). Factors defining a pacemaker region for synchrony in the hippocampus. J. Physiol. 584, 867–883. doi: 10.1113/jphysiol.2007.138131
Yang, X. D., Korn, H., and Faber, D. S. (1990). Long-term potentiation of electrotonic coupling at mixed synapses. Nature 348, 542–545. doi: 10.1038/348542a0
Zlomuzica, A., and Dere, E. (2022). Towards an animal model of consciousness based on the platform theory. Behav. Brain Res. 419:113695. doi: 10.1016/j.bbr.2021.113695
Zlomuzica, A., Plank, L., and Dere, E. (2022). A new path to mental disorders: through gap junction channels and hemichannels. Neurosci. Biobehav. Rev. 142:104877. doi: 10.1016/j.neubiorev.2022.104877
Zlomuzica, A., Plank, L., Kodzaga, I., and Dere, E. (2023). A fatal alliance: glial connexins, myelin pathology and mental disorders. J. Psychiatr. Res. 159, 97–115. doi: 10.1016/j.jpsychires.2023.01.008
Zlomuzica, A., Reichinnek, S., Maxeiner, S., Both, M., May, E., Wörsdörfer, P., et al. (2010). Deletion of connexin45 in mouse neurons disrupts one-trial object recognition and alters kainate-induced g-oscillations in the hippocampus. Physiol. Behav. 101, 245–253. doi: 10.1016/j.physbeh.2010.05.007
Zlomuzica, A., Viggiano, D., Degen, J., Binder, S., Ruocco, L. A., Sadile, A. G., et al. (2012). Behavioral alterations and changes in Ca/calmodulin kinase II levels in the striatum of connexin36 deficient mice. Behav. Brain Res. 226, 293–300. doi: 10.1016/j.bbr.2011.08.028
Keywords: incremental learning, discontinuous performance, conscious cognitive information processing, platform theory of conscious cognitive information processing, animal consciousness, behavioral correlates of consciousness
Citation: Dere E (2024) Insights into conscious cognitive information processing. Front. Behav. Neurosci . 18:1443161. doi: 10.3389/fnbeh.2024.1443161
Received: 03 June 2024; Accepted: 15 July 2024; Published: 25 July 2024.
Reviewed by:
Copyright © 2024 Dere. This is an open-access article distributed under the terms of the Creative Commons Attribution License (CC BY) . The use, distribution or reproduction in other forums is permitted, provided the original author(s) and the copyright owner(s) are credited and that the original publication in this journal is cited, in accordance with accepted academic practice. No use, distribution or reproduction is permitted which does not comply with these terms.
*Correspondence: Ekrem Dere, [email protected] ; [email protected]
Disclaimer: All claims expressed in this article are solely those of the authors and do not necessarily represent those of their affiliated organizations, or those of the publisher, the editors and the reviewers. Any product that may be evaluated in this article or claim that may be made by its manufacturer is not guaranteed or endorsed by the publisher.

Method enables fast, accurate estimates of cardiovascular state to inform blood pressure management
If patients receiving intensive care or undergoing major surgery develop excessively high or low blood pressures, they could suffer severe organ dysfunction. It's not enough for their care team to know that pressure is abnormal. To choose the correct drug to treat the problem, doctors must know why blood pressure has changed. A new MIT study presents the mathematical framework needed to derive that crucial information accurately and in real time.
The mathematical approach, described in a recent study in IEEE Transactions on Biomedical Engineering , produces proportional estimates of the two critical factors underlying blood pressure changes: the heart's rate of blood output (cardiac output) and the arterial system's resistance to that blood flow (systemic vascular resistance). By applying the new method to previously collected data from animal models, the researchers show that their estimates, derived from minimally invasive measures of peripheral arterial blood pressure, accurately matched estimates using additional information from an invasive flow probe placed on the aorta. Moreover, the estimates accurately tracked the changes induced in the animals by the various drugs physicians use to correct aberrant blood pressure.
"Estimates of resistance and cardiac output from our approach provide information that can readily be used to guide hemodynamic management decisions in real time," the study authors wrote.
