Click through the PLOS taxonomy to find articles in your field.
For more information about PLOS Subject Areas, click here .
Loading metrics
Open Access
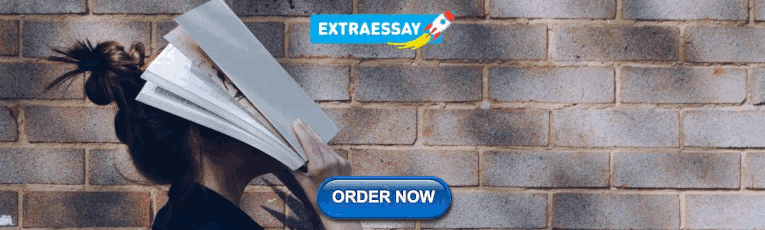
Ethical and Scientific Considerations Regarding Animal Testing and Research
* E-mail: [email protected]
Affiliations Physicians Committee for Responsible Medicine, Washington, D.C., United States of America, Department of Medicine, The George Washington University, Washington, D.C., United States of America
Affiliation Physicians Committee for Responsible Medicine, Washington, D.C., United States of America
- Hope R. Ferdowsian,
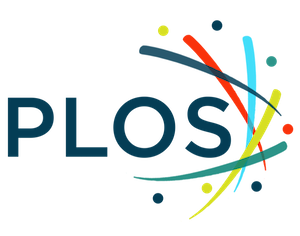
Published: September 7, 2011
- https://doi.org/10.1371/journal.pone.0024059
- Reader Comments
Citation: Ferdowsian HR, Beck N (2011) Ethical and Scientific Considerations Regarding Animal Testing and Research. PLoS ONE 6(9): e24059. https://doi.org/10.1371/journal.pone.0024059
Editor: Catriona J. MacCallum, Public Library of Science, United Kingdom
Copyright: © 2011 Ferdowsian, Beck. This is an open-access article distributed under the terms of the Creative Commons Attribution License, which permits unrestricted use, distribution, and reproduction in any medium, provided the original author and source are credited.
Funding: The authors are grateful to the National Science Foundation (grant SES-0957163) and the Arcus Foundation (grant 0902-34) for the financial support for the corresponding conference, Animals, Research, and Alternatives: Measuring Progress 50 Years Later. The funders had no role in study design, data collection and analysis, decision to publish, or preparation of the manuscript.
Competing interests: HRF and NB are employed by Physicians Committee for Responsible Medicine, which is a non-governmental organization which promotes higher ethical standards in research and alternatives to the use of animals in research, education, and training. Physicians Committee for Responsible Medicine is a nonprofit organization, and the authors adhered to PLoS ONE policies on sharing data and materials.
In 1959, William Russell and Rex Burch published the seminal book, The Principles of Humane Experimental Technique, which emphasized r eduction, r efinement, and r eplacement of animal use, principles which have since been referred to as the “3 Rs”. These principles encouraged researchers to work to reduce the number of animals used in experiments to the minimum considered necessary, refine or limit the pain and distress to which animals are exposed, and replace the use of animals with non-animal alternatives when possible. Despite the attention brought to this issue by Russell and Burch and since, the number of animals used in research and testing has continued to increase, raising serious ethical and scientific issues. Further, while the “3 Rs” capture crucially important concepts, they do not adequately reflect the substantial developments in our new knowledge about the cognitive and emotional capabilities of animals, the individual interests of animals, or an updated understanding of potential harms associated with animal research. This Overview provides a brief summary of the ethical and scientific considerations regarding the use of animals in research and testing, and accompanies a Collection entitled Animals, Research, and Alternatives: Measuring Progress 50 Years Later , which aims to spur ethical and scientific advancement.
Introduction
One of the most influential attempts to examine and affect the use of animals in research can be traced back to1959, with the publication of The Principles of Humane Experimental Technique [1] . William Russell and Rex Burch published this seminal book in response to marked growth in medical and veterinary research and the concomitant increase in the numbers of animals used. Russell and Burch's text emphasized r eduction, r efinement, and r eplacement of animal use, principles which have since been referred to as the “3 Rs”. These principles encouraged researchers to work to reduce the number of animals used in experiments to the minimum considered necessary, refine or limit the pain and distress to which animals are exposed, and replace the use of animals with non-animal alternatives when possible.
Despite the attention brought to this issue by Russell and Burch, the number of animals used in research and testing has continued to increase. Recent estimates suggest that at least 100 million animals are used each year worldwide [2] . However, this is likely an underestimate, and it is impossible to accurately quantify the number of animals used in or for experimentation. Full reporting of all animal use is not required or made public in most countries. Nevertheless, based on available information, it is clear that the number of animals used in research has not significantly declined over the past several decades.
The “3 Rs” serve as the cornerstone for current animal research guidelines, but questions remain about the adequacy of existing guidelines and whether researchers, review boards, and funders have fully and adequately implemented the “3 Rs”. Further, while the “3 Rs” capture crucially important concepts, they do not adequately reflect the substantial developments in our new knowledge about the cognitive and emotional capabilities of animals; an updated understanding of the harms inherent in animal research; and the changing cultural perspectives about the place of animals in society [3] , [4] . In addition, serious questions have been raised about the effectiveness of animal testing and research in predicting anticipated outcomes [5] – [13] .
In August 2010, the Georgetown University Kennedy Institute of Ethics, the Johns Hopkins University Center for Alternatives to Animal Testing, the Institute for In Vitro Sciences, The George Washington University, and the Physicians Committee for Responsible Medicine jointly held a two day multi-disciplinary, international conference in Washington, DC, to address the scientific, legal, and political opportunities and challenges to implementing alternatives to animal research. This two-day symposium aimed to advance the study of the ethical and scientific issues surrounding the use of animals in testing and research, with particular emphasis on the adequacy of current protections and the promise and challenges of developing alternatives to the use of animals in basic research, pharmaceutical research and development, and regulatory toxicology. Speakers who contributed to the conference reviewed and contributed new knowledge regarding the cognitive and affective capabilities of animals, revealed through ethology, cognitive psychology, neuroscience, and related disciplines. Speakers also explored the dimensions of harm associated with animal research, touching on the ethical implications regarding the use of animals in research. Finally, several contributors presented the latest scientific advances in developing alternatives to the use of animals in pharmaceutical research and development and regulatory toxicity testing.
This Collection combines some papers that were written following this conference with an aim to highlight relevant progress and research. This Overview provides a brief summary of the ethical and scientific considerations regarding the use of animals in research and testing, some of which are highlighted in the accompanying Collection.
Analysis and Discussion
Ethical considerations and advances in the understanding of animal cognition.
Apprehension around burgeoning medical research in the late 1800s and the first half of the 20 th century sparked concerns over the use of humans and animals in research [14] , [15] . Suspicions around the use of humans were deepened with the revelation of several exploitive research projects, including a series of medical experiments on large numbers of prisoners by the Nazi German regime during World War II and the Tuskegee syphilis study. These abuses served as the impetus for the establishment of the Nuremberg Code, Declaration of Helsinki, and the National Commission for the Protection of Human Subjects of Biomedical and Behavioral Research (1974) and the resulting Belmont Report [16] – [18] . Today, these guidelines provide a platform for the protection of human research subjects, including the principles of respect, beneficence, and justice, as well as special protections for vulnerable populations.
Laws to protect animals in research have also been established. The British Parliament passed the first set of protections for animals in 1876, with the Cruelty to Animals Act [19] . Approximately ninety years later, the U.S. adopted regulations for animals used in research, with the passage of the Laboratory Animal Welfare Act of 1966 [20] . Subsequent national and international laws and guidelines have provided basic protections, but there are some significant inconsistencies among current regulations [21] . For example, the U.S. Animal Welfare Act excludes purpose-bred birds, rats, or mice, which comprise more than 90% of animals used in research [20] . In contrast, certain dogs and cats have received special attention and protections. Whereas the U.S. Animal Welfare Act excludes birds, rats and mice, the U.S. guidelines overseeing research conducted with federal funding includes protections for all vertebrates [22] , [23] . The lack of consistency is further illustrated by the “U.S. Government Principles for the Utilization and Care of Vertebrate Animals Used in Testing, Research and Training” which stress compliance with the U.S. Animal Welfare Act and “other applicable Federal laws, guidelines, and policies” [24] .
While strides have been made in the protection of both human and animal research subjects, the nature of these protections is markedly different. Human research protections emphasize specific principles aimed at protecting the interests of individuals and populations, sometimes to the detriment of the scientific question. This differs significantly from animal research guidelines, where the importance of the scientific question being researched commonly takes precedence over the interests of individual animals. Although scientists and ethicists have published numerous articles relevant to the ethics of animal research, current animal research guidelines do not articulate the rationale for the central differences between human and animal research guidelines. Currently, the majority of guidelines operate on the presumption that animal research should proceed based on broad, perceived benefits to humans. These guidelines are generally permissive of animal research independent of the costs to the individual animal as long as benefits seem achievable.
The concept of costs to individual animals can be further examined through the growing body of research on animal emotion and cognition. Studies published in the last few decades have dramatically increased our understanding of animal sentience, suggesting that animals' potential for experiencing harm is greater than has been appreciated and that current protections need to be reconsidered. It is now widely acknowledged by scientists and ethicists that animals can experience pain and distress [25] – [29] . Potential causes of harm include invasive procedures, disease, and deprivation of basic physiological needs. Other sources of harm for many animals include social deprivation and loss of the ability to fulfill natural behaviors, among other factors. Numerous studies have demonstrated that, even in response to gentle handling, animals can show marked changes in physiological and hormonal markers of stress [30] .
Although pain and suffering are subjective experiences, studies from multiple disciplines provide objective evidence of animals' abilities to experience pain. Animals demonstrate coordinated responses to pain and many emotional states that are similar to those exhibited by humans [25] , [26] . Animals share genetic, neuroanatomical, and physiological similarities with humans, and many animals express pain in ways similar to humans. Animals also share similarities with humans in genetic, developmental, and environmental risk factors for psychopathology [25] , [26] . For example, fear operates in a less organized subcortical neural circuit than pain, and it has been described in a wide variety of species [31] . More complex markers of psychological distress have also been described in animals. Varying forms of depression have been repeatedly reported in animals, including nonhuman primates, dogs, pigs, cats, birds and rodents, among others [32] – [34] . Anxiety disorders, such as post-traumatic stress disorder, have been described in animals including chimpanzees and elephants [35] , [36] , [37] .
In addition to the capacity to experience physical and psychological pain or distress, animals also display many language-like abilities, complex problem-solving skills, tool related cognition and pleasure-seeking, with empathy and self-awareness also suggested by some research. [38] – [44] . Play behavior, an indicator of pleasure, is widespread in mammals, and has also been described in birds [45] , [46] . Behavior suggestive of play has been observed in other taxa, including reptiles, fishes and cephalopods [43] . Self-awareness, assessed through mirror self-recognition, has been reported for chimpanzees and other great apes, magpies, and some cetaceans. More recent studies have shown that crows are capable of creating and using tools that require access to episodic-like memory formation and retrieval [47] . These findings suggest that crows and related species display evidence of causal reasoning, flexible learning strategies, imagination and prospection, similar to findings in great apes. These findings also challenge our assumptions about species similarities and differences and their relevance in solving ethical dilemmas regarding the use of animals in research.
Predictive Value of Animal Data and the Impact of Technical Innovations on Animal Use
In the last decade, concerns have mounted about how relevant animal experiments are to human health outcomes. Several papers have examined the concordance between animal and human data, demonstrating that findings in animals were not reliably replicated in human clinical research [5] – [13] . Recent systematic reviews of treatments for various clinical conditions demonstrated that animal studies have been poorly predictive of human outcomes in the fields of neurology and vascular disease, among others [7] , [48] . These reviews have raised questions about whether human diseases inflicted upon animals sufficiently mimic the disease processes and treatment responses seen in humans.
The value of animal use for predicting human outcomes has also been questioned in the regulatory toxicology field, which relies on a codified set of highly standardized animal experiments for assessing various types of toxicity. Despite serious shortcomings for many of these assays, most of which are 50 to 60 years old, the field has been slow to adopt newer methods. The year 2007 marked a turning point in the toxicology field, with publication of a landmark report by the U.S. National Research Council (NRC), highlighting the need to embrace in vitro and computational methods in order to obtain data that more accurately predicts toxic effects in humans. The report, “Toxicity Testing in the 21 st Century: A Vision and a Strategy,” was commissioned by the U.S. Environmental Protection Agency, partially due to the recognition of weaknesses in existing approaches to toxicity testing [49] . The NRC vision calls for a shift away from animal use in chemical testing toward computational models and high-throughput and high-content in vitro methods. The report emphasized that these methods can provide more predictive data, more quickly and affordably than traditional in vivo methods. Subsequently published articles address the implementation of this vision for improving the current system of chemical testing and assessment [50] , [51] .
While a sea change is underway in regulatory toxicology, there has been much less dialogue surrounding the replacement of animals in research, despite the fact that far more animals are used in basic and applied research than in regulatory toxicology. The use of animals in research is inherently more difficult to approach systematically because research questions are much more diverse and less proscribed than in regulatory toxicology [52] . Because researchers often use very specialized assays and systems to address their hypotheses, replacement of animals in this area is a more individualized endeavour. Researchers and oversight boards have to evaluate the relevance of the research question and whether the tools of modern molecular and cell biology, genetics, biochemistry, and computational biology can be used in lieu of animals. While none of these tools on their own are capable of replicating a whole organism, they do provide a mechanistic understanding of molecular events. It is important for researchers and reviewers to assess differences in the clinical presentation and manifestation of diseases among species, as well as anatomical, physiological, and genetic differences that could impact the transferability of findings. Another relevant consideration is how well animal data can mirror relevant epigenetic effects and human genetic variability.
Examples of existing and promising non-animal methods have been reviewed recently by Langley and colleagues, who highlighted advances in fields including orthodontics, neurology, immunology, infectious diseases, pulmonology, endocrine and metabolism, cardiology, and obstetrics [52] .
Many researchers have also begun to rely solely on human data and cell and tissue assays to address large areas of therapeutic research and development. In the area of vaccine testing and development, a surrogate in-vitro human immune system has been developed to help predict an individual's immune response to a particular drug or vaccine [53] , [54] . This system includes a blood-donor base of hundreds of individuals from diverse populations and offers many benefits, including predictive high-throughput in vitro immunology to assess novel drug and vaccine candidates, measurement of immune responses in diverse human populations, faster cycle time for discovery, better selection of drug candidates for clinical evaluation, and reductions in the time and costs to bring drugs and vaccines to the market. In the case of vaccines, this system can be used at every stage, including in vitro disease models, antigen selection and adjuvant effects, safety testing, clinical trials, manufacturing, and potency assays. When compared with data from animal experiments, this system has produced more accurate pre-clinical data.
The examples above illustrate how innovative applications of technology can generate data more meaningful to humans, and reduce or replace animal use, but advances in medicine may also require novel approaches to setting research priorities. The Dr. Susan Love Research Foundation, which focuses on eradicating breast cancer, has challenged research scientists to move from animal research to breast cancer prevention research involving women. If researchers could better understand the factors that increase the risk for breast cancer, as well as methods for effective prevention, fewer women would require treatment for breast cancer. Whereas animal research is largely investigator-initiated, this model tries to address the questions that are central to the care of women at risk for or affected by breast cancer. This approach has facilitated the recruitment of women for studies including a national project funded by the National Institutes of Health and the National Institute of Environmental Health to examine how environment and genes affect breast cancer risk. This study, which began in 2002, could not have been accomplished with animal research [55] .
Similarly, any approach that emphasizes evidence-based prevention would provide benefits to both animals and humans. Resource limitations might require a strategic approach that emphasizes diseases with the greatest public health threats, which increasingly fall within the scope of preventable diseases.
It is clear that there have been many scientific and ethical advances since the first publication of Russell and Burch's book. However, some in the scientific community are beginning to question how well data from animals translates into germane knowledge and treatment of human conditions. Efforts to objectively evaluate the value of animal research for understanding and treating human disease are particularly relevant in the modern era, considering the availability of increasingly sophisticated technologies to address research questions [9] . Ethical objections to the use of animals have been publically voiced for more than a century, well before there was a firm scientific understanding of animal emotion and cognition [15] . Now, a better understanding of animals' capacity for pain and suffering is prompting many to take a closer look at the human use of animals [56] .
Articles in the accompanying Collection only briefly touch on the many scientific and ethical issues surrounding the use of animals in testing and research. While it is important to acknowledge limitations to non-animal methods remain, recent developments demonstrate that these limitations should be viewed as rousing challenges rather than insurmountable obstacles. Although discussion of these issues can be difficult, progress is most likely to occur through an ethically consistent, evidence-based approach. This collection aims to spur further steps forward toward a more coherent ethical framework for scientific advancement.
Acknowledgments
The authors thank the conference speakers and participants for their participation.
Author Contributions
Conceived and designed the experiments: HRF NB. Contributed reagents/materials/analysis tools: HRF NB. Wrote the paper: HRF NB.
- 1. Russell WMS, Burch RL (1959) The principles of humane experimental technique. London: Methuen. 238 p.
- View Article
- Google Scholar
- 3. Ibrahim DM (2006) Reduce, refine, replace: the failure of the three R's and the future of animal experimentation. University of Chicago Legal Forum, 2006; Arizona Legal Studies Discussion Paper No. 06-17. Available: http://ssrn.com/abstract=888206 . Accessed 2011 Jan 7.
- 15. Lederer SE (1995) Subjected to science. Baltimore: The Johns Hopkins University Press. 192 p.
- 16. United States (1947) Nuremberg code. Trials of War Criminals before the Nuremberg Military Tribunals under Control Council Law No. 10. Washington, D.C.: U.S. Government Printing Office. Available: http://ohsr.od.nih.gov/guidelines/nuremberg.html . Accessed 2011 Jan 7.
- 17. World Medical Association (1964) Declaration of Helsinki. 18 th WMA General Assembly. Helsinki, Finland: Available: http://history.nih.gov/research/downloads/helsinki.pdf . Accessed 2011 Jan 7.
