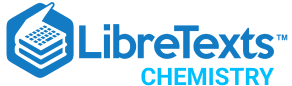
- school Campus Bookshelves
- menu_book Bookshelves
- perm_media Learning Objects
- login Login
- how_to_reg Request Instructor Account
- hub Instructor Commons
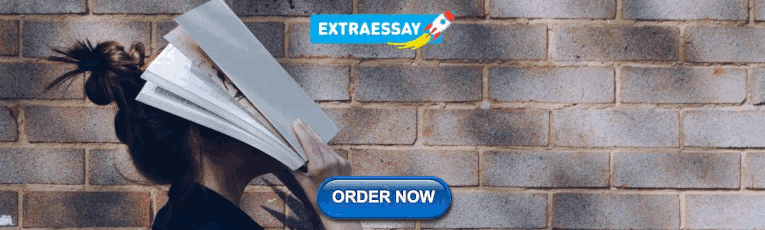
Margin Size
- Download Page (PDF)
- Download Full Book (PDF)
- Periodic Table
- Physics Constants
- Scientific Calculator
- Reference & Cite
- Tools expand_more
- Readability
selected template will load here
This action is not available.
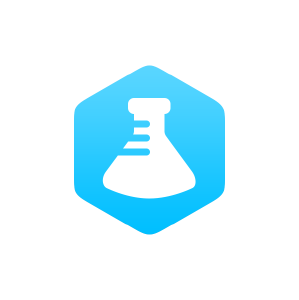
Infrared Spectroscopy Absorption Table
- Last updated
- Save as PDF
- Page ID 22645
The following table lists infrared spectroscopy absorptions by frequency regions.
Contributors and Attributions
- OChemOnline
Near-Infrared Spectroscopy
Cite this chapter.
- Frank S. Parker 2
684 Accesses
2 Citations
In the near-infrared region the absorption process is associated with the overtone and combination bands of vibrational transitions. This region can be studied with the same equipment that is used for electronic spectra, apart from changing from photocell to photoconductive detectors above 1 µ . A review by Kaye (1954; see also, 1955) defines the near-infrared region to be from 0.7 µ to 3.5 µ , and quartz prisms are usable over much of this range. Goddu (1960), Wheeler (1959), McCallum (1964), and Whetsel (1968) have more recently presented reviews of near-infrared spectrophotometry. Wheeler’s is a comprehensive review with references to near-infrared spectra of somewhere near 500 organic compounds of many classes. Whetsel’s review also contains extensive references to organic applications.
This is a preview of subscription content, log in via an institution to check access.
Access this chapter
- Available as PDF
- Read on any device
- Instant download
- Own it forever
- Compact, lightweight edition
- Dispatched in 3 to 5 business days
- Free shipping worldwide - see info
Tax calculation will be finalised at checkout
Purchases are for personal use only
Institutional subscriptions
Unable to display preview. Download preview PDF.
Bakerman, S. and Mitchell, C., Nature 187 , 1033 (1960).
Article CAS Google Scholar
Berger, A., Loewenstein, A., and Meiboom, S., J. Am. Chem. Soc. 81 , 62 (1959).
Besic, F. C., Zimmerman, S. O., and Wiemann, M. R., Jr., J. Dental. Res. 41 , 718 (1962).
Brill, A. S. and Sandberg, H. E., Biophys. J. 8 , 669 (1968).
Chance, B. in Biochemistry of Copper ( J. Peisach, P. Aisen, and W. E. Blumberg, eds.). Academic Press, New York, 1966, p. 293.
Google Scholar
Crowell, E. P., Kuhn, W. F., Resnik, F. E., and Varsel, C. J., Tobacco, New York 152 , 20 (1961).
Day, P., Scregg, G., and Williams, R. J. P., Biopolymers Symp . 1 , 271 (1964).
Day, P., Smith, D. W., and Williams, R. J. P., Biochemistry 6 , 1563 (1967a).
Day, P., Smith, D. W., and Williams, R. J. P., Biochemistry 6 , 3747 (1967b).
Dayhoff, M. O., Perlmann, G. E., and MacInnes, D. A., J. Am. Chem. Soc. 74 , 2515 (1952).
Ehrenberg, A. and Hemmerich, P., Acta Chem. Scand. 18 , 1320 (1964).
Franzen, J. S. and Stephens, R. E., Biochemistry 2 , 1321 (1963).
Gibson, Q. H. and Greenwood, C., J. Biol. Chem. 240 , 2694 (1965).
CAS Google Scholar
Goddu, R. F. in Advances in Analytical Chemistry and Instrumentation, Vol . 1 ( C. N. Reilley, ed.) Interscience, New York, 1960, p. 347.
Griffiths, D. E. and Wharton, D. C., J. Biol. Chem. 236 , 1850 (1961).
Hanlon, S., Biochemistry 5 , 2049 (1966).
Hanlon, S. and Klotz, I. M., Biochemistry 4 , 37 (1965).
Hanlon, S. and Klotz, I. M. “Near Infrared Spectroscopy in Structural Problems of Biochemistry,” in Developments in Applied Spectroscopy, Vol . 6 ( W. K. Baer, A. J. Perkins, and E. L. Grove, eds.). Plenum Press, New York, 1968, p. 219.
Hanlon, S., Russo, S. F., and Klotz, I. M., J. Am. Chem. Soc. 85 , 2024 (1963).
Hart, J. R., Norris, K. H., and Golumbic, C., Cereal Chemistry 39 , 94 (1962).
Hayer, H. Z., Elektrochem . 47 , 451 (1941).
Holman, R. T. and Edmondson, P. R., Anal. Chem. 28 , 1533 (1956).
Kauffman, F. L., J. Am. Oil Chemists’ Soc. 41 , 4 (1964).
Kaye, W., Spectrochim. Acta 6 , 257 (1954).
Kaye, W., Spectrochim. Acta 7 , 181 (1955).
Klotz, I. M. and Frank, B. H., J. Am. Chem. Soc. 87 , 2721 (1965).
Klotz, I. M. and Franzen, J. S., J. Am. Chem. Soc. 84 , 3461 (1962).
Klotz, I. M. and Mueller, D. D., Biochemistry 8 , 12 (1969).
Klotz, I. M. and Russo, S. F. in Protides of the Biological Fluids, Vol . 14 ( H. Peeters, ed.), Elsevier Publ. Co., Amsterdam, 1966, p. 427.
Klotz, I. M., Russo, S. F., Hanlon, S., and Stake, M. A., J. Am. Chem. Soc. 86 , 4774 (1964).
Kotani, M. in Advances in Chemical Physics, Vol. 7: The Structure and Properties of Biomolecules and Biological Systems ( J. Duchesne, ed.), Interscience, New York, 1964, p. 159.
McCabe, W. C. and Fisher, H. F., Nature 207 , 1274 (1965).
McCallum, J. D. in Progress in Infrared Spectroscopy, Vol . 2 ( H. A. Szymanski, ed.) Plenum Press, New York, 1964, p. 227.
Mizushima, S., Simanouti, T., Nagakura, S., Kuratani, K., Tsuboi, M., Baba, H., and Fujioka, O., J. Am. Chem. Soc. 72 , 3490 (1950).
Murthy, A. S. N. and Rao, C. N. R., “Hydrogen Bonding,” Tech. Report No. 1 of the Department of Chemistry , Indian Institute of Technology, Kanpur, India, 1964.
Murthy, A. S. N. and Rao, C. N. R., Appl. Spectrosc. Rev. 2 , 69 (1968).
Pimentel, G. C. and McClellan, A. L., The Hydrogen Bond , W. H. Freeman, San Francisco, 1960.
