
An official website of the United States government
The .gov means it’s official. Federal government websites often end in .gov or .mil. Before sharing sensitive information, make sure you’re on a federal government site.
The site is secure. The https:// ensures that you are connecting to the official website and that any information you provide is encrypted and transmitted securely.
- Publications
- Account settings
Preview improvements coming to the PMC website in October 2024. Learn More or Try it out now .
- Advanced Search
- Journal List
- Front Plant Sci
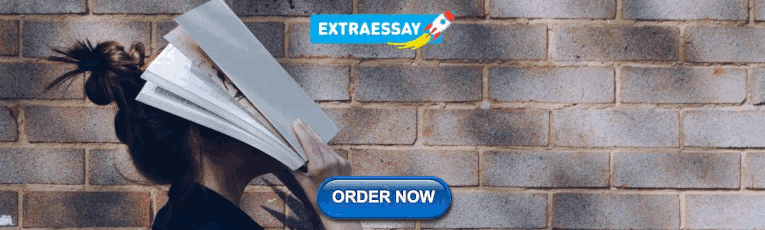
Morphology, Photosynthetic Traits, and Nutritional Quality of Lettuce Plants as Affected by Green Light Substituting Proportion of Blue and Red Light
1 Institute of Environment and Sustainable Development in Agriculture, Chinese Academy of Agricultural Sciences, Beijing, China
2 Key Laboratory of Energy Conservation and Waste Management of Agricultural Structures, Ministry of Agriculture, Beijing, China
Yu-xin Tong
Jun-ling lu, yang-mei li, rui-feng cheng, associated data.
The original contributions presented in the study are included in the article/ Supplementary Material , further inquiries can be directed to the corresponding author/s.
Green light, as part of the photosynthetically active radiation, has been proven to have high photosynthetic efficiency once absorbed by plant leaves and can regulate plant physiological activities. However, few studies have investigated the appropriate and efficient way of using the green light for plant production. Thus, the objective of this study was to investigate a moderate amount of green light, partially replacing red and blue light, for plant growth and development. In this experiment, four treatments were set up by adjusting the relative amount of green light as 0 (RB), 30 (G30), 60 (G60), and 90 (G90) μmol m −2 s −1 , respectively, with a total photosynthetic photon flux density of 200 μmol m −2 s −1 and a fixed red-to-blue ratio of 4:1. Lettuce ( Lactuca sativa cv. ‘Tiberius’) plant growth and morphology, stomatal characteristics, light absorptance and transmittance, photosynthetic characteristics, and nutritional quality were investigated. The results showed that: (1) shoot dry weight increased by 16.3 and 24.5% and leaf area increased by 11.9 and 16.2% under G30 and G60, respectively, compared with those under RB. Plant stem length increased linearly with increasing green-to-blue light ratio; (2) light transmittance of lettuce leaf under treatments employing green light was higher than that under RB, especially in the green region; (3) stomatal density increased, whereas stomatal aperture area decreased with the increase in the relative amount of green light; and (4) carbohydrate accumulation increased under G60 and G90. Soluble sugar contents under G60 and G90 increased by 39.4 and 19.4%, respectively. Nitrate contents under G30, G60, and G90 decreased by 26.2, 40.3, and 43.4%, respectively. The above results indicated that 15–30% green light replacing red and blue light effectively increased the yield and nutritional quality of lettuce plants.
Introduction
Plants perceive not only light intensity and photoperiod but also light quality, including monochromatic and polychromatic light, as ambient growth environment signals that induce a large number of physiological responses (Kami et al., 2010 ). Different light qualities have distinctly different biological effects on plants (Li et al., 2020 ). There is a misconception that green light is less useful for plant photosynthesis, probably because the light absorption of photosynthetic pigments is relatively low within the green region compared with that within the red and blue regions, especially in the plant canopy (McCree, 1972 ; Smith et al., 2017 ). This is also the reason that red and blue light, rather than other lights, are widely used in recently developed plant factories with artificial lighting for plant production (Wang et al., 2016 ). Moreover, the photoreceptors of red and far-red, blue, and UV-B have been identified as phytochromes, cryptochromes and phototropins, and UV Resistance Locus 8 (Bantis et al., 2018 ), respectively, but no specific green light photoreceptor was detected in previous studies. Even so, the vital role of green light affecting plant physiological activities was gradually proved in previous studies (Johkan et al., 2012 ; Wang and Folta, 2013 ; Materová et al., 2017 ).
A recently published review suggested that green light distributed energy among plant leaves and canopies (Smith et al., 2017 ) because green light was capable of reaching deeper and drives CO 2 fixation under plant canopy, whereas most of the red and blue light is generally absorbed by the upper part of plant leaves (Sun et al., 1998 ; Schenkels et al., 2020 ). In addition, a green light could easily induce a shade avoidance syndrome in plants because, in natural conditions, green light transmitted through the plant canopy, causing a sharp decline in blue-to-green (B/G) ratio from the top to the bottom layers of plants (Zadoks et al., 1974 ). Similar to the shading response caused by far-red light, plant stem elongation and leaf area expansion also occurred under green light (Sellaro et al., 2010 ; Zhang et al., 2011 ). Besides, green light shifted cryptochromes from semi-reduced active state caused by blue light to the fully reduced and inactive state. Thus, green light could inactivate blue-mediated responses (Bouly et al., 2007 ; Liu et al., 2010 ; Sellaro et al., 2010 ).
Recent studies suggested that green light should not be ignored in plant growth and development. Kaiser et al. ( 2019 ) reported that, by replacing red and blue with green light, the fresh and dry weights of tomato increased linearly with an increase in the percentage of green light. Schenkels et al. ( 2020 ) have shown that additional green light significantly increased the total fresh and dry weights of plant seedlings, whereas when replacing red and blue with green light, no significant increase was found in dry weight. The above inconsistent results were mainly caused by the different proportions of green light in the light source. According to McCree ( 1972 ), once absorbed by plant leaves, green light showed a higher relative quantum efficiency than blue light. Moreover, the shade avoidance syndrome induced by green light was capable of increasing the stem length and leaf area of plants (Johkan et al., 2012 ). These changes in plant morphology effectively enhance the photons captured by plant leaves, improving the photosynthetic efficiency (Park and Runkle, 2017 ). Thus, investigating a moderate proportion of green light can optimize the photosynthesis of plant leaves to maximize the yield in plant production.
Therefore, this study aimed to investigate the optimal proportion of green light for lettuce growth and development by adjusting the relative amount of green light. Combined red and blue light were used as the fundamental light. Lettuce ( Lactuca sativa cv. ‘Tiberius’) was used in this experiment because it is one of the most popular horticulture vegetables produced in plant factories with artificial lighting. Lettuce morphology, biomass, stomatal characteristics, light absorptance and transmittance, photosynthetic traits, and nutritional quality were evaluated.
Materials and Methods
Plant material and growth condition.
Lettuce ( Lactuca sativa cv. ‘Tiberius’) seeds were sown in sponge blocks filled in plastic trays. Seedlings were developed under fluorescent lamps (TL-D56W, Osram, Munich, Germany) with a light intensity of 150 μmol m −2 s −1 and a photoperiod of 16 h day −1 . Upon emerging three true leaves (15 days after sowing), seedlings were transplanted in a plant factory with artificial lighting (PFAL), in which the day/night temperature was 24/20°C, relative humidity was 60–70%, and the concentration of CO 2 (supplied by a CO 2 gas cylinder) was 1,000 μmol mol −1 . Plants were cultivated in a customized circulation system of nutrient solution with a planting density of 32 plants m −2 . The light-emitting diode (LED) panels (Datang New Energy Technology Co., Ltd., Shenzhen, China) were equipped on cultivation shelves. The distance between the LED panels and the culture bed was 0.3 m. An opaque white plastic reflective film was placed around the LED panels to ensure uniform radiation on the surface of the culture beds and to prevent light pollution from the adjacent treatments. Modified Yamasaki nutrient solution (EC = 1.2 dS m −1 , pH = 5.8) was applied for the plant growth. The circulation system was automatically operated for 1 h day −1 .
Experimental Setup
The photosynthetic photon flux density (PPFD) and red (600–690 nm, peak at 660 nm) to blue (410–490 nm, peak at 450 nm) (R/B) ratio of all treatments were maintained at 200 μmol m −2 s −1 and 4:1, respectively. The relative amount of green light (490–590 nm, peak at 525 nm) of each treatment was adjusted to 0 (RB), 30 (G30), 60 (G60), and 90 (G90) μmol m −2 s −1 ( Table 1 ). The light spectrum of each treatment was presented in Figure 1 . Plants were harvested on day 20 after transplanting. This experiment was repeated three times.
Different light intensity (μmol m −2 s −1 ) of red, blue, and green light of four treatments.

The spectral distribution of different treatments (A) and photograph of lettuce grown under treatments (B) .
Determinations of Plant Growth and Morphology
For the growth and morphology analysis, destructive measurements were taken on five plants of each treatment on day 20 after transplanting. Lettuce samples were separated into shoot and root using sharp scalpels and forceps. The stem length was the average value of stem lengths in fully expanded leaves of each plant. The leaf area was measured by an area meter (LI-3100C, LI-COR Biosciences, Lincoln, NE, USA). The fresh and dry weights of shoot and roots were measured by an electronic balance (Si-234; Denver Instrument, Bohemia, NY, USA). Whole-plant net assimilation was calculated by dividing shoot dry weight by total leaf area for each plant.
Measurements of Light Absorptance and Light Transmittance of Leaves
Light reflectance (Rf) and light transmittance (Tr) were measured on the fully expanded second leaves with a spectroradiometer (Ocean Optics USB2000+, Dunedin, FL, USA) in combination with two integrating spheres (FOIS-1, ISP-REF, Ocean Optics Inc., Dunedin, FL, USA). Light absorptance (Ab) was calculated as: Ab = 1 – (Rf + Tr). The diagram of the measuring apparatus is showed in Supplementary Figure 1 .
Observation of Stomata
Leaf samples were collected from the fully expanded second young leaves of five plants in the same region of each treatment. Leaf stomatal characteristics were measured using the method of Wang et al. ( 2016 ). The stomata of plants are regarded as rhombus. Stomatal aperture area was calculated by long axis length of aperture (Al) and short axis length of aperture (As). Stomatal aperture area = Al × As/2.
Determinations of Photosynthetic Pigments and Photosynthetic Characteristics
From the top to the bottom, the leaves of 2, 4, 6, 8, 10, 12, 14, 16, 18, and 20 from four plants in each treatment were collected at the same point with 1 cm 2 by using a punch. Subsequently, 96% ethanol was used as a solvent for these samples to measure chlorophyll a (Chl a), chlorophyll b (Chl b), and carotenoid content. The absorbance was measured at 665, 649, and 470 nm by using a spectrophotometer (UV-1800, Shimadzu Corp., Kyoto, Japan). Concentrations of chlorophyll and carotenoid were determined using the equations reported by Lichtenthaler and Wellburn ( 1983 ). All of the data measured were used for the normal distribution analysis shown in Figure 7 .

Box-plot and normal distribution of concentrations of total chlorophyll (A) , carotenoid (B) , and Chl a/Chl b (C) of different layers of lettuce under different proportions of green light.
Net photosynthetic rate (P n ), gas exchange (g s ), and intercellular CO 2 concentration (Ci) of lettuce fully expanded second leaves were measured using a portable photosynthetic instrument (LI-6400XT, LI-COR Biosciences, Lincoln, NE, USA) with a transparent leaf chamber (6400-08, LI-COR Biosciences, Lincoln, NE, USA) under the actual light condition of plant growth. The environmental conditions of the leaf chamber were maintained at 24°C, 1,000 μmol m −2 s −1 CO 2 level, and 60–70% relative humidity (Li et al., 2020 ). The order of measurements was arranged randomly for each repetition, and each treatment was repeated three times. P n and g s of lettuce leaves measured in LED leaf chamber were represented by P n−T and g s−T , respectively.
P n and g s to light intensity response curves were taken on the fully expanded second leaves by using the same portable photosynthetic instrument (LI-6400XT, LI-COR Biosciences, Lincoln, NE, USA) with a red–blue LED leaf chamber (6400-02B, LI-COR Biosciences, Lincoln, NE, USA) installed. The leaf chamber temperature, the concentration of CO 2 , relative humidity, airflow rate, and leaf-to-air vapor pressure difference (VPD leaf−air ) in the leaf chamber were 24°C, 1,000 μmol mol −1 , 60–70%, 500 μmol s −1 , and 1.0 ± 0.1 kPa, respectively. P n and g s under different light intensities were measured subsequently. The starting light intensity was 200 μmol m −2 s −1 , followed by 100, 0, 200, 400, 600, 800, 1,000, and 1,200 μmol m −2 s −1 . Light intensity decreasing from the actual growth level to dark and then gradually increasing to high light intensities was needed when measuring light intensity-photosynthesis response curves of plant leaves (Wang et al., 2016 ). The light source was red and blue light with an R/B ratio of 4:1. P n and g s were recorded when P n reached a steady state at each light intensity. Measurement order was arranged randomly for every repetition, and each treatment was repeated three times. P n and g s of lettuce leaves measured in LED leaf chamber were represented by P n−L and g s−L , respectively.
Determinations of Sucrose, Starch, and Nutritional Quality
Lettuce leaves were collected in sample bottles, quick-frozen by using liquid nitrogen, and stored in an ultralow temperature freezer before the end of the dark period. Five plants of each treatment were taken at the end of the light period on day 20 after transplanting. The samples were used to measure sucrose, starch, and nutritional quality contents, including soluble sugar, nitrate, and crude fiber. Sucrose content was determined using the method described by Fils-Lycaon et al. ( 2011 ) and measured at 480 nm. Starch content was measured and calculated according to Clegg ( 1956 ). Soluble sugar content was determined using the method described by Fairbairn ( 1953 ). Nitrate content was determined by the method described by Cataldo et al. ( 1975 ). The ELISA performed by using the double-antibody one-step sandwich method was used to determine the crude fiber content of lettuce leaves. The absorbance was measured at 450 nm wavelength with a spectrophotometer (Shimadzu UV-1800, Shimadzu Corp., Kyoto, Japan).
Statistical Analysis
This experiment was designed as a single factor experiment. A one-way ANOVA was performed for each treatment. When the ANOVA result was significant, Duncan's multiple range test at p < 0.05 was used for mean separation. The linear relationships between plant stem length and G/B ratio were determined by GraphPad Prism 8 (GraphPad Software Inc., San Diego, CA, USA), using simple linear regression. The fitting light intensity response curves of P n and g s with non-linear regression were performed by the GraphPad Prism 8 (GraphPad Software Inc., San Diego, CA, USA), using [Agonist] vs. response–variable slope (four parameters) equation. The data of chlorophyll concentration in different layers of lettuce plants were performed by a box-plot and drawn with the Origin 2020 software (OriginLab Inc., Northampton, MA, USA). The desperation of data is proportional to the height of the box. The lines and dots in the box represent the median and the average values, respectively. The highest and the lowest point of the vertical line represent the maximum and minimum values. All statistical analyses were performed by the IBM SPSS Statistics 26 program (SPSS Inc., Chicago, IL, USA). This experiment was repeated three times.