With further testing leading to regulatory approval, the authors said, the method would be applicable during heart surgeries, liver transplants, intensive care unit treatment and many other procedures affecting cardiovascular function or blood volume.
"Any patient who is having cardiac surgery could need this," said study senior author Emery N. Brown, Edward Hood Taplin Professor of Medical Engineering and Computational Neuroscience in The Picower Institute for Learning and Memory, the Institute for Medical Engineering and Science and the Department of Brain and Cognitive Sciences at MIT. Brown is also an anesthesiologist at Massachusetts General Hospital and a professor of anesthesiology at Harvard Medical School. "So might any patient undergoing a more normal surgery but who might have a compromised cardiovascular system such as ischemic heart disease. You can't have the blood pressure being all over the place."
The study's lead author is electrical engineering and computer science (EECS) graduate student Taylor Baum, who is co-supervised by Brown and Munther Dahleh, William A. Coolidge Professor in EECS.
Algorithmic advance
The idea that cardiac output and systemic resistance are the two key components of blood pressure comes from the two-element Windkessel model. The new study is not the first to use the model to estimate these components from blood pressure measurements, but previous attempts ran into a tradeoff between quick estimate updates and the accuracy of estimates; methods would either provide more erroneous estimates at every beat or more reliable estimates that are updated at minute time scales. Led by Baum, the MIT team overcame the tradeoff with a new approach of applying statistical and signal processing techniques such as "state-space" modeling.
"Our estimates, updated at every beat, are not just informed by the current beat; but they incorporate where things were in previous beats as well," Baum said. "It's that combination of past history and current observations that produces a more reliable estimate while still at a beat-by-beat time scale."
Notably, the resulting estimates of cardiac output and systemic resistance are "proportional," meaning that they are each inextricably linked in the math with another co-factor, rather than estimated on their own. But application of the new method to data collected in an older study from six animals showed that the proportional estimates from recordings using minimally invasive catheters provide comparable information for cardiovascular system management.
One key finding was that the proportional estimates made based on arterial blood pressure readings from catheters inserted in various locations away from the heart (e.g., the leg or the arm) mirrored estimates derived from more invasive catheters placed within the aorta. The significance of the finding is that a system using the new estimation method could in some cases rely on a minimally invasive catheter in various peripheral arteries, thereby avoiding the need for a riskier placement of a central artery catheter or a pulmonary artery catheter directly in the heart, the clinical gold standard for cardiovascular state estimation.
Another key finding was that when the animals received each of five drugs that doctors use to regulate either systemic vascular resistance or cardiac output, the proportional estimates tracked the resulting changes properly. The finding therefore suggests that the proportional estimates of each factor are accurately reflecting their physiological changes.
Toward the clinic
With these encouraging results, Baum and Brown said, the current method can be readily implemented in clinical settings to inform perioperative care teams about underlying causes of critical blood pressure changes. They are actively pursuing regulatory approval of use of this method in a clinical device.
Additionally, the researchers are pursuing more animal studies to validate an advanced blood pressure management approach that uses this method.
They have developed a closed-loop system, informed by this estimation framework, to precisely regulate blood pressure in an animal model. Upon completion of the animal studies, they will apply for regulatory clearance to test the system in humans.
In addition to Baum, Dahleh and Brown, the paper's other authors are Elie Adam, Christian Guay, Gabriel Schamberg, Mohammadreza Kazemi and Thomas Heldt.
The National Science Foundation, the National Institutes of Health, a Mathworks Fellowship, The Picower Institute for Learning and Memory and The JPB Foundation supported the study.
- Heart Disease
- Hypertension
- Today's Healthcare
- Blood Clots
- Personalized Medicine
- Stroke Prevention
- Wounds and Healing
- Laparoscopic surgery
- Robotic surgery
- Minimally invasive procedure
- Blood transfusion
- Blood pressure
- Mechanical ventilation
- Plastic surgery
Story Source:
Materials provided by Picower Institute at MIT . Note: Content may be edited for style and length.