Scarpa, J. S., Mueller, D. D., and Klotz, I. M., J. Am. Chem. Soc. 89 , 6024 (1967).
Schejter, A. and George, P., Biochemistry 3 , 1045 (1964).
Smith, L. and Stotz, E., J. Biol. Chem. 209 , 819 (1954).
Sophianopoulos, A. J., Rhodes, C. K., Holcomb, D. N., and Van Holde, K. E., J. Biol. Chem. 237 , 1107 (1962).
Susi, H., Timasheff, S. N., and Ard, J. S., J. Biol. Chem. 239 , 3051 (1964).
Wharton, D. C. and Tzagoloff, A., J. Biol. Chem. 239 , 2036 (1964).
Wheeler, O. H., Chem. Revs. 59 , 629 (1959).
Whetsel, K. B., Appl. Spectrosc. Rev. 2 , 1 (1968).
Willis, H. A. and Miller, R. G. J. in Molecular Spectroscopy (G. H. Beaven, E. A. Johnson, H. A. Willis, and R. G. J. Miller), Heywood and Company, Ltd., London, 1961, p. 171.
Wilson, D. F., Arch. Biochem. Biophys. 122 , 254 (1967).
Download references
Author information
Authors and affiliations.
Department of Biochemistry, New York Medical College, New York, New York, USA
Frank S. Parker
You can also search for this author in PubMed Google Scholar
Rights and permissions
Reprints and permissions
Copyright information
© 1971 Plenum Press, New York
About this chapter
Parker, F.S. (1971). Near-Infrared Spectroscopy. In: Applications of Infrared Spectroscopy in Biochemistry, Biology, and Medicine. Springer, Boston, MA. https://doi.org/10.1007/978-1-4684-1872-9_2
Download citation
DOI : https://doi.org/10.1007/978-1-4684-1872-9_2
Publisher Name : Springer, Boston, MA
Print ISBN : 978-1-4684-1874-3
Online ISBN : 978-1-4684-1872-9
eBook Packages : Springer Book Archive
Share this chapter
Anyone you share the following link with will be able to read this content:
Sorry, a shareable link is not currently available for this article.
Provided by the Springer Nature SharedIt content-sharing initiative
- Publish with us
Policies and ethics
- Find a journal
- Track your research
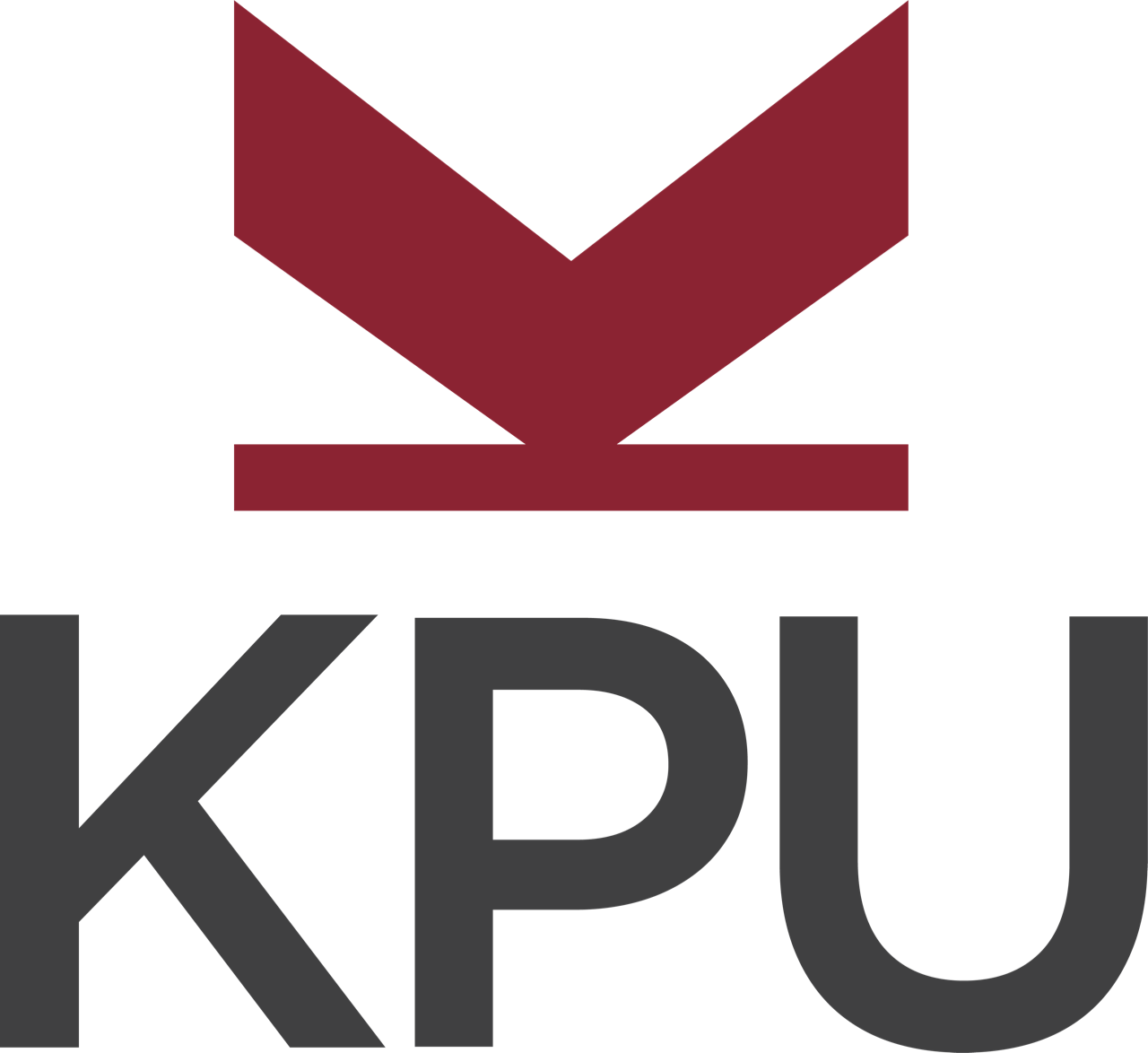
Want to create or adapt books like this? Learn more about how Pressbooks supports open publishing practices.
Chapter 6: Structural Identification of Organic Compounds: IR and NMR Spectroscopy
6.3 IR Spectrum and Characteristic Absorption Bands
With a basic understanding of IR theory, we will now take a look at the actual output from IR spectroscopy experiments and learn how to get structural information from the IR spectrum. Below is the IR spectrum for 2-hexanone.
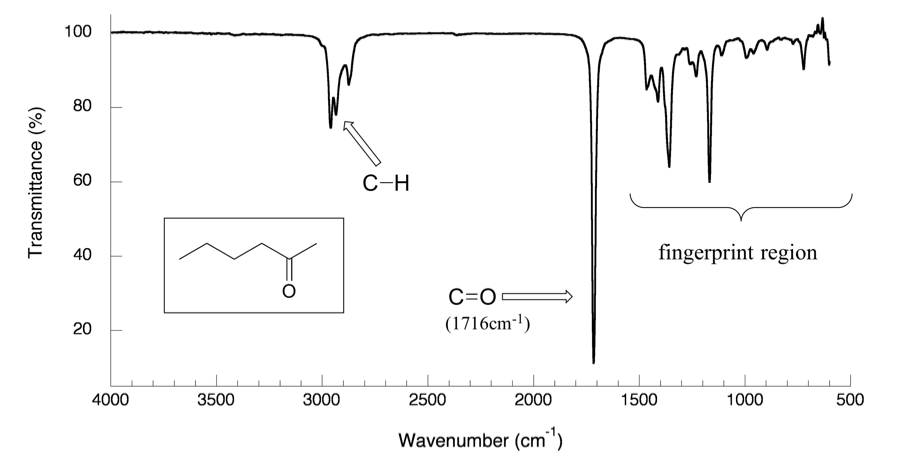
Notes for interpreting IR spectra:
- The vertical axis is ‘% transmittance’, which indicates how strongly light was absorbed at each frequency. The solid line traces the values of % transmittance for every wavelength passed through the sample. At the high end of the axis, 100% transmittance means no absorption occurred at that frequency. Lower values of % transmittance mean that some of the energy is absorbed by the compound and gives downward spikes. The spikes are called absorption bands in the IR spectrum. A molecule has a variety of covalent bonds, and each bond has different vibration modes, so the IR spectrum of a compound usually shows multiple absorption bands.