Growth and Morphology
As shown in Figure 2 , plant fresh weight, leaf area, and shoot dry weight were 12–25% greater under G30 and G60 than those under RB or G90. The increased leaf area accounted for most of the increased plant mass so that the trend of whole-plant net assimilation was nearly constant with the leaf area among four treatments. The stem length of lettuce increased linearly with increasing G/B ratio ( Figure 3 ).

Plant fresh weight (A) , leaf area (B) , shoot dry weight (C) , and whole-plant net assimilation (D) of lettuce plants as affected by the different proportions of green light. Different letters in each column indicate significant differences at p < 0.05, according to the least significant difference (LSD) test.

Stem length of lettuce leaves as affected by green-to-blue (G/B) ratio. Associated correlation of coefficients ( R 2 ) and regression equations presented here indicate significant differences at p < 0.05.
Light Absorptance and Light Transmittance of Lettuce Leaves
As shown in Figure 4 , the lowest light absorptance and highest light transmittance of lettuce leaves were observed in the green range within the visible spectrum of 400–700 nm. Meanwhile, the light transmittance was higher under treatments employing green light than that under RB, whereas the light absorptance was, on the contrary, especially within the green region. The minimal absorptance and maximal transmittance found at the same wavelength point (555 nm) were 80% under G30 and 8% under G60, respectively.

Light absorptance (A) and light transmittance (B) as affected by the different proportions of green light.
Stomatal Characteristics
As shown in Table 2 , the stomatal development was significantly affected by the relative amount of green light. Stomatal density increased by 47, 38, and 71% under G30, G60, and G90, respectively, compared with that under RB, whereas epidermal cell density only increased under G90. With the increase in green proportion from 15 to 45%, the long axis length and short axis length of aperture decreased by 7–25% and 14–51%, respectively. The reduction of long and short axis length of aperture caused a linear decrease in aperture area from 45.1 to 16.5 μm 2 with an increase in the relative amount of green light ( Figure 5 ).
Effects of green light on stomatal characteristics of lettuce.
The data are means ± SDs (n = 5). Different lowercase letters in the same line show significant difference at p < 0.05, according to the least significant difference (LSD) test .

Photos of stomatal characteristics under the different proportions of green light.
Leaf Photosynthetic Pigments
The concentrations of total chlorophyll and carotenoids, and Chl a/Chl b in different leaf layers of lettuce were different. As shown in Figure 6 , these three pigment parameters increased dramatically from the 2nd to the 4th leaf. Meanwhile, the total chlorophyll and carotenoids under G60 and G90 reached their peak at the 4th leaf, whereas they decreased dramatically at the 6th leaf. Then, the curves of the three pigment parameters were relatively flat under G30, G60, and G90 from the 6th to the 14th leaf, and gradually dropped from the 14th to the 20th leaf. A significantly decrease under RB was observed on the three pigment parameters from the 12th to the 20th leaf and finally reached the lowest level among all treatments.

The concentrations of total chlorophyll (A) , carotenoids (B) , and of leaves and Chl a/Chl b (C) of different layers of lettuce plants as affected by the different proportions of green light.
As shown in Figure 7 , the data of the three pigment parameters of lettuce leaves in different layers under G60 are the most compact in all treatments, whether from the difference between the maximum and minimum values or the height of the box. Employing green light, the difference between maximum and minimum values was decreased significantly, though the highest values of the three pigment parameters were observed under RB. These results suggested that green light reduced the gap of the concentrations of pigments between upper and lower leaves of plants, especially when green light accounts for 30% (G60) in the light source.
Leaf Photosynthetic Traits
P n−T under G30 decreased by 6% compared with that under RB, whereas no significant difference in P n−T was found among RB, G60, and G90. g s−T and Ci under treatments employing green light decreased significantly compared with those under RB ( Figure 8 ).

P n−T (A) , g s−T (B) , and Ci (C) under the actual light condition of lettuce leaves as affected by different proportions of green light. Different letters in each column indicate significant differences at p < 0.05, according to the least significant difference (LSD) test.
As shown in Figure 9 , P n−L and g s−L increased with the increasing light intensity. Among treatments employing green light, g s−L increased with an increase in the relative amount of green light. Under G30, g s−L was lower than that under RB, especially when light intensity was lower than 1,000 μmol m −2 s −1 , whereas g s−L under G60 and G90 was higher than that under RB. There was no obvious difference in P n−L among all treatments under the same light intensity ( Figure 9 ).

P n−L (A) and g s−L (B) to light intensity response curves as affected by the different proportions of green light.
Sucrose, Starch, and Nutritional Quality
As shown in Table 3 , an increase in the carbohydrate accumulation of lettuce leaves was found under treatments employing green light compared with that under RB. Sucrose and starch contents increased by 60 and 37% under G60 and by 35 and 40% under G90, respectively, compared with those under RB. In terms of nutritional quality, soluble sugar contents under G60 and G90 increased by 39 and 19%, respectively, compared with that under RB. Nitrate contents of lettuce leaves significantly decreased under treatments employing green light. Nitrate contents under G30, G60, and G90 decreased by 26, 40, and 43%, respectively, compared with that under RB. No significant differences in crude fiber contents were found among all treatments.
The accumulation of carbohydrate and nutritional quality as affected by different relative amount of green light.
Morphology of Lettuce Plants as Affected by Employing Green Light
Light quality, as energy and signal sources, significantly affects plant photomorphogenesis (Bantis et al., 2018 ). Similar to red/far-red (R/FR) ratio, the G/B ratio also could act as a shade signal within a plant canopy and could provide information about the degree of shading, triggering physiological and morphological changes of plants to best intercept available energy (Sellaro et al., 2010 ; Smith et al., 2017 ). In this experiment, lettuce stem length increased with increasing G/B ratio. This result was consistent with the finding that the hypocotyl length of Arabidopsis seedlings increased linearly with increasing G/B ratios within the range of 0.5–1.0 while cultivated in a natural environment (Sellaro et al., 2010 ). The above phenomenon can be explained by the shade avoidance syndrome induced by the green light. As shown in Figure 4 , the light transmittance in the green region was higher than that in the blue region so that an increase in G/B ratio was induced under the lettuce canopy, providing a shade signal for plants. The shade signal can be detected by relevant non-photosynthetic photoreceptors, and it can then induce the shade avoidance syndrome, such as stem elongation and leaf expansion, to intercept more available light energy (Wang and Folta, 2013 ). Kang et al. ( 2016 ) also found that green light increased the leaf length in lettuce plants. Moreover, leaf expansion was also found under G30 and G60 in this study. This was consistent with the finding that lettuce leaf area increased dramatically when green light replaced red and blue light (Kim et al., 2004 ). This kind of morphological changes caused by a high G/B ratio may be attributed to cryptochromes or an unknown role of phytochromes, or perhaps a novel light photoreceptor (Sellaro et al., 2010 ; Zhang et al., 2011 ). However, the leaf area under G90 showed no significant difference with RB. This may be caused by the low yield photon flux density (YPFD) in G90 (Johkan et al., 2012 ).
Gas Exchange of Lettuce Leaves as Affected by Employing Green Light
Stomatal conductance was mainly affected by stomatal density and the degree of stomatal opening (Fanourakis et al., 2015 ). Previous studies have shown that stomatal density in newly developed leaves of C3 plants is independent of their adjacent irradiance (Lake et al., 2001 ; Miyazawa et al., 2006 ). On the contrary, exposure of mature leaves to low light conditions triggered long-distance signaling that controls the stomatal development and caused a reduction in stomatal density in young leaves (Ehonen et al., 2020 ). This phenomenon is called systemic signaling (Lake et al., 2001 ). In this experiment, stomatal density increased with an increase in the relative amount of green light. This should be attributed to green light showing a higher light transmittance to plant canopy in comparison with red and blue light. Meanwhile, the local light intensity in mature leaves of relative lower layers increased with the increasing relative amount of green light. The above result was consistent with the finding that stomatal density increased significantly when blue LEDs were replaced with green LEDs in green leaf lettuce (Son and Oh, 2015 ).
Green light can reverse the stomatal openings stimulated by blue light (Frechilla et al., 2000 ), because the fully oxidized state of the flavin chromophore of cryptochrome in darkness is shifted to a semi-reduced form by blue light, and this semi-reduced form is converted to a reduced form particularly by absorbing green light (Liu et al., 2010 ). This was the reason why stomatal aperture decreased linearly with increasing G/B ratio in this study. This was also the main reason that caused lower g s−T under treatments employing green light than that under RB. However, the stomatal opening could be restored if the green light was followed or replaced by a second blue light in a response analogous to the R/FR reversibility of phytochrome responses (Frechilla et al., 2000 ; Taiz and Zeiger, 2015 ). In this research, the stomatal density of lettuce leaves caused by systemic signaling could not change immediately with the change of light quality; thus, under the same red and blue light condition, g s−L increased with an increase in the relative amount of green light. The above phenomenon gave an efficient way to regulate stomatal behaviors. In future experiments, green light can be considered to increase the stomatal density at the early growth stage of plant leaves, whereas blue light can be considered to increase the stomatal opening at the yield-forming stage of plants so that, especially in plant factories, plant yield can be increased efficiently. Previous studies also demonstrated that stomatal response to the light environment could provide potential targets for increasing photosynthesis, water use efficiency, and overall plant yield (Matthews et al., 2020 ).
Green Light Affected Pigmentation, Light Energy Distribution, and Photosynthetic Characteristics in Lettuce
Most of the previous studies mainly focused on the pigment contents of individual leaves rather than the whole plant. In this study, pigment concentrations of the lettuce leaves in different layers were measured to investigate the effect of green light on pigment concentrations of leaves within the canopy. The light absorptance of red and blue light was higher, but the light transmittance was lower in the upper leaves of lettuce. Thus, the relatively scattered pigment concentrations and Chl a/Chl b were found under RB. Compact distribution of pigment concentrations and Chl a/Chl b under G60 was mainly caused by the maximal light transmittance found under G60, which helped the pigmentation of lower layer leaves of lettuce plants in this study. This was beneficial for whole-plant photosynthesis. In comparison with G60, the lower pigment level of bottom leaves under G30 may be due to the relative lower green light intensity, limiting the irradiance to transmitting through the canopy, whereas under G90, they may be related to a stronger shade condition caused by a higher G/B ratio (Smith and Whitelam, 1997 ). These results suggested that a moderate amount of green light can promote the pigmentation of underlying leaves in the lower layer canopy.
Green light was capable of contributing light energy to photosynthesis both at the leaf level and the canopy level of plants (Smith et al., 2017 ). Up to 80% of green light was detected to pass through chloroplast, when 15–25% of green light was considered to transmit through the canopy of plants, efficiently promoting green light absorbed by chloroplasts either in the deeper mesophyll of a single leaf or lower leaves within the canopy, especially in plants with a folded structure, such as lettuce (Terashima et al., 2009 ). Relative lower light absorptance and higher light transmittance in the green region were also found in this experiment, in comparison with red and blue light. Moreover, higher light transmittance was observed in lettuce leaves under treatments employing green light compared with that under RB, especially in green regions. This result suggested that green light promoted more light energy to transmit through leaves. However, no significant increase in the light transmittance was found by using green light in Ocimum basilicum L. (Schenkels et al., 2020 ). This inconsistent phenomenon may be because the green light accounted for only 12% of the light source in this previous study.
Green light can be transmitted into deeper mesophyll of leaves and promote photosynthesis. In addition, once absorbed by chloroplasts, green light could drive photosynthesis with the close quantum efficiency of red and blue light (McCree, 1972 ; Smith et al., 2017 ). Thus, though the YPFD, which has been used to quantify the net radiation driving photosynthesis, of G60 and G90 was lower than that of RB, no significant differences of P n−T among RB, G60, and G90 were found in this research. However, P n−T under G30 was found to be lower than that under RB. This may be because the YPFD of G30 was lower than that of RB, and the relatively lower amount of green light was not enough to promote photosynthesis in the deep layer of leaves. The relatively lower light absorption found under G30 was also the cause of the lowest P n−T found under G30 in this study. When transferred to a similar red–blue light source, lettuce leaves of four treatments showed no differences on P n−L , though g s−L differed. This was mainly because g s was no longer the limiting factor for plant photosynthesis under high CO 2 levels.
Moderate Amount of Green Light Increased the Biomass in Lettuce
Plant dry mass accumulation was mainly determined by the photosynthetic efficiency of plant leaves and total leaf area of plants. The photosynthetic efficiency was primarily affected by the net photosynthetic rate of leaves. However, the net photosynthetic rate (P n ) under the growth environment did not support the growth results in this study. This phenomenon was also found in previous studies (Kim et al., 2004 ; Son and Oh, 2015 ). The possible explanation for this result was that the P n measured on a single leaf cannot represent the P n of the whole plant (Yorio et al., 2001 ; Li et al., 2020 ). Thus, whole-plant net assimilation was introduced in this study because whole-plant net assimilation has been used to evaluate the photosynthesis of the whole plant (Park and Runkle, 2018 ). In this study, the whole-plant net assimilation under G30 and G60 showed a nearly similar trend to the dry mass. This result may indicate that G30 and G60 had the ability to improve the whole-plant photosynthesis of lettuce plants. Moreover, the total leaf area of plants affects the light energy received by the plants, because the incident radiation interception increases with increasing the total leaf area (Bugbee, 2016 ; Snowden et al., 2016 ). The promotive effects of G30 (15% green light) and G60 (30% green light) on dry matter accumulated by lettuce leaves could be mainly attributed to the increase in leaf area caused by the shade avoidance syndrome, as described in this study. Kim et al. ( 2004 ) also found that fresh and dry weights of the lettuce shoot increased significantly when 24% of red and blue light was replaced with green light.
Moderate Amount of Green Light Increased the Concentration of Carbohydrate and Nutritional Quality in Lettuce
Carbohydrate is the final product of CO 2 absorption in the progress of plant photosynthesis (Taiz and Zeiger, 2015 ). In this experiment, the concentration of carbohydrates under the treatments employing green light was at a higher level than that of P n−T in the difference of statistical analysis. This result implicated replacing red and blue light with 15–45% of green light since the ability of plants to accumulate carbohydrates was stronger under green light than under red and blue light.
In general, higher soluble sugar concentration results in a desirable taste of the plant (Lin et al., 2013 ). In this study, a relatively higher soluble sugar concentration was found under G60. Chen et al. ( 2016 ) also found that supplementing green light to white LEDs increased the soluble sugar content and decreased the nitrate content in green oak leaf lettuce. However, vegetables with high nitrate concentrations are harmful to human health. Especially in China, the allowable highest nitrate concentration of leaf vegetables is 3.0 mg g −1 . In this study, the nitrate concentration of lettuce leaves could be significantly decreased by employing green light. Li et al. ( 2020 ) also found that partially replacing red and blue light with green light decreased the nitrate content in lettuce leaves. Bian et al. ( 2018 ) agreed with the above result that green light could decrease the nitrate contents in lettuce leaves by regulating the expression of some specific genes (e.g., NR and NiR ). Crude fiber, as an important component of a plant cell wall, provided the crisp taste of and nutrients in lettuce for human beings (Taiz and Zeiger, 2015 ). However, no significant influence of green light on lettuce crude fiber was found in this study. Results presented in this study suggested that a moderate amount of green light could enhance the nutritional quality of lettuce plants.
