Nanosuspension: Principles, Perspectives and Practices
Affiliation.
- 1 School of Pharmaceutical Sciences, Lovely Professional University, Phagwara-144411, Punjab, India. [email protected].
- PMID: 26721266
- DOI: 10.2174/1567201813666160101120452
In the last three decades, nano-sizing of hydrophobic drugs has emerged as one of the most commonly used strategies to overcome their solubility and bioavailability related issues. Nanosuspensions offer versatile features and unique advantages over other approaches that have been utilized for this purpose. The unique inherent properties of nanosuspensions have been explored for a wide variety of applications. Commercial production of stable nanosuspensions has been made possible by the use of techniques such as media milling and high pressure homogenization. This article reviews various techniques being employed for production, characterization, merits and limitations of nanosuspensions and mechanisms that play a role in the physicochemical stability of nanosuspensions. The common strategies applied so far to overcome their stability and commercialization related aspects are also highlighted.
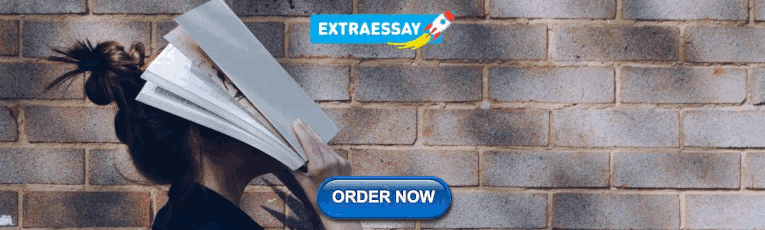
Publication types
- Drug Stability
- Nanoparticles* / administration & dosage
- Nanoparticles* / chemistry
- Nanoparticles* / therapeutic use
- Suspensions / administration & dosage
- Suspensions / chemistry
- Suspensions / therapeutic use
- Suspensions
Advertisement
A comprehensive review of nanosuspension loaded microneedles: fabrication methods, applications, and recent developments
- Published: 06 June 2023
- Volume 53 , pages 475–504, ( 2023 )
Cite this article
- Jyotsna G. Vitore 1 ,
- Sakshi Pagar 2 ,
- Nidhi Singh 3 ,
- Bharathi Karunakaran 1 ,
- Siddharth Salve 1 ,
- Navnath Hatvate 4 ,
- Satish Rojekar 5 &
- Derajram Benival ORCID: orcid.org/0000-0002-3527-9158 1
524 Accesses
3 Citations
Explore all metrics
Nanosuspension-loaded microneedles (MNs) are an emerging and advanced strategy used extensively to formulate lipophilic molecules by embedding them into a suitable polymer matrix for improved transdermal delivery.
Area covered
This review focuses on the nanosuspension/nanocrystal-loaded MN approach for improved delivery of hydrophobic molecules. This review discusses the different types of MNs that can be fabricated, such as dissolving, solid, coated, and hollow MNs and the criteria for the choice of the fabrication method.
Expert opinion
The drug loading of hydrophobic drugs can be significantly improved using this strategy. Recently, nanosuspension/nanocrystal-loaded MNs have been considered a well-recognized approach in drug delivery owing to their numerous advantages, including controlled drug release, non-invasiveness, and ability to be self-administered. The preparation of nanosuspension-loaded dissolving MNs has been widely carried out using knife-casting methods. In the present review, the various characterization methods, including the in vitro, ex vivo and in vivo techniques, are briefly discussed. Further, the review also describes the potential applications of nanosuspension-loaded MNs.
This is a preview of subscription content, log in via an institution to check access.
Access this article
Price excludes VAT (USA) Tax calculation will be finalised during checkout.
Instant access to the full article PDF.
Rent this article via DeepDyve
Institutional subscriptions
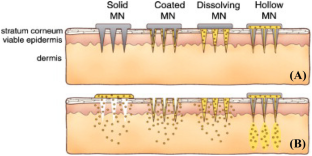
Reprinted from Kim et al. (2012) (Copyright permission obtained)
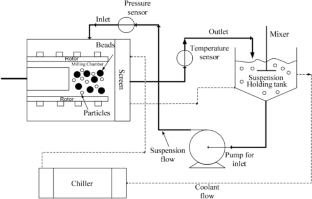
Reprinted from Li et al. ( 2016 ) (Copyright permission obtained)
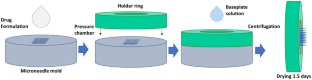
Reprinted from Permana et al. ( 2020 ) (Copyright permission obtained)
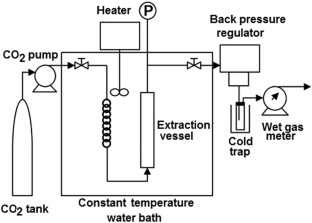
Reprinted from Misra and Pathak ( 2020 ) (Copyright permission obtained)
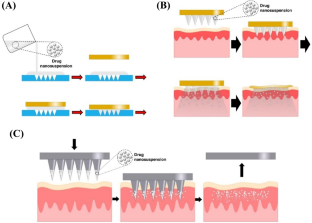
Reprinted from Donnelly and Larrañeta (2018) (Copyright permission obtained)
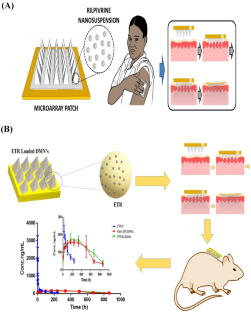
Similar content being viewed by others
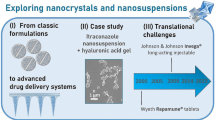
Nanocrystals and nanosuspensions: an exploration from classic formulations to advanced drug delivery systems
Benjamin Rossier, Olivier Jordan, … Carlos Rodríguez-Nogales
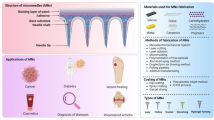
Unravelling the role of microneedles in drug delivery: Principle, perspectives, and practices
Molakpogu Ravindra Babu, Sukriti Vishwas, … Sachin Kumar Singh
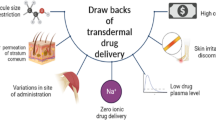
Biomimetic microneedles: exploring the recent advances on a microfabricated system for precision delivery of drugs, peptides, and proteins
Chukwuebuka Emmanuel Umeyor, Varsha Shelke, … Vandana B. Patravale
Data Availability
Not applicable. No research data was used for this publication.
Abdelghany S, Tekko IA, Vora L, Larraneta E, Permana AD et al (2019) Nanosuspension-based dissolving microneedle arrays for intradermal delivery of curcumin. Pharmaceutics 11:308
Article CAS PubMed PubMed Central Google Scholar
Agrawal Y, Patel V (2011) Nanosuspension: an approach to enhance solubility of drugs. J Adv Pharm Technol Res 2:81–87
Article PubMed PubMed Central Google Scholar
Alkilani AZ, McCrudden MTC, Donnelly RF (2015) Transdermal drug delivery: innovative pharmaceutical developments based on disruption of the barrier properties of the stratum corneum. Pharmaceutics 7:438–470
Article CAS PubMed Google Scholar
Altuntaş E, Tekko IA, Vora LK, Kumar N, Brodsky R et al (2021) Nestorone nanosuspension-loaded dissolving microneedles array patch: a promising novel approach for “on-demand” hormonal female-controlled peritcoital contraception. Int J Pharm 614:121422
Article PubMed Google Scholar
Amalraj A, Pius A, Gopi S, Gopi S (2017) Biological activities of curcuminoids, other biomolecules from turmeric and their derivatives—a review. J Tradit Complement Med 7:205–233
Ameri M, Fan SC, Maa Y-F (2010) Parathyroid Hormone PTH (1–34) Formulation that enables uniform coating on a novel transdermal microprojection delivery system. Pharm Res 27:303–313
Anastasiou E, Lorentz KO, Stein GJ, Mitchell PD (2014) Prehistoric schistosomiasis parasite found in the Middle East. Lancet Infect Dis 14:553–554
Andrianov AK, Marin A, DeCollibus DP (2011) Microneedles with intrinsic immunoadjuvant properties: microfabrication, protein stability, and modulated release. Pharm Res 28:58–65
Anjani QK, Sabri AHB, Domínguez-Robles J, Moreno-Castellanos N, Utomo E et al (2022) Metronidazole nanosuspension loaded dissolving microarray patches: an engineered composite pharmaceutical system for the treatment of skin and soft tissue infection. Biomater Adv 140:213073
Aukunuru J, Nanam P, Rambabu B, Sailu C, Aukunuru J (2014) Preparation and characterization of amorphous ezetimibe nanosuspensions intended for enhancement of oral bioavailability. Int J Pharm Investig 4:131
Aust MC, Fernandes D, Kolokythas P, Kaplan HM, Vogt PM (2008) Percutaneous collagen induction therapy: an alternative treatment for scars, wrinkles, and skin laxity. Plast Reconstr Surg 121:1421–1429
Bhakay A, Merwade M, Bilgili E, Dave RN (2011) Novel aspects of wet milling for the production of microsuspensions and nanosuspensions of poorly water-soluble drugs. Drug Dev Ind Pharm 37:963–976
Bystrova S, Luttge R (2011) Micromolding for ceramic microneedle arrays. Microelectron Eng 88:1681–1684
Article CAS Google Scholar
Chen X, Prow TW, Crichton ML, Jenkins DWK, Roberts MS et al (2009) Dry-coated microprojection array patches for targeted delivery of immunotherapeutics to the skin. J Control Release 139:212–220
Chen J, Huang W, Huang Z, Liu S, Ye Y et al (2017) Fabrication of tip-dissolving microneedles for transdermal drug delivery of meloxicam. AAPS PharmSciTech 19:1141–1151
Chingunpitak J, Puttipipatkhachorn S, Chavalitshewinkoon-Petmitr P, Tozuka Y, Moribe K et al (2008) Formation, physical stability and in vitro antimalarial activity of dihydroartemisinin nanosuspensions obtained by co-grinding method. Drug Dev Ind Pharm 34:314–322
Chonkar AD, Rao JV, Managuli RS, Mutalik S, Dengale S et al (2016) Development of fast dissolving oral films containing lercanidipine HCl nanoparticles in semicrystalline polymeric matrix for enhanced dissolution and ex vivo permeation. Eur J Pharm Biopharm 103:179–191
Chu LY, Prausnitz MR (2011) Separable arrowhead microneedles. J Control Release 149:242–249
Costabile G, d’Angelo I, Rampioni G, Bondi R, Pompili B et al (2015) Toward repositioning niclosamide for antivirulence therapy of Pseudomonas aeruginosa lung infections: development of inhalable formulations through nanosuspension technology. Mol Pharm 12:2604–2617
Dabholkar N, Gorantla S, Waghule T, Rapalli VK, Kothuru A et al (2021) Biodegradable microneedles fabricated with carbohydrates and proteins: revolutionary approach for transdermal drug delivery. Int J Biol Macromol 170:602–621
Damiri F, Rahman MH, Zehravi M, Awaji AA, Nasrullah MZ et al (2022) MXene (Ti 3 C 2 T x )-embedded nanocomposite hydrogels for biomedical applications: a review. Materials 15:1666
Dangol M, Yang H, Li CG, Lahiji SF, Kim S et al (2016) Innovative polymeric system (IPS) for solvent-free lipophilic drug transdermal delivery via dissolving microneedles. J Control Release 223:118–125
Davis SP, Martanto W, Allen MG, Prausnitz MR (2005) Hollow metal microneedles for insulin delivery to diabetic rats. IEEE Trans Biomed Eng 52:909–915
DeMuth PC, Su X, Samuel RE, Hammond PT, Irvine DJ (2010) Nano-layered microneedles for transcutaneous delivery of polymer nanoparticles and plasmid DNA. Adv Mater 22:4851–4856
Donnelly RF, Larrañeta E (2018) Microarray patches: potentially useful delivery systems for long-acting nanosuspensions. Drug Discov Today 23:1026–1033
Donnelly RF, Garland MJ, Morrow DI, Migalska K, Singh TR et al (2010a) Optical coherence tomography is a valuable tool in the study of the effects of microneedle geometry on skin penetration characteristics and in-skin dissolution. J Control Release 147:333–341
Donnelly RF, Raj Singh TR, Woolfson AD (2010b) Microneedle-based drug delivery systems: microfabrication, drug delivery, and safety. Drug Deliv 17:187–207
Doraiswamy A, Jin C, Narayan RJ, Mageswaran P, Mente P et al (2006) Two photon induced polymerization of organic–inorganic hybrid biomaterials for microstructured medical devices. Acta Biomater 2:267–275
Du J, Li X, Zhao H, Wang L, Tian S et al (2015) Nanosuspensions of poorly water-soluble drugs prepared by bottom-up technologies. Int J Pharm 495:738–749
Faizi HS, Vora LK, Nasiri MI, Wu Y, Mishra D et al (2022) Deferasirox nanosuspension loaded dissolving microneedles for intradermal delivery. Pharmaceutics 14:2817
Fan Y, Cui Y, Hao W, Chen M, Liu Q et al (2021) Carrier-free highly drug-loaded biomimetic nanosuspensions encapsulated by cancer cell membrane based on homology and active targeting for the treatment of glioma. Bioact Mater 6:4402–4414
FDA (2020) Overview of device regulation, Device Advice Comprehensive Regulatory Assistance. FDA
FDA (2021) Microneedling devices: getting to the point on benefits, risks and safety. FAO
Fomani AA, Mansour RR (2011) Fabrication and characterization of the flexible neural microprobes with improved structural design. Sens Actuators A 168:233–241
Fotooh Abadi L, Damiri F, Zehravi M, Joshi R, Pai R et al (2022) Novel nanotechnology-based approaches for targeting HIV reservoirs. Polymers 14:3090
Gadhiya DT, Patel JK (2021) Bagada AA (2021) An impact of nanocrystals on dissolution rate of lercanidipine: supersaturation and crystallization by addition of solvent to antisolvent. Future J Pharm Sci 7:1–17
Google Scholar
Gao L, Zhang D, Chen M (2008) Drug nanocrystals for the formulation of poorly soluble drugs and its application as a potential drug delivery system. J Nanopart Res 10:845–862
Gill HS, Prausnitz MR (2007a) Coating formulations for microneedles. Pharm Res 24:1369–1380
Gill HS, Prausnitz MR (2007b) Coated microneedles for transdermal delivery. J Control Release 117:227–237
Gill HS, Prausnitz MR (2008) Pocketed microneedles for drug delivery to the skin. J Phys Chem Solids 69:1537–1541
Gittard SD, Narayan RJ, Jin C, Ovsianikov A, Chichkov BN et al (2009) Pulsed laser deposition of antimicrobial silver coating on Ormocer microneedles. Biofabrication 1:041001
Giudice E, Campbell J (2006) Needle-free vaccine delivery. Adv Drug Deliv Rev 58:68–89
Gol D, Thakkar S, Misra M (2018) Nanocrystal-based drug delivery system of risperidone: lyophilization and characterization. Drug Dev Ind Pharm 44:1458–1466
Gora S, Mustafa G, Sahni JK, Ali J, Baboota S (2016) Nanosizing of valsartan by high pressure homogenization to produce dissolution enhanced nanosuspension: pharmacokinetics and pharmacodynamic study. Drug Deliv 23:940–950
Gorantla S, Dabholkar N, Sharma S, Rapalli VK, Alexander A et al (2021) Chitosan-based microneedles as a potential platform for drug delivery through the skin: trends and regulatory aspects. Int J Biol Macromol 184:438–453
Han M, Hyun D-H, Park H-H, Lee SS, Kim C-H et al (2007) A novel fabrication process for out-of-plane microneedle sheets of biocompatible polymer. J Micromech Microeng 17:1184–1191
He J, Zhang Z, Zheng X, Li L, Qi J et al (2021) Design and evaluation of dissolving microneedles for enhanced dermal delivery of propranolol hydrochloride. Pharmaceutics 13:579
Howells O, Blayney GJ, Gualeni B, Birchall JC, Pey FE et al (2022) Design, fabrication, and characterisation of a silicon microneedle array for transdermal therapeutic delivery using a single step wet etch process. Eur J Pharm Biopharm 171:19–28
Huang T, Wang Y, Shen Y, Hui A, Guo Y et al (2020) Preparation of high drug-loading celastrol nanosuspensions and their anti-breast cancer activities in vitro and in vivo. Sci Rep 10:8851
Hwa K-Y, Chang VHS, Cheng Y-Y, Wang Y-D, Jan P-S et al (2017) Analyzing polymeric matrix for fabrication of a biodegradable microneedle array to enhance transdermal delivery. Biomed Microdevices 19:84
Jacob S, Nair AB, Shah J (2020) Emerging role of nanosuspensions in drug delivery systems. Biomater Res 24:3
Jiang J, Gill HS, Ghate D, McCarey BE, Patel SR et al (2007) Coated microneedles for drug delivery to the eye. Investig Ophthalmol vis Sci 48:4038
Article Google Scholar
Kakad SP, Gangurde TD, Kshirsagar SJ, Mundhe VG (2022) Nose to brain delivery of nanosuspensions with first line antiviral agents is alternative treatment option to neuro-AIDS treatment. Heliyon 8:e09925
Karakucuk A, Celebi N, Teksin ZS (2016) Preparation of ritonavir nanosuspensions by microfluidization using polymeric stabilizers: I. A design of experiment approach. Eur J Pharm Sci 95:111–121
Kathuria H, Kochhar JS, Fong MHM, Hashimoto M, Iliescu C et al (2015) Polymeric microneedle array fabrication by photolithography. J vis Exp 105:e52914
Kaushik S, Hord AH, Denson DD, McAllister DV, Smitra S et al (2001) Lack of pain associated with microfabricated microneedles. Anesth Analg 92:502–504
Khare P, Chogale MM, Kakade P, Patravale VB (2022) Gellan gum-based in situ gelling ophthalmic nanosuspension of Posaconazole. Drug Deliv Transl Res 12:2920–2935
Kim YC, Quan FS, Compans RW, Kang SM, Prausnitz MR (2010) Formulation of microneedles coated with influenza virus-like particle vaccine. AAPS PharmSciTech 11:1193–1201
Kim HM, Lim YY, An JH, Kim MN, Kim BJ (2012a) Pharmacology and therapeutics transdermal drug delivery using disk microneedle rollers in a hairless rat model. Int J Dermatol 51:859–863
Kim YC, Park JH, Prausnitz MR (2012b) Microneedles for drug and vaccine delivery. Adv Drug Deliv Rev 64:1547–1568
Kirkby M, Hutton ARJ, Donnelly RF (2020) Microneedle mediated transdermal delivery of protein, peptide and antibody based therapeutics: current status and future considerations. Pharm Res 37:117
Kuk DH, Ha ES, Ha DH, Sim WY, Lee SK et al (2019) Development of a resveratrol nanosuspension using the antisolvent precipitation method without solvent removal, based on a quality by design (QbD) approach. Pharmaceutics 11:688
Kulkarni D, Damiri F, Rojekar S, Zehravi M, Ramproshad S et al (2022) Recent advancements in microneedle technology for multifaceted biomedical applications. Pharmaceutics 14:1097
Kulshreshtha AK, Singh ON, Wall GM (2010) Pharmaceutical suspensions: from formulation development to manufacturing. Springer, New York
Book Google Scholar
Li G, Badkar A, Nema S, Kolli CS, Banga AK (2009) In vitro transdermal delivery of therapeutic antibodies using maltose microneedles. Int J Pharm 368:109–115
Li M, Azad M, Davé R, Bilgili E (2016) Nanomilling of drugs for bioavailability enhancement: a holistic formulation-process perspective. Pharmaceutics 8:17
Li J, Liu B, Zhou Y, Chen Z, Jiang L et al (2017) Fabrication of a Ti porous microneedle array by metal injection molding for transdermal drug delivery. PLoS ONE 12:1–15
Li S, Zhang J, Fang Y, Yi J, Lu Z et al (2020) Enhancing betulinic acid dissolution rate and improving antitumor activity via nanosuspension constructed by anti-solvent technique. Drug Des Dev Ther 14:243–256
Liang L, Chen Y, Zhang BL, Zhang XP, Liu JL et al (2020) Optimization of dip-coating methods for the fabrication of coated microneedles for drug delivery. J Drug Deliv Sci Technol 55:101464
Liu D, Xu H, Tian B, Yuan K, Pan H et al (2012) Fabrication of carvedilol nanosuspensions through the anti-solvent precipitation–ultrasonication method for the improvement of dissolution rate and oral bioavailability. AAPS PharmSciTech 13:295
Liu Q, Guan J, Sun Z, Shen X, Li L et al (2019) Influence of stabilizer type and concentration on the lung deposition and retention of resveratrol nanosuspension-in-microparticles. Int J Pharm 569:118562
Lødeng R, Bergem H (2018) Stabilisation of pyrolysis oils. In: Rossendahl L (ed) Direct thermochemical liquefaction for energy applications. Woodhead Publishing, Cambridge, pp 193–247
Chapter Google Scholar
Long J, Song J, Zhang X, Deng M, Xie L et al (2020) Tea saponins as natural stabilizers for the production of hesperidin nanosuspensions. Int J Pharm 583:119406
Mahajan K, Rojekar S, Desai D, Kulkarni S, Vavia P (2020) Efavirenz loaded nanostructured lipid carriers for efficient and prolonged viral inhibition in HIV-infected macrophages. Pharm Sci 27:418–432
Mahajan K, Rojekar S, Desai D, Kulkarni S, Bapat G et al (2021) Layer-by-layer assembled nanostructured lipid carriers for CD-44 receptor-based targeting in HIV-infected macrophages for efficient HIV-1 inhibition. AAPS PharmSciTech 22:171
Mahesh KV, Singh SK, Gulati M (2014) A comparative study of top-down and bottom-up approaches for the preparation of nanosuspensions of glipizide. Powder Technol 256:436–449
Marin A, Andrianov AK (2011) Carboxymethylcellulose–chitosan-coated microneedles with modulated hydration properties. J Appl Polym Sci 121:395–401
Martin B, Seguin J, Annereau M, Fleury T, Kuen RL et al (2020) Preparation of parenteral nanocrystal suspensions of etoposide from the excipient free dry state of the drug to enhance in vivo antitumoral properties. Sci Rep 10:18059
McCrudden MTC, Larrañeta E, Clark A, Jarrahian C, Rein-Weston A et al (2018) Design, formulation and evaluation of novel dissolving microarray patches containing a long-acting rilpivirine nanosuspension. J Control Release 292:119–129
Menon I, Bagwe P, Gomes KB, Bajaj L, Gala R et al (2021) Microneedles: a new generation vaccine delivery system. Micromachines 12:435
Migdadi EM, Courtenay AJ, Tekko IA, Mc Crudden MTC, Kearney MC et al (2018) Hydrogel-forming microneedles enhance transdermal delivery of metformin hydrochloride. J Control Release 285:142–151
Misra SK, Pathak K (2020) Supercritical fluid technology for solubilization of poorly water-soluble drugs via micro- and nanosized particle generation. ADMET DMPK 8:355–374
PubMed PubMed Central Google Scholar
Moffatt K, Donnelly RF (2021) Microneedle technology. In: Eric C (ed) Drug delivery devices and therapeutic systems. Academic, New York, pp 345–366
Möschwitzer J, Achleitner G, Pomper H, Müller RH (2004) Development of an intravenously injectable chemically stable aqueous omeprazole formulation using nanosuspension technology. Eur J Pharm Biopharm 58:615–619
Mou D, Chen H, Wan J, Xu H, Yang X (2011) Potent dried drug nanosuspensions for oral bioavailability enhancement of poorly soluble drugs with pH-dependent solubility. Int J Pharm 413:237–244
Murakami Y, Shimoyama Y (2017) Production of nanosuspension functionalized by chitosan using supercritical fluid extraction of emulsion. J Supercrit Fluids 128:121–127
Nguyen X, Banga AK (2018) Delivery of methotrexate and characterization of skin treated by fabricated PLGA microneedles and fractional ablative laser. Pharm Res 35:68
Nir Y, Paz A, Sabo E, Potasman I (2003) Fear of injections in young adults: prevalence and associations. Am J Trop Med Hyg 68:341–344
Ohn J, Jang M, Kang BM, Yang H, Hong JT et al (2021) Dissolving candlelit microneedle for chronic inflammatory skin diseases. Adv Sci 8:2004873
Omatsu T, Chujo K, Miyamoto K, Okida M, Nakamura K et al (2010) Metal microneedle fabrication using twisted light with spin. Opt Express 18:17967
Panda BP, Krishnamoorthy R, Bhattamisra SK, Shivashekaregowda NKH, Seng LB et al (2019) Fabrication of second generation smarter PLGA based nanocrystal carriers for improvement of drug delivery and therapeutic efficacy of gliclazide in type-2 diabetes rat model. Sci Rep 9:17331
Panda A, Sharma PK, McCann T, Bloomekatz J, Repka MA et al (2022) Fabrication and development of controlled release PLGA microneedles for macromolecular delivery using FITC-Dextran as model molecule. J Drug Deliv Sci Technol 68:102712
Pardeshi S, Damiri F, Zehravi M, Joshi R, Kapare H et al (2022) Functional thermoresponsive hydrogel molecule to material design for biomedical applications. Polymers 14:3126
Park JH, Choi SO, Seo S, Choy YB, Prausnitz MR (2010) A microneedle roller for transdermal drug delivery. Eur J Pharm Biopharm 76:282–289
Patel HM, Patel BB, Shah CN, Shah DP (2016) Nanosuspension technologies for delivery of poorly soluble drugs—a review. Res J Pharm Technol 9:625–632
Patil AS, Hegde R, Gadad AP, Dandagi PM, Masareddy R et al (2021) Exploring the solvent–anti-solvent method of nanosuspension for enhanced oral bioavailability of lovastatin. Turk J Pharm Sci 18:541–549
Patravale VB, Date AA, Kulkarni RM (2010) Nanosuspensions: a promising drug delivery strategy. J Pharm Pharmacol 56:827–840
Pavan Kumar M, Madhusudan Rao Y, Apte S (2007) Improved bioavailability of albendazole following oral administration of nanosuspension in rats. Curr Nanosci 3:191–194
Pawar K (2017) Microneedles-based devices: regulatory insights. J Pharm Drug Deliv Res 06:1
Permana AD, McCrudden D (2019) Enhanced intradermal delivery of nanosuspensions of antifilariasis drugs using dissolving microneedles: a proof-of-concept study. Pharmaceutics 11:346
Permana AD, Paredes AJ, Volpe-Zanutto F, Anjani QK, Utomo E et al (2020) Dissolving microneedle-mediated dermal delivery of itraconazole nanocrystals for improved treatment of cutaneous candidiasis. Eur J Pharm Biopharm 154:50–61
Permana AD, Paredes AJ, Zanutto FV, Amir MN, Ismail I et al (2021) Albendazole nanocrystal-based dissolving microneedles with improved pharmacokinetic performance for enhanced treatment of cystic echinococcosis. ACS Appl Mater Interfaces 13:38745–38760
Peters K, Leitzke S, Diederichs JE, Borner K, Hahn H et al (2000) Preparation of a clofazimine nanosuspension for intravenous use and evaluation of its therapeutic efficacy in murine Mycobacterium avium infection. J Antimicrob Chemother 45:77–83
Pireddu R, Schlich M, Marceddu S, Valenti D, Pini E et al (2020) Nanosuspensions and microneedles roller as a combined approach to enhance diclofenac topical bioavailability. Pharmaceutics 12:1140
Porwal O (2022) Box-Behnken design-based formulation optimization and characterization of spray dried rutin loaded nanosuspension state of the art. S Afr J Bot 149:807–815