Please note that the direction of the horizontal axis (wavenumber) in IR spectra decreases from left to right. The larger wavenumbers (shorter wavelengths) are associated with higher frequencies and higher energy.
Stretching Vibrations
Generally, stretching vibrations require more energy and show absorption bands in the higher wavenumber/frequency region. The characteristics of stretching vibration bands associated with the bonds in some common functional groups are summarized in Table 6.1 .
Table 6.1 Characteristic IR Frequencies of Stretching Vibrations
The information in Table 6.1 can be summarized in the diagram for easier identification (Figure 6.3b) , in which the IR spectrum is divided into several regions, with the characteristic band of certain groups labelled.
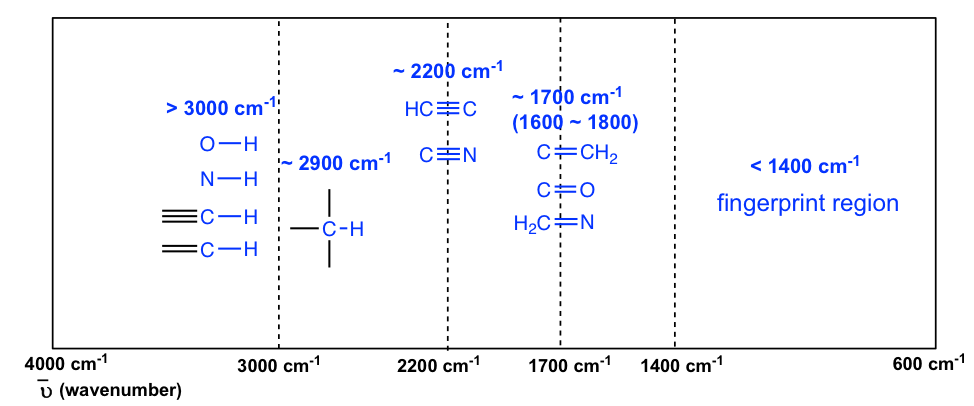
The absorption bands in IR spectra have different intensities that can usually be referred to as strong (s), medium (m), weak (w), broad and sharp. The intensity of an absorption band depends on the polarity of the bond, and a bond with higher polarity will show a more intense absorption band. The intensity also depends on the number of bonds responsible for the absorption, and an absorption band with more bonds involved has a higher intensity.
The polar O-H bond (in alcohol and carboxylic acid) usually shows strong and broad absorption bands that are easy to identify. The broad shape of the absorption band results from the hydrogen bonding of the OH groups between molecules. The OH bond of an alcohol group usually has absorption in the range of 3200–3600 cm -1 , while the OH bond of the carboxylic acid group occurs at about 2500–3300 cm -1 (Figure 6.4a and Figure 6.4c).
The polarity of the N-H bond (in amine and amide) is weaker than the OH bond, so the absorption band of N-H is not as intense or as broad as O-H, and the position is in the 3300–3500 cm -1 region.
The C-H bond stretching of all hydrocarbons occurs in the range of 2800–3300 cm -1 , and the exact location can be used to distinguish between alkane, alkene and alkyne. Specifically:
- ≡C-H (sp C-H) bond of terminal alkyne gives absorption at about 3300 cm -1
- =C-H (sp 2 C-H) bond of alkene gives absorption at about 3000-3100 cm -1
- -C-H (sp 3 C-H) bond of alkane gives absorption at about ~2900 cm -1 (see the example of the IR spectrum of 2-hexanone in Figure 6.3a; the C-H absorption band at about 2900 cm -1 )
A special note should be made for the C-H bond stretching of an aldehyde group that shows two absorption bands: one at ~2800 cm -1 and the other at ~ 2700 cm -1 . It is therefore relatively easy to identify the aldehyde group (together with the C=O stretching at about 1700 cm -1 ) since essentially no other absorptions occur at these wavenumbers (see the example of the IR spectrum of butanal in Figure 6.4d ).
The stretching vibration of triple bonds C≡C and C≡N have absorption bands of about 2100–2200 cm -1 . The band intensity is in a medium to weak level. The alkynes can generally be identified with the characteristic weak but sharp IR absorbance bands in the range of 2100–2250 cm -1 due to stretching of the C≡C triple bond, and terminal alkynes can be identified by their absorbance at about 3300 cm -1 due to stretching of sp C-H.
As mentioned earlier, the C=O stretching has a strong absorption band in the 1650–1750 cm -1 region. Other double bonds like C=C and C=N have absorptions in lower frequency regions of about 1550–1650 cm -1 . The C=C stretching of an alkene only shows one band at ~1600 cm -1 (Figure 6.4b) , while a benzene ring is indicated by two sharp absorption bands: one at ~1600 cm -1 and one at 1500–1430 cm -1 (see the example of the IR spectrum of ethyl benzene in Figure 6.4e ) .
You will notice in Figure 6.3a and 6.3b that a region with the lower frequency 400–1400 cm -1 in the IR spectrum is called the fi ngerprint region . Similar to a human fingerprint, the pattern of absorbance bands in the fingerprint region is characteristic of the compound as a whole. Even if two different molecules have the same functional groups, their IR spectra will not be identical, and such a difference will be reflected in the bands in the fingerprint region. Therefore, the IR from an unknown sample can be compared to a database of IR spectra of known standards in order to confirm the identification of the unknown sample.
Organic Chemistry I Copyright © 2021 by Xin Liu is licensed under a Creative Commons Attribution-NonCommercial-ShareAlike 4.0 International License , except where otherwise noted.
Share This Book

- Keep it simple - don't use too many different parameters.
- Example: (diode OR solid-state) AND laser [search contains "diode" or "solid-state" and laser]
- Example: (photons AND downconversion) - pump [search contains both "photons" and "downconversion" but not "pump"]
- Improve efficiency in your search by using wildcards.
- Asterisk ( * ) -- Example: "elect*" retrieves documents containing "electron," "electronic," and "electricity"
- Question mark (?) -- Example: "gr?y" retrieves documents containing "grey" or "gray"
- Use quotation marks " " around specific phrases where you want the entire phrase only.
- For best results, use the separate Authors field to search for author names.
- Use these formats for best results: Smith or J Smith
- Use a comma to separate multiple people: J Smith, RL Jones, Macarthur
- Note: Author names will be searched in the keywords field, also, but that may find papers where the person is mentioned, rather than papers they authored.