- 18. National Commission for the Protection of Human Subjects of Biomedical and Behavioral Research (1979) The Belmont Report. Washington, D.C.: US Department of Health, Education, and Welfare. Available: http://ohsr.od.nih.gov/guidelines/belmont.html . Accessed 2011 Jan 7.
- 19. Parliament of the United Kingdom (1876) Cruelty to Animals Act 1876. Available: http://www.ncbi.nlm.nih.gov/pmc/articles/PMC1872363/ . Accessed 2011 Jan 7.
- 20. Animal Welfare Act. 7 U.S.C. §§ 2131–2159.
- 22. Institute of Laboratory Animal Resources, Commission on Life Sciences, National Research Council (1996) Guide for the care and use of laboratory animals. Washington, D.C.: National Academy Press. 140 p.
- 23. Office of Laboratory Animal Welfare (2002) Public Health Service policy on humane care and use of laboratory animals. Available: http://grants.nih.gov/grants/olaw/references/phspol.htm#PublicHealthServicePolicyonHumaneCareandUseofLaboratory . Accessed 2011 Jan 18.
- 24. Office of Laboratory Animal Welfare (2002) U.S. Government principles for the utilization and care of vertebrate animals used in testing, research and training. Available: http://grants.nih.gov/grants/olaw/references/phspol.htm . Accessed 2011 Jan 7.
- 25. Gregory NG (2004) Physiology and behavior of animal suffering. Oxford, U.K.: Blackwell Science. 280 p.
- 26. McMillan FD, editor. (2005) Mental Health and Well-Being in Animals. Oxford, U.K.: Blackwell Publishing Professional. 301 p.
- 27. Institute of Laboratory Animal Resources, Commission on Life Sciences, National Research Council (2009) Recognition and alleviation of pain and distress in laboratory animals. Washington, D.C.: National Academy Press. 196 p.
- 31. Panksepp J (2004) Affective neuroscience: the foundations of human and animal emotions. Oxford: Oxford University Press. 480 p.
- 32. Koob GF, Ehlers CL, Kupfers DJ, editors. (1989) Animal Models of Depression. Boston, MA: Birkhäuser. 295 p.
- 39. Shettleworth SJ (1998) Cognition, evolution, and behavior. Oxford, U.K.: Oxford University Press. 704 p.
- 40. deWaal F (2009) The age of empathy: nature's lessons for a kinder society. New York, NY: Random House, Inc. 304 p.
- 44. Burghardt GM (2005) The genesis of animal play: testing the limits. Cambridge, U.K.: MIT Press. 501 p.
- 49. Committee on Toxicity Testing and Assessment of Environmental Agents, National Research Council (2007) Toxicity testing in the 21st century: a vision and a strategy. Washington, DC: National Academy Press. 216 p.
- 55. Dr. Susan Love Research Foundation, National Cancer Institute Cancer Biomedical Informatics Grid (2009) Health of Women Study. Available: http://cabig.cancer.gov/action/collaborations/howstudy/ . Accessed 2011 Jan 10.
- 56. Beauchamp TL, Orlans FB, Dresser R, Morton DB, Gluck JP (2008) The Human Use of Animals: Case Studies in Ethical Choice, 2 nd ed. New York, NY: Oxford University Press. 287 p.
Replacing Animal Testing with Stem Cell-Organoids : Advantages and Limitations
- Open access
- Published: 19 April 2024
Cite this article
You have full access to this open access article
- Guiyoung Park 1 ,
- Yeri Alice Rim 2 , 3 , 4 ,
- Yeowon Sohn 5 ,
- Yoojun Nam ORCID: orcid.org/0000-0003-4583-3455 5 , 6 &
- Ji Hyeon Ju 2 , 3 , 4 , 6
39 Accesses
Explore all metrics
Various groups including animal protection organizations, medical organizations, research centers, and even federal agencies such as the U.S. Food and Drug Administration, are working to minimize animal use in scientific experiments. This movement primarily stems from animal welfare and ethical concerns. However, recent advances in technology and new studies in medicine have contributed to an increase in animal experiments throughout the years. With the rapid increase in animal testing, concerns arise including ethical issues, high cost, complex procedures, and potential inaccuracies.
Alternative solutions have recently been investigated to address the problems of animal testing. Some of these technologies are related to stem cell technologies, such as organ-on-a-chip, organoids, and induced pluripotent stem cell models. The aim of the review is to focus on stem cell related methodologies, such as organoids, that can serve as an alternative to animal testing and discuss its advantages and limitations, alongside regulatory considerations.
Although stem cell related methodologies has shortcomings, it has potential to replace animal testing. Achieving this requires further research on stem cells, with potential societal and technological benefits.
Graphical Abstract
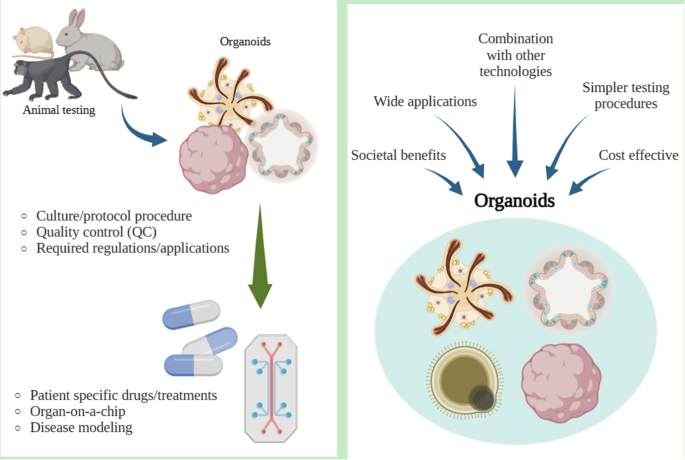
Avoid common mistakes on your manuscript.
Introduction
Historically, animal models have contributed substantially to the advancement and study of vaccines, surgical techniques, and various scientific experiments [ 1 ]. However, owing to the problems associated with animal testing, researchers are now questioning whether animal models and tests are the best options for these procedures. Growing animal testing is ethically concerning amid scientific evolution. According to the Humane Society International Organization, more than 100 million animals are killed annually worldwide for scientific purposes (Humane Society International). The animals used vary depending on their traits and include rats, mice, rabbits, dogs, cats, guinea pigs, zebrafish, swine [ 2 , 3 ].
In December 2022, the U.S. Food and Drug Administration (FDA) announced animal testing is no longer mandatory safety approval of products [ 4 ]. However, products that are used on the human body still require safety testing. In other words, testing for toxicity, compatibility, and safety is compulsory for products; however, animal testing is unnecessary for conducting these tests. In response, research facilities and companies have introduced alternatives such as computer simulations and in silico models. Stem cell therapy has gained popularity throughout the medical field, and various studies are underway to gain deeper knowledge [ 5 ]. With the emergence of this stem cell-based test, alternative methods have also arisen, potentially offering to become a replacement for animal testing.
When comparing test options, alternatives offer more beneficial attributes than animal testing. Non-animal tests are cost-effective, less time-consuming, and simpler procedures than animal tests [ 6 ]. However, most research institutions use animal models. This is because animal testing has been a longstanding experimental approach for decades [ 7 , 8 ]. Efforts are being made to replace animal testing with the use of human cells, as animal testing results often exhibit interspecies differences with humans, thus lacking the ability to reliably predict clinical outcomes. Application of advancing stem cell technology continue, but completely replacing animal experimentation poses significant challenges. Therefore, it is important to conduct further studies to advance the science of alternative testing methods. This review aimed to summarize the use of stem cell technology as an alternative to animal testing and discuss its advantages and limitations.
Current State of Animal Testing
Uses of animal testing.
Animal testing has been used for decades, and in the 21st century, the number of tests has increased considerably [ 2 ]. With approximately 100 million animals used for testing annually worldwide, science has been rapidly evolving. The primary function of animal testing is to test drugs, their toxicity, and their compatibility with the human body to ensure safe use. Hence, pre-launch testing is crucial. Companies and research facilities must subject their products to clinical trials before introducing them to potential customers.
Neurological disorder such as Parkinson’s and Alzheimer’s have also been modeled in animals to understand their mechanisms and to determine suitable treatments [ 9 , 10 , 11 ]. For instance, in the case of Parkinson’s disease, various animal models have been employed, including Caenorhabditis elegans, Zebrafish, and mice. Additionally, genetically modified mice carrying mutations associated with proteins like α-synuclein, Parkin, Pink1, and LRRK2, as well as mice induced with α-Synuclein Pre-Formed Fibril (PFF), are utilized to assess dopaminergic neuronal loss and investigate changes in α-synuclein aggregation. In Alzheimer’s disease, transgenic mice carrying mutations associated with familial Alzheimer’s disease (FAD), such as the 5xFAD model, are commonly used. These models allow for the evaluation of amyloid beta reduction through histological methods and the assessment of drug efficacy using behavioral tests like the Maze, providing insights into underlying disease mechanisms. Animals utilized as disease models contribute significantly to our comprehensive understanding of the mechanisms behind various illnesses, facilitating our grasp of these conditions. Research conducted using these animal disease models has indeed contributed to the discovery and development of treatments. However, it’s scientifically crucial to acknowledge that these animal models often present disparities in lifespans compared to humans and may not entirely mirror the intricate etiology of human diseases. Additionally, while animal experimentation is utilized for various conditions such as cancer, diabetes mellitus, and traumatic brain injury, it’s constrained by its inability to fully capture the nuances of the human immune system and intricate disease mechanisms (Table 1 ).
In addition to modeling diseases, animals are also used to test cosmetics or healing rates of products. In the cosmetics industry, animals are typically used to test skin or eye irritation to assess the safety of these products in humans [ 17 , 18 ]. The Draize test, developed in 1944 to test for such hazards in rabbits [ 19 ], is used to test products such as drugs and balms for wound healing. It involves creating wounds on animals to gauge recovery rates [ 16 ].
Related laws, Guidelines, and Principles
As of 2023, current regulations state that the FDA no longer deems animal tests necessary for evaluating product safety [ 4 ]. This enables companies and research facilities to explore possible non-animal testing when obtaining product approval. Additionally, out of 195 countries worldwide, only 42 have laws or regulations limiting animal testing for products (The Humane Society). Animal testing laws have been implemented by banning animal testing or limiting its use during testing. Europe completely banned cosmetics tested on animal testing in 2013 [ 3 , 20 , 21 ]. This demonstrates a push to limit animal testing; however, the movement remains ineffective because of the absence of laws against animal testing in most countries.
Guidelines for animal experimentation and clinical trials for drug development and safety testing have varied procedures among companies and researchers up to now. So, the Guidance for Industry for Preclinical Safety Evaluation of Biotechnology-Derived Pharmaceuticals from the Center for Drug Evaluation and Research provides guidelines for the safety assessment of products compiled from regulatory standards of several countries. According to these guidelines, preclinical trial researchers should consider factors such as animal species, age, delivery method (dosage, administration, treatment regimen, etc.), and test material stability [ 22 ] (Fig. 1 ).
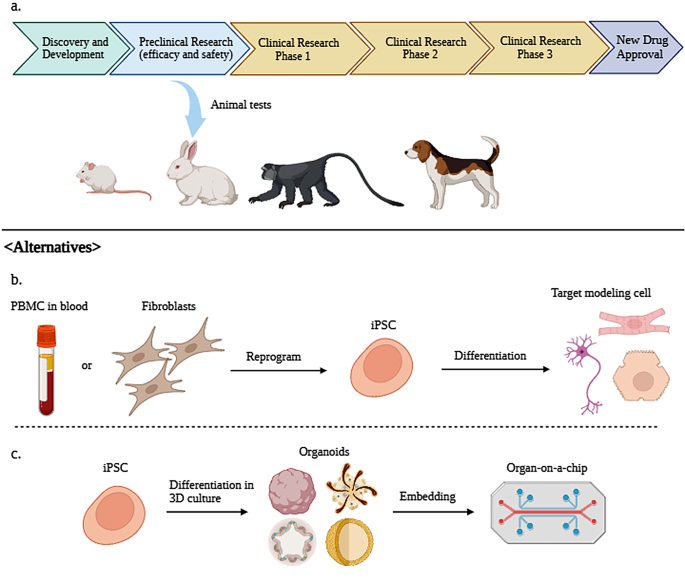
( A ) Procedure of new drug approval as stated by the Food and Drug Administration (FDA). In the preclinical research stage, small, medium, and large animals are usually used for testing new drugs. ( B ) iPSCs that can replacing animal testing. PBMCs or fibroblasts are reprogrammed to iPSCs and subsequently differentiated into target modeling cells such as neurons, cardiomyocytes, and hepatocytes. ( C ) iPSC-derived 3D organoids enable in vitro efficacy and safety testing. Organ-on-a-chip embedded with organoids used in in vitro tests, created using BioRender
The FDA has also provided a drug development process that includes these steps. The first step in drug development is discovering and researching a new drug (discovery and development stage). The second stage is preclinical research, in which drugs have to undergo a series of animal tests (or alternative tests, if possible) for safety. The FDA strongly suggests that animal preclinical trials follow Good Laboratory Practice (GLP). The main elements of GLP are as follows [ 23 ]: appropriate use of qualified personnel, quality assurance, appropriate use of facility and care for animals, proper operating procedures for animals used in trial, individual animal data collection and evaluation, testing product properly handled and analyzed, study proceeds with an approved protocol, data should be collected as outlined in the protocol, and full report prepared after procedures.
To enhance clinical translation, reproducibility issues in preclinical trials, such as biased allocation, insufficient controls, and lack of interdisciplinary, uncharacterized, or poorly characterized supplies [ 24 ]. The third step involves clinical testing on humans to assess safety and efficacy. The fourth and fifth stages comprise FDA post-market safety monitoring for all approved drugs [ 25 ].
Guidelines also suggest the 3R (replacement, reduction, and refinement) principle, which recommends that scientists follow certain criteria during clinical trials. Replacement involves using other testing methods other than animal testing [ 26 ]. In computer models, tissues, or stem cell research, if alternatives to animal testing exist, researchers should prioritize their use. Reduction involves minimizing the number of animal tests [ 26 ]. Questioning the necessity of animal tests during a particular part of our research and reducing their numbers imbues the concept with meaning. Refinement focuses on minimizing stress and providing the best care to animals [ 26 ], including providing proper food, entertainment, and clean well-maintained shelters.
As International efforts for animal replacement methods, research and development into alternative testing methods is already underway in both Europe and the United States, with each regulatory body establishing its own initiatives. In Europe, the European Center for the Validation of Alternative Methods (ECVAM) was founded in 1992, and since 2013, the sale of cosmetics containing ingredients tested on animals has been completely banned. Moreover, there are plans to expand the scope to include medical devices, health supplements, and pharmaceuticals in the future. In the United States, the Interagency Coordinating Committee on the Validation of Alternative Methods (ICCVAM) was established in 2000. The objective is to reduce animal testing by 2025 and eliminate mammalian animal testing entirely by 2035 through innovative advancements in alternative testing methodologies. In 2022, amendments to the Food, Drug, and Cosmetic Act in the United States removed mandatory animal testing requirements in the drug development stage and presented alternative testing methods as viable non-clinical trial options.
Problems/limitations of Animal Testing
A pressing issue with animal testing is the ethical concerns stemming from it. Most studies have demonstrated that these models undergo invasive procedures that often result in pain or even death. Research indicates that animals share pain and emotional capacity with humans [ 27 ]. Thus, sacrificing them for research can appear cruel. Advocates call for equitable treatment, opposing animal testing as inhumane and cruel. Such ethical issues has always followed animal testing and are ongoing [ 28 ].
Moreover, some studies have indicated that animal testing is not an accurate model for medicines or substances, highlighting the need for accurate and efficient testing alternatives that are similar humans. The complexity of human disease mechanisms raises doubts whether animal models can accurately replicate them.
Physiological differences between animals and humans mean a product safe for animals may not guarantee human safety [ 29 ]. Interspecies differences have led to poor results in correlating animal testing with human outcomes, consequently causing several clinical trial failures [ 30 ]. Between 2010 and 2017, clinical trials for drugs had a greater chance of failing phase І, owing to safety and efficacy [ 31 ]. In addition, even if a product passes phase І there is still a 90% rate of failure while undergoing the necessary procedures [ 32 , 33 ]. Prolonged use of animal testing can ultimately endanger humans, as some drugs and products approved through trials were later deemed harmful. Concerns such as high cost and long laborious procedures will be discussed below.
Benefits of Replacing Animal Testing
The main benefits of replacing animal tests with alternatives are as follows: cost-effective, time efficient, less complex testing procedures, and societal benefits.
Stem cell modeling is less expensive than animal testing. The Draize test mentioned before costs approximately $1,800, whereas non-animal testing methods cost considerably less [ 6 ]. Affordable procedures offer renewed chances for past costly research to emerge. A decrease in the cost of procedures would facilitate new drug development, making opportunities for new technologies easier.
Animal testing requires prior preparation that is often complex and time consuming. Several guidelines of various organizations worldwide follow certain principles and procedures. For animal testing, factors such as providing clean and well-maintained shelters, food, necessary supplies for survival, and entertainment are laborious [ 26 ]. Alternatives are time-efficient and less laborious, simpler protocols, and fewer supplies to maintain procedures.
Alternatives to Animal Testing Related to Stem Cells
Organoids are organ-like structures derived from self-organizing stem cells in 3D cell cultures. They exhibit organ-specific characteristics and originate from stem cells undergoing self-organization [ 34 , 35 ]. . They are beneficial over previous 2D cell culture, as they can show near-physiological cellular composition and actions [ 36 ]. Organoids are typically established from embryonic stem cells (ESCs), human pluripotent stem cells (PSCs), and adult stem cells [ 37 , 38 , 39 ]. The potential of organoids as alternatives stems from their correlation with patient reactions to products such as drugs, indicating that they are a promising for rare diseases where clinical trials are impractical [ 39 ]. Organoids have a wide range of applications and are suitable for studies of infectious diseases, hereditary diseases, and toxicity, and can provide personalized medicine for individual patients [ 38 ].