Qin M, Ye G, Xin J, Han W, Li M, Sui X, Dong H, Fu Q, He Z et al (2023) Comparison of in vivo behaviors of intramuscularly long-acting celecoxib nanosuspensions with different particle sizes for the postoperative pain treatment. Int J Pharm 636:1–8
Rabinow BE (2004) Nanosuspensions in drug delivery. Nat Rev Drug Discov 3:785–796
Rahim H, Sadiq A, Khan S, Amin F, Ullah R et al (2019) Fabrication and characterization of glimepiride nanosuspension by ultrasonication-assisted precipitation for improvement of oral bioavailability and in vitro α-glucosidase inhibition. Int J Nanomed 14:6287
Ramadon D, Ulayya F, Sakinah A, Iskandarsyah I, Harahap Y et al (2023) Combination of dissolving microneedles with nanosuspension and co-grinding for transdermal delivery of ketoprofen. Pharmaceuticals 16:378
Rojekar SV, Trimukhe AM, Deshmukh RR, Vavia PR (2021a) Novel pulsed oxygen plasma mediated surface hydrophilizatıon of ritonavır for the enhancement of wettability and solubility. J Drug Deliv Sci Technol 63:102497
Rojekar S, Fotooh Abadi L, Pai R, Mahajan K, Kulkarni S et al (2021b) Multi-organ targeting of HIV-1 viral reservoirs with etravirine loaded nanostructured lipid carrier: an in vivo proof of concept. Eur J Pharm Sci 164:105916
Rojekar S, Pai R, Abadi LF, Mahajan K, Prajapati MK et al (2021c) Dual loaded nanostructured lipid carrier of nano-selenium and etravirine as a potential anti-HIV therapy. Int J Pharm 607:120986
Rojekar S, Vora LK, Tekko IA, Zanutto FV, McCarthy H et al (2021d) Etravirine-loaded dissolving microneedle arrays for long-acting delivery. Eur J Pharm Biopharm 165:41–51
Rojekar S, Abadi LF, Pai R, Prajapati MK, Kulkarni S et al (2022) Mannose-anchored nano-selenium loaded nanostructured lipid carriers of etravirine for delivery to HIV reservoirs. AAPS PharmSciTech 23:230
Sattar A, Chen D, Jiang L, Pan Y, Tao Y et al (2017) Preparation, characterization and pharmacokinetics of cyadox nanosuspension. Sci Rep 7:2289
Saurer EM, Flessner RM, Sullivan SP, Prausnitz MR, Lynn DM (2010) Layer-by-layer assembly of DNA- and protein-containing films on microneedles for drug delivery to the skin. Biomacromol 11:3136–3143
Sharma M, Mehta I (2019) Surface stabilized atorvastatin nanocrystals with improved bioavailability, safety and antihyperlipidemic potential. Sci Rep 9:16105
Sharma P, Zujovic ZD, Bowmaker GA, Marshall AJ, Denny WA et al (2011) Evaluation of a crystalline nanosuspension: polymorphism, process induced transformation and in vivo studies. Int J Pharm 408:138–151
Shegokar R, Singh KK (2011) Surface modified nevirapine nanosuspensions for viral reservoir targeting: In vitro and in vivo evaluation. Int J Pharm 421:341–352
Shekhawat P, Pokharkar V (2019) Risk assessment and QbD based optimization of an eprosartan mesylate nanosuspension: in vitro characterization, PAMPA and in vivo assessment. Int J Pharm 567:118415
Singare DS, Marella S, Gowthamrajan K, Kulkarni GT, Vooturi R et al (2010) Optimization of formulation and process variable of nanosuspension: an industrial perspective. Int J Pharm 402:213–220
Singh SK, Srinivasan KK, Gowthamarajan K, Singare DS, Prakash D et al (2011) Investigation of preparation parameters of nanosuspension by top-down media milling to improve the dissolution of poorly water-soluble glyburide. Eur J Pharm Biopharm 78:441–446
Singh TRR, McMillan H, Mooney K, Alkilani AZ, Donnelly RF (2017) Fabrication of microneedles. In: Dragicevic NI, Maibach H (eds) Percutaneous penetration enhancers physical methods in penetration enhancement. Springer, Berlin, pp 305–323
Sonavane K, Phillips J, Ekshyyan O, Moore-Medlin T, Roberts Gill J et al (2012) Topical curcumin-based cream is equivalent to dietary curcumin in a skin cancer model. J Skin Cancer 2012:1–9
Sun W, Mao S, Shi Y, Li LC, Fang L (2011) Nanonization of itraconazole by high pressure homogenization: stabilizer optimization and effect of particle size on oral absorption. J Pharm Sci 100:3365–3373
Tekko IA, Permana AD, Vora L, Hatahet T, McCarthy H et al (2020) Localised and sustained intradermal delivery of methotrexate using nanocrystal-loaded microneedle arrays: potential for enhanced treatment of psoriasis. Eur J Pharm Sci 152:105469
Trimukhe A, Rojekar S, Vavia PR, Deshmukh RR (2021) Pulsed plasma surface modified omeprazole microparticles for delayed release application. J Drug Deliv Sci Technol 66:102905
Tucak A, Sirbubalo M, Hindija L, Rahic O, Hadziabdic J et al (2020) Microneedles: characteristics, materials, production methods and commercial development. Micromachines 11:961
Ullah A, Kim CM, Kim GM (2018) Porous polymer coatings on metal microneedles for enhanced drug delivery. R Soc Open Sci 5:171609
Vallhov H, Xia W, Engqvist H, Scheynius A (2018) Bioceramic microneedle arrays are able to deliver OVA to dendritic cells in human skin. J Mater Chem B 6:6808–6816
Verma S, Gokhale R, Burgess DJ (2009) A comparative study of top-down and bottom-up approaches for the preparation of micro/nanosuspensions. Int J Pharm 380:216–222
Vora LK, Vavia PR, Larrañeta E, Bell SEJ, Donnelly RF (2018) Novel nanosuspension-based dissolving microneedle arrays for transdermal delivery of a hydrophobic drug. J Interdiscip Nanomed 3:89–101
Vora LK, Moffatt K, Tekko IA, Paredes AJ, Zanutto FV et al (2021) Microneedle array systems for long-acting drug delivery. Eur J Phar Biopharm 159:44–76
Walsh L (2019) Microneedling: a versatile and popular treatment option. J Aesthet Nurs 8:280–284
Wang Y, Zheng Y, Zhang L, Wang Q, Zhang D (2013) Stability of nanosuspensions in drug delivery. J Control Release 172:1126–1141
Wang M, Hu L, Xu C (2017) Recent advances in the design of polymeric microneedles for transdermal drug delivery and biosensing. Lab Chip 17:1373–1387
Wang P, Cao X, Chu Y (2019a) Ginkgolides-loaded soybean phospholipid-stabilized nanosuspension with improved storage stability and in vivo bioavailability. Colloids Surf B 181:910–917
Wang C, Cui B, Guo L, Wang A, Zhao X et al (2019b) Fabrication and evaluation of lambda-cyhalothrin nanosuspension by one-step melt emulsification technique. Nanomaterials 9:145
Watanabe T, Ohno I, Wakiyama N, Kusai A, Senna M (2002) Stabilization of amorphous indomethacin by co-grinding in a ternary mixture. Int J Pharm 241:103–111
Wei F, Wang Q, Liu H, Yang X, Cao W et al (2022) High efficacy combined microneedles array with methotrexate nanocrystals for effective anti-rheumatoid arthritis. Int J Nanomed 17:2397–2412
Wu Y, Vora LK, Mishra D, Adrianto MF, Gade S et al (2022) Nanosuspension-loaded dissolving bilayer microneedles for hydrophobic drug delivery to the posterior segment of the eye. Biomater Adv 137:212767
Xenikakis I, Tsongas K, Tzimtzimis EK, Katsamenis OL, Demiri E et al (2022) Transdermal delivery of insulin across human skin in vitro with 3D printed hollow microneedles. J Drug Deliv Sci Technol 67:102891
Xia D, Quan P, Piao H, Piao H, Sun S et al (2010) Preparation of stable nitrendipine nanosuspensions using the precipitation–ultrasonication method for enhancement of dissolution and oral bioavailability. Eur J Pharm Sci 40:325–334
Xiang H, Xu S, Li J, Pan S, Miao X (2022) Particle size effect of curcumin nanocrystals on transdermal and transfollicular penetration by hyaluronic acid-dissolving microneedle delivery. Pharmaceuticals 15:206
Yadav V, Sharma PK, Murty US, Mohan NH, Thomas R et al (2021) 3D printed hollow microneedles array using stereolithography for efficient transdermal delivery of rifampicin. Int J Pharm 605:120815
Yao L, Zhao X, Li Q, Zu Y, Fu Y et al (2012) In vitro and in vivo evaluation of camptothecin nanosuspension: a novel formulation with high antitumor efficacy and low toxicity. Int J Pharm 423:586–588
Yurdacan HM, Murat Sari M (2021) Functional green-based nanomaterials towards sustainable carbon capture and sequestration. In: Murat Sari M, Cenk T, Hakan CC, Luigi S, Ole T (eds) Sustainable materials for transitional and alternative energy. Gulf Professional Publishing, Oxford, pp 125–177
Zhang J, Xie Z, Zhang N, Zhong J (2017) Nanosuspension drug delivery system: preparation, characterization, postproduction processing, dosage form, and application. In: Ecaterina A, Alexandru Mihai G (eds) Nanostructures for drug delivery. Elsevier, Amsterdam, pp 413–443
Zhang XP, Zhang BL, Chen BZ, Zhao ZQ, Fei WM et al (2022) Dissolving microneedle rollers for rapid transdermal drug delivery. Drug Deliv Transl Res 12:459–471
Download references
Acknowledgements
The authors would like to thank the Department of Pharmaceutics, National Institute of Pharmaceutical Education and Research-Ahmedabad (NIPER-A), Gujarat, 382355, India, for providing resources for literature search and review writing.
Author information
Authors and affiliations.
Department of Pharmaceutics, National Institute of Pharmaceutical Education and Research-Ahmedabad (NIPER-A), Ahmedabad, Gujarat, 382355, India
Jyotsna G. Vitore, Bharathi Karunakaran, Siddharth Salve & Derajram Benival
Department of Pharmaceutical Sciences and Technology, Institute of Chemical Technology, Mumbai, 400019, India
Sakshi Pagar
Department of Pharmaceutics, National Institute of Pharmaceutical Education and Research, Kolkata, 700054, India
Nidhi Singh
Institute of Chemical Technology, Mumbai, Marathwada Campus, Jalna, 431203, India
Navnath Hatvate
Department of Pharmacological Sciences, Icahn School of Medicine at Mount Sinai, New York, NY, 10029, USA
Satish Rojekar
You can also search for this author in PubMed Google Scholar
Contributions
JGV: Data curation, Formal analysis, Methodology, Writing—original draft; SP: Data curation, Formal analysis, Methodology, Writing—original draft; NS: Data curation, Formal analysis, Methodology, Writing—original draft; BK: Data curation, Formal analysis, Methodology, Writing—original draft; SS: Data curation, Formal analysis, Methodology, Writing—original draft; SR, DB: Conceptualization, Data curation, Formal analysis, Validation, Investigation, Visualization, Methodology, Writing—original draft, Writing—review and editing.
Corresponding authors
Correspondence to Satish Rojekar or Derajram Benival .
Ethics declarations
Conflict of interest.
All authors (J.G. Vitore, S. Pagar, N. Singh, B. Karunakaran, S. Salve, N. Hatvate, S. Rojekar, and D. Benival) declare no conflict of interest.
Statement of human and animal rights
This article does not contain any studies with human and animal subjects performed by any of the authors.
Additional information
Publisher's note.
Springer Nature remains neutral with regard to jurisdictional claims in published maps and institutional affiliations.
Rights and permissions
Springer Nature or its licensor (e.g. a society or other partner) holds exclusive rights to this article under a publishing agreement with the author(s) or other rightsholder(s); author self-archiving of the accepted manuscript version of this article is solely governed by the terms of such publishing agreement and applicable law.
Reprints and permissions
About this article
Vitore, J.G., Pagar, S., Singh, N. et al. A comprehensive review of nanosuspension loaded microneedles: fabrication methods, applications, and recent developments. J. Pharm. Investig. 53 , 475–504 (2023). https://doi.org/10.1007/s40005-023-00622-1
Download citation
Received : 19 January 2023
Accepted : 17 May 2023
Published : 06 June 2023
Issue Date : July 2023
DOI : https://doi.org/10.1007/s40005-023-00622-1
Share this article
Anyone you share the following link with will be able to read this content:
Sorry, a shareable link is not currently available for this article.
Provided by the Springer Nature SharedIt content-sharing initiative
- Nanosuspension
- Dissolving microneedles
- Transdermal drug delivery
- Nanocrystals
- Controlled release
- Long-acting
- Find a journal
- Publish with us
- Track your research

An official website of the United States government
The .gov means it’s official. Federal government websites often end in .gov or .mil. Before sharing sensitive information, make sure you’re on a federal government site.
The site is secure. The https:// ensures that you are connecting to the official website and that any information you provide is encrypted and transmitted securely.
- Publications
- Account settings
Preview improvements coming to the PMC website in October 2024. Learn More or Try it out now .
- Advanced Search
- Journal List
- v.27(1); 2019 Jun
Formation of nanosuspensions in bottom-up approach: theories and optimization
Ali ahmadi tehrani.
1 Department of Pharmaceutics, Faculty of Pharmacy, Shahid Sadoughi University of Medical Sciences, Yazd, Iran
2 Pharmaceutical Research Center, Shahid Sadoughi University of Medical Sciences, Yazd, Iran
Mohammad Mahdi Omranpoor
Alireza vatanara.
3 Department of Pharmaceutics, Faculty of Pharmacy, Tehran University of Medical Sciences, Tehran, Iran
Mohammad Seyedabadi
4 Department of Pharmacology, School of Medicine, Bushehr University of Medical Sciences, Bushehr, Iran
Vahid Ramezani
Nanosuspensions, liquid dispersions with nanometer size distribution, are becoming trendy in pharmaceutical practice to formulate poorly water-soluble drugs and to enhance their bioavailability. Generally, nanosuspensions are produced in two main approaches; top-down or bottom-up. The former is based on size-reduction of large particles via milling or high pressure homogenization. The latter is focused on the mechanisms of nucleation and particle growth.
In this review, the critical factors influencing the kinetics or dynamics of nucleation and growth are discussed. Subsequently, the mechanisms of nanosuspension instability as well as strategies for stabilization are elaborated. Furthermore, the effects of stabilizers on key parameters of instability as well as the process of choosing an appropriate stabilizer is discussed.
Steric and electrostatic stabilizations or combination of them is essential for nanosuspensions formulation to prevent coagulation. Accordingly, some characteristics of stabilizers play critical role on stability and optimization of nanosuspensions; i.e., HLB and concentration. Nevertheless, after reviewing various articles, it is ascertained that each formulation requires individual selection of surfactants according to the parameters of the particle surface and the medium.
Conclusions
Based on the results, application of excipients such as stabilizers requires proper optimization of type and concentration. This implies that each formulation requires its own optimization process.
Graphical Abstract

Introduction
Bioavailability is one of the limiting factors in drug development with chemicals having poor water solubility [ 1 – 5 ]. An array of solubilizing agents as well as various techniques have been employed to increase the solubility of such drugs, including surfactants, co-solvents, pH adjusted solutions, emulsions, liposomes, cyclodextrins and solid dispersions [ 6 , 7 ]. However, the majority of these techniques require additional excipients, which themselves can be a safety challenge [ 2 ]. In addition, these techniques offer little or no help in formulations where the parent molecules are poorly soluble in both aqueous and non-aqueous media [ 8 ].
The tendency of solute dissolution in specific solvent is determined by similar or dissimilar molecular interactions between the solute and solvent. In this way, environmental condition such as pressure and temperature influence the cohesion and adhesion force and eventually the intermolecular interactions. Of particular note, any further addition of solute above the solvent capacity leads to precipitation. All together Noyes–Whitney equation (Eq. 1 ), is very practical to get the valuable information about the process of dissolution.
Nanosuspensions, liquid dispersions with nanometer size distribution, help enhance the bioavailability of poorly soluble chemicals. In this regard, particle size as well as size distribution significantly influence the dissolution rate; thereby are among key determinants of the bioavailability. In particular, fine particles with high specific surface areas exhibit faster dissolution rate, which is demonstrated by Noyes–Whitney equation (Eq. 1 ) [ 7 , 9 – 11 ].
According to this equation (Eq. 1 ), dissolution rate of a particle (dc/dt) is inversely related to diffusion distance (h), and directly to the particle surface area (A) and saturation solubility [ 12 ]. Also, solubility is correlated to the rate of dissolution, albeit in some cases the case is more complex. Solubility is thermodynamically defined as the maximum amount of solute that can hold the pure solvent. So, solubility is affected by environmental conditions such as pH, temperature, ionic strength of solvent and solute. However, the rate of dissolution is a kinetic process. This implies that the extent of solubility of one solute can be poor or good independent of the rate of dissolution.
However, according to Ostwald–Freundlich equation (Eq. 2 ), in apposition to microparticles, the saturation solubility and equilibrium solubility of spherical particles with size of smaller than 1000 nm can influence the particle size.
That C s is solubility, C ∞ is solubility of the solid which consist of large particles, σ is interfacial tension substance and ρ is the solid density [ 13 ]. Also, according to Prandtl equation (Eq. 3 ) hydrodynamic boundary layer thickness will decrease and surface specific dissolution rate will increase by reduction of particle size [ 14 ].
Where L is length of the surface in the direction of flow, k is denotes a constant, V is relative velocity of the flowing liquid against a flat surface and h H is the hydrodynamic boundary layer thickness.
Also, the formation of nanosuspensions not only increases the surface area but also enhances the saturation solubility of the solute in medium, resulting in better bioavailability [ 12 , 15 – 18 ]. Moreover, the increase of mucoadhesivity and attachment to surfaces/cell membranes are other characteristics of nanosuspensions aiding in efficacious drug delivery [ 7 , 9 , 10 ]. Furthermore, nanosuspensions require no co-solvents, and allow higher drug loading compared with other formulations [ 19 ]. Better antitumor activity is also reported with nanosuspensions due to higher extravasation and remaining of particles at the vicinity of tumor [ 20 ].
Formulation of pharmaceuticals as nanosuspension was introduced in 1990, and the first product of this form appeared in the market in 2000 [ 10 ]. Ever since, a variety of micro- and/or nano- particles with proper size distribution have been widely used for drug delivery of poorly soluble chemicals [ 21 ]. These included of Rapamune® (sirolimus), Emend® (aprepitant), Megace®ES (Megestrole), Triglide® and Tricor® (fenofibrate), [ 8 , 22 – 24 ]. In the most cases, particles are stabilized using appropriate polymers and/or surfactants in nanosuspensions [ 8 , 25 ].
Generally, nanosuspensions are produced via either top-down or bottom-up processes [ 1 , 2 ]. While the former mainly includes size reduction via milling, the latter involves precipitation and supersaturation. The main factors determining an effective top-down formulation process are elaborated elsewhere [ 26 ]. Here, we review the mechanisms of nanosuspension formulation in bottom-up approach and, also, we elaborate the parameters influencing the quality and stability of these formulations.
Mechanism of nanoparticle formation
Top-down approaches are based on the size-reduction and breaking down of large materials into particles with nanometer dimensions via milling, high pressure homogenization and pulsed laser fragmentation [ 27 , 28 ]. Milling is performed using a rotating instrument in which particles are mixed with milling pearls with constant rotation, resulting in crystals or amorphous particles with reduced size [ 2 , 26 , 29 , 30 ]. However, high speed rotation may generate lots of heat, causing degradation of thermal-sensitive agents [ 2 ]. Also, milling can cause surface activation of drug particles, influencing several physiochemical properties of them such as their flow ability [ 31 ]. High-pressure homogenization (HPH) is also applied for nanocrystal production. In particular, the piston-gap homogenizer and microfluidizer are two main types of homogenizers frequently used for particle size reduction [ 29 ]. In this regard, several parameters are critical in HPH including pressure, cycle number, stabilizer type, temperature of process and stabilizing concentration [ 32 , 33 ]. Pulsed Laser Ablation (PLA) and Pulsed Laser Deposition (PLD) are advanced techniques based on the absorption of the energy by the material and transformation to thermal and/or chemical energy to break (inter) molecular bonds of the bulk material. This method usually leads to smaller particles with a wide size distribution which can be considered a disadvantages [ 28 ].
The Gibbs free energy will change during the size reduction in top-down process due to formation of new surfaces. This will result in thermodynamic instability of nanosuspension. Therefore, proper stabilizers are required to reduce the particle free energy [ 34 ]. The process of top-down nano formulation is discussed elsewhere [ 26 ] and is beyond the scope of this review.
The bottom-up approach is based on precipitation of supersaturated solutions [ 35 ]. It is frequently employed for the production of nanosuspensions both in bulk solutions or in single droplets [ 36 ]. This method is used in a number of pharmaceutical processes such as solvent–anti-solvent technique, supercritical fluid processing, spray drying, and emulsion–solvent evaporation [ 8 , 37 ]. Of particular note, nanoparticles are obtained after several steps including supersaturation, nucleation, diffusion of the solute molecules and nanoparticle growth [ 38 ].
The bottom-up approach offers various advantages such as production of monodisperse particles, i.e., narrow range of size distribution, application of low energy and low processing temperature for thermolabile drugs, and no need for advanced equipment and generally an economically procedure. Recently, some pharmaceutical products have been produced and marketed in this method, such as Griseofulvin (Gris-PEG®) and Nabilone (Cesamet®) [ 39 ]. In addition, an investigation has proved the nanosuspension of Danazol (gonadotropin inhibitor) has more improvement in comparison with micro-suspension of marketed Danazol [ 40 , 41 ]. Interestingly, fenoibrate [ 42 ] and Nitrendipine nanosuspensions were manufactured with the aid of combination methods [ 43 ].
However, generation of different unstable polymorphs, hydrates and solvates such as needle shaped particles caused by rapid growth can be undesirable. Also, application of non-solvents or anti-solvents are not favorable, given that even a few amount of residual medium can lead to physicochemical instability. Therefore, non-solvents are removed either via evaporative precipitation into aqueous solution (EPAS) or supercritical fluid (SCF) which need high pressure pump, temperature and fine nozzle designing.
Combination method
It is, however, noteworthy that some studies suggest a combination of top-down and bottom-up approaches as an optimal method for the particle preparation with size and stability. In this way, NANOEDGE™, H 69, CT (combination technology), H 96 and H 42 technology have been introduced to combine bottom-up and top down technology to reach effective reduction of particle size, e.g., preparation of atorvastatin nanoparticles via combination of anti-solvent precipitation after probe sonication method [ 44 ]. When these two methods are implemented simultaneously, a number of distinct nuclei with a certain size are created and inhibit the formation of nuclei with larger size. In addition, the combination of these methods leads to reach a narrow polydispersity index (PDI) and the range of particle size lies within a certain range that decreases the particle growth [ 2 , 24 , 35 , 44 , 45 ].