Journal of Near Infrared Spectroscopy
- pp. 287-308

A Review of Band Assignments in near Infrared Spectra of Wood and Wood Components
Manfred Schwanninger, José Carlos Rodrigues, and Karin Fackler
Author Affiliations
Manfred Schwanninger Department of Chemistry, BOKU-University of Natural Resources and Life Sciences, Vienna, Muthgasse 18, A-1190 Vienna, Austria
José Carlos Rodrigues Tropical Research Institute of Portugal, Forest and Forest Products Centre, Tapada da Ajuda, 1349-017 Lisboa, Portugal
Karin Fackler Faculty of Technical Chemistry, Institute of Chemical Engineering, Vienna University of Technology, Getreidemarkt 9/166-4, A-1060 Vienna, Austria
- M Schwanninger
- J Rodrigues
- Share with Facebook
- Post on reddit
- Share with LinkedIn
- Add to Mendeley

- Share with WeChat
- Endnote (RIS)
- Save article
- Absorption coefficient
- Absorption spectroscopy
- Diffuse optical spectroscopy
- Diffuse reflectance
- Near infrared
- Near infrared spectroscopy
- Original Manuscript: August 22, 2011
- Revised Manuscript: November 1, 2011
- Manuscript Accepted: November 5, 2011
- Cited By ( 32 )
- Back to Top
Near infrared (NIR) spectra of wood and wood products contain information regarding their chemical composition and molecular structure. Both influence physical properties and performance, however, at present, this information is under-utilised in research and industry. Presently NIR spectroscopy is mainly used following the explorative approach, by which the contents of chemical components and physico–chemical as well as mechanical properties of the samples of interest are determined by applying multivariate statistical methods on the spectral data. Concrete hypotheses or prior knowledge on the chemistry and structure of the sample—exceeding that of reference data—are not necessary to build such multivariate models. However, to understand the underlying chemistry, knowledge on the chemical/functional groups that absorb at distinct wavelengths is indispensable and the assignment of NIR bands is necessary. Band assignment is an interesting and important part of spectroscopy that allows conclusions to be drawn on the chemistry and physico–chemical properties of samples. To summarise current knowledge on this topic, 70 years of NIR band assignment literature for wood and wood components were reviewed. In addition, preliminary results of ongoing investigations that also led to new assignments were included for discussion. Furthermore, some basic considerations on the interactions of NIR radiation with the inhomogeneous, anisotropic and porous structure of wood, and what impact this structure has on information contained in the spectra, are presented. In addition, the influence of common data (pre)-processing methods on the position of NIR bands is discussed. For more conclusive band assignments, it is recommended that wood is separated into its components. However, this approach may lead to misinterpretations when evaluation methods other than direct comparison of spectra are used, because isolation and purification of wood components is difficult and may lead to chemical and structural alterations when compared to the native state. Furthermore, “pure” components have more distinct and symmetric bands that influence the shape of the spectra. This extended review provides the reader with a comprehensive summary of NIR bands, as well as some practical considerations important for the application of NIR to wood.
© 2011 IM Publications LLP
Optica participates in Crossref's Cited-By Linking service . Citing articles from Optica Publishing Group journals and other participating publishers are listed here.
Roger Meder, Editor-in-Chief
Confirm Citation Alert
Field error.
- Publishing Home
- Conferences
- Preprints (Optica Open)
- Information for
- Open Access Information
- Open Access Statement and Policy
- Terms for Journal Article Reuse
- About Optica Publishing Group
- About My Account
- Send Us Feedback
- Optica Home
- Other Resources
- Optica Open
- Optica Publishing Group Bookshelf
- Optics ImageBank
- Optics & Photonics News
- Spotlight on Optics
- Go to My Account
- Login to access favorites
- Recent Pages
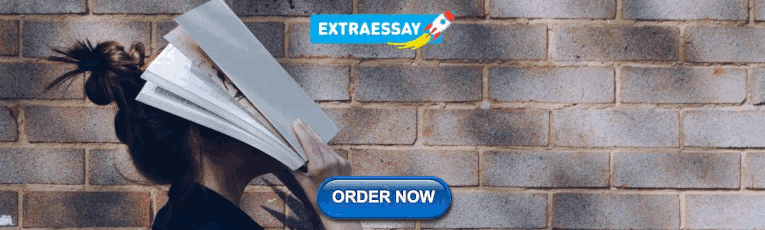
Login or Create Account
Thank you for visiting nature.com. You are using a browser version with limited support for CSS. To obtain the best experience, we recommend you use a more up to date browser (or turn off compatibility mode in Internet Explorer). In the meantime, to ensure continued support, we are displaying the site without styles and JavaScript.
- View all journals
- Explore content
- About the journal
- Publish with us
- Sign up for alerts
- Original Article
- Published: 09 November 2011
Physical Properties of Polymers
In situ near-infrared spectroscopic studies of the structural changes of polyethylene during melting
- Masami Mizushima 1 ,
- Takanobu Kawamura 1 ,
- Kenji Takahashi 1 &
- Koh-hei Nitta 1
Polymer Journal volume 44 , pages 162–166 ( 2012 ) Cite this article
2846 Accesses
22 Citations
Metrics details
Conformational changes occurring during the melting of various polyethylene (PE) materials, including high-density PE (HDPE), linear low-density PE (LLDPE) and low-density polyethylene (LDPE), were investigated using near-infrared spectroscopy. The assignment of PE to the 1650–1900 nm spectral region was suggested, on the basis of computational and experimental data for normal alkanes. The present results suggested that the 1690 and 1710 nm bands should be assigned to the CH 3 groups in branched chains and chain ends, respectively. The HDPE and LDPE crystal lattices have very few defects, in contrast to LLDPE, which has more crystal lattice defects. The chain ends and junctions of LDPE are excluded from the crystal lattice and exist in a thick amorphous layer, whereas LLDPE includes comonomers in its crystalline lattice.
Similar content being viewed by others
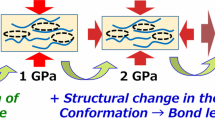
Characteristic changes in the structures and properties of polyimides induced by very high pressures up to 8 GPa
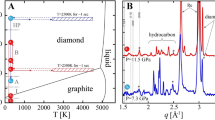
Diamond and methane formation from the chemical decomposition of polyethylene at high pressures and temperatures
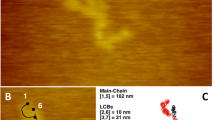
Direct Observation of Long-Chain Branches in a Low-Density Polyethylene
Introduction.
The structural rearrangement and phase transformation of semicrystalline polymers, such as polyethylene (PE) and polypropylene, during annealing and heating have received significant academic and industrial interest. The thermal and melting properties of these semicrystalline polymers have been extensively investigated; however, the molecular mechanisms of melting and reorganization are still not well understood. The main reason for this is the paucity of reported in situ measurements of structural morphology and chain conformation during melting and heating processes.
Fourier transform infrared spectroscopy is a powerful tool for detecting chain conformation and structural change or phase transition during heating and cooling. In situ Fourier transform infrared spectroscopic studies of the conformational changes of semicrystalline polymers such as PE and polypropylene have been conducted. 1 , 2 , 3 The mid-infrared region from 2500–25 000 nm is diagnostic for these polyolefins. However, the very high absorption in the mid-infrared region makes it difficult to assign spectra for industrial polyolefin bulk specimens; very thin films are usually required.
In the near-infrared (NIR) region, we consider absorption and luminescence spectra at wavelengths from 800–2500 nm, in which combination tones and overtones generated by anharmonic molecular vibrations are observed. Their absorption peaks are relatively weak compared with those of the basic tones in the mid-infrared region. These low absorption coefficients enable the nondestructive and direct measurement of vibrational spectra of bulk materials and industrial products. One disadvantage of NIR spectra is the absorption overlap of overtones and combination tones, which complicates the assignment of bands for more complex organic materials.