Recent studies have shown that PSC organoids can form complex brain organoids that are useful for modeling traumatic brain injury [ 15 ]. Organoids derived from PSCs are of various types, including stomach, lung, liver, kidney, cerebral, and thyroid, and can contribute to organ failure or dysfunction. Cancer organoids are cultured from thin tumor sections, which are efficient for studying cancer syndromes [ 34 ]. Organoid studies on Alzheimer’s disease highlight the possibility of using familial or sporadic Alzheimer’s disease induced pluripotent stem cells (iPSCs) to model brain activity [ 40 ]. Thyroid follicles derived from hESCs have the potential to be used as organoids to treat hypothyroidism [ 41 ] (Table 2 ). Technology development of 3D bioprinting organoids is underway, promising better productivity. Bioprinting for organoids includes inkjet-based bioprinting, laser-assisted bioprinting, extrusion-based bioprinting, and photo-curing bioprinting [ 42 ]. Ongoing studies are also exploring 3D printing technology using organoids, offering the possibility of creating organs for patient-tailored services and toxicology research.
However, organoids still possess limitations that render them unsuitable tools to replace animal testing. Organoids lack of vasculature structure affects growth and maturation, leading to differences in behavior compared to the original tissue [ 59 ]. This may result in only partial replication, leading to an incomplete disease model [ 38 ]. Moreover, the complexity and heterogeneity of certain organs, such as the brain or immune system, pose challenges for complete replication in organoid models. This inability to replicate such complexity can affect the translatability of findings from organoid studies to clinical applications. Research and experiments involving organoids often require lengthy culture protocols, which can vary depending on the type of organoid being cultivated. In some extreme cases, organoid culture may extend for months or even years, as seen in examples such as intestinal organoids(8 weeks or more), retinal organoids(6 ~ 39 weeks or more), brain organoids(12 weeks or more), and liver organoids(4 ~ 8 weeks or more) [ 60 , 61 , 62 , 63 , 64 ]. Even after going through the lengthy process, there are sometimes a lack of established organoids in sufficient numbers. This limited availability of organoids can hinder the procedure of functional testing, which can lead to insufficient research outcomes. Organoids also lack the intricate network of connections that can be seen in living organisms. Inter-organ communication is crucial when checking metabolic health, and with organoids lacking such an important factor, it is difficult to create treatments for any abnormalities regarding infection and diseases. Organoids also lack a diverse set of cell types, structural organization, and physiological functions in comparison to functioning organs, which limits the ability to accurately replicate disease processes and responses to treatment [ 59 ]. When compared to animal models, organoids fall behind, as animal models offer a broader view of processes for diseases, immune responses, and systemic effects of treatments. Another noteworthy concern arises from the fact that current production technology for organoids under GMP (Good Manufacturing Practice) standards has yet to be established.
Quality Control of Organoid
For organoids to serve as suitable models for diseases or experimental purposes, quality control (QC) is essential. Accuracy and consistency in production lead to more precise results, ensuring better therapeutic treatments or modeling. If quality control for organoids isn’t established sufficiently, problems such as inconsistent test results, misinterpretation of existing data, wastage of valuable resources, reproducibility issues, unreliable models, and ethical concerns regarding biomedical studies could arise.
Organoid structures and functions can be assessed through multiple methods. Structural assessment of organoids can be performed using bright-field imaging for both quantitative and qualitative research. Additionally, methods such as immunofluorescent staining, transmission electron microscopy, and scanning electron microscopy are also utilized [ 65 , 66 ]. The functionality of organoids can be assessed through qPCR and single-cell or bulk cell RNA sequencing, which provide quantitation of marker gene expression, revealing cell identity and composition [ 67 ]. Assay methods like ELISA and colorimetric assays are useful for secretome quantification while Luciferase essays help measure enzyme activity [ 65 , 68 ]. Staining methods such as Glycosaminoglycan (GAG) staining(specifically for synovial mesenchymal stromal cell (SMSC) organoids), immunofluorescence staining, and Alizarin red staining mainly help with visualizing components within the organoid [ 65 , 68 , 69 ]. There are also more direct methods like implantation to test the in vivo functions of organoids [ 65 , 70 ] (Table 3 ).
Extracellular microenvironment, which contain such things as soluble bioactive molecules, extracellular matrix, and biofluid flow, contributes to the growth rate and formation of organoids. Given the variation in extracellular microenvironments across different types of organoids, it is imperative to modulate the extracellular microenvironment accordingly for each organoid type. This ensures the production of organoids with consistent quality across different production batches [ 71 ].
Regulations/Applications Regarding Organoids from the FDA
While there aren’t any specific regulations regarding organoids from the FDA(Food and Drug Administrations) as of in the recent years, there are two categories of applications that include framework for cell related therapies, which include organoids. There are two applications, Biologics License Application (BLA) and the Investigational New Drug (IND) Application. The BLA, as stated in the official website of FDA, is a request for permission to introduce and deliver for a biologic product(vaccines, somatic cells, gene therapy, tissues, recombinant therapeutic proteins, organoids, etc.) into interstate commerce. Requirements for a BLA includes applicant information, product/manufacturing information, pre-clinical studies, clinical studies, and labeling. The IND application is a request for authorization to administer an investigation drug or biological product to humans. IND had three types: Investigator IND, Emergency Use IND, and Treatment IND which could fall into two categories being commercial or non-commercial. The IND application must contain the following broad areas of information: Animal Pharmacology and Toxicology studies, Manufacturing Information, Clinical protocols and Investigator Information.
When examining the current ongoing clinical trials( ClinicalTrials.gov ) in the application of organoids, it can be noted that they are being utilized in refractory cancers, osteosarcoma, high-grade glioma, advanced breast cancer, and colorectal cancer. This pertains to the utilization of the organoid platform to investigate the sensitivity to various drugs (chemotherapy, hormonal therapy, targeted therapy) by exposing them to each individual agent (or combination of agents). It is anticipated and ongoing to aid in clinical decisions regarding the optimal treatment option for each patient.
Organ-on-a-chip
Organoid chips(OoC) can be regarded as the outcome of merging biology and microtechnology, serving as microfluidic cell culture devices [ 72 , 73 ]. OoC has the ability to mimic the cellular environment, which leads to an examination of their effects on cell communication with more accessibility and ease. The chips are generally designed by collecting cells (primary cells, transformed cell lines, human ESC, or iPSCs) using equipment with pumps(that enable fluid flow), incubators, sensors, and microscopes to monitor and examine the cells in the system [ 49 , 74 ] (Fig. 1 ). Depending on the type or cell or method cells can be aggregated in matrix or matrixless conditions [ 75 ].
Various types of human organ chips, including the liver, heart, eyes, kidneys, bones, intestines, and skin, are used to simulate the breathing motion. Single-organ chips such as liver-on-a-chip and lung-on-a-chip are useful for observing individual chemical reactions [ 53 ]. There are also multiple organ-on-chip, which are organ-chips connected to a vast system [ 76 ]. The main purpose of multi-organ-on-chips is to simulate the entire body, recognizing that a single organ does not represent the entire human system. Using multiple organ-on-chips connected to one system allows the analysis of how various organs communicate with each other.
The U.S. Food and Drug Administration (FDA) and the U.S. National Institutes of Health (NIH) have provided project support for tissue chips for drug screening, including lung-on-a-chip. Additionally, efforts are being made globally to advance the utilization of organoid chips, such as the establishment of the European Organ-on-Chip Society in Europe.
A limitation of OoCs is their complex experimental setup [ 77 ], which can be avoided with clear guidelines or protocols. Cell medium changes also raise concerns about chip environments [ 77 ]. There is also the issue of using animal models to validate OoC systems initially [ 78 ]. To address this, OoC experts recommend forming well-established collaborations with developers, toxicologists, and pharmaceutical companies to explore alternative solutions.
iPSCs(Induced Pluripotent stem Cells)
iPSCs are a recent development in the field of disease modeling. Having traits such as self-renewal and pluripotency, iPSCs can transform into various cells within the human body (Fig. 1 ); thus, reprogramming patient cells creates personalized medicine for specific diseases [ 79 , 80 ]. The ability to produce a large batch of iPSCs with only a small number of patient samples is important [ 81 , 82 ]. The objectives of iPSC models closely align with the 3R principle [ 83 ]. Replacing animal models in research while adhering to reduction and refinement principles is expected to be advantageous.
iPSCs are research to find cures for various diseases and are used as broad disease models (Table 2 ). For example, iPSCs from patients with Parkinson’s disease differentiate into midbrain dopaminergic neurons (DAns) in the substantia nigra pars compacta (SNpc), which can be used to model Parkinson’s disease on a cellular basis [ 43 , 44 , 45 ]. For cardiac diseases, which include a decrease in cardiomyocytes that leads to scar formation and ultimately heart function failure, there are existing studies that explore iPSCs for novel therapeutic cures [ 84 ]. iPSC-derived progenitors such as human HCN4 + and human ESC derived ROR2+, CD13+, KDR+, PDGFRα + cells later generate cardiomyocytes [ 47 ]. For cancer modeling using iPSCs, reprogrammed tumor specimens or iPSCs with premalignant or early genetic lesions can show the stages of cancer [ 49 ]. iPSCs from patients that are healthy and those with Alzheimer’s disease differentiate into the main brain cells, modeling the human brain with a functional blood barrier. Further research could drive drug discovery [ 9 ]. Studies of organ failure or dysfunction have shown that human iPSCs are useful. Research on lung regeneration has shown that endogenous and exogenous stem cells mediate therapeutic results [ 50 ]. Another study focused on the use of liver hepatoblasts, which could help alleviate hepatotoxicity through liver development and hepatic differentiation [ 85 ].
However, iPSCs are still in a relatively early developmental phase and have several limitations. Concerns for researchers regarding iPSCs is in vitro culture adaptation and tumorigenicity, the inability to completely reflect in vivo 3D environments, and the variation of differentiated cells depending on the protocol [ 86 , 87 ]. Quality control of differentiated cells and influencing factors are crucial for iPSC researchers, impacting their applicability as medical models or treatments.
Figure 2 Human diagram showing multiple stem cell-related technologies that can be applied to various human organs.
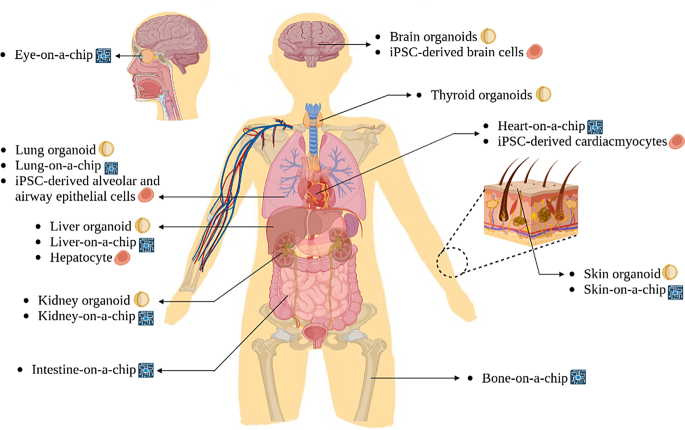
A BioRender diagram depicts diverse stem cell technologies for human organs
Limitations
Stem cell-related methodologies, such as organoids, are a very new technology in the field of animal alternative testing. In the early developmental stage, alternative stem cell models and technologies still require a few years of testing. Animal testing is still used today, owing to its historical role in safety and efficacy assessment. New alternatives have been presented; however, the uncertainty of these methods have caused most researchers to adhere to old protocols. In cases of complex diseases arising from various factors such as cardiovascular, neurodegenerative, and infertility, complete replacement by animal alternative testing methods may still be impractical. In such instances, it is crucial to concurrently employ animal experimentation alongside alternative testing methods utilizing organoids or stem cells to bolster data reliability. As a component of these endeavors, numerous researchers have undertaken disease modeling, such as stroke, utilizing brain organoids and cardiac organoids in in vitro experiments. The solution involves focusing on alternative testing methods [ 88 ]. By transforming old methods and creating alternatives, this shift could be the norm. There has already been a move toward that goal, as the FDA has established a cross-agency working group (The Alternative Methods Working Group) to promote various alternative methods, such as in vivo, in vitro, in silico , or system toxicology modeling [ 89 ]. In the 2021, FDA report titled “Advancing Regulatory Science at FDA,” the most prioritized area is identified as “Advancing Novel Technologies to Improve Predictivity of Non-clinical Studies and Replace, Reduce, and Refine Reliance on Animal Testing.”
Given ongoing research in alternative stem cell-related methods, this appears promising to replace animal testing. These alternatives offer advantages for scientists and the public. However, it is important to acknowledge that iPSCs, organoids, and OoCs each have distinct strengths and limitations. With continued advancements and studies to further understand these issues, these limitations can be avoided.
Data Availability
All data pertaining to this manuscript are included within the article.
Abbreviations
Food and Drug Administration
organ-on-chip
induced pluripotent stem cell
pluripotent stem cell
Embryonic stem cell
Center for Drug Evaluation and Research, GLP, Good Laboratory Practice
Dopaminergic neurons
Substantia Nigra pars compacta
Robinson, N. B., et al. (2019). The current state of animal models in research: A review. International Journal of Surgery , 72 , 9–13.
Article PubMed Google Scholar
Ericsson, A. C., Crim, M. J., & Franklin, C. L. (2013). A brief history of animal modeling. Missouri Medicine , 110 (3), 201–205.
PubMed PubMed Central Google Scholar
Balls, M. (2022). Alternatives to Laboratory animals: Trends in replacement and the three rs. Alternatives to Laboratory Animals , 50 (1), 10–26.
Han, J. J. (2023). FDA modernization Act 2.0 allows for alternatives to animal testing. Artificial Organs , 47 (3), 449–450.
Deinsberger, J., Reisinger, D., & Weber, B. (2020). Global trends in clinical trials involving pluripotent stem cells: A systematic multi-database analysis. Npj Regenerative Medicine , 5 (1), 15.
Article PubMed PubMed Central Google Scholar
Meigs, L., et al. (2018). Animal testing and its alternatives - the most important omics is economics. Altex , 35 (3), 275–305.
Horejs, C. (2021). Organ chips, organoids and the animal testing conundrum. Nat Rev Mater , 6 (5), 372–373.
Veening-Griffioen, D. H., et al. (2021). Tradition, not science, is the basis of animal model selection in translational and applied research. ALTEX , 38 (1), 49–62.
PubMed Google Scholar
Penney, J., Ralvenius, W. T., & Tsai, L. H. (2020). Modeling Alzheimer’s disease with iPSC-derived brain cells. Molecular Psychiatry , 25 (1), 148–167.
Khan, E., Hasan, I., & Haque, M. E. (2023). Parkinson’s Disease: Exploring Different Animal Model Systems . International Journal of Molecular Sciences , 24(10).
Chia, S. J., Tan, E. K., & Chao, Y. X. (2020). Historical Perspective: Models of Parkinson’s Disease . International Journal of Molecular Sciences , 21(7).
Li, Z., et al. (2021). Application of animal models in Cancer Research: Recent progress and future prospects. Cancer Manag Res , 13 , 2455–2475.
Article CAS PubMed PubMed Central Google Scholar
Kottaisamy, C. P. D., et al. (2021). Experimental animal models for diabetes and its related complications—a review. Laboratory Animal Research , 37 (1), 23.
King, A. J. (2012). The use of animal models in diabetes research. British Journal of Pharmacology , 166 (3), 877–894.
Ramirez, S. (2021). Modeling traumatic Brain Injury in Human Cerebral organoids . Cells , 10(10).
Grada, A., Mervis, J., & Falanga, V. (2018). Research Techniques made simple: Animal models of Wound Healing. The Journal of Investigative Dermatology , 138 (10), 2095–2105e1.
Article CAS PubMed Google Scholar
McNamee, P., et al. (2009). A tiered approach to the use of alternatives to animal testing for the safety assessment of cosmetics: Eye irritation. Regulatory Toxicology and Pharmacology , 54 (2), 197–209.
Macfarlane, M., et al. (2009). A tiered approach to the use of alternatives to animal testing for the safety assessment of cosmetics: Skin irritation. Regulatory Toxicology and Pharmacology , 54 (2), 188–196.
York, M., & Steiling, W. (1998). A critical review of the assessment of eye irritation potential using the Draize rabbit eye test. Journal of Applied Toxicology , 18 (4), 233–240.
Fentem, J. H. (2023). The 19th FRAME Annual lecture, November 2022: Safer Chemicals and Sustainable Innovation Will be achieved by Regulatory Use of Modern Safety Science, not by more animal testing. Alternatives to Laboratory Animals , 51 (2), 90–101.
Daneshian, M., et al. (2015). Animal use for science in Europe. Altex , 32 (4), 261–274.
Center for Drug Evaluation and, R., R. Center for Biologics Evaluation and, and H. International Conference on, Guidance for industry: S6 preclinical safety evaluation of biotechnology-derived pharmaceuticals (1997). Rockville, MD: U.S. Dept. of Health and Human Services, Food and Drug Administration, Center for Drug Evaluation and Research: Center for Biologics Evaluation and Research.
Centanni, J. M. (2017). Chap. 2 - Preclinical Animal Testing requirements and considerations . Mesenchymal stromal cells (pp. 37–60). Academic. S. Viswanathan and P. Hematti, Editors.
Ioannidis, J. P. A., Kim, B. Y. S., & Trounson, A. (2018). How to design preclinical studies in nanomedicine and cell therapy to maximize the prospects of clinical translation. Nat Biomed Eng , 2 (11), 797–809.
Van Norman, G. A. (2016). Drugs, devices, and the FDA: Part 1: An overview of approval processes for drugs. JACC Basic Transl Sci , 1 (3), 170–179.
Liebsch, M., et al. (2011). Alternatives to animal testing: Current status and future perspectives. Archives of Toxicology , 85 (8), 841–858.