The optimal proportion of green light, partially replacing red and blue light, for promoting plant growth and development was investigated in this study. Our results showed that plant growth and morphology, light absorptance and transmittance, pigment concentration, stomatal characteristics, photosynthetic characteristics, carbohydrates, and nutritional quality were affected by different proportions of green light. The best green proportion was 30% in G60 in this study because G60 not only increased the leaf expansion and lettuce yield but also improved the accumulation of carbohydrate and nutritional quality. In addition, 15% green light in G30 and 45% green light in G90 also showed some advantages in lettuce growth. G30 increased the leaf expansion and yield and decreased the nitrate content of lettuce plants. G90 increased the accumulation of carbohydrates and decreased the nitrate content in lettuce plants, but no significant influence of G90 on lettuce yield was observed. Thus, from the perspective of economic benefits, the proportion of green light substituting red and blue light should be controlled between 15 and 30% in the production of lettuce, according to this experiment. The more accurate proportion still needs to be explored deeply in future studies.
Data Availability Statement
Author contributions.
LL carried out the measurements, data analysis, and drafted the manuscript. J-lL, Y-mL, and XL participated in part of measurements. Y-xT and R-fC made substantial guide about the experiment design and manuscript revision. All authors contributed to the article and approved the submitted version.
Conflict of Interest
The authors declare that the research was conducted in the absence of any commercial or financial relationships that could be construed as a potential conflict of interest.
Funding. This work was financially supported by the National Key Research and Development Program, Ministry of Science and Technology of China (No. 2020YFE0203600) and the Science and Technology Partnership Program, Ministry of Science and Technology of China (No. KY201702008).
Supplementary Material
The Supplementary Material for this article can be found online at: https://www.frontiersin.org/articles/10.3389/fpls.2021.627311/full#supplementary-material
Supplementary Figure 1
The diagram of the measuring apparatus.
- Bantis F., Smirnakou S., Ouzounis T., Koukounaras A., Ntagkas N., Radaglou K. (2018). Current status and recent achievements in the field of horticulture with the use of light-emitting diodes (LEDs) . Sci. Hortic. 235 , 437–451. 10.1016/j.scienta.2018.02.058 [ CrossRef ] [ Google Scholar ]
- Bian Z., Cheng R., Wang Y., Yang Q., Lu C. (2018). Effects of green light on nitrate reduction and edible quality of hydroponically grown lettuce ( Lactuca sativa L.) under short-term continuous light from red and blue light-emitting diodes . Environ. Expt. Bot. 153 , 63–71. 10.1016/j.envexpbot.2018.05.010 [ CrossRef ] [ Google Scholar ]
- Bouly J. P., Schleicher E., Dionisio-Sese M., Vandenbussche F., Straeten D. V. D., Bakrim N., et al.. (2007). Cryptochrome blue light photoreceptors are activated through interconversion of flavin redox states . J. Biol. Chem. 282 , 9383–9391. 10.1074/jbc.M609842200 [ PubMed ] [ CrossRef ] [ Google Scholar ]
- Bugbee B. (2016). Toward an optimal spectral quality for plant growth and development: the importance of radiation capture . Acta Hortic. 1134 , 1–12. 10.17660/ActaHortic.2016.1134.1 [ CrossRef ] [ Google Scholar ]
- Cataldo D. A., Maroon M., Schrader L. E., Youngs V. L. (1975). Rapid colorimetric determination of nitrate in plant tissue by nitration of salicylic acid 1 . Commun. Soil Sci Plant Anal. 6 , 71–80. 10.1080/00103627509366547 [ CrossRef ] [ Google Scholar ]
- Chen X., Xue X., Guo W., Wang L., Qiao X. (2016). Growth and nutritional properties of lettuce affected by mixed irradiation of white and supplemental light provided by light-emitting diode . Sci. Hort. 200 , 111–118. 10.1016/j.scienta.2016.01.007 [ CrossRef ] [ Google Scholar ]
- Clegg K. M. (1956). The application of the anthrone reagent to the estimation of starch in cereals . J. Sci. Food Agric. 7 , 40–44. 10.1002/jsfa.2740070108 [ CrossRef ] [ Google Scholar ]
- Ehonen S., Hölttä T., Kangasjärvi J. (2020). Systemic signaling in the regulation of stomatal conductance . Plant Physiol. 182 , 1829–1832. 10.1104/pp.19.01543 [ PMC free article ] [ PubMed ] [ CrossRef ] [ Google Scholar ]
- Fairbairn N. J. (1953). A modified anthrone reagent . Chem. Ind. 4 :86. [ Google Scholar ]
- Fanourakis D., Giday H., Milla R., Pieruschka R., Kjaer K. H., Vasilevski A., et al.. (2015). Pore size regulates operating stomatal conductance, while stomatal densities drive the partitioning of conductance between leaf sides . Ann. Bot. 115 , 555–565. 10.1093/aob/mcu247 [ PMC free article ] [ PubMed ] [ CrossRef ] [ Google Scholar ]
- Fils-Lycaon B., Julianus P., Chillet M., Galas C., Hubert O., Rinaldo D., et al.. (2011). Acid invertase as a serious candidate to control the balance source versus (glucose+fructose) of banana fruit during ripening . Sci. Hortic. 129 , 197–206. 10.1016/j.scienta.2011.03.029 [ CrossRef ] [ Google Scholar ]
- Frechilla S., Talbott L. D., Bogomolni R. A., Zeiger E. (2000). Reversal of blue light-stimulated stomatal opening by green light . Plant Cell Physiol. 41 , 171–176. 10.1093/pcp/41.2.171 [ PubMed ] [ CrossRef ] [ Google Scholar ]
- Johkan M., Shoji K., Goto F., Hahida S., Yoshihara T. (2012). Effect of green light wavelength and intensity on photomorphogenesis and photosynthesis in Lactuca sativa . Environ. Exp. Bot. 75 , 128–133. 10.1016/j.envexpbot.2011.08.010 [ CrossRef ] [ Google Scholar ]
- Kaiser E., Weerherm K., Schipper R., Dieleman J. A. (2019). Partial replacement of red and blue by green light increases biomass and yield in tomato . Sci. Hortic. 249 , 271–279. 10.1016/j.scienta.2019.02.005 [ CrossRef ] [ Google Scholar ]
- Kami C., Lorrain S., Hornitschek P., Fankhauser C. (2010). Light-regulated plant growth and development . Curr. Top. Dev. Biol. 91 , 29–66. 10.1016/S0070-2153(10)91002-8 [ PubMed ] [ CrossRef ] [ Google Scholar ]
- Kang W. H., Park J. S., Park K. S., Son J. E. (2016). Leaf photosynthetic rate, growth, and morphology of lettuce under different fractions of red, blue, and green light from light-emitting diodes (LEDs) . Hortic. Environ. Biotechnol. 57 , 573–579. 10.1007/s13580-016-0093-x [ CrossRef ] [ Google Scholar ]
- Kim H. H., Goins G. D., Wheeler R. M., Sager J. C. (2004). Green-light supplementation for enhanced lettuce growth under red- and blue-light-emitting diodes . Hort. Sci. 39 , 1617–1622. 10.21273/HORTSCI.39.7.1617 [ PubMed ] [ CrossRef ] [ Google Scholar ]
- Lake J. A., Quick W. P., Beerling D. J., Woodward F. I. (2001). Plant development: Signals from mature to new leaves . Nature 411 :154. 10.1038/35075660 [ PubMed ] [ CrossRef ] [ Google Scholar ]
- Li L., Tong Y., Lu J., Li Y., Yang Q. (2020). Lettuce growth, nutritional quality, and energy use efficiency as affected by red-blue light combined with different monochromatic wavelengths . Hort. Sci. 55 , 613–620. 10.21273/HORTSCI14671-19 [ CrossRef ] [ Google Scholar ]
- Lichtenthaler H. K., Wellburn A. R. (1983). Determination of total carotenoids and chlorophylls a and b of leaf extracts in different solvents . Biochem. Soc. Trans. 603 , 591–592. 10.1042/bst0110591 [ CrossRef ] [ Google Scholar ]
- Lin K., Huang M., Huang W., Hsu M., Yang Z., Yang C. (2013). The effects of red, blue, and white light-emitting diodes on the growth, development, and edible quality of hydroponically grown lettuce ( Lactuca sativa, L . var. capitata). Sci. Hortic. 150 , 86–91. 10.1016/j.scienta.2012.10.002 [ CrossRef ] [ Google Scholar ]
- Liu B., Liu H., Zhong D., Lin C. (2010). Searching for a photocycle of the cryptochrome photoreceptors . Curr. Opin. Plant Biol. 13 , 578–586. 10.1016/j.pbi.2010.09.005 [ PMC free article ] [ PubMed ] [ CrossRef ] [ Google Scholar ]
- Materová Z., Sobotka R., Zdvihalová B., Oravec M., Nezval J., Karlicky V., et al.. (2017). Monochromatic green light induces an aberrant accumulation of geranylgeranyled chlorophylls in plants . Plant Physiol. Biochem. 116 , 48–56. 10.1016/j.plaphy.2017.05.002 [ PubMed ] [ CrossRef ] [ Google Scholar ]
- Matthews J. S. A., Vialet-Chabrand S., Lawson T. (2020). Role of blue and red light in stomatal dynamic behavior . J. Exp. Bot. 71 , 2253–2269. 10.1093/jxb/erz563 [ PMC free article ] [ PubMed ] [ CrossRef ] [ Google Scholar ]
- McCree K. J. (1972). The action spectrum, absorptance and quantum yield of photosynthesis in crop plants . J. Agric. Meteorol. 9 , 191–216. 10.1016/0002-1571(71)90022-7 [ CrossRef ] [ Google Scholar ]
- Miyazawa S., Livingston N. J., Turpin D. H. (2006). Stomatal development in new leaves is related to the stomatal conductance of mature leaves in poplar ( Populus trichocarpa × P . deltoides) . J. Exp. Bot. 57 , 373–380. 10.1093/jxb/eri278 [ PubMed ] [ CrossRef ] [ Google Scholar ]
- Park Y., Runkle E. S. (2017). Far-red radiation promotes growth of seedlings by increasing leaf expansion and whole-plant net assimilation . Environ. Exp. Bot. 136 , 41–49. 10.1016/j.envexpbot.2016.12.013 [ CrossRef ] [ Google Scholar ]
- Park Y., Runkle E. S. (2018). Far-red radiation and photosynthetic photon flux density independently regulate seedling growth but interactively regulate flowering . Environ. Exp. Bot. 155 , 206–216. 10.1016/j.envexpbot.2018.06.033 [ CrossRef ] [ Google Scholar ]
- Sager J. C., Smith W. O., Edwards J. L., Cyr K. L. (1988). Photosynthetic efficiency and phytochrome photoequilibria determination using spectral data . T ASABE . 31 , 1882–1889. 10.13031/2013.30952 [ CrossRef ] [ Google Scholar ]
- Schenkels L., Saeys W., Lauwers A., Proft M. P. D. (2020). Green light induces shade avoidance to alter plant morphology and increases biomass production in Ocimum basilicum, L . Sci. Hortic. 261 :109002. 10.1016/j.scienta.2019.109002 [ CrossRef ] [ Google Scholar ]
- Sellaro R., Creyp M., Trupkin S. A., Karayekov E., Buchovsky A. S., Rossi C., et al.. (2010). Cryptochrome as a sensor of the blue/green ratio of natural radiation in arabidopsis 1[C] [W] [OA] . Plant Physiol. 154 , 401–409. 10.1104/pp.110.160820 [ PMC free article ] [ PubMed ] [ CrossRef ] [ Google Scholar ]
- Smith H., Whitelam G. C. (1997). The shade avoidance syndrome: multiple responses mediated by multiple phytochromes . Plant Cell Environ. 20 , 840–844. 10.1046/j.1365-3040.1997.d01-104.x [ CrossRef ] [ Google Scholar ]
- Smith H. L., McAusland L., Murchie E. H. (2017). Don't ignore the green light: Exploring diverse roles in plant processes . J. Exp. Bot. 68 , 2099–2110. 10.1093/jxb/erx098 [ PubMed ] [ CrossRef ] [ Google Scholar ]
- Snowden M. C., Cope K. R., Bugbee B. (2016). Sensitivity of seven diverse species to blue and green light: interactions with photon flux . PLoS ONE 11 :e0163121. 10.1371/journal.pone.0163121 [ PMC free article ] [ PubMed ] [ CrossRef ] [ Google Scholar ]
- Son K., Oh M. M. (2015). Growth, photosynthetic and antioxidant parameters of two lettuce cultivars as affected by red, green, and blue light-emitting diodes . Hortic. Environ. Biotechnol. 56 , 639–653. 10.1007/s13580-015-1064-3 [ CrossRef ] [ Google Scholar ]
- Sun J., Nishio J. N., Vogelmann T. C. (1998). Green light drives CO 2 fixation deep within leaves . Plant Cell Physiol. 39 , 1020–1026. 10.1093/oxfordjournals.pcp.a029298 [ CrossRef ] [ Google Scholar ]
- Taiz L., Zeiger E. (2015). Plant Physiology, 5th Edn . Sunderland, MA: Sinauer Assoc Inc. [ Google Scholar ]
- Terashima I., Fujita T., Inoue T., Chow W. S., Oguchi R. (2009). Green light drives leaf photosynthesis more efficiently than red light in strong white light: revisiting the enigmatic question of why leaves are green . Plant Cell Physiol. 50 , 684–697. 10.1093/pcp/pcp034 [ PubMed ] [ CrossRef ] [ Google Scholar ]
- Wang J., Lu W., Tong Y., Yang Q. (2016). Leaf morphology, photosynthetic performance, chlorophyll fluorescence, stomatal development of lettuce ( Lactuca sativa L.) exposed to different ratios of red light to blue light . Front. Plant Sci. 7 :250. 10.3389/fpls.2016.00250 [ PMC free article ] [ PubMed ] [ CrossRef ] [ Google Scholar ]
- Wang Y., Folta K. M. (2013). Contributions of green light to plant growth and development . Am. J. Bot. 100 , 70–78. 10.3732/ajb.1200354 [ PubMed ] [ CrossRef ] [ Google Scholar ]
- Yorio N. C., Goin G. D., Kagie H. R., Wheeler R. M., Sager J. C. (2001). Improving spinach, radish, and lettuce growth under red light-emitting diodes (LEDs) with blue light supplementation . Hort. Sci. 36 , 380–383. 10.21273/HORTSCI.36.2.380 [ PubMed ] [ CrossRef ] [ Google Scholar ]
- Zadoks J. C., Chang T. T., Konzak C. F. (1974). A decimal code for the growth stages of cereals . Weed Res. 14 , 415–421. 10.1111/j.1365-3180.1974.tb01084.x [ CrossRef ] [ Google Scholar ]
- Zhang T., Maruhnich S. A., Folta K. M. (2011). Green light induces shade avoidance synmptoms 1[C] [W] [OA] . Plant Physiol. 157 , 1528–1536. 10.1104/pp.111.180661 [ PMC free article ] [ PubMed ] [ CrossRef ] [ Google Scholar ]
Academia.edu no longer supports Internet Explorer.