Nucleation and particle growth
Particle formation in bottom-up approach is initiated by nucleation followed by particle growth, which is, indeed, considered as the most important step in nanosuspension formulation [ 46 ]. As described by Reiss [ 47 ] and laMer and Dinegar [ 48 ], particle formation begins with short nucleation bursts and consequent molecule-by-molecule diffusion leading to particle growth. However, nucleation may result in either crystals or amorphous nanoparticles.
The first step of nucleation is monomer aggregation in supersaturated systems. So, the likelihood of addition of one monomer to the nucleus is directly proportional to its concentration in the bulk solution as well as its ability to diffuse to the nucleus surface [ 46 ]. In this regard, when the primary nucleus is spherical, the rate of diffusion of monomer ( Q ) follows the Fick’s law (Eq. 4 ); where R is the primary nucleus radius, D is the diffusion coefficient of monomers, C b is the concentration of monomer in the bulk solution, and S 0 is the solubility [ 46 , 49 ].
The free energy per monomer in the solution is higher than that in crystalline phase. Therefore, monomeric molecules tend to aggregate to maintain a low-energy state. Likewise, a small nucleus has higher surface area compared to large ones, resulting in higher interface interaction with solute molecules and higher level of energy. Hence, small nuclei combine together to reduce the system’s free energy, ultimately leading to the particle growth [ 46 ].
It is noteworthy that coagulation of nanoparticle are also determined by other factors, described in Navier-Stokes (NS) equations such as mass, momentum and energy conservation of Newtonian-fluids. NS equations are applicable for incompressible or compressible, low or high speed, inviscid or viscous flows. The compact form of Navier-Stokes equations for Newtonian fluid is (Eq. 5 ):
Where ρ is density, ∂ V ∂ t is change of velocity with time, ( V . ∇ ) V is convective term, ∇P is pressure term (fluid flows in the direction of largest change in pressure), ρg is bode force term (external forces acting on the fluid, such as gravity and electromagnetic) and μ∇ 2 V is viscosity controlled velocity diffusion. This equation (Eq. 5 ) represents how all Newtonian fluids move [ 50 ].
Kinetics of nucleation and particle growth
The rate of formation and growth of the nuclei is determined by Arrhenius model (Eq. 6 ). Here, K is the nucleation constant, E a is the activation energy, and A 0 is the pre-exponential factor. These parameters are, in turn, influenced by the number and orientation of particles, surface tension, medium viscosity, temperature, pressure and external driving forces [ 51 , 52 ]. In particular, E a diminishes at higher supersaturation, leading to higher nucleation probability and speed [ 53 ].
The effect of temperature
It is expected for particle growth to decrease at higher temperatures because of particle re-dissolution. However, the Brownian motions will also increase with temperature, leading to improvement of particle orientation and the force of intermolecular interactions, which are in favor of particle growth. Furthermore, temperature increases the particles hydrodynamic diameter (diameter of particle that is coated with hydrated counter ion), resulting in lower particle repulsion, ultimately leading to particle adhesion and nanoparticles growth. In fact, the effect of temperature on nucleation depends on the degree of supersaturation of solution [ 51 , 52 ].
The effect of surface tension
The rate of nucleation is a critical determinant of particle size and size distribution. In this regard, Arrhenius equation (Eq. 7 ) describes the effects of different variables on nucleation rate [ 54 ]. Of particular note, nucleation rate constant ( dN dt ) depends on the concentration of stabilizers. In fact, increase of stabilizer concentration in the solution decreases surface tension, thereby inhibits nucleation rate and increases crystallization time [ 53 ]. In addition, surfactants alter γ , the surface free energy, thereby modulate the nucleation speed [ 54 ].
The effect of supersaturation
The ratio of anti-solvent to solvent increases at high degree of supersaturation, resulting in a greater nucleation rate, which eventually enhances the number but decreases the size of particles. In fact, higher degree of supersaturation (S in Eq. 7 ) enhances nucleation rate, and eventually results in smaller particle size [ 45 ]. In this regard, small changes in S dramatically alters nucleation rate; Kwon and Hyeon showed that an increase of S by two folds (from 2 to 4) lead to a significant increase of nucleation rate up to 10 70 times [ 54 ]. It is noteworthy that particle growth is directly proportional to both particles count and their size. Thus, a shrink in particle size appears to neutralize the effects of supersaturation on particle number. Nevertheless, the overall outcome of an increase in supersaturation favors particle growth [ 45 ].
The effect of molecular diffusion
The nanoparticles diameter is determined according to LSW (Lifshitz–Slyozov–Wagner) model [ 51 , 55 – 57 ]; where n depends on the formation and growth of the particles.
When n is 2, particle growth is mainly controlled by ions diffusion in the matrix; i.e., diffusion of ions in solution. Meanwhile, when n is 3, the particle growth depends on volumetric diffusion in the matrix; i.e., diffusion of ions on the particle surface. Accordingly, n = 4 implies that dissolution kinetics at both particles surface and the matrix ought to be counted in particle growth, demonstrating dissolving-precipitation phenomena at the solid-liquid interface [ 51 , 56 ]. It is noteworthy that n can never be 1 and the 1/n exponent would always be less than 1, because particle size does not follow first order kinetics model. In fact, a decrease in the surface/volume ratio due to particle growth tends to negatively influence the ripening rate ( k ) due to reducing the available surface for deposition.
In this regard, k is correlated to diffusion coefficient of solute molecules in the medium, bulk solublity of dispersed phase ( C ∞ ), the solid-liquid interfacial tension (γ) and the molar volume of dispersed phase ( V m ) [ 55 ]. As demonstrated in Eq. 9 , k is almost constant in specified isothermal systems.
The surface nucleation, a critical component of particle growth, varies in different systems and depends on the dimensions of length and time, τ and k 1 respectively (Eq. 10 ) [ 58 , 59 ].
The crystal growth depends, also, on the concentration of bulk solution as well as its related concentration to crystal surface. In particular, the rate of crystal growth ( R G ) is determined by Eq. 11 ; where k G is the coefficient of growth rate, C b is the solute concentration in bulk solution, C ∗ is the solute concentration in equilibrium after nanosuspension formation, and g is the total growth condition [ 60 ].
The crystal growth is composed of two sequential steps. The first step involves solute transfer to the interfacial solid-liquid surface; i.e., the diffusion of monomers to the surface. In the next step, the solute integrates with the solution and separates from the crystal lattice.
Thermodynamics of nucleation and particle growth
The effect of free energy.
The bottom-up approach in nanosuspension formulation is based on the supersaturation of the solution followed by precipitation of the nanoparticles. Assessment of the thermodynamics of homogenous nucleation has revealed that the Gibbs free energy of nanoparticles is determined by the sum of surface free energy of nanoparticles and bulk free energy (Eq. 12 ). Accordingly, the bulk free energy ( ∆G v ) is calculated by Eq. 13 . In particular, ∆G v is directly proportional to temperature (T), Boltzmann constant ( k B ), degree of supersaturation (S), and inversely to the molar volume ( v ) [ 46 , 54 , 61 ].
The rate of particle formation, stabilization, and size, depend on the sum of free energy (Eqs. 14 and 15 ). In fact, the higher the total free energy, the lower the rate of particle formation. It should be noted that the surface free energy of particles in solution is more than in crystal form. Thus particles tend to form large agglomerates or precipitate in the form of crystals. Of particular note, the radius of critical nuclei ( r crit ), nuclei with maximal free energy per monomer without further re-dissolution in medium, is directly proportional to their free energy ( ∆G crit ) [ 46 , 54 , 61 ].
The precipitation of nanoparticles correlates with the degree of supersaturation. Indeed, the lower the degree of supersaturation, the slower the rate of nucleation. In this regard, “metastable zone” is a concentration range at which nucleation does not occur regardless of time. During the nanosuspension generation process, the concentration of solute molecules rise to form “clusters” which are prerequisites for nanoparticle formation [ 46 ].
According to the Eq. 7 , the rate of clustering of molecules as well as its thermodynamics depends on the degree of supersaturation and the molar volume of the nucleus. In fact, solute molecule continues to cluster until ∆G crit becomes negative. In this regard, ∆G crit , Gibbs free energy to form the critical nucleus, is calculated by Eq. 16 ; where γ sl is the surface tension between liquid and solid surface [ 46 ].
As demonstrated in Eq. 16 , the degree of supersaturation is inversely related to ∆G crit . Thus, solutions become thermodynamically unstable and tend to crystallize when the degree of supersaturation increases [ 53 ].
The steady state concentration in which clusters are stable ( C n ∗ ) is close to the equilibrium concentration, and is calculated using Eq. 17 . Here, ∆G crit represents the free energy of forming a critical cluster from free monomers, and C tot is the total concentration of substance in the system, which is approximately equal to the free monomer concentration [ 46 ].
For the assessment of ∆G crit , the capillarity approximation has been used. In other words, the free energy of a cluster is established on the free energy of a macroscopic solid and the cluster–water interfacial tension [ 62 ]. The formation of critical clusters per unit of time represents the transport of monomers to the clusters, and is calculated by nucleation rate (J) in unit of bulk volume in steady state concentration (Eq. 18 ); where Z is Zeldovich factor, and ψ ∗ = r crit /(λ + r crit ) [ 46 ].
The nucleation rate is related to diffusion and λ (effective length) as well as the interfacial tension ( γ sl ) and C b / S 0 , which is dependent to degree of supersaturation. It is noteworthy that when a change in λ is compensated by simultaneous change in γ sl , the nucleation rate maintains a constant value [ 46 ].
Some polymers with surface adsorption can enhance nucleation rate. In fact, these polymers ought to modulate one or more of the parameters discussed above. When, a specific polymer produces distinct effects on each parameter, the geometric sum of effects determines the final outcome [ 53 ].
Thermodynamics of surfactant adsorption and nucleation
Generally, nanosuspension is assuming a dispersion of hydrophobic nanoparticles in water as a hydrophilic medium. The enormous increase of surface area due to reduction of particle size results in increase of particles’ free energy while The interfacial tension remains constant, and is considered the main reason of thermodynamic instability of nanosuspensions [ 8 ]. Accordingly, to reduce the total surface energy of the system, nanoparticles tend to accumulate and reduce the total surface area with flocculation aggregation or crystal growth. Thus, crystallization as the main step in bottom-up approach can itself result in instability of nanosuspension, if is not appropriately controlled. In this regard, stabilizers are added to reduce the free energy of the system by decreasing the interfacial tension. This is fulfilled through electrostatic or steric stabilization or both [ 55 ].
The effect of surfactant lipophilicity and concentration
The adsorption of surfactants and polymers on the surface of nanoparticles play a pivotal role in the formation and stabilization of nanosuspensions. Furthermore, not only the surfactant adsorption to the solid surface but also its orientation influences the energy in the case of ∆G, ∆H and ∆S. In this regard, the hydrophilic: lipophilic balance (HLB) of surfactants is a critical parameter [ 63 ]. In fact, a change in the energy levels of the adsorbate species, the interfacial plate, and free surfactant in the bulk solution ultimately leads to the selective partitioning of the adsorbate into the interfacial region [ 63 ].
The mixture of surfactant/polymer and drug crystallites determines the melting point depression, and depends on their concentration, though their relationship is not necessarily linear [ 64 ]. The concentration of surfactant at the interface ( C interface )is in balance with its’ bulk concentration ( C bulk ), and is calculate by Eq. 19 . In this regard, the negative value of Gibbs free energy indicates the favorable conditions for absorption of surfactant from the bulk medium to the interface.
- The effect of dispersion medium
The absorption of surfactants in the solid-liquid interface depends on several factors in the solid plate, in the surfactant, and in the medium. The latter includes the nature of solvent, pH, temperature, ionic strength, lateral interaction between the adsorbed molecules, and presence of other dissolved materials, i.e., electrolytes or short chain additives such as alcohol an urea [ 63 , 65 ].
An array of interactions is demonstrated at the interface such as electrostatic, covalent, hydrogen or non-polar bonds [ 64 , 65 ], among which hydrogen bonds strongly influence the nucleation process [ 53 ]. Albeit, a specific type of interaction is dominant for each polymer. In this regard, polymers like PVP, with only one hydrogen bonding functional group per monomer, are not strongly adsorbed onto the crystal faces, and are less likely to induce habit modification [ 53 ]. For example, Lindfors L. et al., found that the growth rate significantly decreased in the presence of 0.01% ( w /w) PVP, whereas nucleation rate was not affected. This is explained by similar values of D, λ, γ sl , and C b / S 0 with and without PVP, small critical radius, and the fact that there is no significant polymer adsorption on these small particles [ 46 ].
Although hydrogen bonds play the main role in surface adsorption, the sum of all additive forces determines the level of free energy. For instance, Celecoxib is more likely to produce hydrogen bonds with the hydroxyl group of hydroxypropyl cellulose (HPC) than with the ether group of Pluronic F-127 (PLU), but the latter produce stronger effects on crystallization. This indicates that the final effect on crystallization is determined by the sum of interactions [ 64 ]. Thus, ∆G ads is considered when analyzing the surface energy (Eq. 20 ). In fact, the level of energy is a combination of electric attraction force between solid plate and the surfactant ( ∆G elec ), covalent bonding ( ∆G chem ), cohesive chain-chain interaction among adsorbed long chain surfactant species ( ∆G c − c ) and similar interactions between the hydrocarbon chains and hydrophobic sites on the solid plate ( ∆G c − s ), hydrogen bonding ( ∆G H ), and the solvation or de-solvation energy ( ∆ G H 2 O ) [ 65 ].
This implies that several processes at the interface including ion exchange (the exchange of surfactants with opposite charges), ion pairing (the absorption of surfactants in non-occupied positions), acid-base interaction (Lewis acid and base interaction with hydrogen bonds), π electron polarization (the force between side chains with positive charge), dispersion force (such as London and Van Der Waals forces), hydrophobic interactions (interaction between the non-polar side chains of surfactant and hydrophobic surface of nanoparticles) [ 63 ].
The effect of interfacial layer thickness
The thickness of interfacial layer ( τ ) is of notable importance. In this regard, the level of energy at the interface depends on the concentration of surfactant as well as the interfacial thickness (Г i ) (Eq. 21 ) [ 65 ].
The interface is a very dynamic region. In fact, diffusion, convection, adsorption, and desorption of stabilizer on the new surface of nanoparticle can occur simultaneously [ 66 – 68 ]. The physicochemical property of new particle surfaces, medium and surfactant is critical to minimize the Gibbs free energy. Thus, stabilization of a specific nanosuspension demands selection of a stabilizer with appropriate properties [ 69 ]. Moreover, the mixture ought to be incubated for a specific time span to ensure proper coverage of the surface; the length of which depends on physicochemical properties of the surfactant such as molecular weight and hydration value as well as the viscosity of medium [ 70 ].
The effect of surface polarity
Surface polarity influences the surface interaction of not only ionic but also non-ionic surfactants. In fact, the absorption of nonionic surfactant on the articles depends on the chemical potential of bulk solution, and is defined by Flory-Huggins Eq. (Eq. 22 ); where, the s represents surface, and q is the number of solute segments in contact with the surface. The m is the number of segment-surface nearest neighbors divided by the lattice coordination number, and r is the molecular size ratio of solute to solvent. The net interaction between components 1 (water as solvent) and 2 (surfactant as solvate) is expressed as χ 1, 2 . In this regard, χ 1, s − χ 2, s indicates the change of surface contact from solvent to solute, and χ 1 , 2 − χ 1 , 2 s represents the differential strength of solute-solvent interaction in the bulk solution and in the surface phase [ 71 ].
This model is applied for the absorption of nonionic surfactant to the non-polar surface such as polystyrene. In this regard, approximately 20% of free energy of absorption of nonionic surfactants on latex surface is due to the replacement of surface-water contacts with surface-surfactant contacts, i.e., (χ 1, s − χ 2, s ). The remaining 80% is related to the orientation of surfactant molecules at the surface, expressed as χ 1 , 2 − χ 1 , 2 s .
At the interface, the hydrophilic parts of surfactant molecule are in contact with water, whereas hydrophobic parts orient toward particle surface [ 71 ]. This indicates lower possibility of unfavorable contacts of hydrocarbon chain to the aqueous solution. Therefore, the water–surfactant interaction at the surface will be weaker than that in bulk solution (χ s < χ).
The χ parameter is the sum of fractional effects of interactions between water-hydrophilic moiety of surfactant, water-hydrophobic moiety of surfactant, water-water, and hydrophilic-hydrophobic moieties. Hence, χ is related to the HLB of surfactant and the HLB will be HLB = 20 ω hydrophilic in which ω hydrophilic is the weight fraction of hydrophilic part of surfactant [ 71 ].
Nanosuspension instability
The process of particle formation in nanosuspensions generally follows 2 steps, nucleation and particle growth. In this regard, uncontrolled growth can cause instability of the suspension (Fig. 1 ) [ 60 , 61 ]. Instability at some, but not all, levels is reversible with appropriate intervention. Thus, proper control of the processing time is critical to get nanoparticles with desirable size without instability.

Schematic of particle precipitation process. Reprinted with permission from [ 159 ]. Copyright (2009) American Chemical Society
Particle growth in nanosuspensions is explained by various mechanisms, including aggregation [ 55 , 56 ], coalescence [ 56 , 61 , 72 ], crystal growth [ 55 , 60 , 73 ], Ostwald ripening [ 55 , 74 , 75 ], orientated attachment [ 56 , 76 ], self-assembly, and mesoscopic transformation of nanoparticles via a restructuring process [ 77 – 79 ]. Depending on the system, one or several mechanisms may be involved in nucleation and growth of particle size [ 56 ].
Particle agglomeration is the outcome of attractive forces between the particles in the absence of considerable energy barrier; the latter is proven to be more important [ 80 ]. In this regard, high levels of hydrophobicity of particles decrease the wettability of their surface, resulting in aggregation [ 81 ]. Accordingly, hydrophobic particles tend to agglomerate to reduce the system free energy, as shown in the case of naproxen particles [ 82 ].
The lyophobic nanosuspension is produced by dispersion of hydrophobic materials in hydrophilic aqueous medium. In this system, increase of the surface area, because of nano-formulation, enhances the contacts between particles and outer medium, ultimately resulting in high surface energy. This can cause thermodynamic instability [ 8 ] and aggregation of nanoparticles [ 81 ].
Mechanisms of nanosuspension instability/stabilization
As discussed above, the mechanisms of nanoparticle growth and instability are similar. In fact, inappropriate growth can cause instability of the system. Hence, both formation and instability of nanoparticles can be determined by similar variables and principles.
- Nanoparticle growth in lamer mechanism
The nucleation and particles growth is divided into 3 steps according to lamer mechanism. At first, increase in monomer concentration in supersaturated solution causes nucleation bursts in the medium. This results in a decrease of monomer concentration, and if continued, halts the process of nucleation. If monomer concentration is high enough to prevent nucleation arrest, the nuclei will grow due to monomer diffusion of supersaturated solutions [ 54 , 83 ]. Thus, monomer concentration as well as factors influencing its diffusion plays pivotal roles not only in nucleation and growth but also in system stability. In this regard, storage at low temperatures, removal of residual solvents, applications of steric barrier around the particles, higher viscosity and lower diffusion coefficients of the medium ought to reduce monomer diffusion to the surface and inhibit particle growth (Eqs. 9 , 10 and 11 ).
- Ostwald ripening
Ostwald ripening was introduced in 1900 by Gibbs–Thomson law, and is based on the changes in the solubility of nanoparticles according to their size. It can cause significant instability in nanosuspensions [ 2 ], especially in long term storage [ 55 ]. In fact, higher solubility of nanoparticles causes re-dissolving and elimination of small particles. In the meantime, large particles tend to grow inappropriately because of low level of surface energy [ 84 , 85 ].
Owing to application of surfactants in nanosuspension formulations and because of their adsorption at particles surface, Ostwald ripening is illustrated by Lifshitz–Slyozov–Wagner (LSW) theory (Eq. 23 ). Here, r is the radius of spherical nanocrystal, t is the time of radius changing, and K D is the diffusion coefficient. The ω is monomer flux coefficient which demonstrates the ease of material movements in the surfactant layer [ 74 ].
In LSW theory, the term ∫ 0 ∞ ρ 2 h ρ dρ is specific for each system. For instance, in the case of bare-nanocrystals it equals to 9/4. However, if surfactants are utilized, one ought to consider the thickness of surfactant layer on the nanoparticle surface (δ) (Eq. 24 ) [ 74 ].
The kinetics of Ostwald ripening is determined by two basic parameters; the ability of solute molecules to diffuse to the particle surface, and the rate of crystal growth/dissolution by attachment-detachment of the solute. In this regard, the ripening is controlled by the slower step. That is, Ostwald ripening becomes diffusion controlled, if crystal growth/dissolution is rapid; and it becomes interface controlled, when solute diffusion is faster than its incorporation/elimination to/from the particles [ 55 ].
- The effects of surfactants
According to Eq. 24 , surfactants influence diffusion coefficient as well as solubility and surface tension. It is also noteworthy that growth depends on t, though not linearly. Thereby, surfactants have an enormous effect on the process of ripening. In fact, they decrease growth by reducing Ostwald ripening [ 74 ]. In contrast, according to the LSW theory, the concentration of the dispersed phase directly influence the rate of Ostwald ripening [ 2 ]. Therefore, surfactants with higher solubilizing property, causing a rise in particle concentration in bulk solution, increase the rate of Oswald ripening [ 2 ].
- The effects of size distribution
Size distribution is another determinant of particle growth. In this regard, Ostwald ripening requires poly disperse particles with some solubility in medium [ 55 ]. Because large particles tend to grow and small particles tend to fade, a narrow size distribution is essential to prevent particle growth due to Ostwald ripening and to maintain the stability of nanosuspension [ 86 ].
- Digestive ripening
Digestive ripening is another mechanism describing the effects of particle size on the stability of nanosuspensions. In contrast to Ostwald ripening, digestive ripening argues that it is possible to reconstruct particles in order to increase the stability of system. In fact, the ultimate goal is to enhance dissolving of large particles and growth of small particles [ 87 ].
- Finke-Watzky two step mechanism
Finke-Watzky mechanism illustrates nucleation and growth as consequent events. In this mechanism, the particle growth is autocatalytic with the formed nucleus, and is defined by Eqs. 25 and 26 [ 88 ]. The formation of nucleus is a chemical process, and is beyond the scope of this review. However, a fraction of substance A is primarily converted to substance B. Then A and B bind together, and two moles of B are ultimately formed. Consequently, particles grow in size during this process.
- Coalescence and orientated attachment
Coalescence and orientated attachment describe particle growth in a similar manner. The former defines particle attachment with no orientation, yet the latter demands crystallographic alignment of particles for their attachment, including continuous crystallographic planes [ 76 ].
Equation 27 shows the relationship between the rate constant (K 2 ) of orientated attachment reaction and particle radius (r); the N A is the Avogadro constant. In particular, the rate of particle growth is directly proportional to particle radius and diffusion coefficient (D A ) [ 56 ].
Equation 28 represent relationship between rate constant (K 2 ) of orientated attachment reaction and viscosity. On the other hand, the correlation between D A in Eq. ( 27 ) and viscosity is illustrated with Stokes-Einstein model (Eq. 31 ). Also, Eq. 28 argues that particle growth is directly related with temperature (T) and is inversely associated with viscosity ( η ). Furthermore, viscosity of the solvent as well as the solid volumetric fraction ( ϕ ) determine the total viscosity of system (Eq. 29 ), thereby influencing the kinetics of particle growth [ 56 ].
It is noteworthy that viscosity depends also on temperature, according to Arrhenius model described in Eq. 30 [ 56 ]. Thus, the effects of viscosity on growth rate ought to be adjusted to temperature. In fact, increase of viscosity at low temperatures (around the melting point of solvent) significantly inhibits particle growth. In contrast, viscosity tends to remain moderately constant at high temperatures, conferring a linear relationship between growth rate (K 2 ) and temperature (Fig. 2 ) [ 56 ].
- The Influence of solids concentration in dispersion medium

Temperature dependence of the reaction rate constant. Reprinted with permission from [ 56 ]. Copyright (2005) John Wiley and Sons
Solid content of the system is another determinant of viscosity in Einstein equation (Eq. 29 ). In fact, the solid volumetric fraction ( ϕ ), assuming particles as highly dispersed rigid spheres, increases viscosity and, thereby, inhibits particle growth in colloidal nanosuspensions [ 56 ]. It is, therefore, suggested that dilution of nanosuspension, by reducing the total viscosity, promotes particle growth and increase particle size. For instance, Daebis et al., showed that reducing the viscosity of Itraconazol nanosuspension through serial dilution resulted in particle growth from 682.3 nm ± 55 nm (PDI 0.38 ± 0.05) to 828 nm ± 86.3 μm (PDI 0.48 ± 0.104) [ 89 ].
- The effect of stabilizers on system viscosity
According to Stokes-Einstein model (Eq. 31 ), the diffusion of a molecule is inversely proportional to its diameter (d). Also, temperature (T) influences the diffusion through alteration of systems viscosity [ 90 ]. For instance, HPMC enhances the viscosity much more than do Poloxamer 407 and PVA (Fig. 3a ). Hence, the former more effectively inhibits the diffusion of the solute molecules and particle growth [ 90 ]. In contrast, Poloxamer 407, with the lowest viscosity, results in higher growth rate (Eq. 28 ). In this regard, temperature more significantly influences the viscosity of solutions containing HPMC (Fig. (Fig.3b) 3b ) [ 90 ].