Kiani, A. K., et al. (2022). Ethical considerations regarding animal experimentation. Journal of Preventive Medicine and Hygiene , 63 (2 Suppl 3), E255–E266.
Doke, S. K., & Dhawale, S. C. (2015). Alternatives to animal testing: A review. Saudi Pharm J , 23 (3), 223–229.
Akhtar, A. (2015). The flaws and human harms of animal experimentation. Cambridge Quarterly of Healthcare Ethics , 24 (4), 407–419.
Van Norman, G. A. (2020). Limitations of Animal studies for Predicting toxicity in clinical trials: Part 2: Potential Alternatives to the use of animals in preclinical trials. JACC Basic Transl Sci , 5 (4), 387–397.
Dowden, H., & Munro, J. (2019). Trends in clinical success rates and therapeutic focus. Nature Reviews. Drug Discovery , 18 (7), 495–496.
Takebe, T., Imai, R., & Ono, S. (2018). The current status of Drug Discovery and Development as originated in United States Academia: The influence of Industrial and academic collaboration on Drug Discovery and Development. Clinical and Translational Science , 11 (6), 597–606.
Sun, D., et al. (2022). Why 90% of clinical drug development fails and how to improve it? Acta Pharm Sin B , 12 (7), 3049–3062.
Dutta, D., Heo, I., & Clevers, H. (2017). Disease modeling in stem cell-derived 3D Organoid systems. Trends in Molecular Medicine , 23 (5), 393–410.
Wang, X. (2019). Stem cells in tissues, organoids, and cancers. Cellular and Molecular Life Sciences , 76 (20), 4043–4070.
Li, M., & Izpisua Belmonte, J. C. (2019). Organoids - Preclinical models of Human Disease. New England Journal of Medicine , 380 (6), 569–579.
Rookmaaker, M. B., et al. (2015). Development and application of human adult stem or progenitor cell organoids. Nature Reviews Nephrology , 11 (9), 546–554.
Clevers, H. (2016). Modeling Development and Disease with Organoids. Cell , 165 (7), 1586–1597.
Schutgens, F., & Clevers, H. (2020). Human organoids: Tools for understanding Biology and Treating diseases. Annual Review of Pathology: Mechanisms of Disease , 15 , 211–234.
Article CAS Google Scholar
Barak, M., et al. (2022). Human iPSC-Derived neural models for studying Alzheimer’s Disease: From neural stem cells to cerebral organoids. Stem Cell Reviews and Reports , 18 (2), 792–820.
Romitti, M., et al. (2022). Transplantable human thyroid organoids generated from embryonic stem cells to rescue hypothyroidism. Nature Communications , 13 (1), 7057.
Ren, Y., et al. (2021). Developments and opportunities for 3D Bioprinted Organoids. Int J Bioprint , 7 (3), 364.
Laperle, A. H., et al. (2020). iPSC modeling of young-onset Parkinson’s disease reveals a molecular signature of disease and novel therapeutic candidates. Nature Medicine , 26 (2), 289–299.
Avazzadeh, S. (2021). Modelling Parkinson’s Disease: iPSCs towards Better Understanding of Human Pathology . Brain Sci , 11(3).
Stoddard-Bennett, T., & Reijo Pera, R. (2019). Treatment of Parkinson’s Disease through Personalized Medicine and Induced Pluripotent Stem Cells . Cells , 8(1).
Funakoshi, S., & Yoshida, Y. (2021). Recent progress of iPSC technology in cardiac diseases. Archives of Toxicology , 95 (12), 3633–3650.
Matsa, E., Burridge, P. W., & Wu, J. C. (2014). Human stem cells for modeling heart disease and for drug discovery. Science Translational Medicine , 6 (239), 239ps6.
Hnatiuk, A. P., et al. (2021). Human iPSC modeling of heart disease for drug development. Cell Chem Biol , 28 (3), 271–282.
Papapetrou, E. P. (2016). Patient-derived induced pluripotent stem cells in cancer research and precision oncology. Nature Medicine , 22 (12), 1392–1401.
Aboul-Soud, M. A. M., Alzahrani, A. J., & Mahmoud, A. (2021). Induced Pluripotent Stem cells (iPSCs)-Roles in Regenerative therapies, Disease Modelling and Drug Screening . Cells , 10(9).
Sun, W., et al. (2019). Organ-on-a-Chip for Cancer and Immune organs modeling. Adv Healthc Mater , 8 (15), e1900754.
Wang, Y., et al. (2020). Dantrolene ameliorates impaired neurogenesis and synaptogenesis in Induced pluripotent stem cell lines derived from patients with Alzheimer’s Disease. Anesthesiology , 132 (5), 1062–1079.
Beckwitt, C. H., et al. (2018). Liver ‘organ on a chip’. Experimental Cell Research , 363 (1), 15–25.
Shi, W., et al. (2021). Design and evaluation of an in vitro mild traumatic brain Injury modeling System using 3D printed Mini Impact device on the 3D cultured human iPSC derived neural progenitor cells. Adv Healthc Mater , 10 (12), e2100180.
Lee, J., et al. (2020). Hair-bearing human skin generated entirely from pluripotent stem cells. Nature , 582 (7812), 399–404.
Jung, S. Y., et al. (2022). Wnt-activating human skin organoid model of atopic dermatitis induced by Staphylococcus aureus and its protective effects by Cutibacterium acnes. iScience , 25 (10), 105150.
Risueño, I., et al. (2021). Skin-on-a-chip models: General overview and future perspectives. APL Bioeng , 5 (3), 030901.
Aghmiuni, A. I., & Keshel, S. H. (2023). Chap. 1 0 - Eye-on-a-chip , in Principles of Human Organs-on-Chips , M. Mozafari, Editor. Woodhead Publishing. pp. 315–369.
Andrews, M. G., & Kriegstein, A. R. (2022). Challenges of Organoid Research. Annual Review of Neuroscience , 45 , 23–39.
Pleguezuelos-Manzano, C., et al. (2020). Establishment and culture of human intestinal organoids derived from adult stem cells. Current Protocols In Immunology / Edited By John E. Coligan. [Et Al.] , 130 (1), e106.
Wahle, P., et al. (2023). Multimodal spatiotemporal phenotyping of human retinal organoid development. Nature Biotechnology , 41 (12), 1765–1775.
Kathuria, A., et al. (2020). Comparative transcriptomic analysis of cerebral organoids and cortical neuron cultures derived from Human Induced Pluripotent Stem cells. Stem Cells and Development , 29 (21), 1370–1381.
Zhao, J., et al. (2020). APOE4 exacerbates synapse loss and neurodegeneration in Alzheimer’s disease patient iPSC-derived cerebral organoids. Nature Communications , 11 (1), 5540.
Zhu, X., et al. (2021). Liver organoids: Formation strategies and Biomedical Applications. Tissue Eng Regen Med , 18 (4), 573–585.
Zhao, Z. (2022). Organoids Nat Rev Methods Primers , 2.
Drakhlis, L., et al. (2021). Human heart-forming organoids recapitulate early heart and foregut development. Nature Biotechnology , 39 (6), 737–746.
Broutier, L., et al. (2016). Culture and establishment of self-renewing human and mouse adult liver and pancreas 3D organoids and their genetic manipulation. Nature Protocols , 11 (9), 1724–1743.
Sun, Y., et al. (2021). Generating 3D-cultured organoids for pre-clinical modeling and treatment of degenerative joint disease, in Signal Transduct Target Ther (p. 380). England.
Hemeryck, L., et al. (2022). Organoids from human tooth showing epithelial stemness phenotype and differentiation potential. Cellular and Molecular Life Sciences , 79 (3), 153.
Huch, M., et al. (2013). In vitro expansion of single Lgr5 + liver stem cells induced by wnt-driven regeneration. Nature , 494 (7436), 247–250.
Sullivan, K. M., et al. (2022). Extracellular Microenvironmental Control for Organoid Assembly. Tissue Eng Part B Rev , 28 (6), 1209–1222.
Low, L. A., et al. (2021). Organs-on-chips: Into the next decade. Nature Reviews. Drug Discovery , 20 (5), 345–361.
Bhatia, S. N., & Ingber, D. E. (2014). Microfluidic organs-on-chips. Nature Biotechnology , 32 (8), 760–772.
Leung, C. M., et al. (2022). A guide to the organ-on-a-chip. Nature Reviews Methods Primers , 2 (1), 33.
Olgasi, C., Cucci, A., & Follenzi, A. (2020). iPSC-Derived liver organoids: A journey from Drug Screening, to Disease modeling, arriving to Regenerative Medicine . International Journal of Molecular Sciences , 21(17).
Picollet-D’hahan, N., et al. (2021). Multiorgan-on-a-Chip: A systemic Approach to Model and Decipher Inter-organ Communication. Trends Biotechnol , 39 (8), 788–810.
Wang, H., et al. (2021). 3D cell culture models: Drug pharmacokinetics, safety assessment, and regulatory consideration. Clinical and Translational Science , 14 (5), 1659–1680.
Kang, S., Park, S. E., & Huh, D. D. (2021). Organ-on-a-chip technology for nanoparticle research. Nano Converg , 8 (1), 20.
Kim, C. (2015). iPSC technology–powerful hand for disease modeling and therapeutic screen. Bmb Reports , 48 (5), 256–265.
Qian, L., & Tcw, J. (2021). Human iPSC-Based modeling of central nerve System disorders for Drug Discovery . International Journal of Molecular Sciences , 22(3).
Gómez-Lechón, M. J., & Tolosa, L. (2016). Human hepatocytes derived from pluripotent stem cells: A promising cell model for drug hepatotoxicity screening. Archives of Toxicology , 90 (9), 2049–2061.
Blaszkiewicz, J., & Duncan, S. A. (2022). Advancements in Disease modeling and Drug Discovery using iPSC-Derived hepatocyte-like cells . Genes (Basel) , 13(4).
O’Connor, M. D. (2013). The 3R principle: Advancing clinical application of human pluripotent stem cells. Stem Cell Research & Therapy , 4 (2), 21.
Article Google Scholar
Parrotta, E. I. (2020). Modeling Cardiac Disease mechanisms using Induced Pluripotent Stem Cell-Derived cardiomyocytes: Progress, promises and challenges . International Journal of Molecular Sciences , 21(12).
Takebe, T., et al. (2013). Vascularized and functional human liver from an iPSC-derived organ bud transplant. Nature , 499 (7459), 481–484.
Lee, A. S., et al. (2013). Tumorigenicity as a clinical hurdle for pluripotent stem cell therapies. Nature Medicine , 19 (8), 998–1004.
Kramer, N., et al. (2016). Full biological characterization of human pluripotent stem cells will open the door to translational research. Archives of Toxicology , 90 (9), 2173–2186.
Balls, M., Bailey, J., & Combes, R. D. (2019). How viable are alternatives to animal testing in determining the toxicities of therapeutic drugs? Expert Opinion on Drug Metabolism & Toxicology , 15 (12), 985–987.
Administration, U. S. F. D. (2021). Advancing New Alternative Methodologies at FDA . Jan U.S. Food & Drug Administration: FDA website. pp. 1–34.
Download references
Acknowledgements
Not applicable.
This research was supported by a grant of the Korea Health Technology R&D Project through the Korea Health Industry Development Institute (KHIDI), funded by the Ministry of Health & Welfare, Republic of Korea (grant number : HI22C1314).
Author information
Authors and affiliations.
School of Biopharmaceutical and Medical Sciences, Health & Wellness College, Sungshin Women’s University, 55, Dobong-ro 76ga-gil, Gangbuk-gu, Seoul, Republic of Korea
Guiyoung Park
CiSTEM laboratory, Convergent Research Consortium for Immunologic Disease, Seoul St. Mary’s Hospital, College of Medicine, The Catholic University of Korea, Seoul, 06591, Republic of Korea
Yeri Alice Rim & Ji Hyeon Ju
Division of Rheumatology, Department of Internal Medicine, Seoul St. Mary’s Hospital, Institute of Medical Science, College of Medicine, The Catholic University of Korea, 4 3, Seoul, 06591, Republic of Korea
Department of Biomedicine & Health Sciences, Seoul St. Mary’s Hospital, College of Medicine, The Catholic University of Korea, Seoul, 06591, Republic of Korea
Department of Biohealth Regulatory Science, Sungkyunkwan University, Suwon, South Korea
Yeowon Sohn & Yoojun Nam
Yipscell Inc, L2 Omnibus Park, Banpo-dearo 222, Seocho-gu, Seoul, Korea
Yoojun Nam & Ji Hyeon Ju
You can also search for this author in PubMed Google Scholar
Contributions
GP designed the study and wrote the manuscript. YAR, YS and YN edited the manuscript. YN and JHJ approved the final manuscript. All the authors have read and approved the final draft of this manuscript.
Corresponding authors
Correspondence to Yoojun Nam or Ji Hyeon Ju .
Ethics declarations
Ethics approval and consent to participate, consent for publication, competing interests.
The authors declare that they have no competing interests.
Additional information
Publisher’s note.
Springer Nature remains neutral with regard to jurisdictional claims in published maps and institutional affiliations.
Rights and permissions
Open Access This article is licensed under a Creative Commons Attribution 4.0 International License, which permits use, sharing, adaptation, distribution and reproduction in any medium or format, as long as you give appropriate credit to the original author(s) and the source, provide a link to the Creative Commons licence, and indicate if changes were made. The images or other third party material in this article are included in the article’s Creative Commons licence, unless indicated otherwise in a credit line to the material. If material is not included in the article’s Creative Commons licence and your intended use is not permitted by statutory regulation or exceeds the permitted use, you will need to obtain permission directly from the copyright holder. To view a copy of this licence, visit http://creativecommons.org/licenses/by/4.0/ .
Reprints and permissions
About this article
Park, G., Rim, Y.A., Sohn, Y. et al. Replacing Animal Testing with Stem Cell-Organoids : Advantages and Limitations. Stem Cell Rev and Rep (2024). https://doi.org/10.1007/s12015-024-10723-5
Download citation
Accepted : 08 April 2024
Published : 19 April 2024
DOI : https://doi.org/10.1007/s12015-024-10723-5
Share this article
Anyone you share the following link with will be able to read this content:
Sorry, a shareable link is not currently available for this article.
Provided by the Springer Nature SharedIt content-sharing initiative
- Animal Testing Alternatives
- Animal Testing law
- Organ-on-chips
- Find a journal
- Publish with us
- Track your research
Thank you for visiting nature.com. You are using a browser version with limited support for CSS. To obtain the best experience, we recommend you use a more up to date browser (or turn off compatibility mode in Internet Explorer). In the meantime, to ensure continued support, we are displaying the site without styles and JavaScript.
- View all journals
- Explore content
- About the journal
- Publish with us
- Sign up for alerts
- Published: 26 April 2021
Organ chips, organoids and the animal testing conundrum
- Christine Horejs 1
Nature Reviews Materials volume 6 , pages 372–373 ( 2021 ) Cite this article
15k Accesses
27 Citations
117 Altmetric
Metrics details
- Biomaterials
- Medical research
Nature Reviews Materials speaks to Donald Ingber, Founding Director of the Wyss Institute for Biologically Inspired Engineering at Harvard University, about the animal testing conundrum and the importance of human-relevant models in biomedical research.
Animal models are commonly used to study human diseases and treatments; however, they are often limited in their ability to mimic human conditions, in particular, on the molecular and cellular levels. Why are we still using them?
The first and short answer is that we use them because they are there, and because we have used them in the past and we know how to work with animal models. Secondly, many in vitro models are too simplified to predict in vivo conditions. A main power of animal models is the possibility of genetic engineering, based on specific genetic alterations that we suspect may be involved. The question is whether we are fooling ourselves, because we convince ourselves that what we see is what we thought it should be. Indeed, many drugs first go through animal models before reaching clinical trials, and the large majority of those drugs fail in the clinic. Of course, there are also ethical considerations when it comes to animal models.
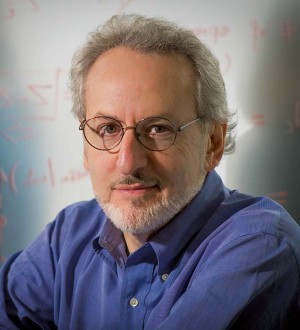
But we are now at a point at which we have much more complex in vitro models available, ranging from organoids to multiphysiological systems and organs-on-chips. Some of these in vitro models are as good or even better than animal models. However, these platforms need to be fit-for-purpose, because they are not generic. There is no in vitro model that can replace all animal models. You need different models even for one organ, such as the lung. One would require distinct models for asthma, pulmonary fibrosis or pulmonary edema, for example. We work with animal models, because they often exhibit phenotypes that appear like those of a human disease, but the underlying molecular and cellular mechanisms can be quite different. Moreover, some technologies, such as therapeutic CRISPR RNAs or monoclonal antibodies do not work in animal models at all, because they are so specific for human target sequences. Similarly, vaccine responses are often not representative in animal models, not even in non-human primates.
However, for a long time there was really no alternative to animal models. Of course, we need to study animal models because of one-health applications, linking animal health, human health and the environment. However, you can also create animal organs-on-chips, such as a bat intestine-on-a-chip. In this way, zoonotic infections, such as COVID-19, can also be studied using in vitro platforms, and you can also undertake genetic engineering in organs-on-chips. We are now at a time, in which we can create in vitro systems that are more relevant to humans or animal models, depending on how we build them.
Many publishers, funding agencies and regulatory agencies often dictate the use of animal models at high numbers. Do you think that these requirements should be challenged?
Absolutely. I think if reviewers were aware that there are alternative models out there, they might demand validation in a human-relevant model rather than in an animal model. Many reviewers, however, do not know about these new in vitro models, such as human organs-on-chips or other types of complex microphysiological systems. Reviewers often make valid points, for example, to consider the organism-level context or pharmacokinetics; however, an animal model may often not be the best model to study these aspects. For example, we found that breathing motions influence the rate of lung cancer growth and invasion in a human lung chip. In this case, it would have been impossible to use an animal model, because, obviously, we cannot keep an animal alive for three weeks without breathing. However, this was demanded by one reviewer.