To browse Academia.edu and the wider internet faster and more securely, please take a few seconds to upgrade your browser .
Enter the email address you signed up with and we'll email you a reset link.
- We're Hiring!
- Help Center
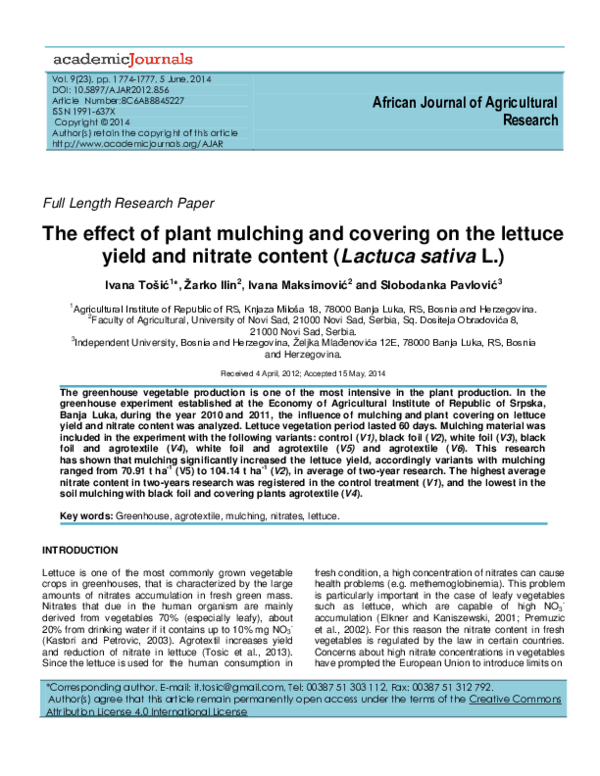
The effect of plant mulching and covering on the lettuce yield and nitrate content (Lactuca sativa L.)

2014, African Journal of Agricultural Research
Related Papers
Agriculture Journal IJOEAR , Milena Yordanova
— The aim of the paper was to present the influence of plant density and different types of organic mulch on growth and yield of Romain lettuce. The field experiment was carried out in 2014 in the experimental field of University of Forestry – Sofia, with Romaine lettuce cv. Yellow beauty. The seedlings were planted at a distance of 30 x 30 cm and two different schemes were tested: parallel planting to form a 4-row bed and a chess planting to form a 7-row bed. For the purpose of the study were used different organic mulches, which were waste products from organic agriculture: barley straw (BS), well-rotted horse manure (HM), walnut wood sawdust (WS) and grass windrow (GW) and non-mulched control plots (NMC). The mulching materials were spread manually in a 5-6 cm thick layer, after strengthen the seedlings of Romain lettuce. On the 60th day after planting was the beginning of harvesting. It was found out that the seven-row bed and mulching influenced growth and yield of Romain lettuce. Mulching with HM has a significant effect on growth and yield. In the cultivation of lettuce in combination with horse manure mulch and seven-row bed, the yield increased by 18% compared to the non-mulched control in the same planting scheme and was 2.3 times higher than the non-mulched control in a four-row bed. A significant effect on the mulched variants was established. Keywords— grass windrow mulch, horse manure mulch, lettuce, seven-row bed, yield.
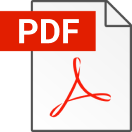
IJMRAP Editor
The study entitled "The Influence of Different Mulching Materials to Soil Physical Properties and on the Growth and Development of Lettuce (Lactuca sativa L.
International Journal of Scientific Research and Management
derajew68 asres
Lettuce (Lactuca sativa L.) is one of the most popular salad crops and occupies the largest production area among salad crops in the world. However, production of lettuce can be limited due to lack of improved production techniques. Farmers in northwestern Ethiopia didn’t apply mulching for lettuce. A field study was conducted at Teda in 2018 to evaluate the effect of different mulching materials on yield and yield component of lettuce (Lactuca sativa L.) under irrigation. Five mulching materials used as a treatment viz: white plastic, black plastic, teff straw, chickpea straw and control (without mulch). The treatments were laid out in Randomized Complete Block Design (RCBD) with four replications. Application of mulching increased leaf width, leaf number, plant height, biological yield, root volume, dry matter and plant height. The highest and lowest yield was recorded for black plastic mulch and no mulch, respectively. The study revealed that the black plastic is the best mulchin...
Zenodo (CERN European Organization for Nuclear Research)
Thelma Ntow
Milena Yordanova
The aim of the paper was to present the influence of plant density and different types of organic mulch on weed infestation and yield of Romain lettuce. The field experiment was carried out in 2014 in the experimental field of University of Forestry – Sofia, with Romaine lettuce cv. Yellow beauty. The seedlings were planted at a distance of 30 x 30 cm and two different schemes were tested: parallel planting to form a 4-row bed and a chess planting to form a 7-row bed. For the purpose of the study were used different organic mulches, which were waste products from organic agriculture: barley straw (BS), well-rotted horse manure (HM), walnut wood sawdust (WS) and grass windrow (GW). Mulched plots were compared with non-mulched control plots (NMC). The mulching materials were spread manually in a 5-6 cm thick layer, after strengthen the seedlings of Romain lettuce. On the 40 th , day after mulching were recorded the number of weeds on each plot. It was found out that mulching with BSM ...
Agriculture Journal IJOEAR
An experiment was conducted to evaluate the effectiveness of different mulching materials on soil moisture retention and yield of lettuce at the greenhouse located at Luyengo campus of the University of Eswatini during the months of January and February, 2019. The treatments consisted of grass mulch (GM), Plastic mulch (PM), leaf debris mulch (LM), and no mulch (NM) which was used as a control. Each of the treatments had four replications. The organic mulch was applied at a thickness of 10 cm, and the plots for experiments were randomly selected. Each plant received 600 cm 3 of water every 3 days using a homemade drip irrigation system (equivalent to 6 mm per irrigation circle). Data on soil moisture content was collected using the gravimetric method every 3 days (before irrigation).The growth parameters of the lettuce plants that were collected weekly were plant height, leaf number and leaf area. Both wet weight and dry weight yield were determined for each plot at the end of the experiment (six weeks after planting). Data collected was coded and entered into SPSS computer software. Data analysis was conducted using the analysis of variance (ANOVA) and the least significance difference (LSD) test to determine if means were significantly different. The results showed that GM treatment had high mean moisture retention at 9.3%. It was followed by PM and LM at 8.9%. The lowest moisture retention was realized from the control (No mulching) at 7.9%.The differences in mean moisture retention was significant between NM and NM (p<0.05). The same pattern was observed for the growth parameters, where GN had highest values and the control had the lowest values. The wet mass yield was highest for GM, at 164.7 g. The yield from LM was 149.3 g. It was followed by PM at 141.3 g. The lowest yield was obtained from the control at 108 g. The difference in mean yields for GM and NM were significant (p<0.05). They were not significantly different for all the other treatments (p>0.050. It was concluded from the experiment that grass mulching resulted in improved moisture retention and high yields.
Scientific Research Publishing
Lettuce (Lactuca sativa L.) is one of the leading vegetables produced by farmers in the Itabaiana region, and thus it is important to study means of sustainable management for the crop. The objective of this work was to evaluate the behavior of lettuce with different sources of organic matter and soil cover. Therefore, it was chosen to cultivate Veronica. The experiment was carried out in the experimental field plot of Project Small Farmer—Great Entrepreneur in the municipality of Itabaiana-SE, in 2010. The design was a randomized block design with four replications, with treatments consisting of five sources of organic matter (organic compost, chicken manure, cattle manure, sheep manure, and castor bean) and a control (no fertilizer), all with and without mulch, totaling 12 treatments. We evaluated the characteristics of plant diameter, plant height, fresh and dry root and shoot matter. We also evaluated the influence on the temperature and soil water tension. All productivity characteristics valuated showed the significant interaction between the type of fertilizer and the mulch, except for root dry weight, where only organic manure was a significant factor. When soil mulch was used, the best source of organic matter for growing lettuce was chicken manure, and in the impossibility of using this cover, we recommend the use of organic compost as a source of organic matter, as it showed the best result in the absence of coverage. The cover age also showed good results in relation to a temperature that promoted lower soil water tensions.
Research, Society and Development
Denise Pedrinho
It was evaluated the effects of different types of mulching of soil in the cultivation of crisphead lettuce (Lactuca sativa L.), cultivar “Lucy Brown”, in the spring period, on weed control, soil temperature and productivity. The experiment was arranged in a randomized block design with four treatments and five replications in plots of 14.0m2. The following coverages were studied: Pennisetum glaucum L., Avena strigosa, Brachiaria ruziziensis, plus the control treatment (soil without mulching). The use of mulching of soil, P. glaucum and B. ruziziensis, proved to be a beneficial practice, reducing the soil temperature by up to 5ºC. There was a lower incidence of weeds with the use of the P. glaucum and A. strigosa coverages. The use of mulch did not provide a significant gain in productivity, however, there was a gain regarding the quality of the product, since the coverage with P. glaucum provided an increase in the size of the head (fresh mass of the commercial head).
Indian Journal Of Agricultural Research
Endang Purbajanti
The objective of this research is to assess the growth and physiological characteristics of lettuce in the delivery of different N-organics. Research was conducted at Faculty of Animal and Agricultural Sciences, Diponegoro University, Indonesia. The materials used were seeds of lettuce (Lettuce sativa L) on Oxisol soil types, with the total N content of 0.18 %, P (18 mg. g-1), K (23 mg. g-1) and C (1.52 %) were utilized. The organic fertilizers applied were cow-, sheep-, and guano manures, and compost and green manure (Leucaena leucocephala). The experimental design was a randomized block design with four replications and five treatments. The treatment dose of fertilizer applied is equivalent to 100 kg N / ha, namely: cow-manure ; sheep-manure; guano manure ; compost and green manure. Green manure, set into the ground is Leucaena leucocephala. The variables measured were the number of leaves, leaf area, leaf area index(LAI), the rate of photosynthesis, Chlorophyll total, anti-oxidan...
Journal of Ornamental plants
edmar franquera
Mulches bring several benefits to lettuce cultivation.A study on the lettuce ‘Red Rapids’ has been conducted to determine the influence of the different colored plastic mulch on its growth. The experiment consisted of five color treatments as follows; treatment 1- metallic silver (control), treatment 2- red, treatment 3- orange, treatment 4- yellow and treatment 5green. Results showed that the colored mulch treatments had significant influenced on the different parameters including: root length at harvest, average leaf length, leaf diameter, plant height, plant weight, number of leaves at harvest and percentage survival. Lettuce grown in red mulch had significantly outperformed the other colored treatments.
RELATED PAPERS
Wayan Deka Cita Santi
Peter Pomozi
Proceedings of the Third Arabic Natural Language Processing Workshop
Hanady Ahmed
Journal of Experimental & Clinical Medicine
Ayhan Dağdemir
Bosnian journal of basic medical sciences / Udruženje basičnih mediciniskih znanosti = Association of Basic Medical Sciences
Mirsada Hukić
Desidoc Journal of Library Information Technology
Fayaz A Loan
Indah Pratiwi
Journal of Environmental Science and Health . Part A: Environmental Science and Engineering and Toxicology
Fayaz Lakhwala
Revista Brasileira de Pesquisa (Auto)Biográfica
elizeu souza
TEXTEH Proceedings
andrej cupar
Dental Research and Management
Ali Nawaz Nawaz
Journal of Agrarian Change
Andrew Flachs
Journal of Oncology
Livia Onofrio
Indonesian Journal for Social Responsibility
Revista De Estudios Sociales
Francisco GDF Leal SGA
Proceedings of the workshop on Human Language Technology - HLT '94
Mitchel Weintraub
Journal of Pharmaceutical Sciences
A.Reza Fassihi
Free Radical Biology and Medicine
Shannon Bailey
Metabolism Open
Dimiter Avtanski
Economics of Transportation
Philip Gayle
Jurnal JTIK (Jurnal Teknologi Informasi dan Komunikasi)
Hery Supiarza
International Journal of Scientific Research in Computer Science, Engineering and Information Technology
V. Rakesh, D. Abhishek, O. Earni Sai, Y. S. H. S. Rohit, R. Venkata Ramana , International Journal of Scientific Research in Computer Science, Engineering and Information Technology IJSRCSEIT
WENDEL ERNESTO LEON ALVARADO
Journal of Thermal Analysis and Calorimetry
Farzin Arianpour
- We're Hiring!
- Help Center
- Find new research papers in:
- Health Sciences
- Earth Sciences
- Cognitive Science
- Mathematics
- Computer Science
- Academia ©2024
Lettuce ( Lactuca sativa L.)
Cite this chapter.
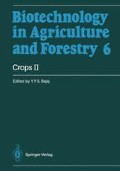
- R. Alconero 2
Part of the book series: Biotechnology in Agriculture and Forestry ((AGRICULTURE,volume 6))
723 Accesses
7 Citations
7 Altmetric
Lettuce ( Lactuca sativa L.) is a domestic annual species in the family Asteraceae (Compositae) cultivated mainly for its fleshy leaves. It forms within the genus Lactuca a somewhat isolated genetic compatibility group with three wild species that also have nine pairs of chromosomes, L. serriola, L. saligna and L. virosa (Thompson et al. 1941; Thompson 1943). Its relationship with L. serriola appears to be very close, having morphologically identical chromosomes that may be largely homologous and with no apparent obstacles to gene flow between them. There are marked sterility barriers between L. saligna on one side and L. serriola and L. sativa on the other, but gene exchange is possible. The genetic relationship with L. virosa is more distant. There are clear differences between it and the three other species in chromosome morphology, and gene exchange is very difficult (Lindqvist 1960b).
This is a preview of subscription content, log in via an institution to check access.
Access this chapter
Institutional subscriptions
Unable to display preview. Download preview PDF.