- Intra particle re-shaping

Dynamic viscosity of crystallization medium containing different polymers ( a ), and at different temperatures ( b ) ( n = 3). Reprinted with permission from [ 90 ]. Copyright (2012) Springer Nature
Intraparticle ripening explains the change of particle shape at the nanomaterial surface over time. It requires specific conditions in which the energy of monomers in bulk solution is lower or equal compared to that in crystal surface. This implies absence of monomer diffusion/influx to the particle surface. However, the irregularity of particle shape can cause subtle differences in surface energy on an individual particle, leading to dissolution of some regions (high energy surfaces) and growth of others (low energy surfaces) within a particle. Thus, an individual particle is constantly eroded at one side and formed at another side [ 91 , 92 ].
The role of particle morphology on stability
Although needle shape crystal structures are more common in bottom-up approach, an array of particle morphologies is possible such as amorphous, crystal or metastable [ 46 , 93 , 94 ]. For instance, preparation of nanoparticles with anti-solvent method produce amorphous, polycrystalline, single crystals or metastable forms [ 90 , 95 – 98 ]. Furthermore, localized amorphous particles are seen in some cases, which are the result of inhibition of crystal growth. This can lead to instability of the nanosuspensions, especially in long term storage [ 90 ].
Generally, application of inorganic materials such as calcium carbonate [ 99 , 100 ], cadmium sulfide [ 101 ], and zinc oxide [ 77 ] results in crystal formation with broad range of crystal habits. In contrast, organic materials in the formulation give amorphous products or crystals with varied shapes [ 90 , 95 ].
The morphology of particles influence their stability through several mechanisms such as Ostwald ripening [ 102 ]. In particular, the mobility of drug molecules in crystal structure is less than that in amorphous form [ 34 ]. Also, the relatively high energy of amorphous structures makes them unstable, thus they tend to transform to more stable crystal form [ 102 ]. The surface energy of crystals will rise, if the localized amorphous regions are generated on the surface [ 103 ]. This increases the chance of re-ordering or re-crystallization, and compromises physicochemical stability during formulation or long term storage [ 2 ]. Therefore, crystal particles confer better stability to the system [ 69 ]. However, this may interfere with the dissolution rate of nanosuspension [ 1 , 23 , 81 , 104 ].
The primary nanoparticles formed during the process are either amorphous or metastable [ 95 ]. The other particles are resulted from the aggregation of primary particles, which explains their varied forms as amorphous, polycrystalline, or single crystals [ 90 , 95 – 98 ]. However, recrystallization after aggregation may change the particles structure [ 96 – 98 ].
- The effect of surfactants
Surfactants or polymers in the formulation can influence the crystal state as well as crystallization rate. For examples, the type and concentration of surfactant has an important impact on crystallinity and size of amylase [ 105 ] and celecoxib particles [ 106 ]. Accordingly, PVP, but not PEG, distinctly improves the in vitro drug release behavior, and causes significant melting point depression and polymorphic change in celecoxib nanocrystals [ 64 ]. In addition, PVP and/or HPMC changed the morphology, size and crystallization rate of bicalutamide and griseofulvin nanoparticles [ 46 , 107 ]. Of particular note, PVP (0.01% w /w) inhibits crystal formation and alters the crystal structure to amorphous particles (Fig. 4 ) [ 46 ]. Surfactants, also, alter the crystal structure of buparvaquone [ 70 ] and isradipine [ 108 ]. In contrast, neither nanosizing nor surfactants influence the crystallinity or morphology of nifedipine particles [ 109 ].

Images from nucleation experiments carried out at an initial concentration drug concentration of 440 μM, corresponding to a supersaturation of 30: a polymer free system; b at the presence of PVP (0.01% w/w). The diameter of the wells is 5 mm. Reprinted with permission from [ 46 ]. Copyright (2008) Elsevier
An array of solvents or dispersion media are employed for the preparation of nanosuspensions, including methanol, acetone, ethanol, acetonitrile, liquid paraffin, isopropylmyristate, propylene glycol dicaprylate, caprylic/capric triglyceride, oleic acid, oleyl alcohol, triacetin, and propylene carbonate [ 108 , 110 , 111 ]. In this regard, the choice of an appropriate organic solvent or dispersion medium is critical to control the size and stability of drug nanocrystals during precipitation [ 110 ]. In fact, the crystal form as well as its behavior is determined by some parameters such as the composition of nanoparticles, the crystallization temperature, and presence of solvents/co-solvents [ 107 ].
Particle growth as well as morphology and behavior are influenced by residual solvents or impurities in the system. Of particular note, solvent polarity decreases the nanoparticle size and growth [ 107 ] In fact, impurities can interact physically or chemically with the surface [ 53 ] or can be incorporated in crystal lattice [ 46 ]. In addition, the hydrate, solvate or anhydrous forms of materials may be produced after nanosuspension formation [ 90 ]. It is noteworthy that the residual solvent or impurities in the particle can cause safety issues [ 2 , 8 ].
- The effect of external energies
The external energies such as sonication and homogenization are also applied to reduce particle size in bottom-up process. In the meantime, they can alter nanoparticle morphology or behavior [ 108 ]. In particular, homogenization can reduce the crystal size or even transform them into cubic or round structures. For instance, homogenization induces formation of amorphous layers around the crystals in buparvaquone nanosuspension [ 70 ]. In addition, simultaneous application of surfactants and homogenization may produce distinct effects on particle coating and morphology [ 70 ].
The role of surface tension and intermolecular forces on stability
Interfacial tension is a key factor in nucleation and growth [ 46 ]. As described previously in Eq. 7 , surface tension ( γ ) is inversely related to nucleation rate (J). Thus, reduction of surface tension increases nucleation rate, eventually decreasing the particle size and growth (crystallization) [ 46 , 112 – 114 ].
Surface tension in a system is defined as the interactions between different surfaces (Young–Laplace model, Eq. 32 ) [ 46 , 71 , 115 ]. Here, γ sl , γ sv , and γ lv represent the surface tension between solid-liquid, solid-vapor, and liquid-vapor interfaces, respectively. In addition, the interaction between particles and medium as well as crystal growth rate decrease with lower contact angel of solid-liquid interface (θ) [ 46 ].
According to Bragg–Williams theory (Eq. 33 ), the interfacial tension is directly related to the sum of intermolecular attractions (χ), including hydrogen, hydrophobic, dipole and Van Der Waals interactions [ 46 , 71 , 115 ]. In addition, the fraction of nearest neighbors in a plane below or above the interface (m) as well as the cross-sectional area per molecule (a) are critical [ 46 , 71 , 115 ]. This implies that the wider the nanoparticle surface, the higher the possibility of Van Der Waals interactions, thus, the higher the surface tension.
- Types of interparticulate forces
The forces between particles influence nanosuspension stability. In fact, interparticle forces alter the rate of adhesion/repulsion and consequently aggregation/disaggregation of nanoparticles in liquid medium. This include repulsive forces such as Van Der Waals, electrostatic, and hard sphere as well as attractive forces such as steric [ 116 ]. The DLVO theory (named after Derjaguin, Landau, Verwey and Overbeek) represents the stability of colloidal nanoparticles according to interparticle forces [ 117 ]. In this regard, interparticle force is the sum of adhesion and cohesion forces (Eq. 34 ) [ 63 ].
- Van Der Waals force
The Van Der Waals force is calculated by Eq. 35 ; where A is Hamaker constant, a is the radius of similar spherical particles, and H represents the minimum distance between two particles [ 118 ]. The Hamaker constant (A) in dispersed medium is replaced by A eff , which depends on the nature of dispersed particles and the medium (Eq. 36 ). The similar polarity of particles and medium results in lower A eff . According to Eq. 35 smaller values of A eff would lead to less attraction between the particles.
This decrease Van Der Waals repulsions, resulting in particle aggregation and instability. In this condition the potential energy will decrease [ 63 ].
- Electrostatic force
Electrostatic force with repulsive nature between two semi-charged particles is calculated by Eq. 37 ; where ε r represents the dielectric constant of the dispersion medium, and the a is hydrodynamic radial. In contrast to Van Der Waals force, the electrical force is always positive. Electrostatic force is greater when the distance between particles ( h ) is minimum. Furthermore, it highly depends on zeta potential ( ψ 0 ), electrolyte content of the medium, and the ratio of particle size to electrical double layer, a /(1/ κ ) = κ a , [ 63 , 119 ].
The effective electrical double layer is called Debye length (1/ κ ), and represents the thickness of stern layer (Eq. 38 ). Here, F is the Faraday constant, C i is the molar concentration of any ion in the solution phase, and Z i is the valence of the ions [ 63 ].
Of particular note, addition of electrolytes, via increase of κ and decrease of V R , can cause coagulation. In contrast, increasing the zeta potential ψ 0 as well as surfactants enhance the system V R and decrease particle coagulation.
Zeta potential is the net charge around the particles after neutralization with opposite ions in the medium [ 120 ]. Indeed, the first absorbed monolayer, the inner Helmholtz layer, is consist of ions with opposite charge to that of nanoparticle surface, and are fixed and dehydrated ions. The second monolayer, the outer Helmholtz layer, is composed of fixed but hydrated ions with similar charge to dispersion medium. Helmholtz layers together are called the Stern layer.
- Adjustment of surface tension and intermolecular forces
Intramolecular forces are controlled via two main mechanisms; electrostatic stabilization or steric hindrance. The former is based on the electrostatic interaction between the particle surface and ionic surfactants or charged polymers [ 121 ]. The later, however, describes adsorption of stabilizers onto the surface of drug nanoparticle via non-covalent forces [ 80 ].
- Electrostatic stabilization
As a general rule, nanosuspensions are stabilized by electrostatic repulsion with zeta potentials of at least 30 mv [ 9 , 70 , 86 , 122 – 124 ]. Furthermore, increase of zeta potential to 60 mv confers more stability to the formulation, whereas vast aggregation occurs in zeta potentials lower than 5 mv [ 125 , 126 ]. However, to stabilize nanosuspensions containing both electrostatic and steric stabilizers, an absolute zeta potential of ±20 mv appear to be sufficient [ 9 , 70 , 86 , 122 , 123 ].
- The effect of ionic strength of dispersion medium
The ionic strength of the medium is a key factor that influences the inter-particles electrostatic repulsive forces and inhibits particle aggregation [ 127 ]. Electrostatic stabilization is a simple and low cost method for nanosuspension stabilization [ 128 ]. According to Eqs. 37 and 38 , increase of the ionic strength reduces the thickness of double layer, leading to a fall in the repulsion force between particles [ 127 ]. The change in ionic strength could be due to ingredients in the formulation or nanosuspension contact with body fluids. In this regard, body fluids exhibit a broad range of pH and electrolyte content [ 125 ]. Also, processing conditions such as heat sterilization [ 129 ] or changing the medium pH [ 130 ] can alter the electrostatic strength. Thus, alternative methods of sterilization such as filtration or buffering systems are recommended to prevent instability of the formulation [ 131 ].
Surfactants/polymers decrease electrostatic force and change the dielectric constant of medium. Therefore, they diminish repulsion between nanoparticles [ 132 ]. Zeta potential is influence by the type as well as the concentration of employed stabilizers or electrolytes in the medium [ 70 ]. In this regard, steric stabilizers, such as HPMC and MC, alter the zeta potential through surface coverage [ 55 ]. As such, negatively or positively charged surfactant/polymers influence the total zeta potential of nanoparticles. For instance, negatively charged ionic surfactants, such as SLS, improves the Helmholts internal layer as well as the negative zeta potential in a concentration dependent manner [ 55 ]. Accordingly, increase of the concentration of low molecular weight chitosan enhances the positive charge at the surface of atorvastatin nanocrystal [ 44 ]. Although, high zeta potential inhibits the nanosuspension aggregation, it may impair interactions between nanoparticles and surfactants with similar charge, resulting in inefficient coverage. In the same manner, electrolytes in the medium can diminish the charged layer around the particles, resulting in a decrease in zeta potential.
Electrical charges of the particles and surfactant are key players in surfactant adsorption to the surface. However, pH, temperature, light, chemical nature and composition of the particle surface and medium influence electrical charge [ 126 , 133 – 136 ]. For instance, change in the medium pH alters zeta potential, and causes repulsion between ibuprofen nanoparticles and HPMC [ 2 ].
- Steric hindrance
A substantial body of evidence indicates that polymers efficiently prevent nanoparticles from aggregation and agglomeration during preparation and/or incubation [ 1 ]. In this regard, the affinity of nanoparticle for the polymer is a critical determinant of the efficacy of stabilization [ 111 ].
Formation of steric barrier around the particles is one of the main mechanisms of stabilizer-induced inhibition of aggregation and agglomeration [ 1 ]. In fact, steric stabilizers decrease particle contacts with the medium as well as attraction forces between particles (Eq. 35 ). Accordingly, polymer-coated drug nanoparticles exhibit lower Brownian motions, which are another mechanism for inhibition of particle attraction and aggregation [ 70 , 137 ]. In addition, polymer adsorption prevents attachment/detachment of molecules of particle surface, thereby inhibiting Ostwald ripening [ 53 , 138 – 140 ]. Also, polymers and nonionic surfactants tend to increase the solution viscosity, which in turn provides a barrier against aggregation (Eq. 28 ).
Several variables of the particle, the medium and the stabilizer influence the efficacy of steric barrier. In this regard, polymers appear to provide a superior steric barrier compared with surfactant shaving small molecular structure such as sodium lauryl sulfate [ 69 , 141 ]. This is explained by more effective inhibition of steric repulsion due to fast/tight adsorption and effective surface coverage [ 89 , 141 , 142 ]. Of particular note, the thickness of polymer layer ought to be about 10 nm in order to provide efficient steric stabilization [ 70 , 143 ].
Adsorption of polymers onto hydrophobic surfaces is due to the hydrophobicity of polymer chain. On the other hand, the hydrophilic moiety of polymer interacts with medium. So, the chain morphology plays a pivotal role in polymer adsorption and steric stabilization [ 69 , 86 ]. In particular, long swinging hydrophilic chains appear to provide better steric stability and protect more efficiently against aggregation [ 144 ]. Furthermore, a range of particles (340–440 nm) were obtained in formulations which differed only on the molecular weight of the stabilizer, chitosan. Indeed, low molecular weight chitosan yielded smaller nanocrystals compared to moderate or high molecular weight counterparts [ 44 ]. However, polymers with higher molecular weight tend to reduce the rate of surface adsorption, which is also an essential determinant of stability [ 34 ].
Application of block copolymers is shown to provide better control/reduction of the particle shape and size [ 145 ]. In this regard, poloxamer is a block copolymer of polyethylene oxide (PEO), as hydrophilic segment, and polypropylene oxide (PPO), as hydrophobic segment. The latter moiety helps surface adsorption, while the former provides steric hindrance surrounding the particles, thus protecting against aggregation [ 89 ].
The efficacy of polymers in particle adhesion depends on temperature. For example, chitosan fails to produce steric stabilization to the atorvastatin nanocrystals at high temperatures (40 °C), resulting in agglomeration [ 45 ]. As such, the protective effects of HPMC against aggregation disappear, when temperature rises to 45 °C due to dehydration of polymer chains or Ostwald ripening [ 55 ].
Steric stabilization has several advantages over electrostatic stabilization. 1: the particles stabilized by steric hindrance are re-dispersible [ 146 , 147 ]; 2: dispersion medium elimination is possible even in high concentration of nano-crystals [ 7 , 148 ]; 3: addition of electrolytes during ‘salting out’ process does not influence the stability of formulation [ 149 , 150 ]; and 4: it can be adopted for systems with multiple phases [ 151 ].
Choosing the appropriate stabilizer
Stabilizers are extensively used in nanosuspension formulations to adjust thermodynamic and/or mechanical parameters in order to prevent or delay agglomeration [ 86 , 152 ]. Surfactants as well as polymers or a mixture of both are employed as stabilizers. In particular, an appropriate stabilizer with proper quantity confer particle stability via steric or electrical barriers, leading to balance of the surface energy [ 68 , 69 , 153 , 154 ].
Surfactants and polymers tend to decrease Van Der Waals forces, resulting in a decrease of Boltzmann constant (K B ), interfacial tension, and crystallization rate [ 155 ]. However, the effect of surfactants/polymers is not limited to the surface tension. Rather, they can increase the viscosity of system [ 53 , 112 ], which interferes with mass diffusion (D) from solution to solid–liquid surface, and decreases the growth rate (Eq. 31 ) [ 56 , 156 – 159 ]. In this regard, particle growth due to higher HPMCs ratio in formulation is ascribed to the increase of viscosity of solution [ 160 ].
An array of steric stabilizers are employed in the formulation of nanosuspensions (Table (Table1), 1 ), including hydroxypropyl methyl cellulose (HPMC) [ 34 , 109 , 161 ], hydroxypropyl cellulose (HPC), polyvinyl pyrrolidone (PVP K-30), Poloxamer (188 and 407), Vitamin E TPGS [ 34 , 162 ], serum proteins (e.g. albumin) [ 45 ] and polyvinyl alcohol (PVA) [ 6 , 7 ]. Accordingly, several electrostatic stabilizers are also used to provide stability to the formulation, including polysorbates (mainly Tween®80), sodium lauryl sulfate (SLS) [ 34 , 124 ], amphoteric surfactant (e.g. lecithin) [ 70 ], lipoid S75 [ 120 ], and eudragit® [ 163 ]. Serum proteins, such as albumin, were also extensively employed as emulsifier to help stabilize oil-in-water emulsions [ 45 , 164 ]. Hypothetically, serum proteins can also provide stability to nanocrystals because of their ability to interact with hydrophobic surfaces. Therefore, they confer steric barriers to nanocrystals aggregation and growth, especially during consecutive centrifugation and drying [ 165 , 166 ].
Stabilizers that used in various nanosuspension formulations
Stabilizers are not equal efficacious for all formulations. For example, PVP is effectively used in nanosuspension formulations of phenytoin [ 167 ], probucol [ 168 ], hydrocortisone acetate [ 53 ], celecoxib [ 64 ], ibuprofen [ 141 ] and griseofulvin [ 107 ]. Conversely, it fails to inhibit aggregation in itraconazole nanosuspensions because of low affinity to the particle surface. In this regard, more viscose polymers such as HPMC and MC have successfully been employed for the stabilization of itraconazole nanocrystals [ 169 ].
Surfactants can supply better wetting of the nanoparticles than do macromolecular hydrocolloids (PVP, HPMC and MC). This indicates better dispersion with the former [ 86 , 89 ]. In addition, high molecular weight polymers (Poloxamer 407) provide higher degree of steric hindrance, more size reduction, and better dispersion compared to their counterparts with short side chains (Poloxamer 188) [ 89 ]. However, Lee et al., reported no correlation between the molecular weight and steric repulsion [ 69 ].
Some nonionic surfactants like Tween®80, Cremophor EL, Pluronic F68, and TPGS are reported to inhibit the activity of P-glycoprotein efflux pumps, which are responsible for backward pumping of the drug molecules and decrease of their intracellular concentration. Also, surfactants enhance drug penetration and increase drug absorption via paracellular route. These mechanisms are additional to the effects of surfactants on particle behavior, and can explain better disposition of the drug molecules in target cells [ 170 ]. However, it should be noted that perturbation of the integrity of cell membrane can cause cytotoxicity.
- Effect of surfactant HLB
Hydrophilic Lipophilic Balance (HLB) is a key feature in surfactants and influences the outcome of their application in formulations [ 2 ]. Actually, it demonstrates the magnitude of water to lipid attraction of a specific surfactant; i.e., those with lower HLB are more lipophilic and vice versa. Thus, HLB values of surfactants can assist in selecting the proper stabilizer. Albeit, there are some studies which highlight that HLB is not an appropriate criterion for stabilizer selection. The HLB values of some stabilizers are summarized in the Table Table2 2 .
The HLB value of some stabilizer which is used for nanosuspensions stability
Generally, nanosuspensions are hydrophobic drugs dispersed in hydrophilic media. Therefore, hydrophobic drug molecules tend to have more interactions with hydrophobic surfactants (low HLB), resulting in more reduction of surface energy, better dissolution, and smaller particles. For instance, there is a positive correlation between the size of ibuprofen nanoparticles, as lyophobic materials, and the HLB value of non-ionic stabilizer in bottom-up approach (Fig. 5 ). Conversely, larger particles are obtained when polymer with higher HLB are implemented [ 2 ].

Particle size of ibuprofen suspensions as a function of HLB value of the stabilizer: precipitation. (♦) HPMCs, (▲) Tween®80, (●) Pluronic F-127 and (■) Pluronic F-68. Reprinted with permission from [ 2 ]. Copyright (2009) Elsevier
The favorable range of stabilizer HLB to get the minimum size and maximum stability of nanosuspension is 10–15 (Tables 1 and and2) 2 ) [ 1 , 2 ]. For example, among SLS (HLB 40), Span40 (HLB 6.7) [ 171 ] and Tween®80 (HLB 15), the latter was the best particle size reducer [ 172 ]. In this regard, surfactants with high HLB impair the ability of ionic stabilizers for size reduction. This is explained by inter-surfactant repulsion because of increase of particle charge density [ 44 ]. This phenomenon was observed in the preparation of atorvastatin nanocrystals; where labrasol® (HLB 12) was the best stabilizer compared with PVP, Tween®40 and Tween®80 with higher HLB [ 44 ]. However, each formulation requires individual selection of surfactants according to the parameters of the particle surface and the medium. It is noteworthy that surfactants with high HLB >14 may have harmful effects such as cytotoxicity, immunogenicity, or poor biodegradability [ 44 , 173 ].
- Effect of surfactant concentration
Application of surfactant with high concentration may adversely influence the nanosuspension formation and stabilization. In this regard, the surfactant solubility limit may cause precipitation at high concentrations. In addition, formation of a third layer around the particles can cause repulsion [ 105 ]. Accordingly, high concentrations of hydrophilic surfactants can promote nanoparticle dissolution through micelle formation, and cause instability via Ostwald ripening [ 69 , 141 , 174 ]. For instance, ibuprofen nanoparticles were more soluble in the presence of SLS, Tween®80 and Pluronic F-127, leading to erosion of small molecules and growth of large ones, i.e., instability of the formulation [ 2 ].
The concentration of stabilizer is a key factor in nanosuspension stability, and requires optimization for each formulation. At low concentrations, the efficacy of stabilizer diminishes because of low potency of surface coverage to form steric hindrance. For instance, the suitable concentration of MC for optimal size reduction and stabilization of itraconazole nanoparticles was 40% [ 89 ]. Moreover dilution of nanosuspension, by decreasing the efficacy of steric stabilizer for surface coverage, induces aggregation [ 89 , 175 ]. In this regards, itraconazole particles grow in size due to dilution of formulation and reduction of HPMC concentration [ 175 ]. Accordingly, insufficient amount of stabilizer is reported to be the cause of agglomeration in zaltoprofen nanosuspension, whereas excess amounts of it induce Ostwald ripening [ 86 ].
Thus, too little or too much surfactant/stabilizer can yield unfavorable particles. In this regard, absence of surfactant in amylose nanosuspension resulted in large particles, whereas small particles of interest were obtained with surfactant at the concentration of 0.5% ( w / v ) [ 105 ]. Conversely, high concentrations of surfactant resulted in large agglomerate [ 105 ]. In the same manner, the optimal concentration of low molecular weight chitosan for the stabilization of atorvastatin was 0.3% ( w /w), and increasing its concentration to 0.4 and 0.5% (w/w) led to particle agglomeration [ 44 ]. The paradoxical agglomeration because of high concentrations of stabilizer is explained by Ostwald ripening as well as increase of osmotic pressure. While the former enhances the solubility and causes erosion of small and growth of large particles, the latter induce attraction between colloidal particles [ 176 ].
- Combination of surfactants
Combination of different surfactants/polymers is implemented when the optimal condition is not conceivable with a single stabilizer. The absorption of surfactant depends on the nature of particle surface. For instance, lyophobic nanoparticles such as tadalafil [ 177 ] and amylose [ 105 ] exhibit hydrophobic surface. So, surfactants with lower HLB tend to bind better on these surfaces. However, hydrophilic surfactants (high HLB) have shown higher steric stabilization. Thus, surfactants with low HLB may not be suitable choices in all cases. In fact, a combination of hydrophilic and hydrophobic surfactants seems to offer a better choice. In this regard, combination of tween®80 (HLB 15) with span80 (HLB 4.3) produced optimal amylose or tadalafil nanoparticles (Table (Table3) 3 ) [ 105 , 177 ]. However, these formulations required different ratios of tween®80 to span80.
Effect of surfactant HLB on mean size and PDI of amylase nanoparticles. Reprinted with permission from [ 105 ]. Copyright (2016) Indian Academy of Sciences
Combination of polymers is shown to reduce particle size more efficiently than either of them alone, demonstrating synergistic effects [ 163 ]. In addition, simultaneous application of steric and electrostatic stabilizers, known as electrosteric stabilization, provides better protection against agglomeration [ 178 ]. For instance, charged polymers (cationic or anionic) such as chitosan confer both electrostatic repulsion and steric stabilization, thus prevent the adhesion of nanoparticles more efficiently [ 44 ]. Chitosan, also, enhances bioavailability due to mucoadhesion [ 179 ]. Accordingly, combination of high-Tg polymers (HPMCP 50 or Eudragit® L100) with surfactants enhances the dissolution rate, stability, and bioavailability of nanoparticulate drugs [ 163 ].