Asking for animal models is often a reflex, because this is how it’s been done for many years, even in cases, in which animal models are known to not replicate human physiology or pathophysiology. It is important to ask what the most important human-relevant experiment is, and if there is an in vitro model available that has been validated for human clinical mimicry, it should be valid to use this model. In my past discussions, even the FDA suggested that in vitro models could be useful if they are equally good as animal models, and not necessarily better, provided they are human and equally robust and validated. The same criteria should also be applied by reviewers for grants and papers.
Do you think there is barrier to using in vitro platforms instead of animals, in particular, for researchers who are not engineers?
People tend to do what they were trained to do, and they use methods they are comfortable with. It takes energy to learn about new technologies, their nuances and limitations. However, nowadays, you really do not need to know engineering to use organ-on-a-chip systems. There are even commercial suppliers. But you do need to adapt to these new systems, maybe run further controls to compare with previous data and to ensure that they are robust. So, yes, there is an activation barrier to using new in vitro platforms. Basically, we need to change the way we do things.
You have developed and worked on organ-on-a-chip systems for many years, and there are now chips available with very high complexity and human mimicry. However, the lack of immune components and systemic investigation is often criticized. Do you think this is valid criticism? And how could it be addressed?
I want to give an example about incorporation of immune responses. We worked on pulmonary edema induced by interleukin-2; it’s dose-limiting toxicity is pulmonary vascular leakage, which we mimicked on a chip. We clearly could show interleukin-2-induced edema without the need of immune cells, which is something you cannot do in an animal model or in a human clinical trial.
Similarly, you can use chips to decouple effects of mechanics and fluid flow. This is essentially a synthetic biology approach at the cell, tissue and organ level that allows us to apply a sort of Einsteinian methodology; that is, identifying the simplest systems that can recapitulate the in vivo complexity of the question you are interested in. For example, if I am interested in epithelial-endothelial crosstalk, I do not necessarily need immune cells.
But of course, we can add immune cells and other components to organs-on-a-chip platforms and we do when we cannot replicate a phenotype without them. For example, you can add circulating immune cells and show recruitment and shear-stress dependent initial adhesion to the endothelium lining our chips. Indeed, I see this as one of the big advantages of these systems. We can add components in one-at-a-time and control them independently. For example, we made a lymphoid follicle-on-a-chip and tested an influenza vaccine. We could replicate germinal centre formation, antibody class switching and IgG production and we detected the same cytokines as seen in humans who received the same commercial vaccine. We are now also testing the effects of new immune adjuvants in these platforms.
… we are now at a point at which we have much more complex in vitro models available, ranging from organoids to multiphysiological systems and organs-on-chips. Some of these in vitro models are as good or even better than animal models
Similarly, we have developed a bone marrow-on-chip, which contains the hematopoietic lineage. Other groups have also incorporated immune cells in organ-on-a-chip systems. Importantly, if you can replicate physiology with a simple system, you’ve got a handle on control.
The power of the microfluidic organs-on-chips we study is that they provide a window on molecular scale activities inside living cells in a physiologically relevant organ context, including tissue-tissue interfaces and relevant cells, as well as fluid flow, air-liquid interfaces and mechanical motions.
Also, using organs-on-chips you can visualize and analyse the same location again and again over time. This is not possible in more complex models, such as organoids or animal models. I see organs-on-chips as living 3D cross-sections of major functional units of human or animal organs, which we can analyse over time. We can even culture living microbiome in contact with living human cells using organs-on-chips. The discovery of the importance of the microbiome is one of the major paradigm shifts in medicine over the last 15 years, and you cannot study the human microbiome in animals, because they have a different microbiome.
So in terms of your initial question, it all comes down to whether your mechanism involves an immunological response. Then, of course, you need to add the relevant components. If you are not sure, it should be one of the variables you explore. But if you know what your response is, and you start simple and mimic the response with high fidelity early on, then you may not need greater complexity.
What are the next important milestones in organs-on-chips, in particular, from a materials science and engineering perspective?
Some of the biggest challenges are the need for more online read-outs and sensors. The idea is to instrument chips so that you can get multiple read-outs of functionality, in particular, cellular, biochemical and even genetic readouts. Multiplexed readouts in real time would change the field.
In addition, higher throughput is needed, with the possibility to analyse multiple individual channels in parallel; also, visualization at high resolution and automated molecular analysis in small volumes. We need to get the most information out of these systems in real time.
Regarding materials science, there is certainly a need for better materials that are optically clear, flexible and biocompatible. We use polydimethylsiloxane (PDMS), because it is simple, cheap and gas-permeable, but it also can absorb certain small drugs. So, alternatives would be welcome. Moreover, coming up with new ways to cheaply mass fabricate these chips is important for commercialization. Similarly, techniques to bond material layers is critical for instrumenting chips to prevent materials from peeling apart in fluid flow.
There is also a proposal to start a new institute in the National Institutes of Health (NIH) focusing on in vitro models, which may help to build up the number of people familiar with this opportunity. This should help to achieve a critical mass of researchers using this technology, who will then act as reviewers of grants and papers. Engaging more researchers may help to push the technology over the top, such that people start looking beyond animal models.
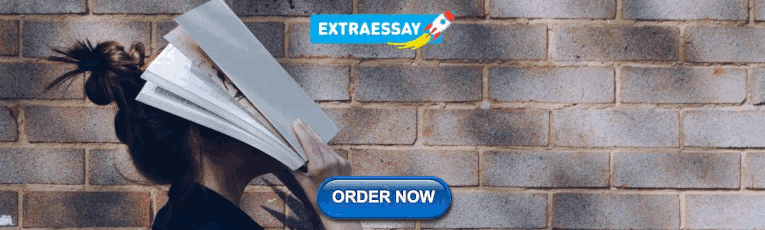
Author information
Authors and affiliations.
Nature Reviews Materials http://www.nature.com/natrevmats/
Christine Horejs
You can also search for this author in PubMed Google Scholar
Corresponding author
Correspondence to Christine Horejs .
Rights and permissions
Reprints and permissions
About this article
Cite this article.
Horejs, C. Organ chips, organoids and the animal testing conundrum. Nat Rev Mater 6 , 372–373 (2021). https://doi.org/10.1038/s41578-021-00313-z
Download citation
Published : 26 April 2021
Issue Date : May 2021
DOI : https://doi.org/10.1038/s41578-021-00313-z
Share this article
Anyone you share the following link with will be able to read this content:
Sorry, a shareable link is not currently available for this article.
Provided by the Springer Nature SharedIt content-sharing initiative
This article is cited by
Replacing animal testing with stem cell-organoids : advantages and limitations.
- Guiyoung Park
- Yeri Alice Rim
- Ji Hyeon Ju
Stem Cell Reviews and Reports (2024)
How human are our models?
Nature Reviews Bioengineering (2023)
Clamping strategies for organ-on-a-chip devices
- Daniel J. Teixeira Carvalho
- Lorenzo Moroni
- Stefan Giselbrecht
Nature Reviews Materials (2023)
Drugging p53 in cancer: one protein, many targets
Nature Reviews Drug Discovery (2023)
Ethanol Causes Cell Death and Neuronal Differentiation Defect During Initial Neurogenesis of the Neural Retina by Disrupting Calcium Signaling in Human Retinal Organoids
- Lingling Ge
Stem Cell Reviews and Reports (2023)
Quick links
- Explore articles by subject
- Guide to authors
- Editorial policies
Sign up for the Nature Briefing: Translational Research newsletter — top stories in biotechnology, drug discovery and pharma.

Public perception of laboratory animal testing: Historical, philosophical, and ethical view
Affiliation.
- 1 School of Pharmacy, Pharmacology Unit, University of Camerino, Camerino, Italy.
- PMID: 33331099
- PMCID: PMC9252265
- DOI: 10.1111/adb.12991
The use of laboratory animals in biomedical research is a matter of intense public debate. Recent statistics indicates that about half of the western population, sensitive to this discussion, would be in favor of animal testing while the other half would oppose it. Here, outlining scientific, historical, ethical, and philosophical aspects, we provide an integrated view explaining the reasons why biomedical research can hardly abandon laboratory animal testing. In this paper, we retrace the historical moments that mark the relationship between humans and other animal species. Then starting from Darwin's position on animal experimentation, we outline the steps that over time allowed the introduction of laws and rules that regulate animals' use in biomedical research. In our analysis, we present the perspectives of various authors, with the aim of delineating a theoretical framework within which to insert the ethical debate on laboratory animals research. Through the analysis of fundamental philosophical concepts and some practical examples, we propose a view according to which laboratory animals experimentation become ethically acceptable as far as it is guided by the goal of improving humans and other animal species (i.e., pets) life. Among the elements analyzed, there is the concept of responsibility that only active moral subjects (humans) have towards themselves and towards passive moral subjects (other animal species). We delineate the principle of cruelty that is useful to understand why research in laboratory animals should not be assimilated to a cruel act. Moreover, we touch upon the concepts of necessity and "good cause" to underline that, if biomedical research would have the possibility to avoid using animals, it would surely do that. To provide an example of the negative consequences occurring from not allowing laboratory animal research, we analyze the recent experience of Covid-19 epidemic. Finally, recalling the principle of "heuristics and biases" by Kahneman, we discuss why scientists should reconsider the way they are conveying information about their research to the general public.
Keywords: 3R principles; Covid-19; animal experimentation; animal rights; moral responsibility.
© 2020 Society for the Study of Addiction.
Publication types
- Historical Article
- Research Support, N.I.H., Extramural
- Animal Experimentation / ethics
- Animal Experimentation / history*
- Animal Rights
- Animal Use Alternatives
- Biomedical Research / ethics
- Biomedical Research / history*
- History, 17th Century
- History, 18th Century
- History, 19th Century
- History, 20th Century
- History, 21st Century
- History, Ancient
- Public Opinion / history*
Grants and funding
- R01 AA014351/AA/NIAAA NIH HHS/United States
- AA014351/AA/NIAAA NIH HHS/United States
- R37 AA017447/AA/NIAAA NIH HHS/United States
- R01 AA017447/AA/NIAAA NIH HHS/United States
- AA017447/AA/NIAAA NIH HHS/United States
Ethical care for research animals
WHY ANIMAL RESEARCH?
The use of animals in some forms of biomedical research remains essential to the discovery of the causes, diagnoses, and treatment of disease and suffering in humans and in animals., stanford shares the public's concern for laboratory research animals..
Many people have questions about animal testing ethics and the animal testing debate. We take our responsibility for the ethical treatment of animals in medical research very seriously. At Stanford, we emphasize that the humane care of laboratory animals is essential, both ethically and scientifically. Poor animal care is not good science. If animals are not well-treated, the science and knowledge they produce is not trustworthy and cannot be replicated, an important hallmark of the scientific method .
There are several reasons why the use of animals is critical for biomedical research:
• Animals are biologically very similar to humans. In fact, mice share more than 98% DNA with us!
• Animals are susceptible to many of the same health problems as humans – cancer, diabetes, heart disease, etc.
• With a shorter life cycle than humans, animal models can be studied throughout their whole life span and across several generations, a critical element in understanding how a disease processes and how it interacts with a whole, living biological system.
The ethics of animal experimentation
Nothing so far has been discovered that can be a substitute for the complex functions of a living, breathing, whole-organ system with pulmonary and circulatory structures like those in humans. Until such a discovery, animals must continue to play a critical role in helping researchers test potential new drugs and medical treatments for effectiveness and safety, and in identifying any undesired or dangerous side effects, such as infertility, birth defects, liver damage, toxicity, or cancer-causing potential.
U.S. federal laws require that non-human animal research occur to show the safety and efficacy of new treatments before any human research will be allowed to be conducted. Not only do we humans benefit from this research and testing, but hundreds of drugs and treatments developed for human use are now routinely used in veterinary clinics as well, helping animals live longer, healthier lives.
It is important to stress that 95% of all animals necessary for biomedical research in the United States are rodents – rats and mice especially bred for laboratory use – and that animals are only one part of the larger process of biomedical research.
Our researchers are strong supporters of animal welfare and view their work with animals in biomedical research as a privilege.
Stanford researchers are obligated to ensure the well-being of all animals in their care..
Stanford researchers are obligated to ensure the well-being of animals in their care, in strict adherence to the highest standards, and in accordance with federal and state laws, regulatory guidelines, and humane principles. They are also obligated to continuously update their animal-care practices based on the newest information and findings in the fields of laboratory animal care and husbandry.
Researchers requesting use of animal models at Stanford must have their research proposals reviewed by a federally mandated committee that includes two independent community members. It is only with this committee’s approval that research can begin. We at Stanford are dedicated to refining, reducing, and replacing animals in research whenever possible, and to using alternative methods (cell and tissue cultures, computer simulations, etc.) instead of or before animal studies are ever conducted.

Organizations and Resources
There are many outreach and advocacy organizations in the field of biomedical research.
- Learn more about outreach and advocacy organizations

Stanford Discoveries
What are the benefits of using animals in research? Stanford researchers have made many important human and animal life-saving discoveries through their work.
- Learn more about research discoveries at Stanford

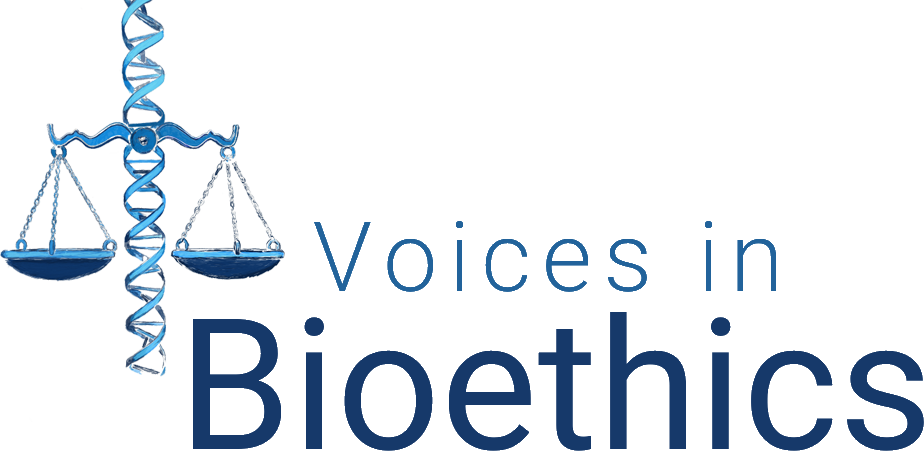
Extending Animal Cruelty Protections to Scientific Research
Article sidebar.
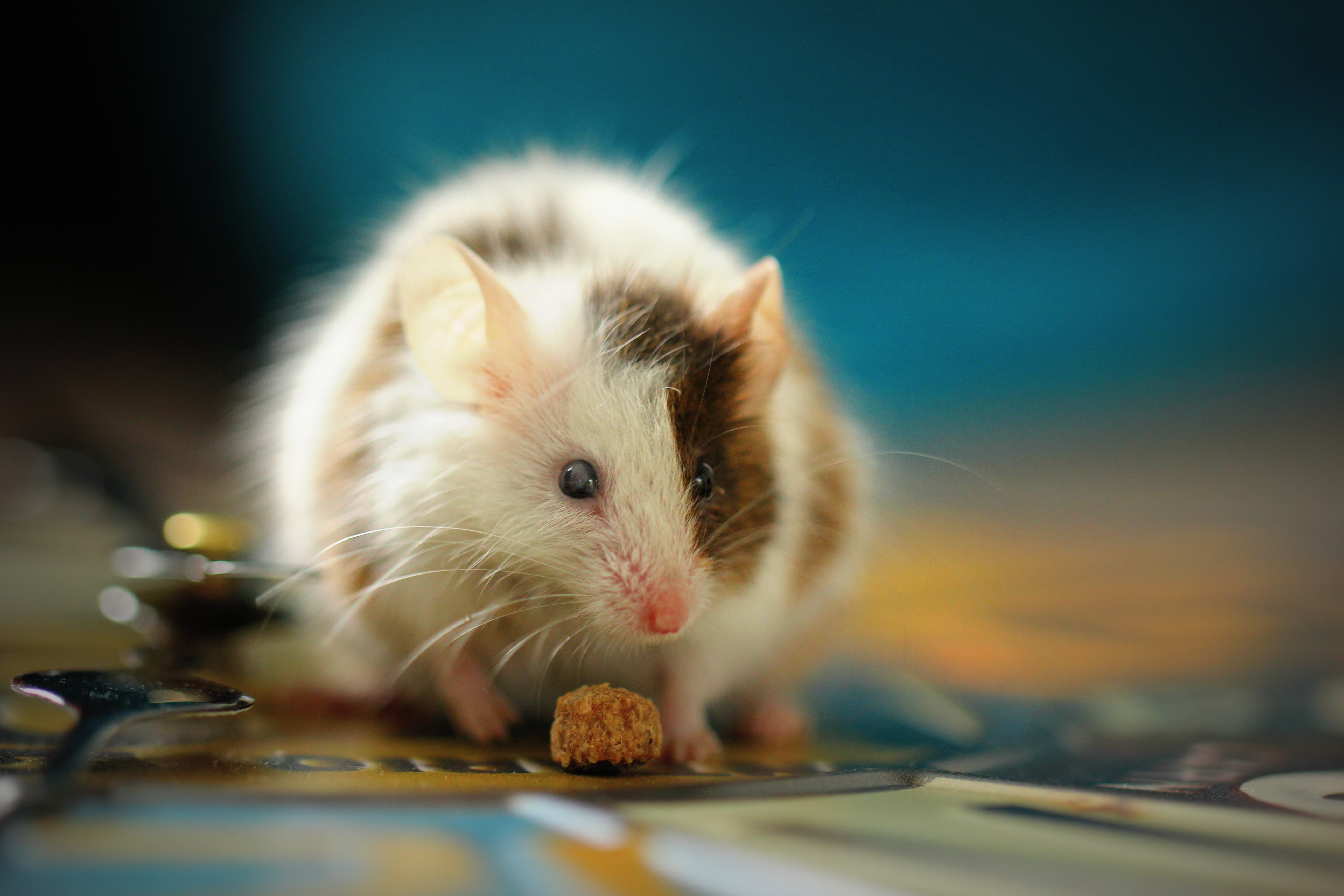
Main Article Content
Photo by Sandy Millar on Unsplash
INTRODUCTION
On November 25, 2019, the federal law H.R. 724 – the Preventing Animal Cruelty and Torture Act (PACT) prohibiting the intentional harm of “living non-human mammals, birds, reptiles, or amphibians” was signed. [1] This law was a notable step in extending protections, rights, and respect to animals. While many similar state laws existed, the passing of a federal law signaled a new shift in public tone. PACT is a declaration of growing societal sentiments that uphold the necessity to shield our fellow creatures from undue harm. Protecting animals from the harm of citizens is undoubtedly important, but PACT does nothing to protect animals from state-sanctioned harm, particularly in the form of research, which causes death and cruelty. It is time to extend and expand protections for animals used in research.