Abawi GS, Robinson RW, Cobb AC, Shail JW (1980) Reaction of lettuce germplasm to artificial inoculation with Sclerotinia minor under greenhouse conditions. Plant Dis 64:668–671
Article Google Scholar
Alconero R (1983) Regeneration of plants from cell suspension of Lactuca saligna, Lactuca sativa , and Lactuca serriola . HortSci 18:305–307
Google Scholar
Bannerot H, Boulidard L, Marrou J, Dutiel M (1969) Etude de l’hérédité de la tolérance au virus de la mosaïque de la laitue chez la varieté Gallega de envierno. Ann Phytopathol 1:219–226
Bensink J (1958) Heading of lettuce (Lactuca sativa L.) as a morphogenetic effect of leaf growth. Proc 15th Int Hortic Congr, Nice, pp 470–475
Berry SF, Lu DY, Pental D, Cocking EC (1982) Regeneration of plants from protoplasts of Lactuca sativa L. Z Pflanzenphysiol 108:31–38
Bohn GW, Whitaker TW (1951) Recently introduced varieties of head lettuce and methods used in their development. US Dep Agric (USDA) Circular 881
Bremer AH, Grana J (1935) Genetische Untersuchungen mit Salat II. Gartenbauwissenschaft 9:231–245
Cox EF, Mee JMT (1976) A comparison of tipburn susceptibility in lettuce under field and glasshouse conditions. J Hortic Sci 51:117–122
Crute IR (1984) The integrated use of genetic and chemical methods for control of lettuce downy mildew ( Bremia lactucae Regel). Crop Protect 3:223–241
Crute IR, Davis AA (1977) Specificity of Bremia lactucae from Lactuca sativa . Trans Br Mycol Soc 69:405–410
Crute IR, Johnson AG (1976) The genetic relationship between races of Bremia lactucae and cultivars of Lactuca sativa . Ann Appi Biol 83:125–137
Dickson MH (1963) Resistance to corky root rot in head lettuce. Proc Am Soc Hortic Sci 82:388–390
Doerschung MR, Miller CO (1967) Chemical control of adventitious organ formation in Lactuca sativa expiants. Am J Bot 54:410–413
Dunn JA (1960) Varietal resistance of lettuce to attack by the lettuce root aphid, Pemphigus bursarius (L). Ann Appi Biol 48:764–770
Durst CE (1930) Inheritance in lettuce. Ill Agric Exp Stn Bull 356
Eenink AH (1974) Resistance in Lactuca against Bremia lactucae Regel. Euphytica 23:411–416
Eenink AH (1976) Breeding research on lettuce in the Netherlands. Proc Eucarpia Meet Leafy vegetables, pp 78–83
Eenink AH, Dieleman FL (1977) Screening Lactuca for resistance to Myzus persicae . Neth J Plant Pathol 83:139–151
Eenink AH, Groenwold R, Dieleman FL (1982) Resistance of lettuce ( Lactuca ) to the leaf aphid Nasonovia ribis nigri . 1. Transfer of resistance from L. virosa to L. sativa by interspecific crosses and selection of resistant breeding lines. Euphytica 31:291–299
Engler DE, Grogan RG (1983) Isolation, culture and regeneration of lettuce leaf mesophyll protoplasts. Plant Sci Lett 28:223–229
Engler DE, Grogan RG (1984) Variation in lettuce plants regenerated from protoplasts. J Hered 75:426–430
FAO (ed) (1985) FAO production yearbook Files. FAO, Rome
Ferakova V (1977) The genus Lactuca L. in Europe. Univ Komenskeho, Bratislava, 122 pp
Gamborg OL, Miller RA, Ojima K (1968) Nutrient requirements of suspension culture of soybean root cells. Exp Cell Res 50:151–158
Article PubMed CAS Google Scholar
Globerson D, Netzer D, Sachs J (1980) Wild lettuce as a source for improving cultivated lettuce. Proc Eucarpia Meet Leafy vegetables, pp 86–96
Hall CB, Stall RE, Burdine HW (1971) Association of Pseudomonas marginalis with pink rib of lettuce. Proc Fla State Hortic Soc 84:163–165
Jagger IC, Whitaker TW, Uselman J J, Owen WM (1941) The imperial strains of lettuce. US Dep Agric (USDA) Circular 596
Kadkade P, O’Connor H J (1976) Interactive effects of growth regulators on organogenesis in lettuce tissue culture. Plant Physiol 57:75
Kadkade P, Siebert M (1977) Phytoehrome-regulated organogenesis in lettuce tissué culture. Nature (London) 270:49–50
Kao KN (1977) Chromosomal behaviour in somatic hybrids of soybean- Nicotiana glauca . Mol Gen Genet 150:225–230
Kishaba AN, Whitaker TW, Vail PV, Toba HH (1973) Differential oviposition of cabbage loopers on lettuce. J Am Soc Hortici 98:367–370
Koevary K, Rappaport L, Morris LL (1978) Tissue culture propagation of head lettuce. Hort Sci 13:39–41
Lindqvist K (1960a) On the origin of cultivated lettuce. Hereditas 46:319–350
Lindqvist K (1960b) Inheritance studies in lettuce. Hereditas 46:387–470
Maxon Smith JW (1965) Breeding improved winter lettuce: a survey of possible parents. Exp Hortic 12:21–31
Maxon Smith JW (1977) Recurring off-types in lettuce: their significance in plant breeding and seed production. Theor Appl Genet 50:79–87
Maxon Smith JW (1980) Lettuce breeding at glasshouse. Crops Res Inst Proc Eucarpia Meet Leafy vegetables, pp 30–35
McCollum GD (1953) Cytogenetic relationships of Lactuca serriola and L. sativa . MS Thesis, Washington State Univ
Morris LL, Kader AA, Klaustermeyer JA, Cheyney CC (1978) Avoiding ethylene concentrations in harvested lettuce. Cal Agricic 32:14–15
CAS Google Scholar
Murashige T, Skoog F (1962) A revised medium for the rapid growth and bioassays with tobacco tissue cultures. Physiol Plant 16:473–497
Netherlands Institute for Horticultural Plant Breeding (ed) (1985) Annu Rep 1984, Wageningen
Newton HC, Sequeira L (1972) Possible sources of resistance in lettuce to Sclerotinia sclerotiorum . Plant Dis Rep 56:875–878
Norwood JM, Crute IR (1985) A comparison of the susceptibility of lettuce cultivars to natural field and artificially induced laboratory infection with downy mildew, Bremia lactucae . Z Pflanzenziicht 95:63–73
Norwood JM, Crute IR, Lebeda A (1981) The location and characteristics of novel sources of resistance to Bremia lactucae Regel (Powdery Mildew) in wild Lactuca L. species. Euphytica 30:659–668
Nothmann J, (1976) Morphology of head formation of cos lettuce ( Lactuca sativa L. cv. Romana) 1. The process of hearting. Ann Bot (London) 40:1067–1072
Pearson OH (1956) The nature of the rogue in 456 lettuce. Proc Am Soc Hortic Sci 68:220–278
Phalen A von der, Crnko J (1965) El virus del mosaico de la lechuga ( Marmor lactucae Holmes) en Mendoza y Buenos Aires. Rev Invest Agr B Aires Ser 52:25–31
Prowidenti R, Robinson RW, Shail JW (1980) A source of resistance to a strain of cucumber mosaic virus in Lactuca saligna L. Horci 15:528–529
Prowidenti R, Robinson RW, Shail JW (1984) Incidence of broad bean wilt virus in lettuce in New York State and sources of resistance. HortSci 19:569–570
Reinert RA, Tingey DT, Carter HB (1972) Ozone induced foliar injury in lettuce and radish cultivars. J Am Soc Hortici 97:711–714
Robinson RW, Mreight JO, Ryder EJ (1983) The genes of lettuce and closely related species. Plant Breed Rev 1:267–293
Ryder EJ (1979a) Leafy salad vegetables. AVI, Westport, Conn
Ryder EJ (1979b) Vanguard 75 lettuce. Hort Sci 14:284–286
Ryder EJ (1979c) Effects of big vein resistance and temperature on disease incidence and percentage of plants harvested of crisphead lettuce. J Am Soc Hortic Sci 104:665–668
Ryder EJ (1980) Lettuce breeding in the United States: A short history. Proc Eucarpia Meet Leafy vegetables, pp 74–77
Sasaki H (1974) Physiological and morphological studies on development of vegetable crops: Organ formation of lettuce tissue cultured in vitro. J Hokkaido Univ Educ Sect IIB 26:17–27
Sasaki H (1979) Physiological and morphological studies on development of vegetable crops. VI. Effect of several auxins cytokinins and cytokinin ribosides on the adventitious bud formation of lettuce hypocotyl tissue cultured in vitro. J Jpn Soc Hortic Sci 48:67–72
Article CAS Google Scholar
Sequeira L (1970) Resistance to corky root rot of lettuce. Plant Dis Rep 54:754–758
Shannon MC, Mreight JD (1984) Salt tolerance of lettuce introductions. HortSci 19:673–675
Sibi M (1976) La notion de programme génétique chez les végétaux supérieurs II Aspect expérimental: obtention de variants par culture de tissue in vitro sur Lactuca sativa L.; apparition de vigueur chez les croisements. Ann Amelior Plantes 26:523–547
Sibi M (1984) Heredity of epigenetic-variant plants from culture in vitro. In: Lange WA, Zeven AC, Hagenboom NE (eds) Efficiency in plant breeding. Pudoc, Wageningen, pp 196–198
Smeets L (1977) Analysis of the differences in growth between five lettuce cultivars marking the development in lettuce breeding for winter production. Euphytica 26:655–659
Thompson RC (1943) Further studies on interspecific genetic relationships in Lactuca . J Agric Res 66:41–48
Thompson RC (1944) Lettuce varieties and culture. USDA Farmers Bull 1953
Thompson RC, Ryder EJ (1961) Description and pedigrees of nine varieties of lettuce. USDA Tech Bull 1244
Thompson RC, Whitaker TW, Kosar WF (1941) Interspecific genetic relationships in Lactuca . J Agric Res 63:91–107
United Kingdom Ministry of Agriculture, Fisheries and Food (ed) (1984) Output and utilization of farm produce in the United Kingdom 1977 to 1983
US Department of Agriculture — USDA (ed) (1984) USDA agricultural statistics. US Gov Print Off, Washington, DC
Webb DF, Torres LD, Fobert P (1984) Interactions of growth regulators, expiant age, and culture environment controlling organogenesis from lettuce cotyledons in vitro. Can J Bot 62:586–590
Whitaker TW (1969) Salads for everyone — a look at the lettuce plant. Econ Bot 23:261–264
Whitaker TW, McCollum GD (1954) Shattering in lettuce — Its inheritance and biological significance. Bull Torrey Bot Club 81:104–110
Whithaker TW, Kishaba AN, Toba HH (1974) Host-parasite interrelatons of Lactuca saligna L. and the cabbage looper, Trichoplusia ni (Hubner). J Am Soc Hortic Sci 99:74–78
White PR (1943) Handbook of plant tissue culture. Cattell, Lancaster, Penn
Book Google Scholar
Wurr DCE, Fellows JR (1984) The growth of three crisp lettuce varieties from different sowing dates. J Agric Sci Cambridge 102:733–745
Zink FW, Duffus JE (1972) Association of beet western yellows and lettuce mosaic viruses with internal rib necrosis of lettuce. Phytopathology 62:1141–1144
Zitter TA, Guzman VL (1974) Incidence of lettuce mosaic and bidens mottle viruses in lettuce and scarole fields in Florida. Plant Dis Rep 58:1087–1091
Download references
Author information
Authors and affiliations.
U.S. Department of Agriculture, New York State Agricultural Experiment Station, ARS, Plant Germplasm Resources, 14456, Geneva, NY, USA
R. Alconero
You can also search for this author in PubMed Google Scholar
Editor information
Editors and affiliations.
New Friends Colony, A-137, 110065, New Delhi, India
Y. P. S. Bajaj
Rights and permissions
Reprints and permissions
Copyright information
© 1988 Springer-Verlag Berlin Heidelberg
About this chapter
Alconero, R. (1988). Lettuce ( Lactuca sativa L.). In: Bajaj, Y.P.S. (eds) Crops II. Biotechnology in Agriculture and Forestry, vol 6. Springer, Berlin, Heidelberg. https://doi.org/10.1007/978-3-642-73520-2_17
Download citation
DOI : https://doi.org/10.1007/978-3-642-73520-2_17
Publisher Name : Springer, Berlin, Heidelberg
Print ISBN : 978-3-642-73522-6
Online ISBN : 978-3-642-73520-2
eBook Packages : Springer Book Archive
Share this chapter
Anyone you share the following link with will be able to read this content:
Sorry, a shareable link is not currently available for this article.
Provided by the Springer Nature SharedIt content-sharing initiative
- Publish with us
Policies and ethics
- Find a journal
- Track your research
Click through the PLOS taxonomy to find articles in your field.
For more information about PLOS Subject Areas, click here .
Loading metrics
Open Access
Peer-reviewed
Research Article
Lettuce ( Lactuca sativa ) productivity influenced by microbial inocula under nitrogen-limited conditions in aquaponics
Contributed equally to this work with: Jessica A. Day, Christian Diener, Anne E. Otwell
Roles Conceptualization, Formal analysis, Investigation, Methodology, Project administration, Supervision, Writing – original draft, Writing – review & editing
Affiliation Institute for Systems Biology, Seattle, Washington, United States of America
Roles Formal analysis, Investigation, Methodology, Software, Visualization, Writing – original draft, Writing – review & editing

Roles Conceptualization, Investigation, Methodology, Writing – original draft, Writing – review & editing
Roles Investigation, Writing – review & editing
Affiliation St. Edward’s University, Environmental Science and Policy, Behavioral and Social Sciences, Austin, Texas, United States of America
Roles Formal analysis, Funding acquisition, Investigation, Methodology, Resources, Supervision, Writing – original draft, Writing – review & editing
Affiliation National Aeronautics and Space Administration, Exobiology Branch, NASA Ames Research Center, Mountain View, California, United States of America
Roles Investigation, Methodology, Resources, Writing – review & editing
Affiliations National Aeronautics and Space Administration, Exobiology Branch, NASA Ames Research Center, Mountain View, California, United States of America, Bay Area Environmental Research Institute, Moffett Field, California, United States of America
Roles Formal analysis, Investigation, Methodology, Software, Writing – original draft, Writing – review & editing
Affiliations National Aeronautics and Space Administration, Exobiology Branch, NASA Ames Research Center, Mountain View, California, United States of America, Blue Marble Space Institute of Science, Seattle, Washington, United States of America
Roles Investigation
Affiliation Seattle Youth Employment Program, Seattle, Washington, United States of America
Roles Conceptualization, Formal analysis, Investigation, Methodology, Software, Visualization, Writing – original draft, Writing – review & editing
* E-mail: [email protected] (SMG); [email protected] (NSB)
Affiliations Institute for Systems Biology, Seattle, Washington, United States of America, eScience Institute, University of Washington, Seattle, Washington, United States of America, Department of Bioengineering, University of Washington, Seattle, Washington, United States of America
Roles Conceptualization, Funding acquisition, Investigation, Methodology, Project administration, Resources, Supervision, Writing – original draft, Writing – review & editing
Affiliations Institute for Systems Biology, Seattle, Washington, United States of America, Department of Microbiology, University of Washington, Seattle, Washington, United States of America, Department of Biology, University of Washington, Seattle, Washington, United States of America, Molecular Engineering and Sciences, University of Washington, Seattle, Washington, United States of America, Lawrence Berkeley National Laboratories, Berkeley, California, United States of America
- Jessica A. Day,
- Christian Diener,
- Anne E. Otwell,
- Kourtney E. Tams,
- Brad Bebout,
- Angela M. Detweiler,
- Michael D. Lee,
- Madeline T. Scott,
- Wilson Ta,
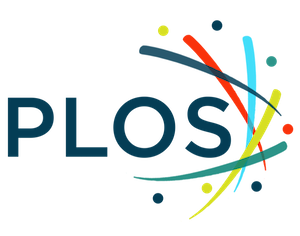
- Published: February 23, 2021
- https://doi.org/10.1371/journal.pone.0247534
- Peer Review
- Reader Comments
The demand for food will outpace productivity of conventional agriculture due to projected growth of the human population, concomitant with shrinkage of arable land, increasing scarcity of freshwater, and a rapidly changing climate. While aquaponics has potential to sustainably supplement food production with minimal environmental impact, there is a need to better characterize the complex interplay between the various components (fish, plant, microbiome) of these systems to optimize scale up and productivity. Here, we investigated how the commonly-implemented practice of continued microbial community transfer from pre-existing systems might promote or impede productivity of aquaponics. Specifically, we monitored plant growth phenotypes, water chemistry, and microbiome composition of rhizospheres, biofilters, and fish feces over 61-days of lettuce ( Lactuca sativa var . crispa) growth in nitrogen-limited aquaponic systems inoculated with bacteria that were either commercially sourced or originating from a pre-existing aquaponic system. Lettuce above- and below-ground growth were significantly reduced across replicates treated with a pre-existing aquaponic system inoculum when compared to replicates treated with a commercial inoculum. Reduced productivity was associated with enrichment in specific bacterial genera in plant roots, including Pseudomonas , following inoculum transfer from pre-existing systems. Increased productivity was associated with enrichment of nitrogen-fixing Rahnella in roots of plants treated with the commercial inoculum. Thus, we show that inoculation from a pre-existing system, rather than from a commercial inoculum, is associated with lower yields. Further work will be necessary to test the putative mechanisms involved.