The synergistic effect of mixed surfactants on nanosuspension stability is explained by better coverage of the particle surface. In this regard, low amounts of Span80 penetrates into the gaps between Tween®80 molecules (Fig. 6 ), resulting in better coating of the nanoparticle surface. In fact, Span80 as inhibits the interactions between polar groups of Tween®80 produces a monolayer around the nanoparticle. This allows maximum coating with minimum concentration of the surfactant, and prevents the perturbation of other parameters of stability due to high concentrations of surfactant [ 177 ]. Alternatively, Tween®80 can be absorbed as the second layer over the internal layer of Span80 coating the particle. This indicates a surfactant bilayer, but requires high concentrations of the surfactants [ 177 ].

Interaction models of surfactants ( white triangles and circles ) Span80 and ( black triangles and circles ) Tween®80. Reprinted with permission from [ 177 ]. Copyright (2014) Springer Nature
Although application of two stabilizers appears to provide better protection, it adds another level of complexity for the optimization of formulation. Particularly, the ratio of polymers ought to be optimized to get the desired level of stability. In this regard, combination of PVP and SLS confer better size reduction. Meanwhile, increase of PVP ratios hampers the stability of formulation [ 180 ]. In fact, PVP seems to inhibit the surface adsorption of SLS at high concentrations. This is explained by the fact that free PVP molecules can interact with SLS molecules due to opposite charges [ 167 , 180 ].
According to the DLVO theory, the effect of mixing sequence has no influence which may be as a result of formation of right energy barrier between the particles and hence have good physical properties [ 117 ].
Drying for particle stability
Dry powder formulations to be reconstituted as nanosuspension are becoming trendy to enhance the stability of system during long term storage. Also, nanosuspensions in dry state are harnessed for the preparation of solid dosage form such as tablets, capsules, and pellets. In this regard, spray drying, freeze drying, spray freeze drying, pelletization and granulation have been successfully employed to transform the liquid nanosuspensions into dry powder [ 106 , 181 ]. In fact, drying appears to suppress the electrostatic activity due to lower effects of electrolytes in dry state [ 128 ].
Various additives such as polymers, surfactants, cryoprotectants and lyoprotectants are used as stabilizer or cryo/thermal-protectant in formulation or drying process. These excipients can alter particle behavior because of surface interaction or change in medium properties [ 106 , 182 – 184 ]. In addition, the selection of carriers should not also perturb the maintenance of physicochemical properties of the nanoparticle after re-dispersion [ 185 ]. In this regard, sugar base cryoprotectants such as sucrose decrease the surface zeta potential of itraconazole loaded poly (ε-caprolactone) nanoparticles by masking the OH group on the surface [ 186 ]. Accordingly, mannitol appears to result in lower polydispersity, more size reduction, and better cryopreservation compared to sucrose [ 89 , 117 ].
Taken together, nanosuspensions are very useful for the formulation of poorly water soluble drug molecules, and can increase their bioavailability, thereby, therapeutic efficacy. The bottom-up approach involves precipitation of supersaturated solutions for naonoparticle formation. Several parameters in the particle, dispersion medium and formulation/storage condition are critical in the stability of nanosuspension. Nucleation and particle growth share similar mechanisms with instability, i.e., the same principles that cause nucleation and particle formation can cause inappropriate growth an agglomeration. Thus, application of excipients such as stabilizers requires proper optimization of type and concentration. This implies that each formulation requires its own optimization process, and if a drying process is employed, one ought to consider the effects additional variables to ensure the desired size and behavior of particles as well as the stability and therapeutic efficacy of formulation.
Acknowledgements
The authors gratefully acknowledge the financial support from Shahid Sadoughi University of Medical Sciences.
Abbreviations
Nomenclature, compliance with ethical standards.
The authors declare that they have no conflict of interest.
Publisher’s Note
Springer Nature remains neutral with regard to jurisdictional claims in published maps and institutional affiliations.
- Open access
- Published: 15 January 2022
A revised analysis of current and emerging Nano suspension technological approaches for cardiovascular medicine
- Gayathri Devi Pilli 1 ,
- Karthikeyan Elumalai ORCID: orcid.org/0000-0002-6259-5332 2 , 3 ,
- Vijey Aanandhi Muthukumar 2 &
- Palani Shanmuga Sundaram 2
Beni-Suef University Journal of Basic and Applied Sciences volume 11 , Article number: 10 ( 2022 ) Cite this article
3223 Accesses
3 Citations
Metrics details
This is an objective critique to give an in-depth description of Nano suspensions. This article is attempting to address the issue of whether or not Nano science is realistic with respect to price, with regards to item costs being added to the endeavor and Lipotropic drugs have proven to be rewarding and Lipo-immunotherapy has proven to be beneficial. In modern times, drug marketing and promotion have become crucial to efficient commercializing of successful molecules, pharmaceutical companies often work to increase the chances of promoting successful drugs, these included cardiovascular drugs because of their widespread usage.
Nano suspension is a Nano metric Colloidal Suspension system i.e., Nano suspensions, in the solid form reaches the bloodstream and Nanoparticle colloids readily available to the target cells. All research on Nanostructures is focused on the four primary dimensions, composition, homogeneity, heterogeneity, elasticity, and agglomeration. Researchers are devising ways to deliver medication and other substances to a damaged cell and diseased region, as well as diagnose the body to pinpoint disease and defects, by way of Nanotechnology.
Short conclusions
The vital analysis of Nano science experiment on Nano suspension is working to achieve the goal of reducing product cost by using Nanotechnology in product development, as it wants to examine the probability of development by utilizing Nanotechnology. The usage of the top-limited technology allows the development of cardiovascular drugs classified under the biopharmaceutical classification system (Class II and Class IV) to use two approaches namely top-down and bottom-up methods.
1 Background
The Oral dosage method acknowledges because it is a straightforward approach, feasible measurements, increase profitability, but precise dosing has a greater likelihood of enhancing certain characteristics. The impetus for innovative approaches is stronger as of late, with research pertaining with a greater focus on drug solubility and bioavailability of Nano suspensions, for example, since its methodologies are novel and various. So, Wettability of new APIs into the market may trigger some difficulties. Controversial approaches that circumventing dissolvability problems include There are many different procedures such as liposomes, micro emulsions, Nano suspension, solid dispersion and particle arrangement that can be used, as well as other different procedures such as co-dissolvability, lipophilic arrangement, and salt development, among others. These methodologies prove not to be valuable in either way with all medications [ 1 , 2 ]. Nano suspension is usually has a favorable -results and when compared to a lipid-based dosing [ 3 ]. Though the widely used method of Nano-suspension drug preparation is "Top- Down extension", the "Bottom-Up" technique could be used to shape a low-dissolvable or a crystalline sort of poorly mobilizable drugs. Amorphous preparation is the most often done utilizing sub-size extension but using the more recently-developed system, which is Nano-suspension an fascinating approach is "Bottom-Up technique, where the particles are reduced to the Nano level, which results in an amorphous suspension. One may reduce the molecular size in a notch by using this Bottom-up technology with low and basic levels of equipment. Thus, stabilization approaches for Nano suspensions should be thoughtfully selected, since they are key factors in their preparation. Sometimes the various polymers and surfactants are used to retain integrity and keep liquids together [ 4 ]. It differs from standard suspension in that the particles range in size between 200 and 600 nm. Due to weak solvency and low solubility, lipophilic substances impair bioavailability. It is usually described as how a medication gets to the blood stream. To design formulation and regulatory specifications that are particularly difficult and critical when dealing with poorly water-soluble substances, solubility and accessibility have had to be more closely examined. At the moment, poorly soluble medications account for approximately one-third of all USP (United States Pharmacopeia) perceived medications. Nanotechnology is defined as innovation on the nanometer scale, which is typically between 0.1 and 100 nm in size. As with things, "Nanotechnology" is called, means "dwarf and “Nano science” is the analysis of nanos and subatomic things Fig. 1 [ 5 ].
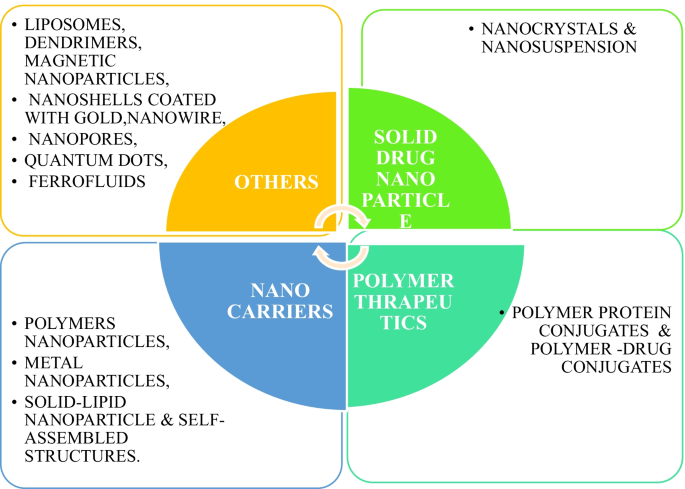
Different types of nanoparticulate-based drug delivery systems
2 Main texts
2.1 development of nano-particle.
A team of chemists in New York City discovered Nano Drugs in the late 1960s, beginning with their discovery as pharmaceutical vehicles first [ 6 ]. They imbedded magnetic molecules into Nano-particles by incorporating magnetic nanoparticles into the Nano-fields. Nanometer particles are developed from natural or man-made polymers and range from 0.5 to 500 Nanometers. Molecular materials consisting of ingredients are entrapped, some of which are held together by molecular bonds, some of which are entwined, and then carried into solution. Nanotechnologies are used in the areas of medication formulation, drug delivery, and drug encapsulation, both of which use Nanoparticles, Nanostructures, Nano suspension, Nano spheres, Nano-capsules, Nano- Emulsion and Quantum dots [ 7 ].
Nanoparticles are colloidal segments with diameters ranging from 1 to 100 nm. They are made up of micro molecular segments in which active segments are broken down, encapsulated, entrapped, adsorbed, or appended. Nano spheres are spheroid rigid, and comprise active ingredients suspended or dissolved inside polymer materials. Nano capsule is designed to contain the drug in the center enclosed by a polymer giving it extra stability. The added value of Nano-capsules and Nanospheres is that they work well with its smaller size and helps simple penetration through vessels and they are suitable for a distribution and targeting can be achieved [ 8 ]. The standard diameter of a Solid lipid nanoparticle ranges from 50 to 1000 nm. A sturdy lipid-based nanoparticle can serve as a vehicle for the transport of lipophilic particles. Through addition of surfactants, the lipid profile is adjusted; the emulsifier used depends on the route of administration. Parenteral administrations are particularly restricted [ 9 ]. Quantum dots are semi-conductors, which exhibit strongly quantum–mechanical features, but have optical and electronic characteristics that are seemingly distinct from those of other semiconductors. Nanoparticles, ranging from 2 to 8 nm in diameter Fig. 2 , 3 and 4 [ 8 , 9 , 10 ].
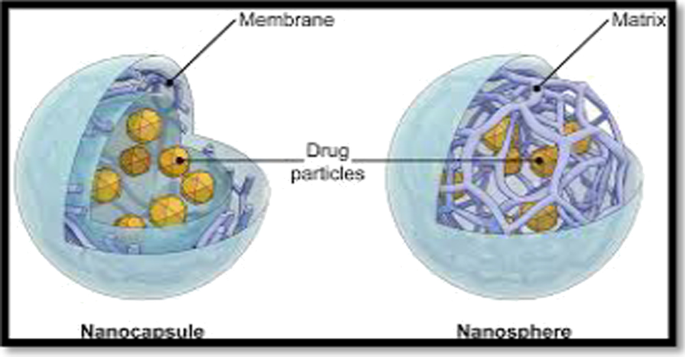
Schematic representation of nanospheres and nanocapsules [ 11 ]
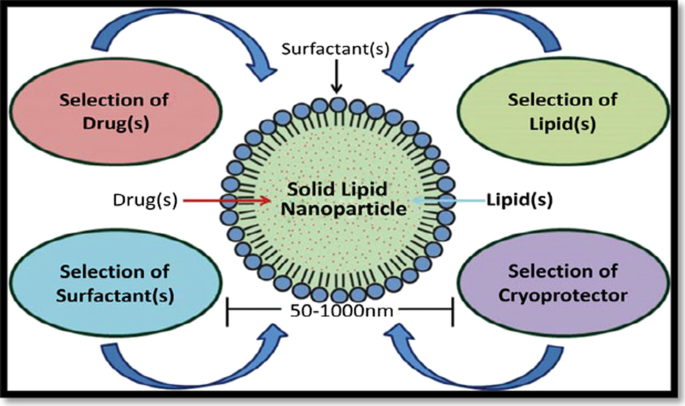
Schematic representation of solid lipid nanoparticle [ 12 , 13 ]
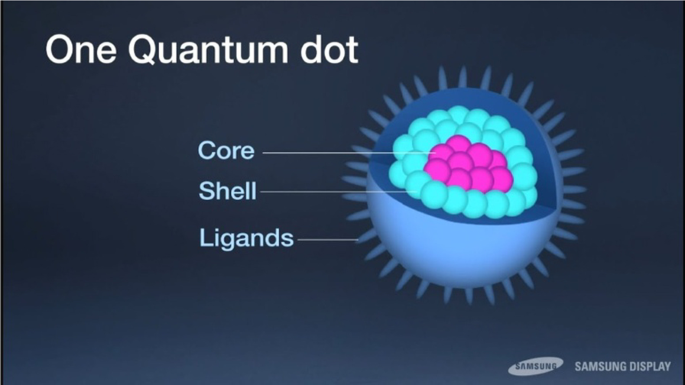
Schematic representation of quantum dots [ 14 , 15 ]
Ceramic Nanoparticles are intertwined biomolecules with a size of less than 50 nm and are a kind of Nanoparticle made of ceramics, which are inorganic, heat-resistant, non-metallic solids that can be made of both metallic and non-metallic mixtures. Ceramic nanoparticles, including in the field of energy supply and transportation, connectivity, infections, Glaucoma, chemotherapy and most often in medical science, have been used to repress the bone and other conditions Fig. 5 [ 12 ].
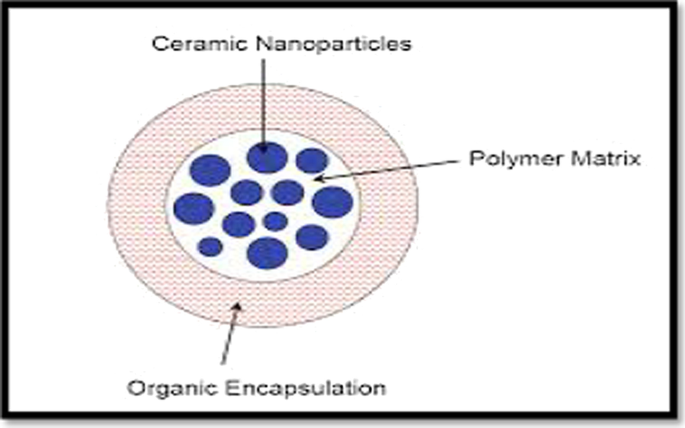
Schematic representation of ceramic nanoparticles [ 16 ]
Liposomes consist of one or more concentrated spheroids of lipophilic bilayers, isolated by natural and human made phospholipids by water or fluid buffer compartment, which are targeted to drug delivery systems. Liposome medicines mitigate systemic toxicity and avoid deterioration at an early stage. Small Uni-lamellar vesicles size 25–100 nm, Large Uni-lamellar vesicles size: larger than 50 Nanometer, Multilamellar vesicles larger than 0.5 nm Fig. 6 [ 13 ].
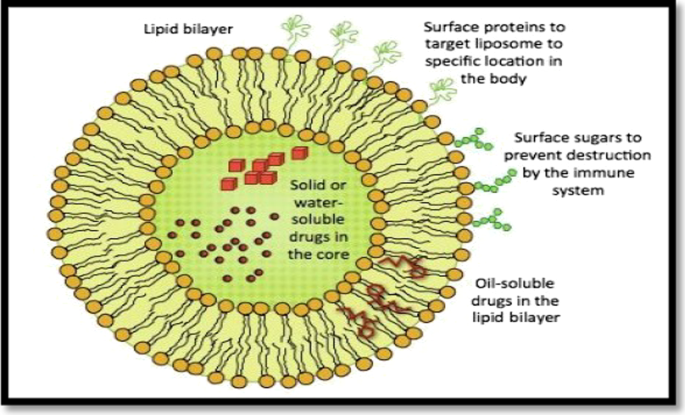
Schematic representation of liposomes [ 17 ]
Polypeptides are a kind of Nano forms, which can be made out of both protein and polymers in polymer blends. Many proteins are naturally formed by the human body, and they have strong metabolizability, so the protein constituents need to have the advantages of bio-activity and bio-decomposition. While proteins are used as therapeutic medicines, the principal limitations are lack of stability and inadequate circulation inside the body. As of late, they have been researched on for improved pharmacological characteristics, including visualization, bio conjugation, gene delivery, and pharmacological separation. Therapeutic agents can be mixed with polymeric vehicles such as polyethylene glycol to yield a wide distribution, better dispersible drugs, lower immunogenicity, regulated release and enriched protection will offer many benefits for therapy [ 14 , 15 ]. Tree-like particles with the characterized cavities are dendrimers, of 1.5 to 10 Nanometers. The dendrimers interact with chemical entities on their surfaces, bind to target molecules, form radio-bioactive, radio-ligands, imaging agents, and acquires aqueous solubility and low toxicity Figs. 7 and 8 [ 16 ].
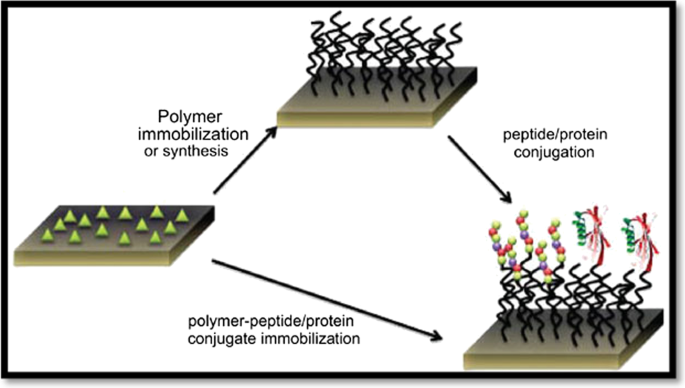
Schematic representation of polymer protein conjugates [ 18 ]
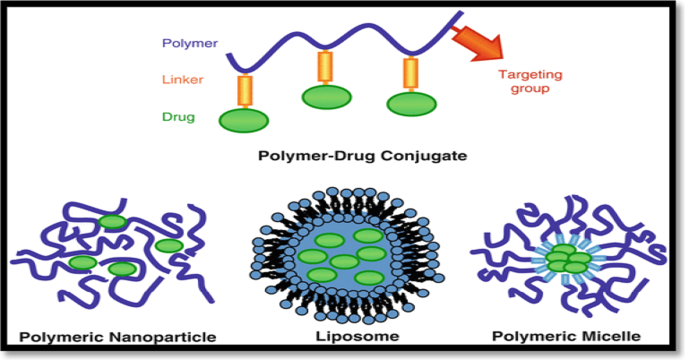
Schematic representation of polymer drug conjugates [ 19 , 20 ]
Magnetic Nanoparticles typically have a magnet material, mostly iron, nickel and cobalt, and a valuable synthetic segments. The Magnetic Nano beads, ranging between 50–200 Nanometers [ 17 , 18 ]. Nan shells claimed to be infrarouge optical activity in relation to gold colloid houses that are made up of a dielectric middle gold sulphide, or silica protected by a stainless steel (iron) sheet, are used in the treatment of carcinomas. Nano-tubes are linear nanostructures made of the 5–10 Nanometer scale of metals, semiconductor or carbon, also single or more layered or adjusted surfaces. A Molecule that has a pore size of 0.3 Nanometers (nm) is a Nano pore, used as a particle singling detector. Ferro fluids are Nano-colloidal fluids consist of small fragments of ferromagnetic or ferrimagnetic particulates trapped in a carrier liquid and coated with surfactants to prevent clumping of each atom. Magnetite, hematite or some other compound comprising iron, or a fluid is composed of Ferro-fluids composed of tiny Nano-size parts (usually 10 nm or fewer). As ferried are used to create a magnetic reaction, the limited amount needed maintains even distribution of Ferro fluid in the carrier fluid. The Ferro fluid is made up by volume of about 5%, 10% and 85%. The fluid contains desirable solids Fig. 9 [ 19 , 20 , 21 , 22 , 23 , 24 , 25 , 26 , 27 , 28 , 29 , 30 ].
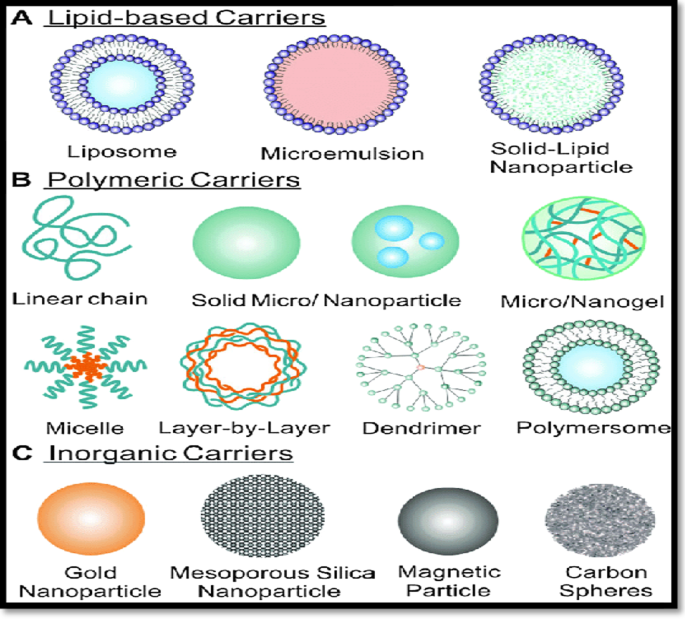
Schematic representation of nanocarriers [ 24 , 25 , 26 , 27 , 28 , 29 , 30 ]
Through using Nanostructure and Nano-spaces in specific areas of study, Nanotechnology connects organic and physical sciences; in particular, in Nano-medicine as well as the drug distribution mechanism. Nanomaterials are a molecules between 1 and 100 Nanometers, which affects the periphery of Nano devices from biosensors, microfluidics, drug delivery and microarrays to tissue designs, etc. In nanoscale, Nanotechnology uses technologies to develop Nanomedicine. Targeted drug transport and Controlled drug delivery enhance the studies of kinetics, dynamics, imprecise toxicity, immunogenicity and biologically recognized frameworks that have advanced in their effectiveness [ 23 ].
2.1.1 Important points for the selection of Nanosuspension medications [ 31 ] may be studied
Molecules that are poorly dissolved and impenetrable.
Large atomic weight and dosage.
Problems with temperature.
2.1.2 Advantages of nanosuspension
Nanosuspension seeks to minimize aqueous dissolution, moderate penetration, and limited efficacy in regards to medications used at BCS levels between II and IV Table 1 [ 24 ].
Nanosuspension should be used with medicines suffering complications in the formulation, as opposed to the lipophilic method.
Reduction of particles to nanometer range enhances the solubility and bioavailability of drugs.
The administration frequency of Nanosuspensions has decreased.
Increased physical and chemical stability of Nanosuspension drugs.
The effects are less caused by pharmaceutical excipients in Nanosuspension.
Nanosuspension are suitable ophthalmic and nasal providers.
Administering parenteral goods intramuscularly or subcutaneously by Nanosuspension their bioavailability enhances with minimal inflammation.
The required crystalline particle condition can be obtained in Nanosuspension preparation.
Nanosuspension facilitates and is cost-effective for large-scale operation [ 25 , 26 , 27 , 28 ].
2.2 Drug overview
While a number of clinical groups are approved medications using lipid detailing innovations, none are used for the better treatment of disease. Cardiovascular medicines are a most normal medicinal classification and constitute an enticing lipid formulation area of more than 100 FDA products. Because of their small size and low toxicity, nanosuspensions improve drug dispersibility and solubilization.
Nanosuspensions have been shown to improve drug solubility, stability, and bioavailability by means of the research presented in this reviews, So cardiovascular medicines have been recommended in the Nanosuspensions. Table 2 [ 29 ].