In such circumstances, Norris et al. 4 proposed a band assignment method for NIR spectra using statistical techniques. Barton and Noda et al. 5 , 6 , 7 , 8 also assigned NIR bands by correlation with IR bands using two-dimensional correlation spectroscopy. Ozaki et al. and Siesler et al. 9 , 10 , 11 , 12 , 13 , 14 , 15 , 16 recently demonstrated that NIR spectroscopy can be useful for the structural characterization of polymeric materials, foods and bio-based materials.
Watanabe et al. 17 , 18 , 19 demonstrated the applicability of NIR spectroscopy for characterizing thermally induced structural changes in PE. They monitored the temperature-induced phase transition of crystalline PE materials using NIR spectroscopy. They demonstrated how NIR spectroscopy could detect the melting behavior of crystalline phases in various PE materials but did not characterize the conformational changes during heating. Few studies have focused on the conformational changes of crystallizable polymers during the melting process. The aim of this study is to explore the conformational change of typical linear and branched PE materials during the melting process using in situ NIR spectroscopy. NIR spectroscopy makes it possible to analyze the molecular or structural state in actual materials and industrial products.
In this study, we assigned NIR bands to PE chains based on computational and experimental spectra of normal alkanes. The conformational changes of PE chains were investigated under a constant heating rate up to the melting point.
Experimental procedure
Commercial-grade samples of high-density polyethylene (HDPE), linear low-density polyethylene (LLDPE) with ethyl branches and low-density polyethylene (LDPE) produced by an autoclave reactor were used. The molecular characteristics of these samples are given in Table 1 .
PE pellets were melted in a hot press at 483 K under 10 MPa for 10 min, with subsequent quenching from the melt to 373 K. The thickness of the compressed molded sheets was ∼ 500 μm.
Characterization
Differential scanning calorimetry (DSC) measurements were carried out using a Diamond DSC (PerkinElmer Inc., Waltham, MA, USA). Samples were heated from 313–473 K at a scanning rate of 20 K min −1 under a N 2 atmosphere. The crystallinity in the weight fraction was determined from fusion enthalpy data, where the equilibrium fusion enthalpy of PE was taken to be 277.1 kJ kg −1 . 20 The density of sheet samples was obtained with the Archimedes method using a METTLER electrobalance XS205 (Mettler Toredo International Inc., Greifensee, Switzerland), with ethanol as the solvent. The melting temperatures, crystallinity and density of the polymer samples are given in Table 2 .
NIR spectral measurements during melting
The experimental setup is illustrated in Figure 1 . A Xe short-arc lamp (USHIO Inc., Tokyo Japan, UXL-300D-O), InGaAs PIN photodiode (Hamamatsu photonics Inc., Shizuoka, Japan, G8371-03) and monochromator (Hamamatsu photonics Inc. CT-10) were used as the light source, detector and monochromator, respectively. A digital oscilloscope (Lecroy Co., Chestnut Ridge, NY, USA, Model 9310 L) was used to capture spectral data. Spectral data from the oscilloscope and monochromator were analyzed with a program written using the Igor 4.0 software package (Wavematrix Inc., Portland, OR, USA). A GP-IB board (CONTEC Co. Ltd., Osaka, Japan, GP-IB(PCI)F) was used for communication between the oscilloscope, monochromator and computer.
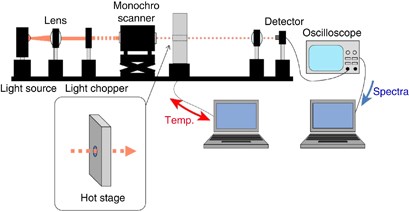
NIR experimental setup. A full color version of this figure is available at Polymer Journal online.
A hot stage (Mettler Toredo International Inc., Greifensee, Switzerland) was placed in the optical pathway. NIR spectra were monitored during heating from 313–473 K at a rate of 2 K min −1 . Spectra were measured at a sweep rate of 600 nm min −1 over the range 1650–1900 nm, where the absorption of the first CH stretching overtone vibration can be detected. 16 Many overlapped absorption bands make it difficult to assign the absorption bands of NIR spectra. For the assignment of NIR bands of PE, we compared NIR spectra of various linear alkane solvents from hexane to tetradecane to that of molten linear HDPE. In addition, we computed the spectra of the fundamental molecular vibration of a linear C14 hydrocarbon and a C14 hydrocarbon with an ethyl group branch at the center of the main chain by using the Gaussian 03W Version 6.0 (GAUSSIAN Inc., Wallingford, CT, USA) software package. On the basis of the above measurements and computation in addition to published data, 16 , 17 , 19 we determined the peak assignments of the major NIR bands for PE.
Results and discussion
NIR spectra for PE samples in the 1650–1900 nm range are shown in Figure 2 ; there are two predominant peaks at 1728 and 1764 nm and various shoulders around these peaks. The intensity of the 1728 nm peak for HDPE with higher crystallinity is larger than the intensity of this peak for LLDPE and LDPE, whereas the 1764 nm peak intensity is comparable among the three PE samples. The intensity of the 1750 nm peak of LDPE and LLDPE is larger than that of HDPE. Peaks at 1728 and 1764 nm for LDPE and LLDPE are broadened in comparison to those for HDPE, which led us to conclude that the two predominant peaks include shoulder peaks associated with chain branching. According to our previous estimation of CH 3 -stretching IR absorptions, the first CH 3 stretching overtone vibration occurs in this region. 21
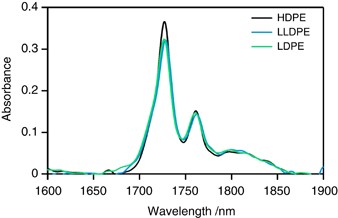
NIR spectra of PE samples. A full color version of this figure is available at Polymer Journal online.
To assign NIR bands to PE, we measured NIR spectra of various linear alkane solvents from hexane to tetradecane, along with molten HDPE. These spectra were measured using optical path lengths of 2 and 0.65 mm, respectively. The intensity was divided by the thickness to yield thickness-independent results. The spectra of various alkanes and molten HDPE are shown in Figure 3 .
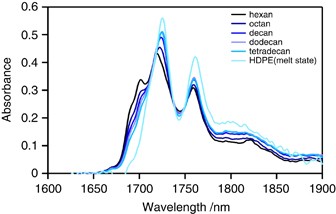
NIR spectra of alkane solvents and molten HDPE of varying lengths. A full color version of this figure is available at Polymer Journal online.
The absorbance of the 1728 and 1764 nm peaks increases with increasing carbon atoms in the main chain, which suggests these peaks arise from the first CH 2 stretching overtone vibration of the main chain. Figure 3 shows that the predominant peaks at 1728 and 1764 nm broaden with decreasing carbon atoms in the main chain. The 1750 nm absorption peak of the solvents is more intense than that for molten HDPE, and the valley between the 1728 and 1764 nm peaks deepens as the number of carbon atoms increases. This finding suggests that some absorptions around the 1740–1750 nm valley result from CH 3 vibration. Shoulder peaks at 1690 and 1710 nm are apparent for linear alkanes but are largely absent in the spectrum of HDPE. Ozaki et al. reported that the 1710 nm absorption is associated with CH 2 units in the amorphous phase. 17 , 19 It is likely that the 1690 and 1710 nm absorptions are associated with the CH 3 groups of chain ends because the intensity of these absorptions decreases with increasing carbon atoms.