Citation: Day JA, Diener C, Otwell AE, Tams KE, Bebout B, Detweiler AM, et al. (2021) Lettuce ( Lactuca sativa ) productivity influenced by microbial inocula under nitrogen-limited conditions in aquaponics. PLoS ONE 16(2): e0247534. https://doi.org/10.1371/journal.pone.0247534
Editor: Zhili He, Sun Yat-Sen University, CHINA
Received: September 15, 2020; Accepted: February 8, 2021; Published: February 23, 2021
This is an open access article, free of all copyright, and may be freely reproduced, distributed, transmitted, modified, built upon, or otherwise used by anyone for any lawful purpose. The work is made available under the Creative Commons CC0 public domain dedication.
Data Availability: R notebooks and scripts to reproduce the analysis starting from the raw FASTQ files are provided at https://github.com/gibbons-lab/aquaponics . The analysis of the validation samples can be found in the same repository. More complex algorithms such as the Expectation-Maximization algorithm are available in a dedicated R package along with documentation at https://github.com/gibbons-lab/mbtools . Raw sequencing data (study and validation data) has been deposited in NCBI Sequence Read Archive (SRA) under the project ID PRJNA641448.
Funding: This research was possible due to the support and resources provided by Ray Williams and the Black Farmers Collective, Jeff King and the Microsoft Giving Campaign, Fred Hutchinson Cancer Research Center, Seattle Youth Employment Program, CrowdRise donors, the National Science Foundation (NSF MSB-1237267, MCB-1616955, MCB-1518261, DB-1262637, DB-1565166, MCB-1330912), a Washington Research Foundation Distinguished Investigator Award (supporting CD and SMG), and a Scientific Innovation Fund grant from the NASA Office of the Chief Scientist to Brad M. Bebout. The funders had no role in study design, data collection and analysis, decision to publish, or preparation of the manuscript.
Competing interests: The authors have declared that no competing interests exist.
Introduction
Sustainable food production has been on the rise in recent decades as traditional agricultural practices, which contribute to large-scale environmental degradation and enormous resource consumption, fall short of fulfilling the demands of our growing human population [ 1 ]. Aquaponics offers a sustainable alternative to traditional food production methods by combining hydroponic plant cultivation with aquaculture in a semi closed-loop system [ 2 ] that minimizes water and fertilizer use, increases agricultural efficiency [ 3 ], and does not require arable land. Central to the health of fish and plants in these systems are microorganisms, which drive many critical functions such as nitrogen cycling, plant growth promotion, disease resistance, and nutrient uptake; however, a deeper understanding of microbial community composition and function in aquatic agricultural systems is central to engineering and scaling-up efficient, sustainable food systems with low natural resource dependence [ 4 ]. While some work has been conducted on microbial communities in hydroponics [ 5 ] and aquaponics [ 4 , 6 ], our knowledge of the microbial ecology of aquaponics is mainly grounded in soil-based agricultural research [ 7 – 9 ].
Interest among researchers and growers in aquaponic microbes has been focused on initiating nitrogen cycling and promoting plant growth via inoculation with plant growth promoting microbes (PGPMs). For this reason, one of two inoculation strategies are traditionally used to initiate cycling: 1) addition of commercially-derived nitrifying bacteria ( Nitrosomonas , Nitrobacter , and Nitrospira ) or 2) transfer of established bacteria from existing, healthy aquaponic systems. Despite the inclusion of PGPMs, a 2018 international survey found that 84.4% of aquaponic growers observed disease in their systems, of which 78.1% could not identify the causal agent [ 10 ]. Therefore, understanding the effect that microbial transfer has on production in aquaponics is crucial not only to establish best practices and increase commercial profitability by way of improving efficiency, but also to decrease loss due to disease. Of the growers who observed disease, a mere 6.2% used pesticides or biopesticides against plant pathogens and relied, instead, on non-curative actions, likely due to a lack of knowledge among aquaponic growers regarding disease control measures [ 10 ]. Knowledge of key associations between microbial genera and productivity throughout early stages of system establishment could enable the development of diagnostic tools for monitoring microbiome composition, potentially aiding in early detection and prevention of system collapse.
Institute for Systems Biology (ISB) established Project Feed 1010 ( PF1010 ) to promote education and research around sustainable food systems, such as aquaponics, to help combat global food insecurity. Through ISB high school internships supported by the Seattle Youth Employment Program, small-scale aquaponic experiments were designed and carried out in collaboration with researchers at the National Aeronautics and Space Administration (NASA) Ames Research Center to test how two inoculation strategies impacted productivity. The Institutional Animal Care and Use Committee (IACUC) protocol limited the number of fish per system to prioritize animal welfare, which meant that our aquaponic systems were nitrogen-limited compared to commercial systems. We examined how microbiome transfer from either pre-existing systems or commercial inoculum promoted or impeded plant productivity. We compared lettuce ( Lactuca sativa var . crispa ) production in these two distinct systems—those inoculated with the biofilter media from an established, fully cycled aquaponic system (“established inoculum treatment” or “EIT”) and those inoculated with a commercially-available microbial consortium (“commercial inoculum treatment” or “CIT”).
Results and discussion
An overview of the aquaponics systems and experimental design is shown in Fig 1A . Over the 61-day study period, lettuce growth (height, number of leaves, and root length) was significantly reduced in all EIT replicates compared to growth in CIT replicates ( Fig 1B–1D ). Physicochemical properties did not significantly differ across aquaponic systems over the study period ( S1 Fig , all individual Welch t-test p>0.07), making it unlikely that the observed growth disparity can be explained by system-wide biogeochemical parameters. Therefore, we explored potential associations between microbial community composition and plant growth parameters.
- PPT PowerPoint slide
- PNG larger image
- TIFF original image
(A) Aquaponics sampling timeline and system design. Left shows aquaponics system design and right sampling strategy. Gray circles on top denote experimental events and every black empty circle denotes a single sample. One fish feces sample could not be extracted at day 14 and was resampled at day 30. (B) Representative images of L . sativa plants and roots after a month of growth in systems with different inocula. (C) Plant growth over time. Each dot denotes the average plant height for a single aquaponic system taken at the indicated time point. Measures from the same tank are connected by lines. Gray line denotes growth in the prior aquaponics system that was used as the source for EIT. (D) Plant growth measures by inoculum. Each point denotes an average value measured in a single tank at a single time point (n = 104, 22, 105 for leaves, root length, and plant height, respectively). Stars denote significance under a Mann-Whitney U test (all p<0.001).
https://doi.org/10.1371/journal.pone.0247534.g001
We first investigated whether microbiome transfer affected the nitrogen transformation process. As anticipated, all systems showed low levels of nitrogen due to the limited number of fish allowed per system to maintain compliance with IACUC. One CIT system (tank 3) showed a transient nitrogen spike associated with the death of one fish ( Fig 2A ). However, areas under the nitrate curves (AUNC) were not significantly different between EIT and CIT tanks (Welch t-test p = 0.3) and AUNC variance was explained slightly better by the number of dead fish in each tank (R 2 of 0.37 vs 0.23, see Materials and methods ). Similarly, there was no significant difference in the number of fish that died between CIT and EIT systems. Previous studies of established aquaponic systems found a ubiquitous presence of nitrifiers such as Nitrosomonas , Nitrobacter , and Nitrospira , albeit in low abundances, and these organisms are described as major drivers of plant growth [ 6 , 11 ]. We only detected nitrifying taxa in the established inoculum itself, as well as in two EIT samples from days 21 and 61, respectively ( Fig 2B ). Instead, biofilter samples were dominated by Proteo- and Cyanobacteria ( S2 Fig and Fig 2C ). Even though our protocol was validated to detect nitrifiers ( S3 Fig ), we also found no nitrifiers in the commercial inoculum, which was dominated by Rhodanobacter , a genus containing known denitrifiers [ 12 ]. This suggests that the improved plant growth in CIT systems was likely independent of nitrifying bacteria. We also observed low nitrogen levels in our endpoint plant nutrient analyses ( S2 Table ) and water chemistry ( S1 Fig ), suggesting that nitrogen was limiting. This finding could explain the increased abundance of nitrogen fixers, such as Rahnella , and reduced abundance of nitrifying species ( Fig 2B and 2C ). We hypothesize that in nitrogen-limited aquaponic systems, nitrogen fixing bacteria may play an important growth-promoting role in supplementing the limited ammonia produced by fish by fixing atmospheric nitrogen and producing additional ammonia, which is a well-known nitrogen source for L . sativa [ 13 ].
(A) Shown are measurements for ammonia, nitrite and nitrate throughout the experiment. Colored lines denote smooth fit from a LOESS regression and filled areas denote 95% confidence intervals of the regression. Numbers of the panels denote tanks. Tanks 1–3 are CIT whereas tanks 4–6 are EIT. Dashed lines denote the three time points used for microbiome sampling. Boxed numbers denote the number of fish that died in the tank. (B) Relative abundance (fraction of total reads per sample) of known nitrifying taxa. (C) Abundances of ubiquitous bacterial genera across the samples (present in at least 2 samples at an abundance >300 reads). Colors of cells denote the normalized abundance on a base 10 log-scale. Sample names are composed of sampling group ID (e.g. T1), compartment (B = biofilter, F = fish feces, R = root, Comm = commercial inoculum) and tank number (1–6). Orange arrows denote genera of interest. Black fill color denotes initial root sample from rock wool cubes (not part of inoculation strategy).
https://doi.org/10.1371/journal.pone.0247534.g002
In examining whether microbiome transfer affected establishment of microbial communities in new systems, we found alpha-diversity increased with time in all compartments and achieved similar values for CIT and EIT tanks in biofilters and roots at day 61 ( Fig 3A ). This temporal development of alpha-diversity was not an artefact of a bias due to sequencing depth ( S4 Fig ). Aquaponic compartments each had distinct microbial compositions ( Fig 3B ). A total of 44% of variation in beta-diversity was explained by a combination of compartment (25%), inoculum (11%), and an interaction term of the two (8%; all PERMANOVA p values < 0.02). Conversely, prior studies in nitrogen-replete systems found that the microbial composition of different compartments in established systems, with the exception of fish feces, were quite similar [ 6 ].
(A) Alpha-diversity (Shannon index) over time. Colors and shapes denote initial microbial sources and sampling compartments respectively. All samples were rarefied to 3,000 reads each. (B) (B) PCoA plot of individual samples from all time points. Colors and shapes are the same as in panel A. All samples were rarefied to 3,000 reads each. Ellipses denote 95% confidence interval from Student t-distribution separating compartments. (C) Significant associations (FDR adjusted p<0.05) between bacterial genera and plant growth metrics or inoculum. Points denote the association coefficient in the respective regression and error bars denote the standard error of the coefficient. Fill color denotes average abundance across all samples. (D) Significant associations (FDR adjusted p<0.05) between bacterial genera and measures associated with nitrification. Circles denote the association coefficient in the respective regression and error bars denote the standard error of the coefficient. Fill color denotes average abundance across all samples. (E) Time course of selected genera associated with plant growth and inoculum. Time point zero is shared between all samples and denotes initial inoculum for biofilter and initial plant microbial composition for roots. Only genera with more than 300 reads in at least 2 samples were considered in A-E (see Materials and methods ).
https://doi.org/10.1371/journal.pone.0247534.g003
Given the differences in microbial composition between EIT and CIT systems, we hypothesized there may be a negative effect of microbial transfer from a prior system on plant growth, which acts independent of nitrogen concentration and cycling timeline. Thus, we investigated whether the difference in plant growth could be explained by an enrichment in potentially growth-inhibiting bacteria from the established system inoculum. This microbiome-inhibition hypothesis is similar to negative plant-soil feedback (NPSF), which has long been studied in both field and agricultural soils [ 14 – 17 ]. NPSF is thought to be characterized by enrichment of species-specific plant pathogens or the accumulation of allelochemicals that limit plant productivity in successive generations grown in the same soil [ 14 – 17 ]. This self-inhibitory process promotes plant community diversity by allowing sub-dominant species to thrive [ 14 , 15 ], and has served as a justification for crop rotations as a practice in soil-based agriculture [ 18 ]. Thus, we hypothesize that a similar negative feedback phenomenon may be responsible for the reduced aquaponics productivity.
In order to explore putative biotic mechanisms for the growth-promotion and negative-feedback hypotheses outlined above, we examined whether there were distinct bacterial genera in the plant roots that were associated with inoculum source and plant growth phenotypes. To that end we conducted negative binomial likelihood-ratio tests (LRT) between plant phenotypes and microbial abundances with DESeq2 [ 19 ]. Association tests between bacterial genus-level abundances and plant growth measures revealed that three highly abundant genera in the roots, namely Pseudomonas , Rahnella and Serratia , were significantly associated (LRT FDR corrected p<0.05) with plant growth ( Fig 3C , see Materials and methods ). Pseudomonas and Serratia relative abundances were higher in the EIT systems and were thus associated with diminished plant growth, whereas Rahnella was more abundant in CIT systems and was therefore associated with improved plant growth ( Fig 3C ). Some isolated incidents of fish death were observed across all systems, though it is unclear if this led to altered nitrate abundances (area under the nitrate curve, Spearman rho = 0.73, p = 0.1). However, no bacterial abundances in the roots were correlated with fish mortality, ammonia, or nitrate levels (all FDR corrected p>0.1), suggesting that establishment of the rhizosphere microbiota was independent of fish mortality or system-wide nitrogen cycling ( Fig 3D ). Although Pseudomonas , Rahnella and Serratia were all associated with plant growth metrics, only members of the genus Pseudomonas were 1) previously described as plant pathogens [ 20 – 22 ] and 2) found in both initial inoculum types. Across time points, we observed a relatively uniform accumulation of Pseudomonas in biofilters, with all biofilter microbiomes consisting of 5–30% Pseudomonas ( Fig 3E ). However, detection of Pseudomonas in the rhizosphere was highly dependent on inoculum type. EIT rhizosphere microbiomes were dominated by Pseudomonas (with 50–80% Pseudomonas , LRT FDR-corrected p<0.05), whereas CIT rhizosphere microbiomes contained less than 20% Pseudomonas . Instead, CIT rhizospheres were enriched for Aeromonas and nitrogen-fixing Rahnella , which were associated with improved plant growth ( Fig 3E ).