Journal Reference :
- Taylor E. Baum, Elie Adam, Christian S. Guay, Gabriel Schamberg, Mohammadreza Kazemi, Thomas Heldt, Emery N. Brown. Dynamic Estimation of Cardiovascular State From Arterial Blood Pressure Recordings . IEEE Transactions on Biomedical Engineering , 2024; 1 DOI: 10.1109/TBME.2024.3408808
Cite This Page :
Explore More
- 'Dancing Molecules' Heal Cartilage Damage
- Robotics and How Flies Assess Winds
- Lampreys: 'Jaw-Dropping' Evolutionary Origin
- Making AI 1,000 Times More Energy Efficient
- Controlling Mosquitoes Through Genetic Breeding
- Hidden Elements from Ancient Alchemy Lab
- Drug Shows Promise in Clearing HIV from Brain
- Nitrogen Emissions Have a Net Cooling Effect
- New Zealand's Flightless Birds Retreating
- Ice Sheets Melt Fast, Regrow Slowly: Sponginess
Trending Topics
Strange & offbeat.
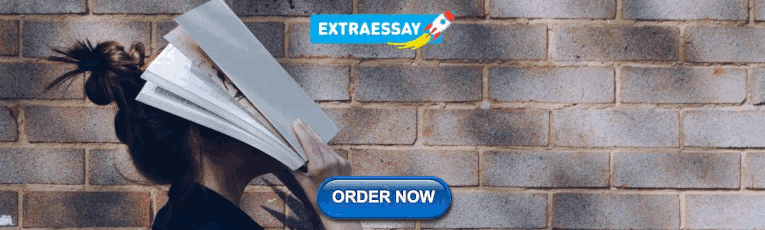
IMAGES
VIDEO
COMMENTS
Here, we consider how an animal's problem-solving ability could be impacted by its development, and what future work needs to be done to understand the development of problem solving. We argue that, considering how many different factors are involved, focusing on individual animals, and individual variation, is the best way to study the ...
Animals must be able to solve problems to access food and avoid predators. Problem solving is not a complicated process, often relying only on animals exploring their surroundings, and being able to learn and remember information. However, not all species, populations, or even individuals, can solve problems, or can solve problems in the same way.
This handbook lays out the science behind how animals think, create, calculate, and remember. It provides concise overviews on major areas of study, such as animal communication and language, memory and recall, social cognition, social learning and teaching, and numer-ical and quantitative abilities, as well as innovation and problem-solving.
The physical world poses a number of problems for animals to solve. On a daily basis, animals must find food, avoid predators, and seek shelter. Solving these problems requires cognitive capacities.
Animal Cognition: Describes the mental capacities of nonhuman animals and the study of those ... Call Josep, Tomasello Michael. Domestic dogs (Canis familiaris) coordinate their actions in a problem-solving task. Animal Cognition. 2013c; 16:273-85. doi: 10.1007/s10071-012-0571-1. [Google Scholar] Bshary Redouan, Brown Culum. Fish cognition. ...
The evolutionary origin of innovative abilities is unclear, however, because innovating implies coping with problems the animal has not experienced before. A possibility is to consider innovation as an emergent property that results from the combination of cognitive and noncognitive traits that have coevolved as part of a life-history syndrome ...
All animals solve problems and many use tools, but the underlying cognitive processes may differ. Understanding taxonomic differences and similarities in cognitive aspects of problem solving and tool use, for example, causal understanding, continues to be an important research challenge, and may provide insight into treatment of human cognitive disorders.
Abstract. Cognitive processes such as perception, learning, memory and decision making play an important role in mate choice, foraging and many other behaviours. In this review, I summarize a few key ideas about animal cognition developed in a recent book (Shettleworth 1998, Cognition, Evolution and Behaviour) and briefly review some areas in ...
The authors administered a battery of cognitive tests to 15 passerine species to show that performance in problem-solving tasks is most closely associated with innovations in the wild.