The fundamental molecular vibrations of methylene chains corresponding to each wavelength peak were calculated using the Gaussian 03W Version 6.0 (GAUSSIAN) software package, to confirm the assignment of these NIR bands. IR spectra calculated by the Gaussian software were converted to the vibrational overtone spectra, by adjusting them to the experimental NIR spectrum of the HDPE melt. Calculated spectra for a linear C14 hydrocarbon and C14 hydrocarbon with a central ethyl group branch were obtained. These spectra showed that the 1728 and 1764 nm peaks correspond to the first overtone of the CH 2 asymmetric and symmetric stretching vibrations of the main chain, respectively. This result is consistent with assignments in previous reports. 16 The calculated spectra indicate that the 1764 nm peak becomes more intense in the trans state and that absorption at 1750 nm arises from the symmetric vibration of CH 3 and gauche conformation CH 2 units of the main chain. The simulation results also indicate that the CH 3 vibration occurs in the 1690–1710 nm region. The 1690 nm peak was assigned to the CH 3 asymmetric vibration of the short-chain branches, and the peak at 1710 nm was assigned to the symmetric vibration of terminal CH 3 groups. Computational assignments of NIR peaks for linear C14 and ethyl-branched C14 molecules are summarized in Table 3 . These assignments support our earlier conclusions from experimental results that shoulder peaks at 1690, 1710 and 1750 nm are largely absent in molten HDPE, which has few terminal CH 3 groups. Figure 3 shows that the NIR bands at 1710 and 1690 nm associated with the CH 3 groups of LLDPE and LDPE shift to 1715 and 1705 nm, respectively.
Figure 4 shows NIR spectra under a constant heating rate. The intensity of the 1728 nm peak decreases, and that of the 1764 nm peak increases with increasing temperature. The magnitude of the intensity change depends on the weight fraction of the crystalline phase before melting. The temperature sensitivity of the 1728 and 1764 nm peaks of HDPE is considerably higher than those of LDPE and LLDPE.
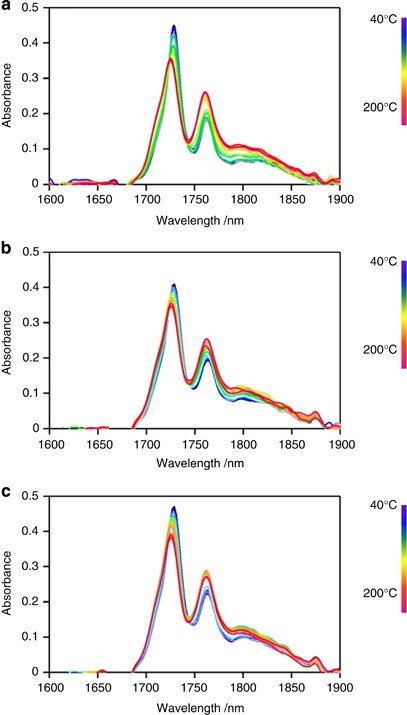
The temperature dependence of the NIR spectra of ( a ) HDPE, ( b ) LLDPE and ( c ) LDPE at a 2 K min −1 constant heating rate. A full color version of this figure is available at Polymer Journal online.
The 1650–1900 nm NIR region is thought to be composed of 5 peaks at 1705, 1715, 1728, 1750 and 1764 nm. Spectral decomposition was performed using the Voigt function implemented in the Igor 4.0 software package (Wavematrix). Figure 5 shows the component peaks obtained from curve fitting. The intensities of the 1705, 1715 and 1750 nm peaks of LDPE and LLDPE are greater than those of HDPE. This is due to the high terminal CH 3 group content of LDPE and LLDPE because chain branching in this sample is much larger than that in HDPE. Figure 6 shows integrated intensities of the 1705, 1715, 1728, 1750 and 1764 nm peaks plotted against temperature. Each peak intensity was normalized by the total intensity of the five peaks. The DSC profile is also shown.
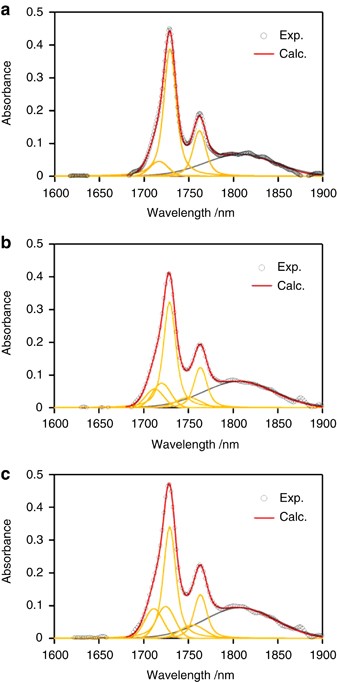
Typical curve-fitting results for the NIR spectra of ( a ) HDPE, ( b ) LLDPE and ( c ) LDPE: ○ denote experimental values; red lines denote the calculated spectra; orange and gray lines denote the six component peaks. A full color version of this figure is available at Polymer Journal online.
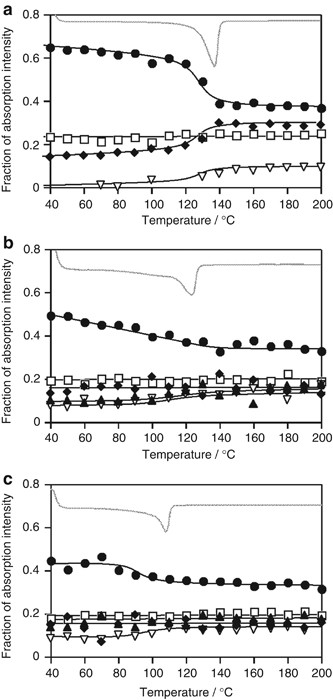
Variation of the fraction of NIR peak intensity for ( a ) HDPE, ( b ) LLDPE and ( c ) LDPE under a 2 K min −1 constant heating rate and DSC curves: the symbols denote the fraction of NIR intensity ( ▴ : 1690 nm, ♦: 1710 nm, •: 1728 nm, ▿ : 1750 nm, □: 1764 nm); the gray line denotes the DSC curve.
Figure 6a shows that as the temperature increases, the intensity of the HDPE CH stretching peak at 1728 nm decreases near the melting point of the DSC curve. This suggests that the asymmetric CH 2 stretching vibration in the crystal lattice becomes active upon melting. In contrast, the intensity of the 1715 and 1750 nm peaks ascribed to terminal CH 3 and gauche conformation CH 2 units in the main chain, respectively, exhibits a stepwise increase near the DSC melting peak. This result suggests that melting releases the chain ends within crystalline lamellae and cilia chains embedded into crystalline lamellae, thus making it possible to change from the trans to gauche conformations. The 1705 nm peak ascribed to the CH 3 group of short branches is too weak to detect for HDPE.
The intensity of the LLDPE peak at 1728 nm continuously decreases, reflecting the broad melting behavior seen in the DSC profiles in Figure 6b . In contrast to HDPE, the intensity of the LLDPE terminal CH 3 peak at the 1715 nm peak is largely unchanged near the melting point, indicating that the molecular mobility of chain ends in the solid state is similar to that in the melted state. It suggests that there are many dangling chains or long cilia chains within the amorphous layer, which is plausible because the crystallinity of LLDPE is much lower than that of HDPE. The 1705 nm peak intensity (short branches) slightly increases near the DSC melting peak, indicating the inclusion of short-chain branches in the crystal lattice before melting. 22
Figure 6c shows that the 1705 nm peak intensity is almost independent of melting, and that the 1728 nm peak intensity decreases near the DSC melting peak for LDPE. This behavior is similar to that for HDPE but different from that of LLDPE, indicating that most branches of LDPE are sufficiently long that chain ends and junctions are completely excluded from the crystal lattice and exist in the thick amorphous layers. The monotonous decrease of the 1728 nm peak for LLDPE suggests the inclusion of defects or comonomers in the crystalline lattice.