There is a long history of animal experimentation in the US, but no meaningful ethical protections of animals emerged until the 20 th century. Proscription of human experimentation and dissection led to animals bearing the brunt of harm for scientific and medical progress. For instance, English physician William Harvey discovered the heart did not continuously produce blood but instead recirculated it; he made this discovery by dissecting and bleeding out living dogs without anesthesia. [2] Experiments like this were considered ethically tenable for hundreds of years. Philosophers like Immanuel Kant, Thomas Aquinas, and Rene Descartes held that humans have no primary moral obligations to animals and that one should be concerned about the treatment of an animal only because it could indicate how one would treat a human. [3] During the 20 th century, as agriculture became more industrialized and government funding for animal research increased, the social demand for ethical regulations finally began to shift. In 1966, the Animal Welfare Act (Public Law 89-544) marked the first American federal legislation to protect laboratory animals, setting standards for use of animals in research. [4]
There has been progress in the field of animal research ethics since Harvey’s experiments, but much work remains. In the US alone, there are an estimated 20 million mice, fish, birds, and invertebrates used for animal research each year that are not regulated by the Animal Welfare Act. [5] Instead, the “3Rs Alternatives” approach (“reduce, replace, and refine”) [6] is one framework used to guide ethical treatment of animals not covered by federal protections. Unfortunately, unpacking the meaning and details of this approach only leads to ambiguity and minimal actionable guidance. For instance, an experimenter could reduce the number of animals used in research but subsequently increase the number of experiments conducted on the remaining animals. Replace could be used in the context of replacing one species with another. Refining is creating “any decrease in the severity of inhumane procedures applied to those animals, which still have to be used.” [7] The vague “ any ” implies that even a negligible minimization would be ethically acceptable. [8] An experimenter could technically follow each of the “3Rs” with minimal to no reduction in harm to the animals. One must also consider whether it is coherent to refer to guidelines as ethical when they inevitably produce pain, suffering, and death as consequences of research participation.
Other ethical guides like Humane Endpoints for Laboratory Animals Used in Regulatory Testing [9] encourage researchers to euthanize animals that undergo intractable pain or distress. This is a fate that an estimated one million animals face yearly in the US. [10] However, to use the word “humane” in this context contradicts the traditional meaning and undermines the integrity of the word. Taking living creatures, forcing them to experience intractable pain and suffering for human benefit, and killing them is the antithesis of what it means to be humane. During one of my Animal Ethics classes as a graduate student, our cohort visited an animal research facility to help inform our opinions on animal research. We observed one of the euthanasia chambers for lab mice – an enclosed metal lab bench with a sign above describing methods for euthanasia if CO 2 asphyxiation were to fail. The methods included decapitation, removal of vital organs, opening of the chest cavity, incision of major blood vessels, and cervical dislocation. [11] Behind us were rows and rows of see-through shoebox-sized containers housing five mice in each little box. Thousands of mice were packed together in this room for the sole purpose of breeding. If the mice were not the correct “type” for research, then they were “humanely” euthanized. “Humane,” in this context, has been deprived of its true meaning.
One can acknowledge that animal research was historically necessary for scientific progress, but those that currently claim these practices are still required must show empirically and undoubtedly this is true. As of now, this is not a settled issue. In the scientific community, there is contention about whether current animal research is actually applicable to humans. [12] Many drug researchers even view animal testing as a tedious barrier to development as it may be wholly irrelevant to the drug or medical device being tested. Since 1962, the FDA has required preclinical testing in animals; it is time to question whether this is necessary or helpful for drug development.
The scientific community should stop viewing animal testing as an unavoidable evil in the search for medical and technological innovation. PACT should be amended and extended to all animals and the FDA should modify the requirement for preclinical animal testing of all drugs and medical devices. It is time to encourage the scientific community to find alternative research methods that do not sacrifice our fellow animals. We use animals as test subjects because, in some sense, they resemble humans. But, if they are indeed like humans, they should receive similar protections. Science builds a better world for humans, but perhaps it is time for science to be more inclusive and build a better world for all creatures.
[1] Theodore E. Deutch, “Text - H.R.724 - 116th Congress (2019-2020): Preventing Animal Cruelty and Torture Act,” legislation, November 25, 2019, 2019/2020, https://www.congress.gov/bill/116th-congress/house-bill/724/text.
[2] Anita Guerrini, “Experiments, Causation, and the Uses of Vivisection in the First Half of the Seventeenth Century,” Journal of the History of Biology 46, no. 2 (2013): 227–54.
[3] Bernard E. Rollin, “The Regulation of Animal Research and the Emergence of Animal Ethics: A Conceptual History,” Theoretical Medicine and Bioethics 27, no. 4 (September 28, 2006): 285–304, https://doi.org/10.1007/s11017-006-9007-8; Darian M Ibrahim, “A Return to Descartes: Property, Profit, and the Corporate Ownership of Animals,” LAW AND CONTEMPORARY PROBLEMS 70 (n.d.): 28.
[4] Benjamin Adams and Jean Larson, “Legislative History of the Animal Welfare Act: Introduction | Animal Welfare Information Center| NAL | USDA,” accessed November 3, 2021, https://www.nal.usda.gov/awic/legislative-history-animal-welfare-act-introduction.
[5] National Research Council (US) and Institute of Medicine (US) Committee on the Use of Laboratory Animals in Biomedical and Behavioral Research, Patterns of Animal Use , Use of Laboratory Animals in Biomedical and Behavioral Research (National Academies Press (US), 1988), https://www.ncbi.nlm.nih.gov/books/NBK218261/.
[6] Robert C. Hubrecht and Elizabeth Carter, “The 3Rs and Humane Experimental Technique: Implementing Change,” Animals: An Open Access Journal from MDPI 9, no. 10 (September 30, 2019): 754, https://doi.org/10.3390/ani9100754.
[7] Hubrecht and Carter.
[8] Hubrecht and Carter.
[9] William S. Stokes, “Humane Endpoints for Laboratory Animals Used in Regulatory Testing,” ILAR Journal 43, no. Suppl_1 (January 1, 2002): S31–38, https://doi.org/10.1093/ilar.43.Suppl_1.S31.
[10] Stokes.
[11] “Euthanasia of Research Animals,” accessed April 21, 2022, https://services-web.research.uci.edu/compliance/animalcare-use/research-policies-and-guidance/euthanasia.html.
[12] Neal D. Barnard and Stephen R. Kaufman, “Animal Research Is Wasteful and Misleading,” Scientific American 276, no. 2 (1997): 80–82.
Chad Childers
MS Bioethics Candidate Harvard Medical School Center for Bioethics
Article Details

This work is licensed under a Creative Commons Attribution 4.0 International License .
- Share full article
Advertisement
Supported by
student opinion
Is Animal Testing Ever Justified?
The E.P.A. recently said it would move away from requiring the testing of potentially harmful chemicals on animals. Do you support the decision?

By Natalie Proulx
Find all our Student Opinion questions here.
On Sept. 10, the Environmental Protection Agency said it would move away from requiring the testing of potentially harmful chemicals on animals, a decision that was hailed by animal rights groups but criticized by environmentalists and researchers who said the practice was necessary to rigorously safeguard human health.
What are your thoughts on animal testing? Do you think it is ever justified? Why or why not?
In “ E.P.A. Says It Will Drastically Reduce Animal Testing ,” Mihir Zaveri, Mariel Padilla and Jaclyn Peiser write about the decision:
The E.P.A. Administrator Andrew Wheeler said the agency plans to reduce the amount of studies that involve mammal testing by 30 percent by 2025, and to eliminate the studies entirely by 2035, though some may still be approved on a case-by-case basis. The agency said it would also invest $4.25 million in projects at four universities and a medical center that are developing alternate ways of testing chemicals that do not involve animals. “We can protect human health and the environment by using cutting-edge, ethically sound science in our decision-making that efficiently and cost-effectively evaluates potential effects without animal testing,” Mr. Wheeler said in a memo announcing the changes. The E.P.A. has for decades required testing on a variety of animals — including rats, dogs, birds and fish — to gauge their toxicity before the chemicals can be bought, sold or used in the environment.
The article continues:
The practice of testing with animals has long prompted complex debates driven by passionate views on morality and scientific imperative. Reaction to Tuesday’s announcement was no different. “We are really excited as this has been something we’ve wanted for quite some time,” said Kitty Block, the president and chief executive of the Humane Society of the United States, an animal protection organization. “The alternatives are the future. They’re more efficient and save lives.” Kathleen Conlee, the vice president of animal research issues at the Humane Society, said the E.P.A.’s move is “broad-sweeping and significant.” “This is the first time a government agency has made such a commitment and timelined its specific goals along the way,” Ms. Conlee said. “There’s been a lot of positive action among other federal agencies, but we want to see all government agencies take this step.” Tracey Woodruff, a professor at the University of California, San Francisco’s school of medicine, said current alternatives to animal testing are somewhat useful. But Dr. Woodruff, who worked at the E.P.A. from 1994 to 2007, said only animal testing — a process honed over decades — was robust enough to gauge chemicals’ impacts on people of various ages, genetics and health backgrounds. “I definitely think we should be investing more in this research,” she said, referring to alternative testing. “But it’s really not ready for making decisions yet — at least the way that E.P.A. is making decisions.” Jennifer Sass, a senior scientist at Natural Resources Defense Council, an environmental advocacy group, said she was very concerned by the announcement. Dr. Sass said animals were still necessary to study chronic conditions, like cancer and infertility. Cells in a petri dish cannot yet replace whole living systems, she said. “The E.P.A.’s deadline is arbitrary,” Dr. Sass said. “Our interest isn’t in speed, it’s getting it right. We want proper animal testing because we don’t want harmful chemicals to end up in our food, air and water.”
Students, read the entire article, then tell us:
Do you support the decision by the E.P.A. to move away from requiring the testing of potentially harmful chemicals on animals? Or do you think animal testing is still necessary to regulate harmful substances that can have adverse effects on humans?
How important is it to you that the toxicity of chemicals and other environmental contaminants is rigorously studied and regulated? Why? Do you think not testing on animals hinders those efforts?
The Food and Drug Administration, the National Institutes of Health and the Department of Veterans Affairs are among the government agencies that still rely on animal testing. Do you think animal testing is important in these sectors or any others? Why or why not?
Do you think animal testing is ever justified? If so, what should be the criteria for when, how and on what animals testing is done?
Students 13 and older are invited to comment. All comments are moderated by the Learning Network staff, but please keep in mind that once your comment is accepted, it will be made public.
Natalie Proulx joined The Learning Network as a staff editor in 2017 after working as an English language arts teacher and curriculum writer. More about Natalie Proulx
105 Animal Testing Essay Topic Ideas & Examples
Looking for interesting animal testing topics to research and write about? This field is truly controversial and worth studying!
- 🌶️ Titles: Catchy & Creative
- 🐶 Essay: How to Write
- 🏆 Best Essay Examples
- 📌 Good Topics to Research
- 🎯 Most Interesting Topics to Write about
❓ Animal Testing Research Questions
In your animal testing essay, you might want to explore the historical or legal perspective, focus on the issue of animal rights, or discuss the advantages or disadvantages of animal testing in medicine, pharmacology, or cosmetic industry. We’ve gathered the most creative and catchy animal testing titles and added top animal testing essay examples. There are also useful tips on making and outline, formulating a thesis, and creating a hook sentence for your animal testing essay.
🌶️ Animal Testing Titles: Catchy & Creative
- What would life be like without animal testing?
- Animal testing: the cruelest experiments.
- AWA: why does not it protect all animals?
- What if animals experimented on humans?
- In the skin of a guinea pig: a narrative essay.
- Opposing animal testing: success stories.
- Animal-tested products: should they be destroyed?
- What have we gained from experiments on animals?
- Animal testing and cancer research: past and present.
🐶 Animal Testing Essay: How to Write
Animal testing has been an acute problem for a long time. Scientists and pharmaceutical firms use this approach to test cosmetics, foods, and other products people use daily.
Essays on animal testing are important because they highlight the significance of the problem. Writing outstanding animal testing essays requires extensive research and dedication.
We have prepared some do’s and don’ts for your excellent essay. But first, you should select a topic for your paper. Here are the examples of animal testing essay topics you can choose from:
- The question of animal intelligence from the perspective of animal testing
- Animal testing should (not) be banned
- How animal testing affects endangered species
- The history and consequences of animal testing
- The controversy associated with animal testing
- Animal Bill of Rights: Pros and cons
- Is animal testing necessary?
Remember that these animal testing essay titles are just the ideas for your paper. You are free to select other relevant titles and topics for discussion, too. Once you have selected the problem for your essay, you can start working on the paper. Here are some do’s of writing about animal testing:
- Do extensive preliminary research on the issue you have selected. You should be aware of all the problems associated with your questions, its causes, and consequences. Ask your professor about the sources you can use. Avoid relying on Wikipedia and personal blogs as your primary sources of information.
- Develop a well-organized outline and think of how you will structure your paper. Think of the main animal testing essay points and decide how you can present them in the paper. Remember to include introductory and concluding sections along with several body paragraphs.
- Start your paper with a hooking sentence. An animal testing essay hook should grab the reader’s attention. You can present an interesting question or statistics in this sentence.
- Include a well-defined thesis statement at the end of the introductory section.
- Your reader should understand the issue you are discussing. Explain what animal testing is, provide arguments for your position, and support them with evidence from your research.
- Discuss alternative perspectives on the issue if you are working on a persuasive essay. At the same time, you need to show that your opinion is more reliable than the opposing ones.
- Remember that your paper should not be offensive. Even if you criticize animal testing, stick to the formal language and provide evidence of why this practice is harmful.
There are some important points you should avoid while working on your paper. Here are some important don’ts to remember:
- Avoid making claims if you cannot reference them. Support your arguments with evidence from the literature or credible online sources even if you are writing an opinion piece. References will help the reader to understand that your viewpoint is reliable.
- Do not go over or below the word limit. Stick to your professor’s instructions.
- Avoid copying the essays you will find online. Your paper should be plagiarism-free.
- Avoid making crucial grammatical mistakes. Pay attention to the word choice and sentence structures. Check the paper several times before sending it for approval. If you are not sure whether your grammar is correct, ask a friend to look through the paper for you.
Do not forget to look at some of our free samples that will help you with your paper!
Animal Testing Hook Sentence
Your animal testing essay should start with a hook – an opening statement aiming to grab your reader’s attention. A good idea might be to use an impressive fact or statistics connected to experiments on animals:
- More than 100 million animals are killed in US laboratories each year.
- Animal Welfare Act (AWA) does not cover 99% animals used in experiments: according to it, rats, birds, reptiles, and fish are not animals.
- More than 50% adults in the US are against animal testing.
🏆 Best Animal Testing Essay Examples
- Animal Testing: Should Animal Testing Be Allowed? — Argumentative Essay It is crucial to agree that animal testing might be unethical phenomenon as argued by some groups; nonetheless, it should continue following its merits and contributions to the humankind in the realms of drug investigations […]
- Should Animals Be Used in Medical Research? It is therefore possible to use animals while testing the dangers and the toxicity of new drugs and by so doing; it is possible to protect human beings from the dangers that can emanate from […]
- Cosmetic Testing on Animals The surface of the skin or near the eyes of such animals is meant to simulate that of the average human and, as such, is one of easiest methods of determining whether are particular type […]
- The Debate on Animal Testing The purpose of this paper is to define animal testing within a historical context, establish ethical and legal issues surrounding the acts, discuss animal liberation movements, arguments in support and against the act of animal […]
- Animal Testing and Environmental Protection While the proponents of animal use in research argued that the sacrifice of animals’ lives is crucial for advancing the sphere of medicine, the argument this essay will defend relates to the availability of modern […]
- Negative Impacts of Animal Testing In many instances it can be proofed that drugs have been banned from the market after extensive research on animal testing and consuming a lot of cash, because of the dire effects that they cause […]
- Animal Experiments and Inhuman Treatment Although the results of such a laboratory may bring answers to many questions in medicine, genetics, and other vital spheres, it is frequently a case that the treatment of such animals is inhumane and cruel. […]
- Animal Testing in Medicine and Industry Animal testing is the inescapable reality of medicine and industry. However, between human suffering and animal suffering, the former is more important.
- Preclinical Testing on Animals The authors argue that despite the recent decline in the level of quality and transparency of preclinical trials, the scientific communities should always rely on animal testing before moving to human subjects and the subsequent […]
- Using Animals in Medical Research and Experiments While discussing the use of animals in medical research according to the consequentialist perspective, it is important to state that humans’ preferences cannot be counted higher to cause animals’ suffering; humans and animals’ preferences need […]
- Animal Testing: History and Arguments Nevertheless, that law was more focused on the welfare of animals in laboratories rather than on the prohibition of animal testing.
- Laboratory Experiments on Animals: Argument Against In some cases, the animals are not given any painkillers because their application may alter the effect of the medication which is investigated.