Research such as that recently conducted by Audet et al. [1] is an important contribution to the increasing interest in the application of animal cognition studies to conservation and management outcomes.This is because innovative behaviour, such as the ability to find solutions to novel problems, is important in determining which species are able to either effectively invade or persist in ...
Twenty-five years ago, Animal Cognition released its first volume. This new peer-reviewed journal sought to fill an open niche in scientific publishing for studies "investigating how animal (including human) minds function and how they evolved" (Czeschlik 1998, p.1).The inaugural editor, Tatiana Czeschlik, declared that the scope of this new journal was to publish studies that sought "to ...
Abstract. In recent years there has been a burgeoning interest in the use of cognitive concepts to account for the performances of animals in complex test situations both in the laboratory and in the field. The recent volume stemming from the Guggenheim Conference on animal cognition (1984) published within six years of the major works by O ...
Cognitive abilities (such as learning, memory, inhibitory control, problem-solving, and social cognition) are important traits in almost all aspects of an animal's life, from finding food to ...
Animal cognition encompasses the mental capacities of non-human animals including insect cognition. ... where they could only get bananas by removing a box. The chimpanzee, Köhler observed, "has special difficulty in solving such problems; he often draws into a situation the strangest and most distant tools, and adopts the most peculiar ...
Carnivores often engage in seemingly intelligent behaviors, such as the cooperative hunting of prey (18, 19).Nevertheless, with the exception of domestic dogs, carnivores have largely been ignored in the animal cognition literature ().Mammalian carnivores comprise an excellent taxon in which to assess the relationship between brain size and problem-solving ability and test predictions of ...
In contrast, humans have "floodlight" cognition, allowing us to use thought processes in new ways and to apply the solution of one problem to another situation. While animals can transfer across ...
Animals learn a great deal of valuable information from conspecifics in the wild. Wild animals, especially birds and primates exhibit an impressive array of cognitive abilities: tool use, spatial learning, memory, discrimination, observational and social learning, imitation, and cultural transmission.
Such opportunities for innovative problem-solving, alongside other challenges necessitating flexibility in behaviour, have led to the popular notion that urban environments favour species and individuals that are 'buffered' by superior cognitive abilities [10,11], perhaps even leading to a cognitive arms race between people and animals ...
Tiny clumps of cells show basic cognitive abilities, and some animals can remember things after losing their head. ... Such problem-solving could be dismissed as simple algorithms, but other ...
A natural approach of animal creativity through insightful problem-solving may offer a panel of how physiological, contextual, cultural and developmental variables related to each other to produce new behaviors. The spontaneous interconnection of acquire behaviors is an Insightful Problem-Solving model based on the new combination and/or chaining of behaviors that were previously and ...
5 Comparative cognition: reasoning and problem-solving. Current section: 5.1 Animal problem-solving: using tools. 5.2 Cooperating elephants. 5.3 New Caledonian crows. 5.4 Collaborative problem-solving: the role of emotions. ... 5.1 Animal problem-solving: using tools. From the earliest, most primitive stick or piece of rock, to the most ...
Traditionally, conservation and cognition have been disparate research disciplines. However, Audet et al.'s recent research contributes to an increasing body of evidence that innovative behaviours may determine the ability of species to respond to rapid environmental change, identifying an opportunity for cognition research to directly contribute to conservation outcomes.
Abstract. Brain size predicts problem-solving ability in mammalian carnivores. Despite considerable interest in the forces shaping the relationship between brain size and cognitive abilities, it remains controversial whether larger-brained animals are, indeed, better problem-solvers.
One is the ability to form nested scenarios, an inner theater of the mind that allows us to envision and mentally manipulate many possible situations and anticipate different outcomes. The second ...
In conclusion, insight and associated discontinuities in learning or problem solving behavior can be regarded as the successful endpoint, respectively, outcome of conscious cognitive information processing, that might be used to time tag conscious cognitive processing and to investigate the underlying neurophysiological processes and substrates.
A new mathematical method, validated with experimental animal data, provides a fast, reliable and minimally invasive way of determining how to treat critical blood pressure changes during surgery ...