In summary, we saw two divergent but not necessarily mutually exclusive signals associated with inoculum type and aquaponic productivity. First, the genus Pseudomonas was enriched within biofilters across all systems, but only dominated the L . sativa rhizosphere in systems inoculated with microbes from an established aquaponic system, suggesting that EIC-specific growth-inhibitory pseudomonads may be responsible for a reduced yield in EIC-treated systems. However, our MinION sequencing data cannot resolve taxonomy beyond the genus level, so we are unable to verify whether or not specific bacterial pathogens were present in our systems. Furthermore, it is possible that viral, fungal, or other eukaryotic plant pathogens could be enriched in the established inoculum, as we did not collect data on non-bacterial microbiota in this study. Second, we saw enrichment of nitrogen-fixing Rahnella in CIT rhizospheres. Rahnella species are known to form associations with plant roots, promote plant growth, and have been found with other diazotrophs in nitrogen-fixing root nodules [ 23 , 24 ]. Given the low levels of nitrate and ammonia in our aquaponic systems, Rahnella strains may have promoted L . sativa growth.
Overall, further work is necessary to distinguish whether associations between aquaponics productivity and inoculum treatment are due to bacterial plant pathogens, growth-promoting microbes, or factors including other plant pathogens (e.g., viral, fungal, protist) or archaeal species (such as ammonia-oxidizing archaea), which were not quantified in our study. Additional studies with larger sample sizes, multi-omic data collection, and bacterial isolate characterization will be necessary to identify pathogenic or growth-promoting strains, establish causality, and test our mechanistic hypotheses. However, our findings indicate that CIT aquaponics systems showed greater yields when compared to EIT systems under nitrogen-limiting conditions and therefore that established inocula are not optimal for nitrogen-limited aquaponic systems. The decreased yield (an approximate 36-fold decrease in plant biomass; S1 Table ) observed in this study could be financially devastating to aquaponic farmers [ 25 ]. While most commercial aquaponic systems are run under higher nitrogen levels than in our study, it is likely that other research institutions will follow similar animal welfare standards in future aquaponic studies and therefore nitrogen will be limited. Therefore, our work is an important step towards characterizing aquaponic systems operated for research purposes, where more controlled experiments can be performed compared to commercial systems. Moving forward, metagenomic analyses, metabolomics, strain isolation, and whole-genome sequencing will provide deeper insights into the specific strains, functional genes, and small molecules involved in both positive and negative plant-inoculum feedbacks [ 26 ] and could lead to targeted strategies for engineering the microbial ecology of aquaponics systems to improve yield and resource use efficiency.
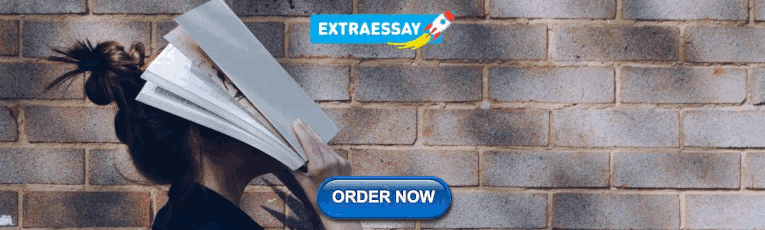
Materials and methods
Aquaponic system design.
Six independent aquaponics systems were constructed using a 4-compartment design ( Fig 1A ), with three replicate systems treated with a commercial inoculum and three with an established inoculum, in an open-air greenhouse in Seattle, Washington. These treatments will henceforth be labeled CIT (commercial inoculum treatment) and EIT (established inoculum treatment). Each system housed one aquaculture tank connected to one hydroponic unit utilizing the deep water culture method. These units were separated by a biofilter, which housed approximately 60 L of K3 filter media, as well as an 18 L solids filter. The experiment commenced when two adult Red Nile Tilapia ( Oreochromis niloticus ) were added to each system. On the same day, the 6 systems were divided into two sets of triplicate systems and inoculated with either Microbe-Lift commercial bacteria (tanks 1–3; “CIT”) or established microbes from an existing aquaponic system (tanks 4–6; “EIT”). Four weeks later, 13 lettuce seedlings, which had been sprouted in rockwool cubes outside the systems 3 weeks earlier, were added to the floating trays (Beaver Plastics 28-hole 2ft x 4ft Lettuce Raft) in each system and allowed to grow 35 days.
Animal care
Prior on-site research conducted at the Institute for Systems Biology under IACUC protocol number NB.01a.16 (“Educational Aquaponics”; OLAW #A4355-01 and AAALAC #001363) provided guidelines that were closely followed in this off-site study. Water chemistry, space, and fish food were all determined and maintained to optimize living conditions for all Nile Tilapia ( Oreochromis niloticus ) used in this study, and fish health and behavior were observed and recorded regularly. Fish were fed Stage 3 Intermediate AquaNourish Omnivorous Fish Feed pellets (Star Milling Co., Perris, California, USA; 37% crude protein, 10% crude fat, 2.2% crude fiber, 11% ash) every other day in an increasing amount (between 10–40 pellets per fish) during nitrogen cycling, so as not to produce excess, toxic ammonia at initial stages of cycling. Each approximately 203 L system housed two fish with a total length of 44 inches. To maintain compliance with our IACUC protocol, which prioritizes animal welfare, only two fish per system were used (the sum of their lengths being approximately 44cm). Because this stocking density is lower than that of most commercial aquaponic systems, this experiment was run under relatively nitrogen-limited conditions. Throughout this study, 5 fish died unexpectedly due to unknown causes in tanks 1, 2, 3, and 6. Water chemistry was ruled out as a cause, as there were no associations between water chemistry fluctuations and subsequent fish deaths. One possible cause may be stress-induced infection by a fish pathogen such as Saprolegnia or Aphanomyces . Although 16S rRNA analysis is not capable of detecting these fungal pathogens, Pseudomonas and Aeromonas were increasingly enriched throughout the study. Both of these genera are known to include species which can mitigate oomycete diseases in aquaculture by acting as an antipathogen agent [ 27 ]. Fish deaths were included as factors in our statistical analyses, to ensure that our reported results were independent of these adverse events.
Inoculation of systems
Systems in the CIT were inoculated with Microbe-lift (Cape Coral, Florida, USA) commercial bacteria, marketed as containing Nitrosomonas , Nitrobacter , and Nitrospira , whereas EITs were inoculated with biofilter media from a previously established, highly-efficient L . sativa -producing aquaponic system where nitrogen had been fully cycled for approximately 2 years. To inoculate these systems, 10 bioballs (approximately 105.8 ng/mL bacteria DNA) from the existing aquaponic system were collected and transferred to the biofilter of each new EIT system. On the same day, 30 mL of commercial bacteria (company recommendation) was added to the CIT biofilters.
Water chemistry maintenance
Throughout the study period, water chemistry (pH, temperature, ammonia, nitrite, nitrate, chlorine, hardness, and alkalinity) and plant growth metrics (number of leaves, plant height, root length, and presence of disease or discoloration) were collected 3–4 times per week ( S1 Fig ). Water chemistry data was collected using test strips (Tetra EasyStrips 6-in-1 Aquarium Test Strips and Tetra EasyStrips Ammonia Aquarium Test Strips) and a multi-parameter probe (Hanna Instruments ® GroLine Waterproof Portable pH/EC/TDS Meter). To account for evaporation and plant use, an average of 126.5 L of aerated, dechlorinated tap water was added to each tank throughout the experimental period. Approximately 45g of Instant Ocean ® Sea Salt (Blacksburg, VA) was added to each tank 5 days into the experiment to reach a conductivity of approximately 900 uS/cm.
Lettuce phenotypes
Height, number of leaves, discoloration, and pest pressure, were recorded for each plant every other day at a consistent time. Height was measured from the base of each stem to the natural crest of the tallest leaf. Leaf count included cotyledons and emerging buds. Root length was recorded at the midpoint and end of the experiment and was measured from the base of each rockwool cube to the longest root of each plant.
Sample collection
Microbiome samples were strategically collected throughout the 61 day study period and analyzed from 3 compartments of each system: 1) plant roots in the grow bed, where root-associated bacteria are located, 2) biofilter media in the biofilter where bacterial nitrifiers typically carry out nitrification, and 3) fish feces from the solids filter, where gut-associated bacteria can be found ( Fig 1A ). Sampling time points were reflective of key chemical species transformations occurring during nitrification, and therefore supposed microbial community shifts, across the study period (pre-cycling, during cycling, and post-cycling; Fig 1A ). The experiment ended 61 days after microbial inoculation and plants were harvested. At the end of the experiment, wet and dry mass (biomass) of plants were collected ( S1 Table ). Nutrient analysis of dried leaf samples was performed by the University of Missouri Soil and Plant Testing Lab ( S2 Table ).
Sample processing
Time point 0 for the roots was taken from rockwool cubes that seedlings were growing in prior to transfer to the aquaponic systems. Nine rockwool cubes were collected from the grow tray and all liquid was squeezed from the rockwool into a 50 mL falcon tube. Tubes were centrifuged at 4,000 xg for 10 min, supernatant was discarded, and pellets were flash frozen in liquid nitrogen and stored at -80C until DNA extraction. The final time point for the roots was taken at the time of system disassembly (and plant harvest). Roots were clipped at the base of all plants in each system and collected in 50 mL falcon tubes. PBS was added to the tube and samples were sonicated at low frequency (intensity 1) for 5 minutes total (five 30 second bursts, each followed by a 30 second rest period) in order to remove cells from roots. Following sonication, roots were removed from the tube and samples were centrifuged at 4,000 xg for 10 min, supernatant was removed, and pellets were flash frozen in liquid nitrogen and stored at -80C until DNA extraction.
Fish feces.
Fish feces were collected from the bottom of the aquaponic solids filter using a 25 mL serological pipette. Samples were centrifuged at 4,000 xg for 10 minutes to pellet the feces samples, supernatant was discarded, and pellets were flash frozen in liquid nitrogen and stored at -80C until DNA extraction.
Ten bioballs were collected from the established aquaponics biofilter using sterile forceps and stored in PBS. A single bioball was vortexed at maximum speed for 2 minutes in a 50 mL falcon tube and then sonicated at low frequency (intensity 1) for 5 minutes total (five 30 second bursts, each followed by a 30 second rest period). Following sonication, the bioball was removed from the tube and another bioball was added in, vortexed, and sonicated. This process was repeated until cells from 10 bioballs were collected for each sample. Cells from the 10 bioballs were then collected as a single pellet through centrifugation at 4,000 xg for 10 minutes, supernatant was discarded, and pellets were flash frozen in liquid nitrogen and stored at -80C until DNA extraction.
Analysis of nitrogen cycling
Continuous nitrogen measures for each tank were reduced to areas under the curve (AUCs) using the trapezoidal method. To identify the dominant covariates associated with nitrogen cycling, nitrate AUCs were regressed against a binary inoculum covariate and the number of dead fish with a linear model in R (formula “nitrate ~ inoculum + dead_fish”). Explained variance of each term was obtained from an ANOVA analysis on the fitted model.
DNA extraction, 16S rRNA sequence analysis
Microbial genomic DNA was isolated from samples using two similar extraction kits marketed specifically for bacterial DNA isolation from agricultural environments (Samples T0F1, T0F2, T0F3, T0F4, T0F6, and T0B with Qiagen PowerSoil kit and all others with PowerBiofilm kit). 16S rRNA genes were amplified using universal primers suggested by MinION (27F 5’–AGAGTTTGATCMTGGCTCAG and 1492R 5’–CGGTTACCTTGTTACGACTT ) [ 28 ]. A MinION Nanopore Sequencer was used for sequence analysis.
Amplicons were aliquoted to a starting concentration of 1 ug and were further processed and sequenced according to ONT’s 1D Native barcoding genomic DNA (with EXP-NBD103 and SQK-LSK108) protocol (v. NBE_9006_v103_revN_21Dec2016). Processing started with an AMPure XP bead purification step and proceeded to end-repair/dA-tailing, barcoding ligation, and adapter ligation steps.
Basecalling for the raw MinION files was performed by Albacore (v2.0.2) to yield the corresponding FASTQ files. Reads were processed using “filterAndTrim” methods from DADA2 [ 29 ]. The first 10 bp of the 5’ end were trimmed from all reads as they generally showed lower qualities. Raw reads were also trimmed to a maximum length of 1.5 kbp (the expected length of the 16S gene). Reads with more than 200 expected errors under Illumina error model (based on [ 30 ]) or more than 2 ambiguous base calls (“N” bases) were removed from the analysis (~70% of all reads passed these filters). PCR chimeras were removed using yacrd version 0.5.1 [ 31 ]. However, yacrd removed very few sequences (<1%) because the prior length cutoff likely removed the majority of PCR chimeras. The filtered reads were then aligned to the complete SILVA 16S database using minimap2 with the Oxford Nanopore preset allowing up to 100 alternative alignments per read [ 32 , 33 ].
Validation and mapping improvement for high error rates
We observed acceptable read qualities with a median of 15 corresponding to an average error rate of 3% under the Illumina model. However, quality measures are based on a predictive model and the nominal error rate is usually above 10% [ 34 ]. In order to evaluate the accuracy of nanopore sequencing on the full length 16S gene we used a small validation data set consisting of two biological replicates of a long-running established aquaponic system sequenced with the same nanopore protocol as well as V4-V5 Illumina amplicon sequencing. V4-V5 sequencing data (515F 5’–GTGYCAGCMGCCGCGGTAA , 926R 5’–CCGYCAATTYMTTTRAGTTT ; [ 35 ]) were analyzed using the DADA2 pipeline and used as the ground truth. Due to the high error rate of nanopore sequencing we expected a lot of spurious assignments when mapping to a 16S reference database such as SILVA. In order to limit those spurious matches, we employed an Expectation-Maximization (EM) algorithm similar to what is used in kallisto but without correction for gene length due to the fixed length of the 16S gene [ 36 ] (implementation available at https://github.com/Gibbons-Lab/mbtools ). Applying the EM algorithm led to a reduction of unique mappings for low abundance cutoffs compared to a “naive” counting algorithm that just uses the highest scoring match ( S3A Fig ). We observed good agreement of estimated abundances between Illumina and Nanopore sequencing down to the genus level if the taxon was observed in both sequencing technologies ( S3B Fig , R 2 between 0.5–0.8, Spearman rho between 0.73–0.85, all ANOVA p<10 −6 ). However, we also observed many spurious mappings only present in the nanopore data, with generally low abundances ( S3B and S3C Fig ). We found that using an abundance cutoff of read 300 counts removed >96% of all spurious mappings across all taxa ranks and >97% of all spurious mappings on the genus rank. This cutoff did not depend on library size, which itself varied between ~9.8K to ~125K reads per sample. Thus we employed this abundance cutoff throughout the manuscript. The final abundances as estimated by the EM algorithm were then annotated by the SILVA taxonomy down to the strain level where available and collapsed on the genus level [ 37 ]. We also verified whether the nanopore 16S primers were capable of identifying the same nitrifiers that were amplified by the V4-V5 primers used in the Illumina run. We found that all known nitrifying taxa identified by Illumina sequencing were also found in the nanopore data ( S3D Fig ).