The 1764 nm peak intensity (CH 2 trans) in HDPE, LLDPE and LDPE is independent of temperature, suggesting that it is not sensitive to the melting of the crystal lattice. It appears that a certain concentration of trans conformation sequences exists that are independent of heating, up to the melting point.
Conclusions
The NIR spectra of PE materials during heating were acquired to directly examine the molecular behavior during melting. The utility of NIR spectroscopy in investigating conformational changes of different crystallizable PE samples has been demonstrated. NIR bands of PE in the 1650–1900 nm region were assigned with the aid of computer-simulated molecular vibrations and the experimentally measured NIR spectra of alkane solvents. The following conclusions were reached:
Peaks at 1728 and 1764 nm result from the first overtone of the CH 2 asymmetric and symmetric stretching vibrations of the main chain, respectively. The 1764 nm peak has a high spectral intensity in the trans state, and absorption at 1750 nm is associated with the symmetric vibration of CH 3 and gauche conformation of CH 2 groups of the main chain. The 1705 nm peak was assigned to the CH 3 asymmetric vibration of short branches, and that at 1715 nm was assigned to the terminal CH 3 symmetric vibration.
The 1728 nm peak is associated with CH 2 units within the crystal lattice. The molecular motion of HDPE chain ends becomes active near the melting point. The chain ends and junctions of LDPE are completely excluded from the crystal lattice and exist in the thick amorphous layer. In contrast, the crystal lattice of LLDPE includes defects or comonomers. The 1764 nm peak intensity is resistant to heating and melting, suggesting that a constant amount of trans sequence CH 2 units always exists.
Zhu, X., Yan, D. & Fang, Y. In Situ FTIR spectroscopic study of the conformational change of isotactic polypropylene during the crystallization process. J. Phys. Chem. B 105 , 12461–12463 (2001).
Article CAS Google Scholar
Plass, M., Streck, R., Nieto, J. & Siesler, H. W. Rheo-optical FT-IR spectroscopy of LLDPE: effect of comonomer and composite materials. Macromol. Symp. 265 , 166–177 (2008).
Parthasarthy, G., Sevegney, M. & Kannan, R. M. Rheooptical Fourier transform infrared spectroscopy of the deformation behavior in quenched and slow-cooled isotactic polypropylene films. J. Polym. Sci. B 40 , 2539–2551 (2002).
Ben-Gera, I. & Norris, K. H. Direct spectrophotometric determination of fat and moisture in meat products. J. Food Sci. 33 , 64–67 (1968).
Barton II, F. E., Himmelsbach, D. S., Duckwoeth, J. H. & Smith, M. J. Two-dimensional vibration spectroscopy: correlation of mid- and near-infrared regions. Appl. Spectrosc. 46 , 420–429 (1992).
Noda, I., Dowrey, A. E. & Marcott, C. Recent developments in two-dimensional infrared (2D IR) correlation spectroscopy. Appl. Spectrosc. 47 , 1317–1323 (1993).
Noda, I., Liu, Y., Ozaki, Y. & Czarnecki, M. A. Two-dimensional Fourier transform near-infrared correlation spectroscopy studies of temperature-dependent spectral variations of Oleyl alcohol. J. Phys. Chem. 99 , 3068–3073 (1995).
Noda, I. Determination of two-dimensional correlation spectra using the Hilbert transform. Appl. Spectrosc. 54 , 994–999 (2000).
Hoffmannn, U., Pfeifer, F., Okretic, S., Völky, N., Zahedi, M. & Siesler, H. W. Rheo-optical Fourier transform infrared and Raman spectroscopy of polymers. Appl. Spectrosc. 47 , 1531–1539 (1993).
Article Google Scholar
Schade, C., Heckmann, W., Borchert, S. & Siesler, H. W. Determination of orientational states in impact-polystyrene specimens by near-infrared polarization spectroscopy. Polym. Eng. Sci. 46 , 381–383 (2006).
Watari, M. & Ozaki, Y. Prediction of ethylene content in melt-state random and block polypropylene by near-infrared spectroscopy and chemometrics: comparison of a new calibration transfer method with a slope/bias correction method. Appl. Spectrosc. 58 , 1210–1218 (2004).
Watari, M. & Ozaki, Y. Calibration models for the vinyl acetate concentration in ethylene-vinyl acetate copolymers and its on-line monitoring by near-infrared spectroscopy and chemometrics: use of band shifts associated with variations in the vinyl acetate concentration to improve the models. Appl. Spectrosc. 59 , 912–919 (2005).
Watari, M. & Ozaki, Y. Practical calibration correction method for the maintenance of an on-line near-infrared monitoring system for molten polymers. Appl. Spectrosc. 60 , 529–538 (2006).
Miller, C. E. Near-infrared spectroscopy of synthetic polymers. Appl. Spectrosc. Rev. 26 , 277–339 (1991).
Furukawa, T., Watari, M., Siesler, H. W. & Ozaki, Y. Discrimination of various poly(propylene) copolymers and prediction of their ethylene content by near-infrared and Raman spectroscopy in combination with chemometric methods. J. Appl. Polym. Sci. 87 , 616–625 (2003).
Sato, H., Shinoyama, M., Kamiya, T., Amari, T., Šašić, S., Ninomiya, T., Siesler, H. W. & Ozaki, Y. Near infrared spectra of pellets and thin films of high-density, low-density and linear low-density polyethylenes and prediction of their physical properties by multivariate data analysis. J. Near Infrared Spectrosc. 11 , 309–321 (2003).
Watanabe, S., Dybal, J., Tashiro, K. & Ozaki, Y. A near-infrared study of thermally induced structural changes in polyethylene crystal. Polymer 47 , 2010–2017 (2006).
Watanabe, S., Noda, I. & Ozaki, Y. Thermally induced conformational changes in polyethylene studied by two-dimensional near-infrared-infrared hetero-spectral correlation spectroscopy. J. Mol. Struct. 883–884 , 173–180 (2008).
Watanabe, S., Noda, I. & Ozaki, Y. Thermally induced conformational and structural disordering in polyethylene crystal studied by near-infrared spectroscopy. Polymer 49 , 774–784 (2008).
Wunderlich, B. & Dole, M. Specific heat of synthetic high polymers. VIII. Low pressure polyethylene. J. Polym. Sci. 24 , 201–213 (1957).
Krimm, S. Infrared spectra of high polymers. Fortschr. Hochpolym. Forch. 2 , 51 (1960).
Salazer, J. M. & Calleja, F. J. B. On the Inclusion of Chain Defects in the Polyethylene Lattice A statistical Approach. Polym. Bull. 2 , 163–167 (1980).
Google Scholar
Download references
Author information
Authors and affiliations.
Division of Material Sciences, Graduate School of Natural Science and Technology, Kanazawa University, Kanazawa, Japan
Masami Mizushima, Takanobu Kawamura, Kenji Takahashi & Koh-hei Nitta
You can also search for this author in PubMed Google Scholar
Corresponding author
Correspondence to Koh-hei Nitta .
Rights and permissions
Reprints and permissions
About this article
Cite this article.
Mizushima, M., Kawamura, T., Takahashi, K. et al. In situ near-infrared spectroscopic studies of the structural changes of polyethylene during melting. Polym J 44 , 162–166 (2012). https://doi.org/10.1038/pj.2011.100
Download citation
Received : 01 July 2011
Revised : 01 September 2011
Accepted : 02 September 2011
Published : 09 November 2011
Issue Date : February 2012
DOI : https://doi.org/10.1038/pj.2011.100
Share this article
Anyone you share the following link with will be able to read this content:
Sorry, a shareable link is not currently available for this article.