Prevalence and incidence rates in cardiovascular [ 30 ]. The angina pectoris has a reduced flow of blood to the heart and therefore produces chest pain, much like a blockage in the heart. The Arrhythmia, a sporadic heart beat or a cardiac band, or a pulse that is too low 60 beats per minute, bradycardia or tachycardia, a rhythm which is too high 100 beats per minute, is known as excessive heart beating. Congenital coronary heart disease is a problem with the heart's ability and shape that occurs at birth and affects the heart muscle. The Cardiac attack occurs due to blockages in the supply of blood through the heart muscle as blood clots build in the arteries. A Cardiac failure arose owing to a progressive disease of pumping inability of the heart. Cardiomyopathy is a disorder in which a heart contraction finds it more difficult to transfer blood to the remainder of the body. The following are the different forms of cardiomyopathy. Hypertrophic cardiomyopathy occurs as a consequence of a blockage at one part of the heart for no apparent reason. Dilated cardiomyopathy is triggered by an artery blockage. Restrictive cardiomyopathy is caused by ventricle rigidity. The effect of elevated tension is broken heart syndrome [ 23 , 24 , 25 , 26 , 27 ]. Mitral reverse flux results from the reverse flux of the blood from the ventricle to the atrium and may also occur from the atrium to the lungs, leading to respiratory problems and straining the hearts muscles leads to arrhythmia. Atrial fibrillation is a form of erratic pulse with a fast heart rhythm that may lead to more serious heart complications. Rheumatic disease results from rheumatic fever that damages connective tissue, especially damaging heart valves. An Aneurysm is a condition in which a mass forms in an artery. The disease of arteriosclerosis involves cholesterol or other compounds plunging through and into the artery. In Raynaud's disease, arteries temporarily block blood supply, such as in cold weather, causing numbness in certain areas of the body. An ischemic stroke occurs as blood clots in a blood vessel in the brain. Buerger's disease is a rare infection that can trigger gangrene in the arteries and veins of the arms and legs [ 28 , 29 , 30 ].
2.3 Tremendency for nanosuspension
2.3.1 bottom-up.
Bottom-up Approach and Top-down Process are two methods for organizing Nanosuspension. In terms of planning, the bottom-up scale-up process employs the Precipitation methodology. The particles are acquired in an amorphous form in this method, which is from the atom stage to the solid object, by using techniques such as anti-solvent or temperature changes, or both depending on the necessity. This method produces particles dissolved in miscible anti-solvent and crystals; the only drawback is that the substance must be dissolved in more than one solvent [ 32 , 33 , 34 , 35 , 36 ].
2.3.2 Top-down
There are two forms of Top-Down strategies: Media Milling and High-Pressure Homogenization. Dry milling and Media milling: The Nanosuspension is prepared in this method by using a high-pressure mill to shorten particle weight. This mills are made up of tiny milling balls/spherical rotators, and the fluid drug is moved into this milling region at high pressure and controlled temperature, and the particle size is decreased due to centrifugal forces inside the mill. Glass, zirconium oxide, or massively cross-linked polystyrene resin was used to make the spherical rotators. For medications that are difficult to dissolve, Nanosuspension employs a dry grinding process. Polyvinylpyrrolidone (PVP), Polyethylene glycol (PEG), Hydroxypropyl methylcellulose (HPMC), and Cyclodextrin subsidiaries are among the dissolvable polymers and co-polymers used. Dry granulating is often cost-effective and can be performed without the use of organic solvents. It is the most widely used for the preparation of Nanosuspension of certain ineffectively water-insoluble drugs [ 37 , 38 , 39 ].
2.3.3 High-pressure homogenization
The particle size is limited in the high-pressure homogenization method by going through a small size pore at high pressure. The technique is based on the cavitation forces of drug particles in the fluid level. These forces are powerful enough to convert the drug from micro atoms to Nano atoms [ 40 , 41 , 42 ].
It is classified into two types such as,
Disso Cubes (Homogenization in water media)
Nano-Edge & Nano-Jet (Homogenization in lipophilic media)
2.3.4 Disso-cubes
In the piston-gap, homogenizing reduction in size relies on cavitation rules. Disso-Cubing was engineered to be homogenizing for piston-gap environments. Particles are being diminished by force and even by particle bombardment [ 43 , 44 , 45 , 46 ]. A thin void of 25 μm is abruptly filled by scattering in a chamber of 3 cm in diameter. The determining variable of the device is cavitation in the Disso-Cubes. The stream through a chamber is very steady under the law of Bernoulli. The drop in diameter from 3 cm to 25 μm raises dynamic pressure and reduces the static pressure below the water-water breakdown limit. Drug Nanocrystals are mostly based on variables such as temperature, no homogenization cycles and strain of homogenization. However, the drop in static pressure is not necessary for cavitation instead of water, oils or an oily boiling point to be homogenized to decrease the particle size and milling operation. The acquired results have been close to Disso-Cubes and thus should be used in milder conditions for thermo labile compounds [ 47 , 48 , 49 ].
2.3.5 Nanoedge
In Nanoedge technology, medication particles are dissolved in solvents that are dissolved and mixed with a precipitated anti-solvent and homogenized to limit the growth of crystals. The downside of this approach is that it can be corrected by the homogenization of long-term equilibrium. The mixture of precipitation, homogenization and evaporation is thus the Nanoedge and these approaches combine to produce smaller particle sizes and improved stability [ 50 ].
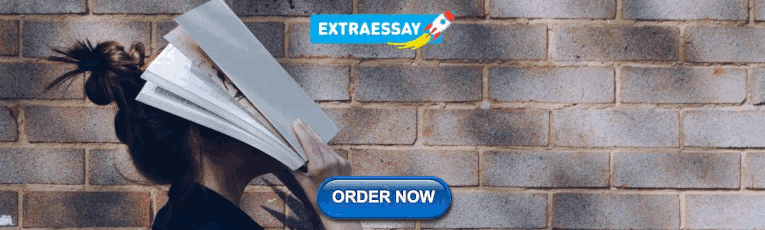
2.3.6 Nano jet
The technology uses a closed unit, which splits the Nanosuspension flow into segments and bombards each other at high pressure, which reduces the size of the particle due to this process. During this procedure, the strong shear pressure causes a reduction in particle size. M110L and M110S micro fluidizers are the instruments used in this Nano-jet. The main obstacle to this approach is the fluidizer that the element comprises smaller particles in a bigger division [ 51 ].
2.3.7 Solvent-evaporation technique
A drug is combined with the polymer solvent and the surfactant containing mixture is dissolved by the water. To precipitate the particles, an evaporation process is required. By going via high-pressure stirrers, crystal growth and production may be slowed [ 52 ].
2.3.8 Precipitation technique
The most well-known precipitation method is the anti-solvent procedure under which the compound is disintegrated into a solvent, which is organically dissolved and mixed with a miscible anti-solvent. The shapes of blending vary significantly [ 53 , 54 , 55 ].
2.3.9 Supercritical-fluid process
A supercritical fluid (SF) is commonly described as a liquid with a critical temperature (Tc) and considerable pressure (Tp). Nanosuspension is prepared using two processes: a rapid expansion of supercritical solution phase (RESS) and a supercritical anti-solvent process, followed by precipitation of the compressed anti-solvent process (PCA). As the solvent dries, the ions supersaturates and precipitate as fine crystals. The supercritical anti-solvent treatment employs a supercritical liquid in which a substance is ineffectively soluble, as well as a drug solvent that is often miscible with the supercritical liquid [ 56 ]. The disadvantages of the aforementioned techniques include the use of hazardous solvents, elevated levels of surfactants as opposed to other processes, and particle crystallization due to high super-saturation, which can result in undesirable polymorph [ 57 , 58 , 59 ].
2.4 Patents filed: nanotechnology patents Of 2018 at the USPO & EPO statistics
According to 2019 studies, the share of nanotechnology patents in total patents filed at two patent offices was relatively 2.5 percent to 3 percent, despite a slight increase in recent years. These statistics suggest that a significant proportion of scientific achievements in the fields of nanotechnology still remains at the level of scientific article publication [ 60 ].
2.5 Applications
2.5.1 intravenous administration.
The intravenous management route offers fast, quick, increased bioavailability and prevents metabolism at first passes. It is the preferred path for medicines not consumed or decayed in the GIT. Nanosuspension increases clinical efficiency and reduces cost-effectiveness by improving dosage proficiency and reduced infusion sizes.
2.5.2 Bio-accumulation improvement
The drug's low oral use rate is attributed to weak dissolvability, penetration, or durability inside the GIT. Nanosuspension addresses the issue of poor bioactivity by addressing the issues of weak dissolvability and weak membrane penetration. Higher bioavailability and long-term physical stability of Nanosuspension can improve therapeutic results. The drug's dissolvability improves as the surface area of the drug particles increases from micrometer to nanometer scale. The Nanosuspension increases disintegration of lyophilized Nanosuspension material in (90 percent in 20 min) association with rough powder disintegration in (15 percent in 20 min).
2.5.3 Pulmonary administration
Nanosuspension are electronic or ultrasonic nebulizers used for pulmonary medication distribution. Because of their Nano scale and the adhesives used in Nanosuspension, they have higher selectivity, less drug loss, and a longer residence period at the target point. Pulmonary Nanosuspension increase medication diffusion and disintegration, which improves bioaccumulation and predicts unintended drug deposition in the mouth and pharynx.
2.5.4 Ocular administration
Ocular drug delivery as a Nanosuspension has been concentrated to provide a gentle and long-lasting release of the prescription. For viable treatments, the incorporation of bio-degradable polymer induces delayed residual period in the eye sac. Drug Nanoparticle adhesives have been shown to reduce drug degradation.
2.5.5 Drug targeting
Nanosuspension may also be used for a targeted drug delivery mechanism by concentrating on the particle surface region, and adjusting the stabilizer can effectively change the in vivo behavior. The medications are soaked up by the phagocytic cell, allowing for drug targeting.
2.5.6 Mucoadhesive nanosuspension
As the suspension diffuses through the fluid media and rapidly reaches the mucosal surface, the Nanosuspension is controlled. Nanosuspension bind to the intestinal surface through "bio-adhesion," and the repository procedure begins immediately. The Nanosuspension adhesiveness aids in enhancing bioavailability while still adhering to the Noyes-Whitney state.
3 Conclusions
One of the main challenges is achieving a goal in finding an appropriate nanosuspension preparation approach with low energy input and erosion contamination, such as the bottom-up method and top-down method. This review dwells upon both top-down and bottom-up technologies for nanosuspension preparation. The characters and advantages of drug nanosuspension, corresponding to the characterization techniques, solidification methods, and drug delivery dosage forms, were discussed in detail. We conclude that the drugs which are belonging to the BCS CLASS II and IV were most eligible to prepare in the form of Nanosuspension so that solubility problems can be overcome and ultimately bioavailability also increase suspensions were also made. Higher bioavailability and long-term physical stability of Nanosuspension can improve therapeutic results. Nanosuspension increases clinical efficiency and reduces cost-effectiveness by improving dosage proficiency and reduced infusion sizes.
Availability of data and materials
Not applicable.
Abbreviations
Polyvinylpyrrolidone
Polyethylene glycol
Hydroxypropyl methylcellulose
Bio pharmaceutics classification system
Roya Y, Krasimir V, Spomenka S (2015) Nanosuspension technologies for delivery of poorly soluble drugs. J Nanomater 2015:1–13
Google Scholar
Jayanta KP, Gitishree D, Leonardo FF, Estefania VRC, Maria PRT et al (2018) Nano based drug delivery system: recent developments and future prospects. J Nanobiotechnol 71:1–33
Smita SA, Sagar TM, Saudagar RB (2017) Review article Nanosuspension an overview. Int J Curr Pharm Res 9:19–23
Sachin SP, Bajirao RD, Sandip PN, Rajan SS (2017) Nanosuspension technologies for delivery of drugs. Nano Nanotechnol Res 4(2):59–66
Chaudhari SP, Kamble SC, Mahajan RA, Jagdale S, Ratnaparkhi MP (2010) Nanosuspension: a novel drug delivery system. J Pharm Res 3(2):241–246
Mitesh P, Arpit S, Patel NM, Patel MR, Patel KR (2011) Nanosuspension: a recent approach for nano drug delivery system. In J Curr Pharm Rev Res 3(4):96–101
Diana S, Debora F, Joana LR, Lígia RR (2017) Nanotechnology: a revolution in targeted drug delivery. Int J Bas Clin Pharm 6(12):2766–2773
Girish BS, Rakesh PP, Prajapati BG, Nikunjana AP (2011) Solid lipid nanoparticles and nano lipid carriers: as novel solid lipid based drug carrier. Int Res Pharm 2(2):40–52
Mukerjee S, Ray S, Thakur RS (2009) Solid lipid nanoparticles: a modern formulation approach in drug delivery system. Int J Pharm Sci 71(4):349–358
Menaka J (2018) Current trends in industrial scale synthesis of quantum dots and its application in electronics. In Handbook of nanomaterials for industrial applications, pp 381–385
Suffredini G, East JE, Levy LM (2013) Studied a new applications of nanotechnology for neuroimaging citied in March 2013. Am J Neuroradiol 35:7. https://doi.org/10.3174/ajnr.A3543
Article Google Scholar
Aura IMV, Teresa GQ, Rosa ENA, Laura-SAT VC (2012) Polymeric and ceramic nanoparticles in biomedical applications. J Nano Technol 2012:1–10
Asif ISF, Jasjeet KS, Sanjula B, Shweta D, Javed A (2012) Nanostructured lipid carriers system: recent advances in drug delivery. J Drug Target 20:813–830
Iriny E, Colson Y, Grinstaff M (2019) Polymer-drug conjugate therapeutics: advances, insights and prospects uses. Nat Rev Drug Dis Rev 18:273–294
Monika LG, Priyanka GP, Pradum PI (2020) Polymer-drug conjugates as nanomedicine: a review. Int J Poly Mater Poly Biol 69:990–1014
Ardhendu KM (2021) Dendrimers in targeted drug delivery applications: a review of diseases and cancer. Int J Poly Matrt Poly Biol 70(4):287–297
Kai W, Diqing S, Jinming L, Renata S, Wang JP (2019) Magnetic nanoparticles in nanomedicine: a review of recent advances. Nanotechnology 30(50):502003
Ting G, Junxing H, Chenglin Z, Weizhong T, Hong Y, Xingmao J, Jun Y, Yujuan S, Yanhong X, Xuefeng B, Xiaoqian F (2018) The recent advances of magnetic nanoparticles in medicine. J Nanotechnol 2018:1–8
Gul R, Zainab N, Asad M, Salma B, Anwar HAS, Shabeer AM, Ghulam A (2019) An overview of the recent progress in the synthesis and applications of carbon nanotubes. J of Car Res 5(1):1–31
Yong W, Dali Z, Qiulin T, Michal XW, Li-Qun G (2011) Nanopore-based detection of circulating microRNAs in lung cancer patients. Int Nat Nanotechnol 6:668–674
Azadeh S, Hashemi ASMGJMM (2020) Laser-assisted cancer treatment, in bio-engineering approaches to cancer diagnosis and treatment. Sci Direct 6:131–156
Dubrovskiy AA, Balaev DA, Shaykhutdinov KA, Bayukov OA, Pletnev ON, Yakushkin SS, Bukhtiyarova GA, Martyanov ON (2015) Size effects in the magnetic properties of e-Fe 2 O 3 nanoparticles. J Appl Phys 118:213901–213917
Patel AP, Patel JK, Patel K, Deshmukh AB, Mishra BR (2011) A review on drug nanocrystals a carrier free drug delivery. Int J Res Ayurveda Pharm 2(2):448–458
CAS Google Scholar
Boubbou KE (2018) Magnetic iron oxide nanoparticles as drug carriers: clinical relevance. Nanomedicine 13:953–971
PubMed Google Scholar
Sarabjeet SS, Hicham F, Baljit S (2007) Nanotechnology-based drug delivery systems. J Occup Med Toxicol 2:16
Sneha D, Srinivas B, Rajashree H (2018) Preparation and characterization of oral nanosuspension loaded with curcumin. Int J Pharm Sci 10(6):90–95
Patravale VB, Abhijit AD, Kulkarni RM (2004) Nanosuspension: a promising drug delivery strategy. J Pharm Pharmacol 56(7):827–840
CAS PubMed Google Scholar
Sachin SP, Bajirao RD, Sandip PN, Rajan SS (2017) Nanosuspension technology in drug delivery system. Nanosci Nanotechnol Res 4(2):59–66
Md. Saddam Hussain, Abdul Baquee Ahmed And Jiban Debnath (2020) Nanosuspension: A Promising Drug Delivery System For Poorly Water Soluble Drug And Enhanced Bioavailability. IJSPR (2020), Volume 11, Issue 10.
Zhao H, Li R (2008) Effect of water absorption on the mechanical and dielectric properties of nano-alumina filled epoxy nanocomposites, composites Part A. Appl Sci Manuf 39:602–611
Lakshmi P, Kumar GA (2010) Studied a nanosuspension technology: a review citied in september 2010. Int J Pharm Pharmaceut Sci 2(4):3540
Venkatesha T, Rajesh C, Sekar M, Jeevanandham S, Reza KH, Nagi Reddy BKV (2011) Nanosuspension: ideal approach for the drug delivery of poorly water soluble drugs. Der Pharm Let 3(2):203–213
Stanekzai A, Vikrant SCK, Pankaj K (2019) Nanosuspension as a promising approach to enhance bioavailability of poorly soluble drugs: an update. J Drug Deliv Therap 9(2):574–582
Chowdary KPR, Madhavi BLR (2005) Novel drug delivery technologies for insoluble drugs. Ind Drug 42(9):557–563
Cornelia MK, Rainer H (2006) Drug nanocrystals of poorly soluble drugs produced high-pressure homogenizations. Eur J Pharm Biol 62(1):3–16
Krause KP, Kayser O, Mader K, Gust R, Muller RH (2000) Heavy metal intamination of nanosuspensions produced by highpressure homogenizations. Int J Pharm 196(2):169–172
Elaine MML, Gary GL (2008) Drug nanoparticles: formulating poorly water-soluble compounds. Toxicol Pathol 6(1):43–48
Desmond H, Keiko O, David JC, Chan HK, Raper JA, Ye L, Yun J (2010) Pure drug nanoparticles in tablets: what are the dissolution limitations. J Nan Res 12:1743–1754
Jain D, Khatri C, Rani A (2010) Fly ash supported calcium oxide as recyclable solid base catalyst for Knoevenagel condensation reaction. Fu Process Technol 91:1015–1021
Irene P, Ruggero B, Ferdinando G (2006) Solid-state chemistry and particle engineering with supercritical fluids in pharmaceutics. Eur J Pharm Sci 27:299–310
Arun KN, Deecaraman M, Rani C, Mohanraj KP (2009) Preparation and solid state characterization of atorvastatin nanosuspensions for enhanced solubility and dissolution. Int J Pharm Technol Res 1:1725–1730
Chauhan NN, Niraj VP, Suthar SJ, Jayvadan KP, Manish PP (2012) Micronization of BCS class–II drugs by various approaches for solubility enhancement—a review. Res J Pharm Technol 5(8):999–1005
Li W, Yang Y, Tian Y, Xu X, Chen Y (2011) Preparation and in vitro/in vivo evaluation of revaprazan hydrochloride nanosuspension. Int J Pharm 408:157–162
Jorvekar P, Pathak AA, Chaudhari PD (2012) Formulation development of aceclofenac loaded nanosuspension by three square (32) factorial design. Int J Pharm Sci Nanotechnol 4:1575–1582
Banavath H, Sivarama RK, Tahir A, Sajid A, Pattnaik G (2011) Nanosuspension: an approach to enhance solubility of drugs. Int J Pharm Sci Res 2(2):81–87
Shegokar R, Müller RH (2010) Nanocrystals: industrially feasible multifunctional formulation technology for poorly soluble actives. Int J Pharm 399:129–139
Srilatha K, Prabhavathi DB, Lingaiah N, Prasad R, Sai PP (2012) Bio-diesel production from used cooking oil by two-step heterogeneous catalyzed process. Bio Technol 119:306–311
Prasanna L, Kumar G (2010) Nanosuspension technology: a review. Int J Pharm Pharm Sci 2(4):35–40
Xiaohui P, Jin S, Mo L, Zhonggui H (2009) Formulation of nanosuspensions as a new approach for the delivery of poorly soluble drugs. Curr Nano 5:417–427
Mohanty S, Boga PK (2009) Role of nanoparticles in drug delivery system. Int J Res Pharm Biomed Sci 1(2):41–66
Ashaben P, Kishore C, Vibhuti A, Ashim KM (2013) Ocular drug delivery systems: an overview. World J Pharmacol 2(2):47–64
Ensign LM, Cone R (2012) Nanosuspensions: potent vehicles for drug delivery and bioavailability enhancement of lipophilic drugs. J Pharm Res 5(3):1548–1554
Gupta A, Goyal S (2012) Nanosuspension—a novel approaches. Drug Deliv Syst 12(1):50–64
Prabhakar C, Bala KK (2011) A review on nanosuspensions in drug delivery. Int J Pharm Biol Sci 2:549–558
Sainia A, Jata S, Shekhawat D, Kumar A, Dhayala V, Agarwal D (2017) Oxime-modified aluminium(III) alkoxides: potential precursors for gamma-alumina nano-powders and optically transparent alumina film. Mater Res Bull 93:373–380
Belekar R, Dhoble S (2018) Activated alumina granules with nanoscale porosity for water defluoridation. Nan Struct Nano Object 16:322–328
Satya SS, Chandu BR, Ashis KM (2015) Formulation development and optimization of nanosuspension of simvastatin for improved solubility by nanomilling. J Pharm Sci Technol 5:78–87
Xu B, Wang L, Liu Y, Zhu H, Wang Q (2018) Preparation of high strength and transparent nanocomposite hydrogels using alumina nanoparticles as cross-linking agents. Mater Lett 228:104–107
Kiran T, Chintha S, Jithan A (2015) Formulation, optimization and evaluation of oral nanosuspension tablets of nebivolol hydrochloride for enhancement of dissoluton rate. Der Pharm Lett 7(3):71–84
Sandhya J, Pavani A, Raja RR (2014) Formulation and evaluation of nanosuspension of nisoldipine. Int J Pharm Sci Rev Res 24(1):177–181
Download references
Acknowledgements
Author information, authors and affiliations.
Department of Pharmaceutics, School of Pharmaceutical Sciences, Vels Institute of Science, Technology and Advanced Studies, Pallavaram, Chennai, 600 117, India
Gayathri Devi Pilli
Department of Pharmaceutical Chemistry and Analysis, School of Pharmaceutical Sciences, Vels Institute of Science, Technology and Advanced Studies, Pallavaram, Chennai, 600 117, India
Karthikeyan Elumalai, Vijey Aanandhi Muthukumar & Palani Shanmuga Sundaram
Faculty of Pharmacy, Bharath Institute of Higher Education and Research, Selaiyur, Chennai, 600 073, India
Karthikeyan Elumalai
You can also search for this author in PubMed Google Scholar
Contributions
GDP: Collected Literature. KE: Designed the work. VAM: Prepared the Manuscript. PSS: Done the Language Edition. All authors have read and approved the manuscript.
Corresponding author
Correspondence to Karthikeyan Elumalai .
Ethics declarations
Ethics approval and consent to participate, consent for publication, competing interest.
The authors declare no competing interest in this study.
Additional information
Publisher's note.
Springer Nature remains neutral with regard to jurisdictional claims in published maps and institutional affiliations.
Rights and permissions
Open Access This article is licensed under a Creative Commons Attribution 4.0 International License, which permits use, sharing, adaptation, distribution and reproduction in any medium or format, as long as you give appropriate credit to the original author(s) and the source, provide a link to the Creative Commons licence, and indicate if changes were made. The images or other third party material in this article are included in the article's Creative Commons licence, unless indicated otherwise in a credit line to the material. If material is not included in the article's Creative Commons licence and your intended use is not permitted by statutory regulation or exceeds the permitted use, you will need to obtain permission directly from the copyright holder. To view a copy of this licence, visit http://creativecommons.org/licenses/by/4.0/ .
Reprints and permissions
About this article
Cite this article.
Pilli, G.D., Elumalai, K., Muthukumar, V.A. et al. A revised analysis of current and emerging Nano suspension technological approaches for cardiovascular medicine. Beni-Suef Univ J Basic Appl Sci 11 , 10 (2022). https://doi.org/10.1186/s43088-022-00193-4
Download citation
Received : 26 July 2021
Accepted : 04 January 2022
Published : 15 January 2022
DOI : https://doi.org/10.1186/s43088-022-00193-4
Share this article
Anyone you share the following link with will be able to read this content:
Sorry, a shareable link is not currently available for this article.