- Animal Testing From Medical and Ethical Viewpoints Striving to discover and explain the peculiarities of body functioning, already ancient Greeks and Romans resorted to vivisecting pigs; the scientific revolution of the Enlightenment era witnessed animal testing becoming the leading trend and a […]
- Negative Impacts of Animal Testing To alter these inhumane laws, we should organize a social movement aiming at the reconsideration of the role of animals in research and improvement of their positions.
- Animal Testing: Long and Unpretty History Nevertheless, that law was more focused on the welfare of animals in laboratories rather than on the prohibition of animal testing.
- Animal Testing as an Unnecessary and Atrocious Practice Such acts of violence could be partially excused by the necessity to test medications that are developed to save human lives however, this kind of testing is even more inhumane as it is ineffective in […]
- Animal Testing for Scientific Research Despite the fact that the present-day science makes no secret of the use of animals for research purposes, not many people know what deprivation, pain, and misery those animals have to experience in laboratories.
- Animal Testing and Ethics I believe it is also difficult to develop efficient legislation on the matter as people have different views on animal research and the line between ethical and unethical is blurred in this area.
- Animal Testing: History and Ethics Moreover, in the twelfth century, another Arabic physician, Avenzoar dissected animals and established animal testing experiment in testing surgical processes prior to their application to man. Trevan in 1927 to evaluate the effectiveness of digitalis […]
- Animal Testing Effects on Psychological Investigation In this context, ethical considerations remain a central theme in psychological research.”Ethics in research refers to the application of moral rules and professional codes of conduct to the collection, analysis, reporting, and publication of information […]
- Genetic Modification and Testing: Ethical Considerations It is done on a molecular level by synthesizing DNA, generating sequences and then inserting the received product into the organism which will be the carrier of the outcome. Another possibility is that the time […]
- Animal Testing: Why It Is Still Being Used The major reason for such “devotion” to animal testing can be explained by the fact that alternative sources of testing are insufficient and too inaccurate to replace conventional way of testing.
- Effects of Animal Testing and Alternatives Another challenge to the proponents of animal testing is related to dosage and the time line for a study. Animal rights values rebuff the notion that animals should have an importance to human beings in […]
- Ethics Problems in Animal Experimentation In spite of the fact that it is possible to find the arguments to support the idea of using animals in experiments, animal experimentation cannot be discussed as the ethical procedure because animals have the […]
- Animal Testing: Ethical Dilemmas in Business This means that both humans and animals have rights that need to be respected, and that is what brings about the many dilemmas that are experienced in this field.
- Should animals be used for scientific research? Therefore, considering the benefits that have been accrued from research activities due to use of animals in scientific research, I support that animals should be used in scientific research.
- Use of Animals in Research Testing: Ethical Justifications Involved The present paper argues that it is ethically justified to use animals in research settings if the goals of the research process are noble and oriented towards the advancement of human life.
- Ethical Problems in Animal Experimentation The banning of companies from testing on animals will force the manufacturers to use conventional methods to test their drugs and products.
- Utilitarianism for Animals: Testing and Experimentation There are alternatives in testing drugs such as tissue culture of human cells and hence this is bound to be more accurate in the findings.
- Use of Animals in Biological Testing Thus, these veterinarians have realized that the results that are realized from the animal research are very crucial in the improvement of the health of human being as well as that of animals.
- Medical Research on Animals Should be Forbidden by Law Vaccines and treatment regimes for various diseases that previously led to the death of humans were all discovered through research on animals.
- Experimentation on Animals However, critics of experimenting with animals argue that animals are subjected to a lot of pain and suffering in the course of coming up with scientific breakthroughs which in the long run may prove futile.
- Psychoactive Drug Testing on Animals The alterations in behavioral traits of animals due to psychoactive drugs are primarily attributed to the changes in the brain functions or inhibition of certain brain components in animals which ultimately translates to changes in […]
📌 Good Animal Testing Topics to Research
- Monkeys Don’t Like Wearing Makeup: Animal Testing In The Cosmetics Industry
- Animal Testing – Should Animal Experimentation Be Permitted
- Essay Animal Testing and In Vitro Testing as a Replacement
- Animal Testing : A Better Knowledge Of Human Body
- The Importance Of Animal Testing For Evaluating Consumer Safety
- The Issues on Animal Testing and the Alternative Procedures to Avoid the Use of the Inhuman Experimentation
- An Alternative to the Harsh and Unnecessary Practices of Animal Testing for Products, Drugs, Chemicals and Other Research
- The Unethical Use of Animals and the Need to Ban Animal Testing for Medical Research Purposes in the United States
- An Argument in Favor of Animal Testing for the Purpose of Clinical Research
- An Argument Against Animal Testing and the Banning of the Practice in the United States
- The Debate About the Ethics of Animal Testing and Its Effects on Us
- An Argument in Favor of Animal Testing as Beneficial to Human Health Research
- Animal Testing and the Reasons Why It Should Be Illegal
- The Principles of the Animal Testing From the Human Perspective
- The Ethical Issues on the Practice of Animal Testing to Test Cosmetics and Drugs
- Stopping Animal Testing and Vivisection by Passing a Bill against Animal Cruelty
🎯 Most Interesting Animal Testing Topics to Write about
- An Argument Against Animal Testing of Consumer Products and Drugs
- The Consequences and Unethical Practice of Animal Testing for Medical Training and Experiments
- How Do The Contributions Of Animal Testing To Global Medical
- Ways To Improve Animal Welfare After Premising The Animal Testing
- Animal Testing – Necessary or Barbaric and Wrong?
- Animal Testing And Its Impact On The Environment
- Animal Testing and Its Contribution to the Advancement of Medicine
- Cosmetics and Animal Testing: The Cause of Death and Mistreatment
- Animal Testing And People For The Ethical Treatment Of Animals
- Animal Rights Activists and the Controversial Issue of Animal Testing
- A History and the Types of Animal Testing in the Medical Area
- Argumentation on Medical Benefits of Animal Testing
- An Analysis of the Concept of Animal Testing Which Lowers the Standard of Human Life
- Is The Humane Society International Gave For Animal Testing
- A Discussion of Whether Animal Testing Is Good for Mankind or Violation of Rights
- The Ethics Of Animal Testing For Vaccine Development And Potential Alternatives
- The Good and Bad of Human Testing and Animal Testing
- What Should the Government Do About Animal Testing?
- Why Does Animal Testing Lower Our Standard of Living?
- Should Animals Be Used in Research?
- Why Should Animal Testing Be Accepted in the World?
- How Does Technology Impact Animal Testing?
- Why Should Animal Testing Be Illegal?
- Should Animal Testing Remain Legal?
- Why Should Animal Testing Be Banned?
- Can the Animal Testing Done to Find Cures for Diseases Be Humane?
- Does Animal Testing Really Work?
- Why Can’t Alternatives Like Computers Replace Research Animals?
- Should Animal Testing Continue to Test Cures for Human Diseases?
- How Does Animal Testing Effect Medicine?
- Should Animal Testing Continue or Be Stopped?
- What Are Advantages and Disadvantages of Animal Testing?
- Why Can Animal Testing Save Our Lives?
- Is Stem Cell Research Beginning of the End of Animal Testing?
- Do Beauty Products Suffer From Negative Publicity if They Conduct Trials on Animals?
- Should Medicine Trials Be Conducted?
- Can Results of Animal Testing Be Generalized to Adults?
- What Are the Origin and History of Animal Testing?
- Why Are Animals Needed to Screen Consumer Products for Safety When Products Tested by Alternative Methods, Are Available?
- How Much Does an Animal Suffer Due to Testing?
- What Is the Effectiveness of Animal Rights Groups in Stopping Animal Testing?
- How Do We Learn From Biomedical Research Using Animals?
- Who Cares for Animals in Research?
- How Do Laboratory Animal Science Professionals Feel About Their Work?
- Why Are There Increasing Numbers of Mice, Rats, and Fish Used in Research?
- How Can We Be Sure Lost or Stolen Pets Are Not Used in Research?
- Why Do Clinical Trials in Humans Require Prior Animal Testing?
- Chicago (A-D)
- Chicago (N-B)
IvyPanda. (2023, November 9). 105 Animal Testing Essay Topic Ideas & Examples. https://ivypanda.com/essays/topic/animal-testing-essay-examples/
"105 Animal Testing Essay Topic Ideas & Examples." IvyPanda , 9 Nov. 2023, ivypanda.com/essays/topic/animal-testing-essay-examples/.
IvyPanda . (2023) '105 Animal Testing Essay Topic Ideas & Examples'. 9 November.
IvyPanda . 2023. "105 Animal Testing Essay Topic Ideas & Examples." November 9, 2023. https://ivypanda.com/essays/topic/animal-testing-essay-examples/.
1. IvyPanda . "105 Animal Testing Essay Topic Ideas & Examples." November 9, 2023. https://ivypanda.com/essays/topic/animal-testing-essay-examples/.
Bibliography
IvyPanda . "105 Animal Testing Essay Topic Ideas & Examples." November 9, 2023. https://ivypanda.com/essays/topic/animal-testing-essay-examples/.
- Animal Abuse Research Topics
- Cruelty to Animals Titles
- Humanism Research Ideas
- Animal Ethics Research Ideas
- Meaning of Life Essay Ideas
- Animal Rights Research Ideas
- Cloning Questions
- Vegetarianism Essay Ideas
- Animal Welfare Ideas
- Bioethics Titles
- Wildlife Ideas
- Extinction Research Topics
- Hunting Questions
- Genetic Engineering Topics
- Zoo Research Ideas

An official website of the United States government
The .gov means it's official. Federal government websites often end in .gov or .mil. Before sharing sensitive information, make sure you're on a federal government site.
The site is secure. The https:// ensures that you are connecting to the official website and that any information you provide is encrypted and transmitted securely.
- Publications
- Account settings
- Browse Titles
NCBI Bookshelf. A service of the National Library of Medicine, National Institutes of Health.
National Research Council (US) and Institute of Medicine (US) Committee on the Use of Laboratory Animals in Biomedical and Behavioral Research. Use of Laboratory Animals in Biomedical and Behavioral Research. Washington (DC): National Academies Press (US); 1988.
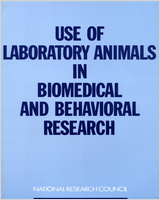
Use of Laboratory Animals in Biomedical and Behavioral Research.
- Hardcopy Version at National Academies Press
3 Benefits Derived from the Use of Animals
Animal studies have been an essential component of every field of medical research and have been crucial for the acquisition of basic knowledge in biology. In this chapter a few of the contributions of such studies in biomedical and behavioral research will be chronicled. These descriptions should be viewed within the context of the vast improvements in human health and understanding that have occurred in the past 150 years. For example, since 1900 the average life expectancy in the United States has increased by 25 years (U.S. National Center for Health Statistics, 1988). This remarkable increase cannot be attributed solely to animal research, as much of it is the result of improved hygiene and nutrition, but animal research has clearly been an important contributor to improved human health.
Despite the many advances and the projected results that will come through the use of animals, some individuals question the value of using animal models to study human disease, contending that the knowledge thus gained is insufficiently applicable to humans. Although experiments performed on humans would provide the most relevant information (and are used in clinical research conducted on humans when appropriate), it is not possible by commonly accepted ethical and moral standards or by law to perform most experiments on humans initially. It is true that not every experiment using animals yields immediate and practical results, but the advances that will be described in this chapter provide evidence that this means of research has contributed enormously to the well-being of humankind.
As a result of the acquisition of information and the development of techniques achieved through the use of animals, poliomyelitis is no longer a major threat to health in the United States. The number of cases of paralytic polio in the United States has declined as a result of vaccinations from 58,000 in 1952 to only 4 in 1984 (Office of Technology Assessment, 1986). Unfortunately, polio is still a major threat to health where the vaccine is not used. Indeed, in a number of African, Asian, and South American countries, the incidence of the disease has been rising, despite the availability of the vaccine (Cockburn and Drozdov, 1970). An estimated 500,000 cases occur around the world each year (Salk, 1983).
The use of rhesus monkeys for the study of polio began when Landsteiner and Popper (1909) showed that injection of spinal cord material from patients dying of polio caused paralysis in the animals. Flexner and Lewis (1909) promptly confirmed this result. To learn how to immunize monkeys to protect them against infection, researchers first used live virus, then formalin-inactivated virus from infected brain suspensions, and eventually modified live virus. A major breakthrough occurred when Enders, Weller, and Robbins (1949) showed that the virus could be propagated in cultured cells of non-neural origin. That set the stage for mass production of viruses that could be made into formalin-inactivated Salk vaccine or the modified live-virus Sabin vaccine (Salk, 1983).
Although the use of monkeys in polio research has decreased considerably, they are still essential to the production of both live and killed polio vaccines, which are routinely produced in monkey kidney cell cultures. The live vaccine is tested for neurovirulence in monkeys, and the killed vaccine is routinely tested for safety in monkeys.
- Acquired Immune Deficiency Syndrome
The recent emergence of acquired immune deficiency syndrome (AIDS) as a major health threat exemplifies not only the unpredictability of research needs, but also the criticality of animals in research. The similarity of simian AIDS, identified in rhesus monkeys at two primate centers, to human AIDS has allowed the disease in monkeys to serve as a model for the human disease. In monkeys, the virus that causes the disease has been isolated, infectibility studies have been conducted, and some experiments have provided preliminary indications of the possibility of vaccine development. This animal model might prove useful for testing the efficacy and safety of vaccines and therapeutic agents developed to prevent or treat the human disease (Institute of Medicine, 1986).
Recently, a new virus called feline T-lymphotropic lentivirus has been discovered. It resembles morphologically the human immunodeficiency virus (HIV) that causes AIDS, although differing antigenically, and causes a disease naturally in cats similar to AIDS. Thus, infected cats might prove useful as animal models for the study of certain aspects of human AIDS (Pedersen et al., 1987).
- Transplantation
The transplantation of skin, corneas, and various internal organs could not have become a safe and standard procedure without the knowledge of the biology of transplantation immunology acquired through the use of experimental animals. Some 30,000 Americans now alive have transplanted kidneys, and others survive with transplanted hearts and livers or retain their sight because of corneal transplants.
The treatment of burn victims was of particular importance to the British during World War II, and British biologist P. B. Medawar (1944) undertook to find relief for them through the transplantation of skin. For one of his models, he used freemartin cattle. A freemartin is a sexually maldeveloped female calf that is born as a twin of a normal male calf; male hormones that reach it through placental vessels usually make it sterile (Lillie, 1917). Experimentation showed that skin and other tissues could be transplanted with good, lasting success between the male and freemartin twins at any stage in their lives (Anderson et al., 1961). They were "tolerant" of each other's tissues because of prenatal exposure to each other's tissue antigens. Medawar and his colleagues sought to induce such tolerance in newborn mice. When newborns received skin transplants or received bone marrow from unrelated animals, they became forever "tolerant" of the new tissue (Brent et al., 1976). That discovery signaled a new era in immunology, with wide ramifications for health and the treatment of disease not only in humans, but also in animals.
Through a systematic study of the surface immune markers of specially bred strains of mice, Snell and Benacerraf provided the basis for much of the understanding that has led to the success of organ transplantation (Benacerraf, 1981).
In the past, young women with chronic pyelonephritis, patients with genetic polycystic disease, and people suffering from the aftermath of streptococcal infections were all vulnerable to chronic renal failure and death. Those people benefited from the invention of "artificial kidneys," which periodically washed blood and removed poisonous substances from it. The recipients of the benefit, however, had to undergo frequent, laborious, and uncomfortable procedures and had to rely on hospitals and mechanical devices.
The first extensive work with renal transplantation was reported in 1955 (Hume et al., 1955). At first, transplanted kidneys were rejected unless they were exchanged between identical twins. However, studies in dogs showed that administration of the drug 6-mercaptopurine after transplantation would prolong the survival of a transplanted organ from an unrelated person. This use of immunosuppressants ushered in the modern era of transplantation (Starzl and Holmes, 1964). These compounds, having been studied first in animals and proved to be effective, are now used in human transplant recipients.
The study of tissue antigens proceeded at the same time as transplantation work, first in mice and then in humans. Inbred (isogeneic) strains of mice had been created by repeated brother-sister matings. Ultimately, these strains became genetically identical, and the exchange of tissues and organs became possible. In the study of minor genetic differences between such strains, it became clear that some genes specify the cell-surface structures responsible for tissue recognition and rejection. "Transplantation antigens" can now be identified by tissue typing, and the most appropriate donors can be chosen for transplantation in both humans and animals.
A second revolution in transplantation was ushered in by the development of cyclosporin. This immunosuppressive agent was first used successfully in humans in 1983, after five years of toxicity and efficacy testing in mice, rats, and other animals. Since it became available for heart transplantation, survival after transplantation has improved significantly (Kupiec-Weglinski et al., 1984). Further progress is now occurring with monoclonal antibodies that seem to immobilize the cell-surface markers responsible for recognition and rejection. The hope is that such monoclonal antibodies, which have been developed and maintained in animals, will make it unnecessary to resort to complete immunosuppression of a transplant recipient. This would reduce the occurrence of infection and increase the rates of survival of transplanted organs.
- Cardiovascular-Renal Systems
Dogs have traditionally been used in cardiovascular-renal studies because of their relatively large size, which facilitates experimental procedures. For example, an early model of hypertension was produced by partially occluding the renal artery in dogs. Studies of renal function that use clearance techniques in unanesthetized animals are most often done in dogs. In the last two decades, however, some mutant rats have proved exceedingly valuable as animal models of human disease. The Brattleboro rat is an excellent example. It has diabetes insipidus and must drink 70 percent of its body weight in water each day. It cannot produce vasopressin, a hormone that plays an essential role in the kidneys' ability to regulate water excretion and blood pressure. Research on the Brattleboro rat has greatly increased our understanding of vasopressin's role in kidney and cardiovascular function, and that understanding might lead to the development of better drugs (and drugs with fewer side effects) for the treatment of clinical disorders (Sokol and Valtin, 1982).