Provided by the Springer Nature SharedIt content-sharing initiative
- melting process
- near-infrared
- polyethylene
This article is cited by
High-throughput nir spectroscopic (nirs) detection of microplastics in soil.
- Andrea Paul
- Lukas Wander
- Ulrike Braun
Environmental Science and Pollution Research (2019)
Quick links
- Explore articles by subject
- Guide to authors
- Editorial policies

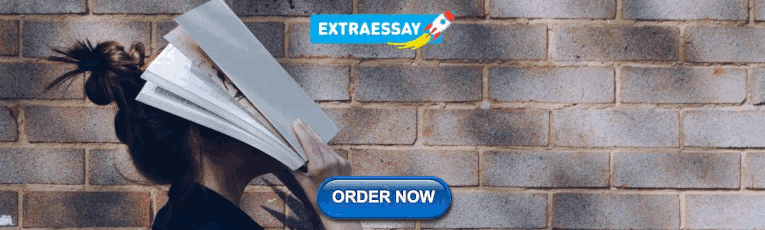
IMAGES
VIDEO
COMMENTS
2. Chart of Near-IR Absorption Bands. THIRD OVERTONE REGION FIRST OVERTONE REGION SECOND OVERTONE REGION COMBINATION BANDS REGION. CH. 4thOvertone. NH. 3rdOvertone. OH. 2ndOvertone.
%PDF-1.6 %âãÏÓ 157 0 obj > endobj 176 0 obj >/Filter/FlateDecode/ID[29E5AF2A7F9D0F4196F4DB70B6DBCA78>89F80795035FDF4A95A9FB23652E2839>]/Index[157 33]/Info 156 0 R ...
Infrared Spectroscopy Absorption Table is shared under a CC BY-NC-SA 4.0 license and was authored, remixed, and/or curated by LibreTexts. Back to top \(^1H\) NNR Solvent Shifts
Download scientific diagram | NIR band assignment table. Source: Metrohm Monograph 8.108.5026EN-A guide to near-infrared spectroscopic analysis of industrial manufacturing processes. Metrohm AG ...
An infrared spectroscopy correlation table (or table of infrared absorption frequencies) is a list of absorption peaks and frequencies, typically reported in wavenumber, for common types of molecular bonds and functional groups. In physical and analytical chemistry, infrared spectroscopy (IR spectroscopy) is a technique used to identify chemical compounds based on the way infrared radiation is ...
Near-Infrared Spectroscopy Table 2.2. Band Assignments of the First Overtone of the NH Stretching Vibration (Klotz and Franzen, 1962; Hanlon et al., 1963; Klotz et al., 1964; Hanlon and Klotz, 1965) Band assignments Peptide H-bond Protonated peptide Peptide-acid H-bond Chemical state of - NH in polypeptides " C / I N H / " o " C / I N H / "
Table 6.1 Characteristic IR Frequencies of Stretching Vibrations. The information in Table 6.1 can be summarized in the diagram for easier identification (Figure 6.3b), in which the IR spectrum is divided into several regions, with the characteristic band of certain groups labelled.. Figure 6.3b Approximate IR Absorption Range. The absorption bands in IR spectra have different intensities that ...
Table 6 The characteristic absorption band and structure assignment of 9 terpenoid compounds. ... J. C. & Fackler, K. A review of band assignments in near infrared spectra of wood and wood ...
Figures and tables; ... complicated near infrared spectra?—Recent progress in spectral analysis methods for resolution enhancement and band assignments in the near infrared region", J. Near Infrared Spectrosc. 9(2), 63 (2001). 10.1255/jnirs.295. Crossref. ISI. Google Scholar. 79.
Near-IR absorption spectrum of dichloromethane showing complicated overlapping overtones of mid IR absorption features.. Near-infrared spectroscopy (NIRS) is a spectroscopic method that uses the near-infrared region of the electromagnetic spectrum (from 780 nm to 2500 nm). Typical applications include medical and physiological diagnostics and research including blood sugar, pulse oximetry ...
Table of Characteristic IR Absorptions m=medium, w=weak, s=strong, n=narrow, b=broad, sh=sharp frequency, cm -1 bond functional group 3640-3610 (s, sh) O-H stretch, free hydroxyl alcohols, phenols
Infrared Spectroscopy. 1. Introduction. As noted in a previous chapter, the light our eyes see is but a small part of a broad spectrum of electromagnetic radiation. On the immediate high energy side of the visible spectrum lies the ultraviolet, and on the low energy side is the infrared. The portion of the infrared region most useful for ...
The different band assignments are listed in Table 5 [31]. At the beginning, the spectrum of the initial mixture displays an absorption peak of the epoxy group at 4530 cm −1 , an absorption peak ...
Tables. View Options. Get access. Access options. If you have access to journal content via a personal subscription, university, library, employer or society, select from the options below: ... Complicated near Infrared Spectra?—Recent Progress in Spectral Analysis Methods for Resolution Enhancement and Band Assignments in the near Infrared ...
1. Introduction. Near-infrared (NIR; 1000-2500 nm; 10,000-4000 cm −1) spectroscopy might be summarized as a vibrational spectroscopy technique [1,2,3] that occupies a somewhat peculiar spot across the field of bioscience.On the one hand, it has earned the status of tool-of-choice in various applications concerning the qualitative and quantitative assessment of bio-related samples, e.g ...
IR and Raman Spectra-Structure Correlations. Peter J. Larkin, in Infrared and Raman Spectroscopy (Second Edition), 2018 4.1 Review of Selected Carbonyl Species. Carboxylic acid dimers are characterized by an IR band near 1700 cm −1, and a Raman band near 1660 cm −1, which derive from the asymmetric (out-of-phase) and the symmetric (in-phase) C O stretch in the dimer, respectively.
Band assignment is an interesting and important part of spectroscopy that allows conclusions to be drawn on the chemistry and physico-chemical properties of samples. To summarise current knowledge on this topic, 70 years of NIR band assignment literature for wood and wood components were reviewed. In addition, preliminary results of ongoing ...
Near infrared (NIR) spectra of wood and wood products contain information regarding their chemical composition and molecular structure. Both influence physical properties and performance, however, at present, this information is under-utilised in research and industry. Presently NIR spectroscopy is mainly used following the explorative approach, by which the contents of chemical components and ...
represented the absorption band from the NIR spectroscopy and the wavelength range is 680 nm until 1280 nm. Let's evaluate on wavy point of the spectrum by referring to Table 1, the near infrared band assignment table and others research references for more understanding about the overtone of the stretching vibration for rice spectra.
The band near 5600 cm -1 could refer to a higher hydrophilicity of the positively scoring samples (Table 3). Acid and Acid/H 2 O 2 pre- treatment, with samples scoring similarly on PC2 are ...
Table 3 Assignments of near-infrared absorbance peaks of C 14 H 30 and 7-ethyl-C 14 H 29 Full size table Figure 4 shows NIR spectra under a constant heating rate.
Download Table | List of the most significant infrared (FTIR) bands and related functional groups assigned for from publication: Computer Assisted Examination of Infrared and Near Infrared Spectra ...
The intrinsic complexity of NIR spectra, resulting from a large number of overlapping bands that create a strongly convoluted lineshape [], makes them difficult for detailed interpretation, which remains a hindrance in a number of applications [].Extensive studies established characteristic vibrational frequency ranges and comprehensive band assignment tables for MIR or Raman spectra [21,22].