Provided by the Springer Nature SharedIt content-sharing initiative
- Nano suspension
- Micro emulsions
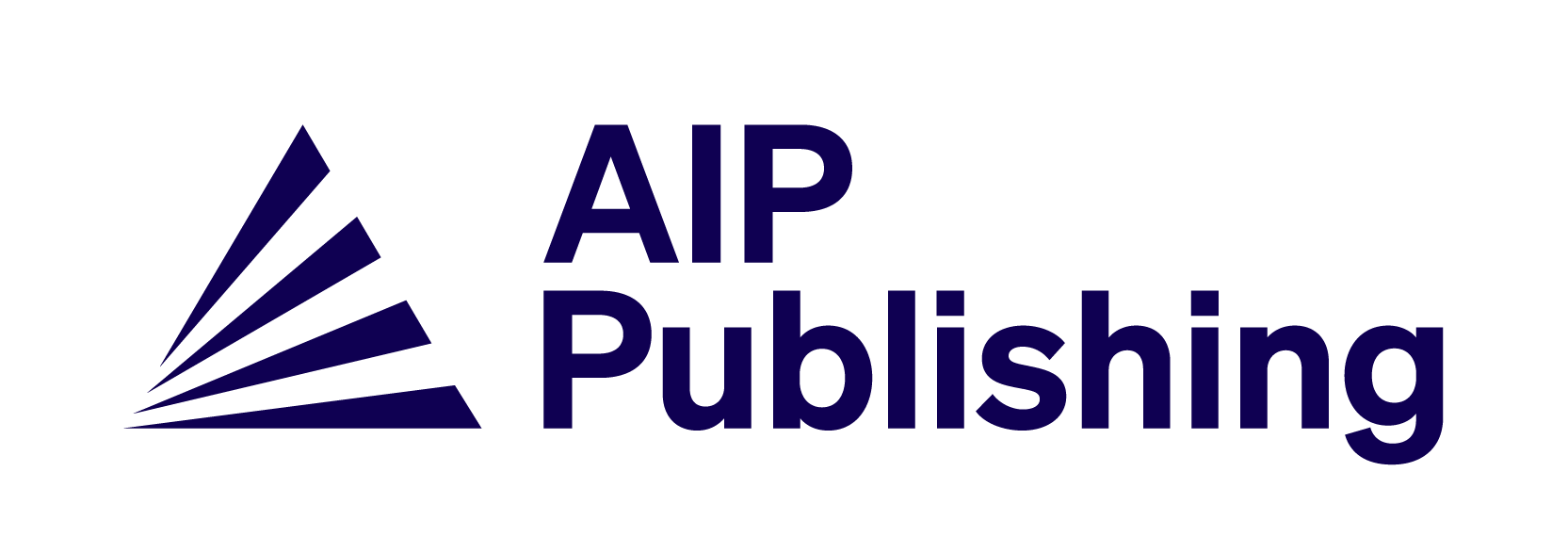
Nanosuspension as a promising drug delivery approach for the antidiabetic drug: An inclusive review on technology and future aspects
- Article contents
- Figures & tables
- Supplementary Data
- Peer Review
- Reprints and Permissions
- Cite Icon Cite
- Search Site
Pooja Sharma , Sujit Bose , Akhil Moudgil , Divya Arora , Sushila , Manish Vyas , Shivalika , Mamta Devi , Bhupendra Tomar; Nanosuspension as a promising drug delivery approach for the antidiabetic drug: An inclusive review on technology and future aspects. AIP Conf. Proc. 8 September 2023; 2800 (1): 020177. https://doi.org/10.1063/5.0163115
Download citation file:
- Ris (Zotero)
- Reference Manager
Nanosuspension is a part of nanotechnology which is a submicron colloidal dispersion of pharmaceutically active ingredients in a liquid phase having a size range below 1 um, and which is stabilized by surfactants and polymers. Most of the newly developed drugs are water-insoluble, show poor bioavailability. Glimepiride is an anti-diabetic drug that belongs to the sulfonylurea class, which is used to treat type II diabetes mellitus. Glimepiride increases insulin secretion by acting on the β-cells of the pancreas. Glimepiride binds to sulphonylurea receptors which are present on β-cell on the plasma membrane, which close the ATP-sensitive potassium channel leading to depolarization of the cell membrane. So there is the opening of voltage-gated calcium channel due to which there is an influx of calcium ions causes the secretion of the preformed insulin molecule. It is categorized under biopharmaceutical classification system class II drug, having poor solubility and high permeability. In this review, different methods were studied to formulate the nanosuspension of glimepiride to increase the solubility of glimepiride.
Sign in via your Institution
Citing articles via, publish with us - request a quote.
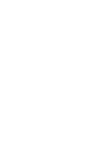
Sign up for alerts
- Online ISSN 1551-7616
- Print ISSN 0094-243X
- For Researchers
- For Librarians
- For Advertisers
- Our Publishing Partners
- Physics Today
- Conference Proceedings
- Special Topics
pubs.aip.org
- Privacy Policy
- Terms of Use
Connect with AIP Publishing
This feature is available to subscribers only.
Sign In or Create an Account
- Open access
- Published: 20 May 2020
A Design of Experiment (DoE) based approach for development and optimization of nanosuspensions of telmisartan, a BCS class II antihypertensive drug
- E. Bhargav ORCID: orcid.org/0000-0001-7799-4427 1 ,
- G. Chaithanya Barghav 2 ,
- Y. Padmanabha Reddy 1 ,
- Chintamaneni Pavan kumar 2 ,
- P. Ramalingam 1 &
- C. Haranath 3
Future Journal of Pharmaceutical Sciences volume 6 , Article number: 14 ( 2020 ) Cite this article
3621 Accesses
5 Citations
Metrics details
The present study was aimed to enhance the solubility and dissolution of BCS class II drug, telmisartan (TEM), by nanoformulation approach. Several attempts were made to develop a nanosuspension by bottom-up and top-down techniques. In our study, we found in situ nanoamorphization technique to be incompatible and hence was not selected for further development of nanoformulation. Bottom-up techniques such as anti-solvent precipitation and emulsification solvent evaporation methods failed to reduce the size of the drug to nanoform by HPMC E15 and PVP K-25 at 1500–2000 rpm but resulted in micron-sized particles.
However, the acid-base neutralization method has produced nanosuspension with a particle size of 243.9 nm and 0.119 PDI. Formulation and analytical development were carried out by statistical factorial design using the Design-Expert software (version 11.0). The nanosuspensions remained stable even after 90 days without any aggregations with particle size 338.1 nm and PDI 0.146. Zeta potential of optimized formulation was found to be − 16.2 mV. Drug content and its release were estimated by the developed and validated in-house HPLC method. In vitro drug diffusion studies on the optimized formulation have shown a drug release of 82.6% by the end of 3 h, whereas plain drug suspension has shown only 42.8% release, indicating a 2-fold increase of drug diffusion with nanosuspension. Ex vivo drug permeation studies performed using excised goat gastric mucosa revealed much faster permeation of TEM from nanosuspension than the plain drug suspension.
Conclusions
Hence, from the results, it can be concluded that TEM, when formulated by acid-base neutralization method as a nanosuspension, leads to enhanced solubility, dissolution, and stability.
Hypertension, a worldwide epidemic at present, is not a disease in itself rather it is an important risk factor for serious cardiovascular disorders including myocardial infarction, stroke, heart failure, and peripheral artery disease. An estimate of 26% of the world population is suffering from hypertension, and this toll was expected to rise to 29% by 2025 [ 1 ]. Telmisartan (TEM), a highly selective angiotensin II (AII) type 1 (AT1) receptor antagonist, is approved for the treatment of hypertension, either as monotherapy or in combination with other antihypertensive agents showing a greater reduction in blood pressure when compared with losartan. It is also effective in terms of reducing blood sugar levels and cholesterol. However, it belongs to BCS class II, which is sparingly soluble in water and has a high log P value [7.7], and thereby it exhibits poor bioavailability and stability [ 2 ]. Formulating of TEM as nanosuspensions is an attractive approach, where particles ranging from size 200–500 nm are sterically stabilized by surface stabilizers, resulting in improved saturation solubility, dissolution rate, oral bioavailability, stability, therapeutic efficiency, reduced side effects, and reduced toxicity [ 3 ].
Bottom-up and top-down are the methods generally used in the preparation of nanosuspensions. The top-down methods include ball milling and high-pressure homogenization. Combinative technologies are introduced to produce even smaller particles [ 4 ]. Media milling, a nanosuspension formulating technique, has a limitation of product contamination due to erosion. A high-pressure homogenization technique consumes high energy. Many hydrophobic drugs are weakly acidic or basic, which thus imparts pH-dependent solubility to them [ 5 ].
A literature survey revealed that TEM solubility was enhanced by the nanosuspension approach by the media milling technique; the solution was stirred at 700 rpm for 18 h. By evaporative anti-solvent precipitation technique, the nanocrystals were formulated by a mixture of various surfactants and polymers at 4000 rpm using a mechanical stirrer; further stirring continued for 2 h at 10,000 rpm [ 6 ]. The above-stated technique media milling is applicable for laboratory scale and possesses several scalable difficulties for large scale development. Experimentation and optimization were carried out by factorial design which included the selection of variables. HPMC E15 and stirring speed were selected as major factors; particle size and cumulative percentage drug release were opted as responses. Analytical method development for analysis of dissolution samples was performed by opting flow rate and pH as factors and retention time and USP plate count as responses. Hence, the present study was aimed at developing and optimizing TEM nanosuspension by 2 2 factorial design with midpoints to enhance its solubility, dissolution rate, and hence improved bioavailability.
TEM obtained as a gift sample from Connexions Life sciences, Bangalore. HPMC E15 and PVP K-25 were procured from SD Fine-Chem Limited. All the reagents used for analytical development in the study were of analytical grade and were obtained from Sigma Aldrich, Bangalore.
HPLC method development of TEM
Agilent HPLC (contained UV–VISIBLE detector Model) with the EZChrom Elite software was used for analyzing in vitro dissolution samples and for data collection and integration. A reverse-phase Ecosil-C18 column (250 × 4.6 mm, 5 μm) was used for the TEM chromatographic separation at 25 °C. The flow rate was selected as one of the factors in the experimental design, and it was set at 0.80 to 1.0 ml/min, and detection was done at a wavelength of 291 nm. Twenty microliter was the injection volume [ 7 ].
Validation as per ICH guidelines was performed for the developed method to assess various parameters like the specificity in which placebo sample solutions were prepared in the same manner as in sample preparation and were injected into the chromatographic system without TEM at various concentrations, and the chromatogram data was recorded.
Linearity was determined by preparing seven standard TEM solutions with concentrations ranging from 50 to 150% of the target standard concentration were prepared and injected into the chromatographic system, and the coefficient of determination was calculated. The precision of the developed method was confirmed by repeatability and intermediate precision. Intraday and interday analyses were performed by repeating the method for three times in the same day and on three successive days. The absorbance was determined and percentage RSD was calculated; six sample solutions (TEM) were prepared at lower (50%) and higher (150%) levels and three intermediate sample solutions (100%) to assess accuracy.
Robustness was determined by using two mobile phases, one containing 90% acetonitrile and the other containing 110% acetonitrile, and the system suitability parameters were assessed by standard solution injection; flow rate robustness was assessed by increasing the flow rate from 0.8 to 1.0 ml/min. The effect of pH on robustness was assessed by injecting the solutions consisting of the mobile phase of varying pH from 4.4 to 4.7 into the chromatographic system.
Standard and blank solution preparation
The standard solution was prepared by dissolving the drug TEM 40 mg into a 25-ml volumetric flask containing phosphate buffer of 15 ml and acetonitrile of 10 ml (60:40 v/v), sonicated for 5 min, and then filtered through 0.45-μm membrane filter. The concentration of the solution was found to be 1.6 mg/ml. The blank solution was prepared as similar to standard but without the pure drug in the ratio of 60:40% v/v mixture of phosphate buffer and acetonitrile, the same solution used as mobile phase [ 8 ].
Sample preparation
The collected dissolution sample was transferred to a 25-ml volumetric flask. The sample was then dissolved and diluted with pH 4.4 phosphate buffer: acetonitrile (60:40 v/v ) and subjected to sonication for 10 min, filtered through a 0.45-μm membrane filter [ 8 ].
Solubility studies
The solubility studies were performed by placing the pure drug in excess quantity and optimized nanosuspension in two different flasks which consist of 10 ml of distilled water. The samples present in the sealed flasks were placed on the orbital shaker for agitation (Cintex Industrial Corporation, Mumbai) at room temperature for 48 h. Then, the centrifugation of samples was done to separate the undissolved content. The analysis was carried out by the above developed HPLC technique for the quantification of TEM present in the supernatant layer [ 7 ].
Drug-excipient compatibility studies
The compatibility between the drug and the excipients (HPMC E15, sodium hydroxide, sodium bicarbonate) was studied via FT-IR, using Bruker alpha E ATR spectrophotometer (KBr pelletization method) [ 7 ].
Formulation development of TEM nanosuspensions
- Bottom-up anti-solvent precipitation method
Accurately weighed 100 mg of pure TEM was dissolved in 2 ml of solvent (chloroform) at room temperature. The above solution was poured into a beaker having a fixed volume (100 ml) of anti-solvent (water) containing 100 mg of polymer (HPMC E15) at room temperature with a stirring speed of 1500 rpm using mechanical Remi stirrer. The above solution was stirred continuously for 2 h to evaporate the solvent. The formed nanosuspension was transferred into a container and stored at 25 °C until further use. The same procedure was adopted using PVP K-25 as a stabilizer, instead of HPMC E15 [ 9 ].
- Emulsification solvent evaporation method
To 5 ml of chloroform, 100 mg of TEM was added and dissolved at room temperature. The resulting solution was added into a 50 ml aqueous solution of 100 mg HPMC E15 at the same temperature under high-speed homogenization (8000 rpm) for 5 min. After homogenization, the preparation was subjected to ultrasonication for 15 min, followed by mechanical stirring (10 min) for the evaporation of the organic solvent. The nanosuspension was obtained after centrifugation for 10 min at 4000 rpm. The same procedure was adopted using PVP K-25 as a stabilizer instead of HPMC E15 [ 10 ].
- Acid-base neutralization method
TEM (100 mg) was weighed and dissolved in 5 ml mixture of hydrochloric acid solution (3 N) and chloroform (1:5 v/v). HPMC E15 was dispersed in 100 ml of sodium hydroxide (0.0375 N, 1% w/v) solution. The acidic drug solution was added dropwise to the sodium hydroxide solution under moderate stirring (1500 rpm) for 1 h to produce the nanosuspension [ 11 ].
The selected drug is highly lipophilic. The solubility of the drug in aqueous solutions is strongly pH-dependent, with maximum solubility observed at high and low pH. In the pH range of 3–9, it is poorly soluble. According to the Henderson-Hasselbalch equation, an alteration of 1 unit in pH value will cause 10 times to change in solubility of weak electrolyte drugs [ 12 ]. It is possible to accomplish an acid-base neutralization method. The formulations produced nanosized particles by opting the acid-base neutralization method.
Experimental design
Based on the literature survey and trials, the acid-base neutralization method was further selected for the formulation of TEM nanosuspensions. From the preliminary experimental trials, it was observed that with a change in speed (1500–2000 rpm) there was no change in particle size. However, change in the stirring time resulted in reduced particle size. It was also observed that stabilizer concentration (HPMC E15) and speed of stirring [ 13 ] were major factors that affected the formation of nanosized particles. Particle size and in vitro drug release were selected as responses. Hence, the above parameters were selected as factors and experimented with low and high levels by opting 2 2 factorial design with midpoint using the Design-Expert software (version 11).
Particle size and Zeta potential
The particle size and Zeta potential of nanosuspensions were measured using HORIBA SCIENTIFIC SZ-100 ZETA SIZER at 25 °C. The samples were diluted (1:500) prior to analysis with distilled water and were measured in triplicates [ 7 ].
Drug content
Nanosuspension equivalent to 40 mg of drug was transferred into a 25-ml volumetric flask consisting of chloroform: methanol (1:5 v/v), and the flask was shaken for 20 min and was filtered. The filtrate was diluted using 0.1 N HCl: methanol (1:9 v/v), and the drug content was estimated by the above developed HPLC method [ 7 ].
In vitro drug release studies
The dissolution study of nanosuspension has been performed by using USP type–II, paddle apparatus. The accurately measured volumes of nanosuspension (5 ml) were transferred into the dissolution apparatus (Electrolab TDT-08 L, India) consisting of 900 ml of 0.1 N HCl which served as medium and operated at a speed of 50 rpm at 37 ± 0.5 °C. Five milliliters of the sample was withdrawn periodically and replaced with an equal volume of fresh medium up to 12 min. Collected samples were then filtered using the 0.1-μm PTFE syringe filter [ 7 ] (Whatman Inc., Clifton., USA).
Scanning electron microscopy (SEM)
Scanning electron microscopy (SEM, Thermo Fisher Scientific India Private Ltd., Mumbai, India) studies were conducted to study the morphology of suspended nanoparticles. The sampling cell contained a thin film of a nanosuspension solution. At × 300 magnification, images were taken with Zeiss tabletop scanning electron microscopy [ 7 ].
As per ICH Q1A (R2) guidelines, the stability studies were performed for the optimized formulations. The formulations were placed in bottles and stored at 40° ± 2 °C/75 ± 5% RH and evaluated for changes in particle size at the end of 30, 60, and 90 days [ 14 ].
In vitro drug diffusion studies
Franz diffusion apparatus was used to perform diffusion studies. A fresh goat mucosa was collected from a local slaughter shop, within an hour of excision and was taken into the lab in normal saline and used as a biological membrane. TEM equivalent to 100 mg nanosuspension was placed on the biological membrane fixed to the diffusion apparatus; 0.1 N HCl was used as a diffusion medium maintained at 37 ± 0.5 °C and rotated at 50 rpm. At predetermined time intervals, 3 ml of samples was withdrawn and replaced with the fresh medium and analyzed the drug concentration by the above developed HPLC method. The study was conducted for both plain suspension and TEM nanosuspension [ 15 ].
Ex vivo permeability studies
A fresh goat mucosa was collected from local slaughter shop (Anantapuramu, Andhra Pradesh, India), within an hour of excision and was taken into the lab in normal saline. The collected mucosa was cleaned properly and with continuous aeration; at 37 °C, the tissue was placed in an organ bath. The organ tube (receptor compartment) filled with 30 ml of 0.1 N HCl. At predetermined time intervals, 3 ml of samples was withdrawn and replaced with fresh medium and analyzed the drug concentration by the developed HPLC technique. The study was conducted for both plain suspension and TEM nanosuspension [ 15 ].
The organ tube (beaker with 30 ml of 0.1 N HCl) used as a receptor compartment. A part of the tissue in the form of a sac filled with TEM nanosuspension and placed in an organ tube with continuous aeration to study the permeation of drug (ex vivo permeability studies mimics the in vivo behavior by aeration to assess the permeation of drug). In vitro diffusion and ex vivo permeability studies were the methods to study the amount of drug that diffuses or permeates at a given time which serves as alternate to in vivo studies and provides information to conduct in vivo studies.
The optimization for analytical development was performed by selecting flow rate and pH as factors and retention time and USP plate count as responses. Initial trials with the change in the composition of the mobile phase, pH, and flow rate resulted in 3 min retention time; hence, the run time of the sample was fixed at 10 min. The absorbance was measured at 291 nm. Twenty microliter was the injection volume. Standard and sample solution was injected separately into HPLC, and major peak areas were recorded. The peak areas were taken to quantitate the amount of drug present in the sample. Further, based on the amount of TEM percentage, cumulative percentage drug release was calculated. No interferences were observed for placebo and diluents of the sample at TEM retention times that confirmed specificity. The R 2 value was 0.999 which exhibited that the calibration curve was linear in the concentration range of 150–450 μg/ml (Fig. 1 ). Relative standard deviation (RSD), theoretical plates, and tailing factor were found to be 0.15%, 7651, and 1.82%. Assay values of the drug were in the range of 97.5 to 98.6% that indicated the developed method was precise. Accuracy results revealed that at each level the average recovery was between 96.8%, 97.2%, and 98.2% with RSD as ≤ 1% that confirmed the accuracy. System suitability results revealed that the developed method was robust with slight variations.
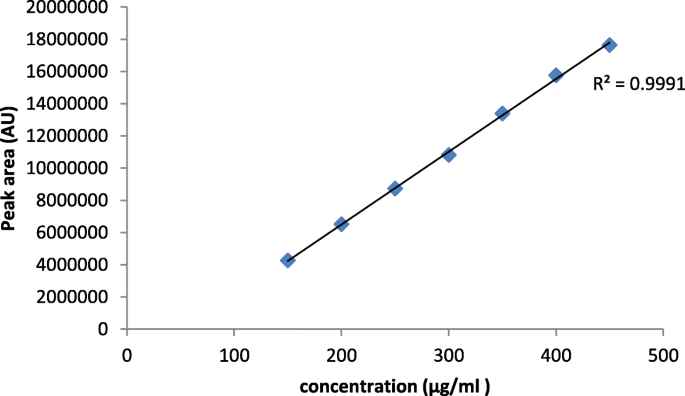
Linearity graph of telmisartan
In comparison to the plain TEM, there was an exponential increase in saturation solubility (0.1 N HCl). The optimized nanosuspension and plain TEM exhibited 0.0438 mg/ml and 0.0089 mg/ml solubility, respectively, in 0.1 N HCl, an almost 5-fold increase in solubility of nanosuspension upon comparison with TEM plain drug.
The spectrum of pure TEM showed a distinct absorption band for the carbonyl group C=O at 1695.90 cm −1 and the O–H band at 3060.85 cm −1 . The spectra with sodium bicarbonate and TEM showed a change in the C=O bond that the frequency of C=O was shifted from 1695.90 to 1722.52 cm −1 . It is an indication of strong hydrogen bonding interactions, and it has shown incompatibility with the drug.
HPLC experimental design and optimization
Flow rate and pH were selected as factors set at 0.8 to 1.0 ml/min and 4.4 to 4.7, respectively. Retention time and USP plate count were selected as responses.
Retention time
The mode F value of 107.77 implied the model was significant; there is only a 0.01% chance that a model F value this large could occur due to noise. Values of Prob > F , less than 0.05, indicated model terms are significant (Table 1 ).
In this study, A, B, and AB were significant model terms. The pre R 2 of 0.9374 was in reasonable agreement with the adj R 2 of 0.9697. An adequate precision ratio greater than 4 is desirable. The ratio of 24.193 indicated an adequate signal. This model can be used to navigate the design space. Retention time data was analyzed and exhibited a negative coefficient for flow rate and a positive coefficient for pH (Table 2 ). A positive sign represents the synergistic effect and a negative sign with an antagonistic effect. Retention times of samples were recorded and were within 2–3 min.
USP plate count
It was observed that the effects were above the t value indicated significant factors. The model F value of 28.19 implied that the model was significant. In this case, A, B, and AB were significant model terms where Prob > F was less than 0.0500 (Table 3 ). The pred R 2 of 0.9334 was in agreement with the adj R 2 of 0.8908. The adequate precision ratio of 27.272 indicated an adequate signal in which this model can be used to navigate the design space. Flow rate and pH exhibited a positive effect. From the interaction plots, it was displayed that relative slopes of the factors exhibited significant interaction flow rate and pH.
Optimized formula (analytical development)
After ANOVA and contour plot analysis (Fig. 2 ), the design yielded 12 best formulations with predicted values. Based on the desirability (> 0.990), the above formulation Table 4 was selected, and experimentally the study was conducted. The results were recorded and exhibited closeness in theoretical and experimental values, hence selected as an optimized formula.
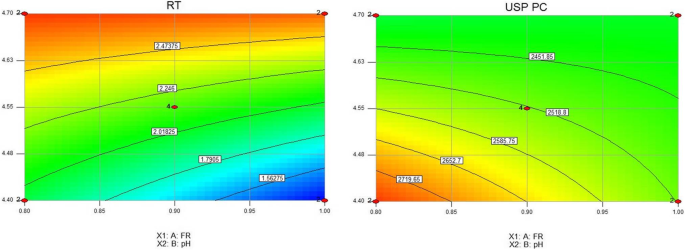
Contour plot showing the effect of flow rate and pH on retention time and USP plate count
The nanoparticles formulated by bottom-up anti-solvent precipitation method and emulsification solvent evaporation methods exhibited an average particle size of 1067 nm and 1377.1 nm, respectively, and micronized particles with PI of 0.352 and 0.619, respectively. Hence, these methods were not selected for further study.
Surface stabilizers were selected by determining the particle size of the formulated nanosuspensions with HPMC E15 and PVP. But by PVP, the particle size could not be reduced to below 500 nm; hence, HPMC was selected to further carry out DoE in which the concentrations of HPMC were set from 0.5 to 1.0 mg.
Experimental design (formulation development)
Based on the number of factors and levels, 2 2 factorial design with a midpoint was selected to investigate their effects using the Design-Expert software (version 11) (Table 5 ). The design contained experimental 8 runs which included 2 2 factorial design and mid-levels. The prepared TEM nanosuspension by all the mentioned above three methods was found to be milky white without any signs of interactions.
The particle size of nanosuspension ranged from 243.9 to 565.5 nm and PDI in the range of 0.119 to 0.450. These nanosized formulations developed by acid-base neutralization method enhanced solubility and dissolution rate. Zeta potential of the optimized formulation was found to be − 16.2 mVs (Fig. 3 )
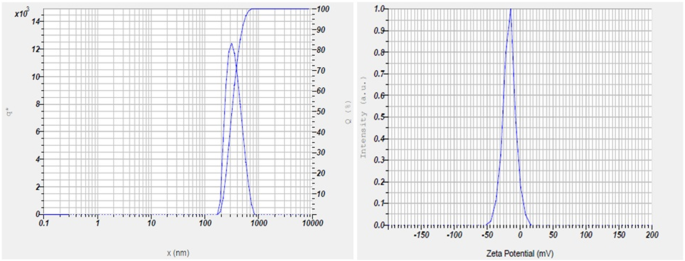
Particle size analysis and Zeta potential of stabilized formulation by acid-base neutralization method after 90 days
Particle size
The model F value of 2152.42 implied the model was significant; there was only a 0.01% chance that a model F value this large could occur due to noise. Values of Prob > F , less than 0.05, indicated model terms are significant (Table 6 ). In this study, A, B, and AB were significant model terms. The pre R 2 of 0.9995 was in reasonable agreement with the adj R 2 of 0.9985. An adequate precision ratio greater than 4 is desirable. The ratio of 204.162 indicated an adequate signal. This model can be used to navigate the design space. Particle size data was analyzed and exhibited a negative coefficient for HPMC E15 and the positive coefficient for stirring speed (Table 7 ). A positive sign represents the synergistic effect and a negative sign with an antagonistic effect. From the interaction plots, it was displayed that relative slopes of the factors exhibited significant interaction with HPMC E15 and speed.