The development of open-heart surgery is but one of many examples of the value of using laboratory animals. Working with cats and dogs, Gibbon built the forerunner of the present-day heart-lung machine (Deaton, 1974), which makes open-heart surgery possible. His research in the early 1930s included clamping off more and more of an animal's vasculature and detouring its blood through the heart-lung machine. The machine was further improved by the incorporation of a roller pump developed by DeBakey (DeBakey and Henly, 1961), which allowed the entire circulation to be shunted through the machine, which added oxygen to the animal's blood. The pump was first used and perfected in the animal laboratory and is now a standard, essential component of the heart-lung machine. As a result of these developments, more than 80 percent of infants born with congenital cardiac abnormalities now can be treated surgically and can lead normal lives.
Replacement of heart valves and segments of large arteries in the treatment of valvular heart disease was made feasible by dog studies done in the late 1940s and early 1950s (Gay, 1984). Before diseased heart valves could be replaced in patients, scientists had to study their design and use in animals. As with so many other drugs and operations, physicians and surgeons would not consider applying them to patients until they had proved safe and effective in animals, nor would the public accept them until their safety was proved. Each decade since then has seen improvements in the design, installation, and performance of these valves and other prosthetic devices. Because the ideal valve has not yet been developed, research is still in progress in many laboratories to further improve its capacities.
- Nervous System
The human brain is a structure of extraordinary complexity. Each of its 200 billion neurons (nerve cells) makes a few thousand to several hundred thousand connections with other neurons, muscles, or glands. Neurons use large amounts of metabolic energy to carry out a host of functions: the generation and conduction of impulses; the synthesis, transport, secretion, and uptake of transmitters; and the modification of structure and synaptic efficacy in response to activity and environmental perturbations (Kandel and Schwartz, 1985).
Many basic aspects of neuronal development can be studied in cell and tissue cultures, in brain slices, and in simple invertebrate neuronal systems. Computer simulations and noninvasive human studies can also provide important data on fundamental mechanisms of learning and memory. Yet there is no adequate substitute for animal studies in attempts to understand the complex behavioral and cognitive functions of the brain in health and disease.
Movement and Function
Our understanding of the nervous system and approaches to rational therapy of its disorders could not have come about without animal studies initiated by the physiologist Charles Sherrington (Eccles and Gibson, 1979). His studies on reflex mechanisms of the spinal cord in cats were continued by Eccles (1957), who described how excitatory and inhibitory processes work in the central nervous system. Today, neurosurgeons can remove some brain tumors with minimal damage to the motor system in part because scientists such as Sherrington discovered that localized electrical stimulation of the exposed brain of the dog could elicit discrete movements of the limbs.
Neurologists and neurosurgeons now examine electrical signals from the brain to diagnose and treat epilepsy, study levels of consciousness, localize brain tumors, diagnose multiple sclerosis, and study learning disabilities in children. Moreover, the applications of such essential tools for diagnosis and therapy as computed axial tomographic (CAT) scans and magnetic resonance imaging (MRI) were developed with research animals (Kandel and Schwartz, 1985).
The study of the nervous system and behavior is one of the major frontiers of modern science. A good deal is known about the anatomy and physiology of the brain and nervous system, but much remains to be learned about it as an organized assemblage of neurons and about how it is affected by environmental stimulation. The following examples provide an idea of how animals are used in studies of such subjects.
Postnatal Development of the Visual Cortex and the Influence of Environment
Hubel and Wiesel shared the Nobel Prize in 1981 for their studies of vision in cats and monkeys, including the development of visual functions in young animals (Barlow, 1982). The visual cortex of monkeys is not fully developed at birth; nerve cells are still growing and making connections with other nerve cells. In this process, normal development depends on visual stimulation during a critical period in early postnatal life.
As in humans, each eye of a monkey sees a slightly different view of the same object; normal binocular vision gives the impression of depth. If early in postnatal life one eye is occluded, the nerve cells for that eye in the visual cortex do not develop normally. Most of the nerve cells become responsive only to the open eye, as shown in recordings from cells of the visual cortex of anesthetized animals. In normal development, the visual cortex consists of alternating bands of reactive neurons from the right and left eyes; but in a monkey with an occluded eye, the regular alternation is weakened, and most neurons react only to the normal eye. These anatomical and physiological changes are the basis of blindness in the occluded eye.
Children with congenital cataracts or clouding of the ocular media for other reasons demonstrate a similar dependence of human vision on visual stimulation. Testing after restoration of normal vision has shown that the acuity of the previously occluded eye is reduced; the earlier in life the eye was occluded, the greater the degree of deficit. Animal experiments have also shown that correction of strabismus (squint) by surgery should be performed early in, or certainly before the end of, the critical period of eye-brain development to ensure normal vision (Wiesel, 1982).
The close correlation between the effects of visual deprivation observed in animals and the effects observed in the clinic suggests that they are based on similar physiological mechanisms. This correlation has been helpful in developing appropriate measures of prevention and treatment of neural eye disorders.
Another subject of behavioral research is memory. An estimated 5 percent of people over the age of 65 have severe limitations or even failures of memory and cognition; another 10 percent of the people over 65 have mild to moderate cognitive problems (Coyle et al., 1985). Specific conditions, such as Korsakoff's syndrome and Alzheimer's disease, affect mental functions and can cause extreme memory loss. Research on animals is improving the understanding of the mechanisms of such losses. In turn, this increased understanding has led to the discovery of some drugs that show promise of counteracting the losses. Most of the knowledge about the neurotransmitters involved in these diseases has also been derived from studies of the brains and nervous systems of animals.
Primates are phylogenetically closer to humans than are other mammals. Their behavioral capabilities are in keeping with the greater development and complexity of their brains. Primates also have age-related decrements in memory function. Generally, memory impairment with advancing age first appears as a failure of immediate memory, the recall of events that have just occurred. Transmitter chemicals of the α-adrenergic class, like clonidine, were first found to improve memory performance in macaques and aged rodents. Clonidine has now also proved effective in improving the memory of patients with Korsakoff's syndrome. Those findings suggest a new approach to the treatment of patients with memory disorders, and they have provided a new option for clinical trials with patients suffering from Alzheimer's disease (Arnsten and Goldman-Rakic, 1985).
Pain is a common symptom of disease in humans and animals. It is important that medical science develop more effective methods of pain management than are now available. Much pharmacological research has focused on the production of drugs with potent analgesic properties, and much research on pain—particularly that concerned with analgesics, acupuncture efficacy, hypnosis, and so on—has been carried out on human subjects for over a century. Research using animals is necessary, however, if unsolved problems are to be adequately addressed.
Although many experiments that study pain must involve pain for the animal, researchers have developed methods that are as humane as possible within the context of the experiment. For example, the slightest reflex movement of the tail of a rat or mouse is objective evidence that a noxious stimulus applied to the skin of the tail has attained threshold intensity. Reflex behavior, such as the tail-flick, is a useful index of the comparative effectiveness of analgesics, as well as of the effects of manipulating chemical messengers in the central pain pathways (Willis, 1985).
The understanding of intrinsic brain mechanisms of pain and its modification will require the use of modern techniques for cell marking and pathway tracing, immunocytochemical and microphysiological methods, and sophisticated behavioral studies. Paradoxically, many investigations of pain can be explored in anesthetized animals. Thanks to psychophysical studies in humans that were replicated in animals, neuroscientists have been able to trace the nerve fibers from skin, muscle, and internal organs that are specific carriers of ''pain signals.'' With such a powerful handle on the input end of the pain system, the passage and transformation of pain signals can be explored in complex neuronal organizations in anesthetized animals. It is also possible to study the central systems that control the passage of pain signals to higher levels of the central nervous system. Finally, isolation and identification of the transmitters, structure, and other components of the neurochemical machinery of the brain involved in pain perception and its modification can be elucidated (Willis, 1985).
Increasing recognition that behavioral factors play a significant role in many current health problems—for example, drugs and alcohol abuse, eating disorders, effects of stress, cardiovascular disease, and mental and psychiatric ailments—has led to the development of animal models for experimental and biological analysis as part of the emerging field of behavioral medicine (Hamburg et al., 1982).
- Other Benefits for Humans
The preceding examples provide a sampling of the contributions that research using animals has made to the improvement of human health and the acquisition of knowledge. Many others could be cited—for example, the development of medicinals such as the sulfonamides (Hubbard, 1976); the development of life-support systems for premature infants (Coalson et al., 1982; deLemos et al., 1985; Escobedo et al., 1982); and the increase in understanding of learning (Miller, 1985; Pavlov, 1927; Skinner, 1938; Thorndike, 1898), nonlinguistic communication (Gardner and Gardner, 1969; Romski et al., 1984), drug abuse (Deneau et al., 1969; National Institute of Drug Abuse, 1984; Seevers, 1968), and nervous system regeneration. Many examples of such benefits are also chronicled in publications such as those by Gay (1986), Leader and Stark (1987), and Paton (1984).
- Benefits for Animals
One might have the impression that animal research is conducted only with the aim of alleviating human suffering. The conduct of extensive research in veterinary schools and other institutions indicates that that is not the case. Most research on domestic farm animals is undertaken to increase the productivity and quality of animal products. Research is also undertaken to reduce the suffering and increase the overall well-being of animals, particularly companion animals. Examples include current research on Potomac fever in horses, the development of ivermectin to eradicate parasitic diseases in a variety of animals, and the development of vaccines for feline leukemia virus and canine parvovirus.
Research aimed at human illnesses has also had immeasurable benefits for animals. A host of immunizations and antibiotics have proven applicable to the therapy of animal diseases (Paton, 1984). Kidney transplantation, cardiovascular treatments, chemotherapeutics, and narcotics are widely applicable, as are the insights gained from genetic research (Gorman, 1988).
One example of the benefits of biomedical research for animals can be found in the propagation of endangered species. The ability to transfer embryos, eliminate parasitism, treat illnesses, and use anesthetic advances has improved the health and survival of many species. The knowledge gained from genetic studies has allowed appropriate management of species that are endangered or have disappeared in the wild. For example, the ability to identify the sex of birds has been essential in the management of the whooping crane and the California condor. Research into obstacles to successful breeding in captivity has markedly reduced the need for importation of many species, especially monkeys. For example, among nonhuman primate species used in research, there were 7,908 births in 1984 in the United States, compared with 2,198 in 1973 (Johnsen and Whitehair, 1986).
Animal research has resulted in enormous benefits for humans and animals. The searching and systematic methods of scientific inquiry have greatly reduced the incidence of human disease and have substantially increased life expectancy. Those results have come largely through experimental methods based in part on the use of animals, as illustrated by the many examples cited in this chapter.
At the same time, much obviously remains to be learned. Further studies in such areas as cancer, heart disease, diabetes, AIDS, dementias, and the development of vaccines and chemotherapeutic agents will continue to require the use of animals.
- Cite this Page National Research Council (US) and Institute of Medicine (US) Committee on the Use of Laboratory Animals in Biomedical and Behavioral Research. Use of Laboratory Animals in Biomedical and Behavioral Research. Washington (DC): National Academies Press (US); 1988. 3, Benefits Derived from the Use of Animals.
- PDF version of this title (664K)
In this Page
Recent activity.
- Benefits Derived from the Use of Animals - Use of Laboratory Animals in Biomedic... Benefits Derived from the Use of Animals - Use of Laboratory Animals in Biomedical and Behavioral Research
Your browsing activity is empty.
Activity recording is turned off.
Turn recording back on
Connect with NLM
National Library of Medicine 8600 Rockville Pike Bethesda, MD 20894
Web Policies FOIA HHS Vulnerability Disclosure
Help Accessibility Careers
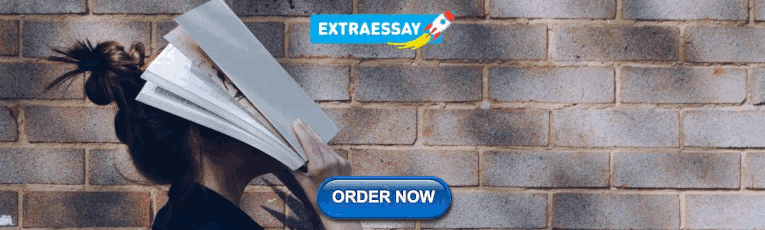
IMAGES
VIDEO
COMMENTS
Introduction. Annually, more than 115 million animals are used worldwide in experimentation or to supply the biomedical industry. 1 Nonhuman animal (hereafter "animal") experimentation falls under two categories: basic (i.e., investigation of basic biology and human disease) and applied (i.e., drug research and development and toxicity and safety testing).
Genzel et al. , in particular, take issue with the proposal for a European ban on animal testing. Finally, there is a danger in bypassing animal research in developing new vaccines for diseases such as COVID-19 . The purpose of this paper is to show that, while animal research is necessary for the health of both humans and animals, there is a ...
Opposing Viewpoints Online Collection, Gale, 2021. Animal experimentation, also called animal testing, has contributed to many important scientific and medical discoveries. Breakthroughs include the development of many antibiotics, insulin therapy for diabetes, modern anesthesia, vaccines for whooping cough and other diseases, the use of ...
According to the UK Home Office ( 12 ), in the year 2016, 48.6% of the animal tests in medical research were conducted for genetically oriented studies. Moreover, 28.5% of the medical research involving animal testing was for basic biological research, 13.5% was for regulatory. testing, 8.6% was for translating research from animals to humans ...
animals in research has been changing based on the latest. FIGURE 3 Use of inferior organisms as an alternative method to. the use of vertebrate animals. A nimAl experimentAtion: A look into ...
Ethical Considerations and Advances in the Understanding of Animal Cognition. Apprehension around burgeoning medical research in the late 1800s and the first half of the 20 th century sparked concerns over the use of humans and animals in research , .Suspicions around the use of humans were deepened with the revelation of several exploitive research projects, including a series of medical ...
These requirements have established animal tests as a paradigm, and as a result it is hard to make a move toward alternative testing methods. Yet, in the second half of the 20th century, and especially so far in the 21st century, changes in public opinion have been witnessed due to growing concerns about the use of laboratory animals.
Various groups including animal protection organizations, medical organizations, research centers, and even federal agencies such as the U.S. Food and Drug Administration, are working to minimize animal use in scientific experiments. This movement primarily stems from animal welfare and ethical concerns. However, recent advances in technology and new studies in medicine have contributed to an ...
Animal-based testing has been the gold standard for research for decades, and it remains an important requirement for establishing the safety and efficacy of products being brought to market today.
Medical research. Nature Reviews Materials speaks to Donald Ingber, Founding Director of the Wyss Institute for Biologically Inspired Engineering at Harvard University, about the animal testing ...
The use of laboratory animals in biomedical research is a matter of intense public debate. Recent statistics indicates that about half of the western population, sensitive to this discussion, would be in favor of animal testing while the other half would oppose it. Here, outlining scientific, historical, ethical, and philosophical aspects, we ...
There are several reasons why the use of animals is critical for biomedical research: • Animals are biologically very similar to humans. In fact, mice share more than 98% DNA with us! • Animals are susceptible to many of the same health problems as humans - cancer, diabetes, heart disease, etc. • With a shorter life cycle than humans ...
Abstract. Animal testing in the cosmetic industry is still practiced daily by several companies. across the world subjecting animals to painful and cruel tests. The negative impacts of. animal ...
The phrase "animal testing" refers to the range of experiments performed on living animals for the purpose of studying diseases and biology, the effectiveness of newly developed pharmaceuticals and medications, and the safety of consumer products like cosmetics, cleaners, and food additives. In the context of biomedical research, animal ...
animal testing is the application of a. research idea, by specific techniques, on. a number of animals, in a time interval, in a specific area, which ends with one or. more conclusions. (Fig.1 ...
On November 25, 2019, the federal law H.R. 724 - the Preventing Animal Cruelty and Torture Act (PACT) prohibiting the intentional harm of "living non-human mammals, birds, reptiles, or amphibians" was signed. [1] This law was a notable step in extending protections, rights, and respect to animals. While many similar state laws existed ...
Abstract. The use of non-human animals in biomedical research has given important contributions to the medical progress achieved in our day, but it has also been a cause of heated public, scientific and philosophical discussion for hundreds of years. This review, with a mainly European outlook, addresses the history of animal use in biomedical ...
Testing for skin irritation and corrosion closely parallels the Draize eye test. The test substance is applied to a rabbit's shaved skin, then removed after four hours. The skin is observed for up to 14 days. Today this test can be fully replaced with what is known as a nonanimal tiered ap-proach.
The E.P.A. Administrator Andrew Wheeler said the agency plans to reduce the amount of studies that involve mammal testing by 30 percent by 2025, and to eliminate the studies entirely by 2035 ...
For comparison, a USDA/APHIS census estimated that a total of 17-22 million animals were used in research and testing in the US in 1983 (US Congress, Office of Technology Assessment 1986).However, a more recent independent estimate suggests up to 80 million animals used, in part due to the advent of transgenic animals (Carbone 2004).The USDA publishes annual reports on animal usage in ...
Using animals in research and to test the safety of products has been a topic of heated debate for decades. According to data collected by F. Barbara Orlans for her book, In the Name of Science: Issues in Responsible Animal Experimentation, sixty percent of all animals used in testing are used in biomedical research and product-safety testing (62). ). People have different feelings for animals ...
Nutricia Research also set up a joint project on the use of these models with the University of Clermont-Ferrand in France. In addition, Danone Nutricia Research has developed one in vitro gastrointestinal model to simulate the upper tract for internal use. These models are widely used in our research on food, reducing the need for animal testing.
Essays on animal testing are important because they highlight the significance of the problem. Writing outstanding animal testing essays requires extensive research and dedication. We have prepared some do's and don'ts for your excellent essay. But first, you should select a topic for your paper.
Animal studies have been an essential component of every field of medical research and have been crucial for the acquisition of basic knowledge in biology. In this chapter a few of the contributions of such studies in biomedical and behavioral research will be chronicled. These descriptions should be viewed within the context of the vast improvements in human health and understanding that have ...