Drug content for all the formulations ranged from 95.7 to 99.8% and met the specifications as per I.P.
In vitro drug release
It was observed that the effects were above the t value indicated significant factors. The model F value of 66.01 implied that the model was significant. In this case, A, B, and AB were significant model terms where Prob > F was less than 0.0500 (Table 8 ). The pred R 2 of 0.8993 was in agreement with the adj R 2 of 0.9512. The adequate precision ratio of 19.715 indicated an adequate signal in which this model can be used to navigate the design space. HPMC E15 exhibited positive sign, and stirring speed exhibited a negative effect.
The formulations, F1 to F5, exhibited drug release in the range of 60.5 to 100.2% (Fig. 4 ).
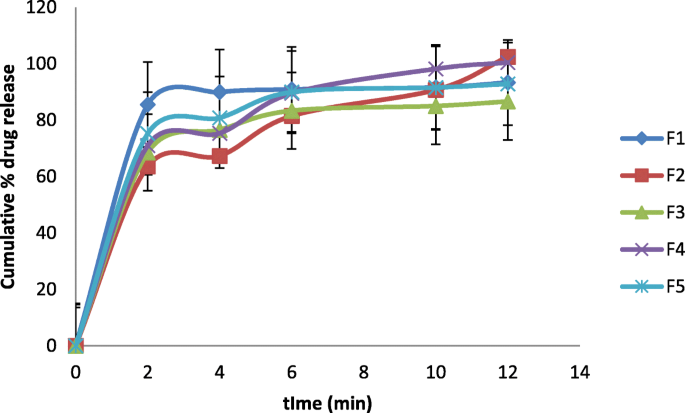
Cumulative percentage release of drug from formulations F1 to F5. Values were expressed mean ± SD, n = 6
Optimized formula (formulation development)
After ANOVA and contour plot analysis (Fig. 5 ), the design yielded 10 best formulations with predicted values. Based on the desirability (> 0.990), the above formulation Table 9 was selected, and experimentally the study was conducted. The results were recorded and exhibited closeness in theoretical and experimental values, hence selected as an optimized formulation.
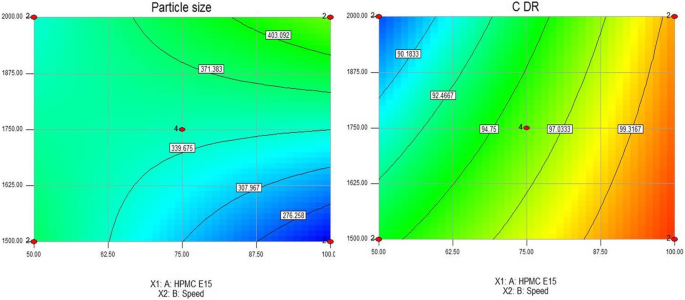
Contour plot showing the effect of HPMC E15 and stirring speed on particle size and cumulative percentage drug release
The nanosuspensions remained stable even after 90 days (nanosize) without any aggregations with a particle size of 338.1 nm and PDI 0.146.
TEM plain drug suspension was prepared by dispersing 40 mg of the drug in 3 ml of distilled water. After 3 h, 82.6% of the drug was diffused from TEM nanosuspension, and plain drug suspension diffused 42.8%, an indicator of almost 2-fold increase in diffusion.
Saturation solubility studies revealed that nanosizing of TEM produced a significant increase in solubility. The overall increase in saturation solubility in all media could be attributed to the increased dissolution pressure which was described by the Ostwald-Freundlich equation which correlates particle size with saturation solubility and states that with decreasing particle size the dissolution pressure increases thereby increasing the saturation solubility of the solute. FT-IR spectra of optimized TEM nanosuspension showed carbonyl group at 1641.16 cm −1 and disappearance of O–H band; it was due to interaction with HPMC E15, indicated the formation of strong protective polymer stabilizer coat over drug nanoparticles to prevent the aggregation. It can be confirmed that the drug was compatible with sodium hydroxide. Retention behavior changes with a change in the pH of the buffer. The drug is nonpolar, and its elution was influenced by the mobile phase (polar). The flow rate and pH were adjusted, and it was observed that an increase in flow rate reduced the retention time; hence, the parameters were optimized to exhibit a high flow rate. An increase in pH increased USP plate count; hence, higher pH is selected. Further, an increase in flow rate and pH gave better symmetric resolved peaks without tailing to yield shorter retention time and increased USP plate count. Initial screening studies indicated the change in particle size by change in stirring speed. Hence, the concentration of HPMC and stirring speed were identified as critical formulation parameters. Particle size and cumulative percentage drug release were identified as responses. The enhanced solubility and dissolution rate by acid-base neutralization method might be due to the formation of nanosized particles. Zeta potential of optimized formulation indicated macroscopic stability of suspensions as the value was within ± 30 mVs.
It has been observed that an increase in the concentration of HPMC E15 decreased particle size and increased drug release, and increase in stirring speed increased particle size and decreased drug release. Both the particle size and PI value decreased significantly with the increase in stabilizer concentration. Although the particle size increased when the total stabilizer concentration increased from 1.0%, there were no significant differences. The concentration of stabilizer is important to the successful production nanosuspensions, which means that a sufficient amount of stabilizer must adsorb onto the particle surface to provide a barrier to agglomeration. By intermolecular hydrogen bonds formation between TEM and HPMC E15, crystal growth was inhibited. By the mechanism of electrostatic, steric forces, and by undergoing adsorption of the HPMC E15 at the surface of the drug, by rapid nucleation and formation of the metastable zone, the creation of turbulence by mechanical stirrer leads to the prevention of crystals formation. An increase in Gibbs free energy, reduction of particle size, and enhanced solid-liquid interfacial area produced thermodynamically stable TEM nanosuspension. Both particle size and PDI decreased at 1500 rpm on further increasing the speed; the particle size increased due to damage to the stabilizer layer and might have induced agglomeration. In the present study, chloroform was selected as the solvent due to its higher solubilization potential for TEM and high volatility. Rapid evaporation of the solvent is thus possible which results in higher supersaturation and rapid nucleation, all prerequisites for ultra-fine crystal size. Hydrophilic polymers used as stabilizers stabilized the nanoparticles by steric stabilization. Pareto chart is a useful tool to identify statistically significant effects by t value limits; the effects were above the t value indicated factors were significant. The normal probability plot residuals followed a normal distribution and followed a straight line. It was evident from the plots of residuals versus predicted and residuals versus run that error terms were not associated with one another, and it adequately showed that the model fits the data obtained from software. Predicted versus actual plot data points exhibited a split by the 45° line indicated significance. Cook’s distance provides valuable information regarding the measurement of regression effect changes if the case is deleted, large values should be identified which were produced by recording errors and should be corrected. In this case, no large values were observed; it indicated the data without any errors (Fig. 6 ). SEM analysis indicated that particles were nearly spherical and were in the nano range (Fig. 7 ). TEM plain drug suspension was prepared by dispersing 40 mg of the drug in 3 ml of distilled water. Enhanced diffusion from TEM nanosuspensions might be due to increased solubility and dissolution rate of the drug in nanoform which might improve bioavailability (Fig. 8 a). Permeation studies proved that after 3 h of diffusion, 85.3% of drug diffused from TEM nanosuspension and plain drug suspension diffused 40.6% (Fig. 8 b). It clearly showed that the drug permeation from TEM nanosuspension was much faster than plain drug suspension; it is a clear indicator for enhancement in bioavailability. It indicated that the presence of TEM in nanoform and with suitable adsorption of stabilizers improved the permeation than plain drug suspension which is a clear indicator for enhanced stability and bioavailability.
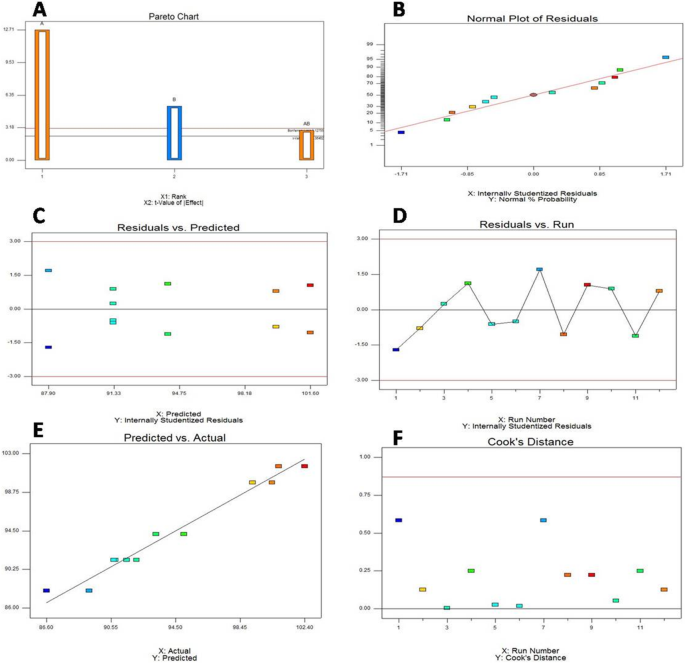
Statistically significant plot effects of responses. a Pareto chart. b Normal probability plot residuals. c Residuals versus predicted. d Residuals versus run. e Predicted versus actual plot. f Cooks distance
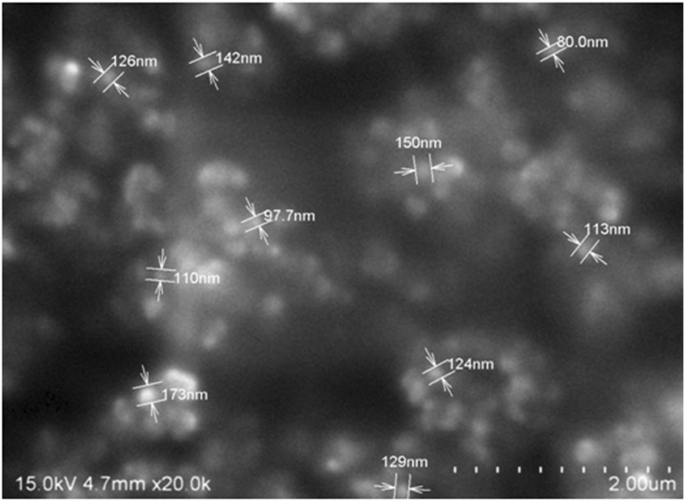
SEM image exhibiting morphology and particle size
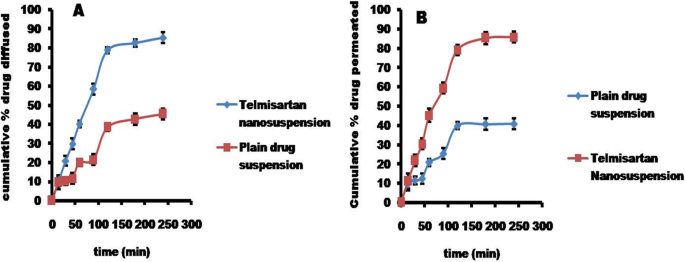
Cumulative percentage drug diffused. a Ex vivo diffusion studies. b Ex vivo permeation studies. Values were expressed mean ± SD, n = 3
The formulated and optimized TEM nanoformulation upon further evaluation (in vivo studies) might be useful to effectively treat hypertension with enhanced bioavailability which is commonly prescribed (TEM) by the prescribers rather than in the form of tablets that are available in the market. The study can be extensively studied and developed by computational algorithmic artificial neural network technology and by molecular dynamic and simulation studies to further optimize the formulation at lower cost with in-built quality.
Availability of data and materials
All data and material are available upon request.
Abbreviations
Design of experiment
Degrees of freedom
High-performance liquid chromatography
Hydroxypropyl methylcellulose
Poly Dispersity Index
Probability
Polytetrafluoroethylene
Polyvinylpyrrolidone
Telmisartan
Chockalingam A, Campbell NR, Fodor JG (2006) Worldwide epidemic of hypertension. Can J Cardiol 22(7):553–555
PubMed PubMed Central Google Scholar
Bajaj A, Rao MR, Pardeshi A, Sali D (2012) Nanocrystallization by evaporative antisolvent technique for solubility and bioavailability enhancement of telmisartan. AAPS pharmscitech 13(4):1331–1340
CAS PubMed PubMed Central Google Scholar
Xu Y, Liu X, Lian R, Zheng S, Yin Z, Lu Y (2012) Enhanced dissolution and oral bioavailability of aripiprazole nanosuspensions prepared by nanoprecipitation/homogenization based on acid-base neutralization. Int J Pharm 438(1-2):287–295
CAS PubMed Google Scholar
Chen H, Khemtong C, Yang X, Chang X, Gao J (2011) Nanonization strategies for poorly water-soluble drugs. Drug Discov Today 16(7-8):354–360
Kocbek P, Baumgartner S, Kristl J (2006) Preparation and evaluation of nanosuspensions for enhancing the dissolution of poorly soluble drugs. Int J Pharm 312(1-2):179–186
Rao M, Bajaj A (2014) Study of effect of variables on particle size of telmisartan nanosuspensions using box-Behnken design. Drug Res 64(12):663–667
CAS Google Scholar
Vuppalapati L, Cherukuri S, Neeli V, Reddy Yeragamreddy P, Reddy Kesavan B (2016) Application of central composite design in optimization of valsartan nanosuspension to enhance its solubility and stability. Curr Drug Deliv 13(1):143–157
Peraman R, Kalva B, Reddy YP, Sharma H (2016) Analytical quality by design approach in selection of method variables for simultaneous analysis of ciprofloxacin and hydrocortisone by LC method using Taguchi method. Anal Chem Lett 6(1):1–12
Liu D, Xu H, Tian B, Yuan K, Pan H, Ma S (2012) Fabrication of carvedilol nanosuspensions through the anti-solvent precipitation–ultrasonication method for the improvement of dissolution rate and oral bioavailability. AAPS Pharmscitech 13(1):295–304
Hoa LTM, Chi NT, Nguyen LH, Chien DM (2012) Preparation and characterisation of nanoparticles containing ketoprofen and acrylic polymers prepared by emulsion solvent evaporation method. J Exp Nanosci 7(2):189–197
Daebis N, El-Massik M, Abdelkader H (2015) Formulation and characterization of itraconazole oral nanosuspension: methyl cellulose as promising stabilizer. Ely J Pharm Res 1(1):102
Google Scholar
Lindfors L, Skantze P, Skantze U, Rasmusson M, Zackrisson A, Olsson U (2006) Amorphous drug nanosuspensions - inhibition of Ostwald ripening. Langmuir 22(3):906–910
Sun W, Mao S, Shi Y, Li LC, Fang L (2011) Nanonization of itraconazole by high pressure homogenization: stabilizer optimization and effect of particle size on oral absorption. J Pharm Sci 100(8):3365–3373
Ahuja BK, Jena SK, Paidi SK, Bagri S, Suresh S (2015) Formulation, optimization and in vitro–in vivo evaluation of febuxostat nanosuspension. Int J Pharm 478(2):540–552
Thakkar HP, Patel BV, Thakkar SPJJoP, Sciences B (2011) Development and characterization of nanosuspensions of olmesartan medoxomil for bioavailability enhancement. J Pharm Bioallied Sci 3(3):426.
Download references
Acknowledgements
The authors thank DIST-FIST facility, RERDS-CPR, Raghavendra Institute of Pharmaceutical Education and Research for providing necessary facilities to carry out the research work.
Not applicable.
Author information
Authors and affiliations.
RERDS-CPR, Raghavendra Institute of Pharmaceutical Education and Research, Anantapuramu, 515721, India
E. Bhargav, Y. Padmanabha Reddy & P. Ramalingam
Department of Pharmaceutics, Raghavendra Institute of Pharmaceutical Education and Research, Anantapuramu, 515721, India
G. Chaithanya Barghav & Chintamaneni Pavan kumar
Department of Industrial Pharmacy, Raghavendra Institute of Pharmaceutical Education and Research, Anantapuramu, 515721, India
C. Haranath
You can also search for this author in PubMed Google Scholar
Contributions
E Bhargav (EB), G Chaithanya Barghav (GB), Y Padmanabha Reddy (YP), P Ramalingam (PR), C. Haranath (CH), Chintamaneni Pavan kumar (CP)
EB and CP designed the work, title based on literature survey. GB assisted in literature survey and formulation development. The work was started by screening the variables and studying its impact on responses. EB, YP, and CH assisted in formulation design by experimental software (Design expert Version 11) for experimentation. It was optimized by identifying the better formula with cost effectiveness and by contour plots. PR assisted on usage of the Design expert software (version 11), widely used software for optimization with regulatory acceptability, interpretation of analytical data work, and statistical data. EB interpreted the formulation and analytical data. GB performed ex vivo studies. After completion of studies, the manuscript writing was carried out. The work was finally drafted and revised. CP helped in manuscript writing and drafting. All authors have read and approved the manuscript.
Corresponding author
Correspondence to E. Bhargav .
Ethics declarations
Competing interest.
No competing interests to declare.
Ethics approval and consent to participate
Not applicable
Consent for publication
Additional information, publisher’s note.
Springer Nature remains neutral with regard to jurisdictional claims in published maps and institutional affiliations.
Rights and permissions
Open Access This article is licensed under a Creative Commons Attribution 4.0 International License, which permits use, sharing, adaptation, distribution and reproduction in any medium or format, as long as you give appropriate credit to the original author(s) and the source, provide a link to the Creative Commons licence, and indicate if changes were made. The images or other third party material in this article are included in the article's Creative Commons licence, unless indicated otherwise in a credit line to the material. If material is not included in the article's Creative Commons licence and your intended use is not permitted by statutory regulation or exceeds the permitted use, you will need to obtain permission directly from the copyright holder. To view a copy of this licence, visit http://creativecommons.org/licenses/by/4.0/ .
Reprints and permissions
About this article
Cite this article.
Bhargav, E., Chaithanya Barghav, G., Padmanabha Reddy, Y. et al. A Design of Experiment (DoE) based approach for development and optimization of nanosuspensions of telmisartan, a BCS class II antihypertensive drug. Futur J Pharm Sci 6 , 14 (2020). https://doi.org/10.1186/s43094-020-00032-2
Download citation
Received : 07 January 2020
Accepted : 07 May 2020
Published : 20 May 2020
DOI : https://doi.org/10.1186/s43094-020-00032-2
Share this article
Anyone you share the following link with will be able to read this content:
Sorry, a shareable link is not currently available for this article.
Provided by the Springer Nature SharedIt content-sharing initiative
- Bioavailability
- Ex vivo studies
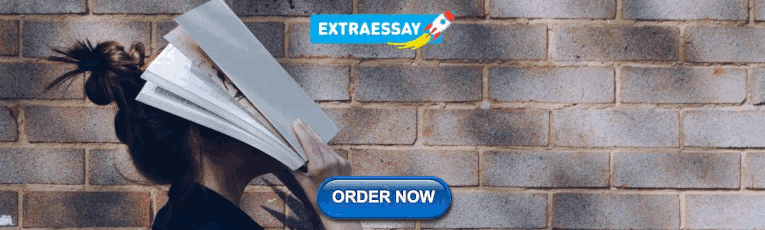
IMAGES
VIDEO
COMMENTS
PREPARATION OF NANOSUSPENSION. For the preparation of nanosuspensions, mostly two methods namely "Bottom up technology" and "Top down technology" are used, as shown in Figure 1.[] Bottom up technology is an assembling method to form nanoparticles like precipitation, microemulsion, melt emulsification method and top down technology involves the disintegration of larger particles into ...
PDF | On Jan 1, 2017, Srinivasa Rao Yarraguntla and others published Nanosuspensions: A Review | Find, read and cite all the research you need on ResearchGate
Solubility proves to be a major hurdle for the successful development and commercialization of new drug products. Since 40% of the active substances being identified through the new paradigm in ...
The solubility of nanosuspension was enhanced five times. 57% and 18% of RTV were dissolved in FeSSIF medium for nanosuspension and coarse powder. C max and AUC 0−t values in nanosuspension displayed an 8.9- and a 12.5-fold increase, respectively, compared to the coarse powder, and a 1.9- and 2.1-fold increase, respectively, compared to the ...
Rapid advancement in drug discovery process is leading to a number of potential new drug candidates having excellent drug efficacy but limited aqueous solubility. By virtue of the submicron particle size and distinct physicochemical properties, nanosuspension has the potential ability to tackle many formulation and drug delivery issues typically associated with poorly water and lipid soluble ...
Nanosuspension has arisen as a remunerative, lucrative as well as a potent approach to improve the solubility and bioavailability of poorly aqueous soluble drug entities. Several challenges are still present in this approach which need more research. The prime aim of this review is to identify such challenges that can be rectified in the future.
Nanosuspension is a submicron colloidal dispersion of drug. particles. A pharmaceutical nanosuspension is defined as very finely. colloid [1], biphasic [2], dispersed solid drug particles in an ...
Nanosuspension: Principles, Perspectives and Practices Curr Drug Deliv. 2016;13(8):1222-1246. doi: 10.2174/1567201813666160101120452. Authors Sachin Kumar ... This article reviews various techniques being employed for production, characterization, merits and limitations of nanosuspensions and mechanisms that play a role in the physicochemical ...
Nanocrystals and nanosuspensions have become realistic approaches to overcome the formulation challenges of poorly water-soluble drugs. They also represent a less-known but versatile platform for multiple therapeutic applications. They can be integrated into a broad spectrum of drug delivery systems including tablets, hydrogels, microneedles, microparticles, or even functionalized liposomes ...
The homogenizing phase can be carried out using a single homogenizing valve (suitable for scattering treatment), or a double homogenizing valve (suggested when you have to use emulsion and for controlling viscosity whenever requested) as shown in Fig. 4.Curcumin nanoparticles containing 50% soluplus prepared by the HPH method showed a 16-fold increase in solubility compared to the original form.
The formulation of ibuprofen as a nanosuspension, ... Research work was supported by the Ministry of Higher Education, Science and Technology of the Republic of Slovenia. ... Intravenous itraconazole emulsions produced by SolEmuls technology. Eur. J. Pharm. Biopharm., 56 (2003), pp. 29-36. View PDF View article View in Scopus Google Scholar ...
The preparation of nanosuspension-loaded dissolving MNs has been widely carried out using knife-casting methods. In the present review, the various characterization methods, including the in vitro, ex vivo and in vivo techniques, are briefly discussed. Further, the review also describes the potential applications of nanosuspension-loaded MNs.
Nanosuspension is colloidal dispersion of nano-sized drug particles stabilized by surfactants. They can also define as a biphasic system consisting of pure drug particles dispersed in an aqueous vehicle. The diameter of suspended particle is less than 1μm in size [5].
oral, ocular, brain, topical, buccal, nasal and transdermal ro utes are currently undergoing extensive research. Oral drug delivery of nanosuspension with receptor mediated endocytosis has the promising ability to resolve most permeability limited absorption and hepatic first-pass metabolism related issues adversely affecting bioavailability.
Aher et al. Int J Curr Pharm Res, Vol 9, Issue 3, 19-23 19 Review Article NANOSUSPENSION: AN OVERVIEW SMITA S. AHER *1, SAGAR T. MALSANE 2, R. B. SAUDAGAR 1 1*Department of Pharmaceutical ...
nm = 10-9m= 10-7 cm = 10-6 mm. Micron = 10-6m= 10-4 cm = 10-3mm 4. For a long duration of time micronization of poorly soluble drugs by colloid mills or jet mills was preferred. The overall particle size distribution ranges from 0.1μm to approximately 25μm, only negligible amount being below 1μm in the nanometer range.
Generally, nanosuspension is assuming a dispersion of hydrophobic nanoparticles in water as a hydrophilic medium. The enormous increase of surface area due to reduction of particle size results in increase of particles' free energy while The interfacial tension remains constant, and is considered the main reason of thermodynamic instability ...
Article Received on 30/08/2020 Article Revised on 21/09/2020 Article Accepted on 11/10/2020 INTRODUCTION nanosuspension Nanosuspension is combination of two words i.e. Nano ... Biomaterials Research Nanosuspension contributes a significant role in administering different drug entities through a variety of routes involves oral, transdermal ...
Pulmonary Nanosuspension increase medication diffusion and disintegration, which improves bioaccumulation and predicts unintended drug deposition in the mouth and pharynx. 2.5.4 Ocular administration. Ocular drug delivery as a Nanosuspension has been concentrated to provide a gentle and long-lasting release of the prescription.
nanosuspension not only solves the problems of low solubility and. bioavailability, but also affects the drug's pharmacokinetics and thus. improves drug safety and effectiveness. Nanosuspensions ...
Nanosuspension is a part of nanotechnology which is a submicron colloidal dispersion of pharmaceutically active ingredients in a liquid phase having a size range below 1 um, and which is stabilized by surfactants and polymers. Most of the newly developed drugs are water-insoluble, show poor bioavailability.
The present study is aimed to formulate and evaluate celecoxib oral nanosuspension to improve the bioavailability of. the drug with varying concentrations of surfactants and co surfactants. The ...
The dissolution study of nanosuspension has been performed by using USP type-II, paddle apparatus. The accurately measured volumes of nanosuspension (5 ml) were transferred into the dissolution apparatus (Electrolab TDT-08 L, India) consisting of 900 ml of 0.1 N HCl which served as medium and operated at a speed of 50 rpm at 37 ± 0.5 °C.