Diversity metrics
Diversity estimates often depend on the library size (sequencing depth) of the sample. Library sizes ranged from ~9.8K reads to >125K reads across samples. In order to rule out bias based on library size, we applied rarefaction to 9000 reads to all samples. We also used rarefaction curves to judge whether sampling depth was sufficient to saturate the alpha diversity measure, which we found to be the case for all samples (see plateau in S4 Fig ).
Association testing
Read abundances across samples were normalized using the DESeq2 “poscounts” normalization strategy (similar to a centered log ratio transformation) [ 19 ]. Association tests were performed using DESeq2 with a prior filtering step to remove bacterial genera with either very low average abundances or low prevalence across samples (mean abundance >10 across all samples and present in at least 2 samples). This more permissive filter was used to allow for effective shrinkage for DESeq2. Significance was judged by a negative binomial log ratio test (LRT) comparing the full model to a model lacking the association term. Finally, we only considered significant tests for those genera which showed abundances larger than the default cutoff of 300 reads in at least two samples. Association tests between bacterial genus-level abundances and response variables were performed for each of the 3 environments (biofilters, roots, fish feces). The tested response variables were 3 plant growth measures (height, root length, number of leaves), inoculum type (CIT, EIT), and putative confounders (number of dead fish, area under ammonia and nitrate curves). False discovery rate was controlled by the Benjamini-Hochberg method [ 38 ]. No associations in the fish feces passed an FDR cutoff of 0.1.
Supporting information
S1 fig. water chemistry, environmental parameters, and system inputs measured throughout the study period..
Lines denote LOESS smoothed curves for each inoculum and bands denote 95% confidence intervals of the regression. Indicated p-values were obtained from individual t-tests of CIT vs EIT systems.
https://doi.org/10.1371/journal.pone.0247534.s001
S2 Fig. Relative abundances for the 12 most abundant phyla in the data set.
Sample names are composed of sample group ID (e.g. T1), compartment (B = biofilter, F = fish feces, R = root, Comm = commercial inoculum) and tank number (1–6). Panels denote inoculum as used in Fig 1 of the main text.
https://doi.org/10.1371/journal.pone.0247534.s002
S3 Fig. Validation of nanopore sequencing on a set of aquaponics biofilter samples that were sequenced using Illumina and nanopore technologies (2 replicates each).
(A) In low abundances, the Expectation-Maximization (EM) algorithm identifies fewer unique references than the “naive” strategy of just selecting the highest scoring read. Each dot denotes the number of unique 16S sequences in the SILVA database that pass the abundance cutoff. (B) Abundances across sequencing protocols. Each dot denotes the abundance of a single taxon at the indicated taxonomic rank. Blue lines denote a linear regression for the taxa found in both sequencing technologies and gray errors denote the 95% confidence interval of the regression. The red boxplot summarizes the distribution of spurious mappings in the nanopore data (absent in the Illumina data). (C) Distribution of false positive mappings (mappings not observed in the Illumina data) in nanopore sequencing. The dashed red line denotes the used abundance cutoff that removed >96% of those spurious mappings. (D) Abundances of nitrifying taxa in both sequencing protocols. Dots denote the sum in the two replicates. Abundances smaller than one denote taxa not detected in Illumina sequencing.
https://doi.org/10.1371/journal.pone.0247534.s003
S4 Fig. Rarefaction curves for all samples.
Points denote alpha diversity estimate (Shannon index) after subsampling to the specified depth. Lines denote LOESS smoothing regression lines for each individual sample. Colors and panels denote sampling time point and aquaponics system compartment, respectively. Endpoint of each curve denotes the actual depth of each sample.
https://doi.org/10.1371/journal.pone.0247534.s004
S1 Table. Final wet mass and dry mass (biomass) of L . sativa leaves in all replicates.
https://doi.org/10.1371/journal.pone.0247534.s005
S2 Table. Amount of 9 key nutrients found in L . sativa leaves at the end of the 61-day study period.
*Low concentrations compared to L . sativa soil-grown sufficiency range. **High concentrations compared to L . sativa soil-grown sufficiency range.
https://doi.org/10.1371/journal.pone.0247534.s006
Acknowledgments
This research was made possible due to strategic collaborations fostered by Claudia Ludwig.
- 1. Searchinger T, Waite R, Hanson C. Creating a Sustainable Food Future: A Menu of Solutions to Feed Nearly 10 Billion People by 2050. World Resources Institute; 2018 Dec.
- View Article
- Google Scholar
- PubMed/NCBI
- 10. Stouvenakers G, Dapprich P, Massart S, Jijakli MH. Plant Pathogens and Control Strategies in Aquaponics. In: Goddek S, Joyce A, Kotzen B, Burnell GM, editors. Aquaponics Food Production Systems: Combined Aquaculture and Hydroponic Production Technologies for the Future. Cham: Springer International Publishing; 2019. pp. 353–378.
- 19. Love MI, Huber W, Anders S. Moderated estimation of fold change and dispersion for RNA-seq data with DESeq2.
- 28. Stackebrandt E, Goodfellow M. Nucleic acid techniques in bacterial systematics. John Wiley & Son Ltd; 1991.
ORIGINAL RESEARCH article
Microbiological and nutritional analysis of lettuce crops grown on the international space station.
- 1 AECOM Management Services, Inc., LASSO, Kennedy Space Center, Merritt Island, FL, United States
- 2 NASA UB, Kennedy Space Center, Merritt Island, FL, United States
The ability to grow safe, fresh food to supplement packaged foods of astronauts in space has been an important goal for NASA. Food crops grown in space experience different environmental conditions than plants grown on Earth (e.g., reduced gravity, elevated radiation levels). To study the effects of space conditions, red romaine lettuce, Lactuca sativa cv ‘Outredgeous,’ plants were grown in Veggie plant growth chambers on the International Space Station (ISS) and compared with ground-grown plants. Multiple plantings were grown on ISS and harvested using either a single, final harvest, or sequential harvests in which several mature leaves were removed from the plants at weekly intervals. Ground controls were grown simultaneously with a 24–72 h delay using ISS environmental data. Food safety of the plants was determined by heterotrophic plate counts for bacteria and fungi, as well as isolate identification using samples taken from the leaves and roots. Molecular characterization was conducted using Next Generation Sequencing (NGS) to provide taxonomic composition and phylogenetic structure of the community. Leaves were also analyzed for elemental composition, as well as levels of phenolics, anthocyanins, and Oxygen Radical Absorbance Capacity (ORAC). Comparison of flight and ground tissues showed some differences in total counts for bacteria and yeast/molds (2.14 – 4.86 log 10 CFU/g), while screening for select human pathogens yielded negative results. Bacterial and fungal isolate identification and community characterization indicated variation in the diversity of genera between leaf and root tissue with diversity being higher in root tissue, and included differences in the dominant genera. The only difference between ground and flight experiments was seen in the third experiment, VEG-03A, with significant differences in the genera from leaf tissue. Flight and ground tissue showed differences in Fe, K, Na, P, S, and Zn content and total phenolic levels, but no differences in anthocyanin and ORAC levels. This study indicated that leafy vegetable crops can produce safe, edible, fresh food to supplement to the astronauts’ diet, and provide baseline data for continual operation of the Veggie plant growth units on ISS.
Introduction
Crop production in space may be a necessary and desirable component of future exploration systems ( MacElroy et al., 1992 ; Kliss et al., 2000 ). Fresh produce can be grown in situ to supplement a stored, packaged diet, and crops may provide beneficial nutrients as well as dietary variety. Veggie is a small plant growth chamber designed and built by Orbital Technologies Corporation (Now Sierra Nevada Corp., Madison, WI, United States) to grow vegetable crops in space ( Morrow et al., 2005 ; Morrow and Remiker, 2009 ).
The first Veggie plant growth chamber was launched to the International Space Station (ISS) in April, 2014 along with eighteen plant (rooting) pillows for the VEG-01 experiment. Veggie is a simple plant growth facility that uses LED lights and fans to circulate ISS air through the plant growth volume. A transparent, extensible bellows attached to the light unit via magnets contains the growing plants and any debris, and directs air flow from the bottom of the canopy to the top of the growth volume ( Figure 1 ). Screens remove large particles from the cabin air before it passes through the plant growth chamber, however, no level of filtration is present, so plants growing in Veggie are exposed to any microbial or chemical constituents present in the ISS environment. More details about the Veggie facility can be found in Massa et al. (2016) . For the VEG-01 and VEG-03 demonstration tests, plants were grown from seeds in plant pillows. Plant pillows are small growing bags that interact with a root mat water reservoir on the Veggie baseplate. Pillows contain a calcined clay substrate mixed with controlled release fertilizer and wicks for seed attachment. Surface sanitized seeds are glued into these plant pillow wicks, and pillows are packaged for flight under sterile air. Further details of seed and pillow preparation are provided by Massa et al. (2017b) .
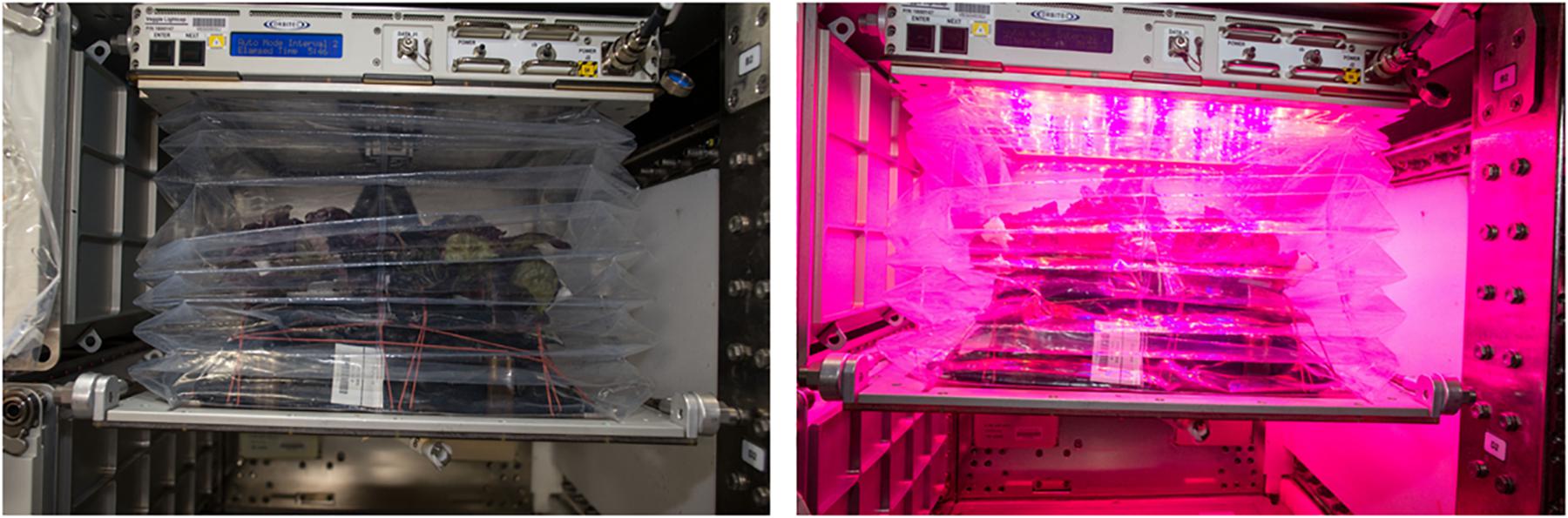
Figure 1. Veggie payload containing a crop of mature red romaine lettuce in Veggie pillows aboard the ISS with the light panel off (left) and red light panel on (right) . Bellows are in the up position.
A major focus of our research in the past several years has been to identify crop candidates with high potential for dietary supplementation, given the constraints associated with the current space diet. Ongoing testing has indicated that some essential human nutrients are either deficient in the processed and pre-packaged space foods (e.g., potassium, Vitamin K) or may degrade to inadequate levels over the times required for long duration missions (e.g., Vitamin B1, Vitamin C) ( Cooper, 2013 ). Several of these nutrients may be supplemented through fresh salad crops and will provide antioxidants and phytochemicals in a natural, whole-food form. Previous studies with salad crops have focused on combinations of environmental conditions (e.g., light, temperature, CO 2 concentration) needed to optimize plant growth for space settings ( Richards et al., 2004 , 2006 ). While the macro nutritional value of crops was often evaluated in productivity studies (calories, protein, fats, and carbohydrates), micro nutritional data are limited ( McKeehen et al., 1996 ; Wheeler et al., 1996 , 1997 ). In order to utilize “pick-and-eat” produce such as the lettuce crops grown in VEG-01 and 03 as a supplemental food for space, nutritional assessment is essential. In this study we compare the nutritional quality of red romaine lettuce ( Lactuca sativa , cv. Outredgeous) grown on ISS to ground controls grown under the same ISS environmental conditions including relative humidity, CO 2 concentration and temperature. Within a Veggie chamber, conditions such as humidity and temperature can vary from ISS conditions outside the chamber, for example, the temperature and humidity inside the Veggie tend to be slightly elevated compared to the open cabin air. These differences may have an impact on the elemental, nutritional and microbiological components of the plant. Increased temperatures have been shown to decrease macronutrient content in red leaf lettuce while increasing flavonoids and phenolics ( Sublett et al., 2018 ). Studies have shown that increased humidity initiates a positive response in biomass yield in many agricultural crops while concomitantly decreasing transpiration rate altering the uptake of water and some nutrients ( Tibbitts, 1979 ; Gislerød and Mortensen, 1990 ).
Microbiological testing is an integral part of any food safety program to verify quality, production and proper handling processes but few studies exist for space grown plants ( Hummerick et al., 2010 ). We conducted microbiological testing of Veggie-grown produce to understand potential risks to astronauts as consumers. Good agricultural practices and procedures are in place to minimize microbial contamination of crops grown in Veggie, and microbial testing is one tool to verify the effectiveness of these processes ( Hummerick et al., 2011 ). Plant-growth conditions, the surrounding environment, mineral nutrition, and plant species can all influence the microbial populations living on plant surfaces ( Lindow and Brandl, 2003 ). A variety of microorganisms can be found on fresh produce, most as part of the normal flora of the plant with no adverse effect on humans if consumed ( Heaton and Jones, 2008 ; Leff and Fierer, 2013 ; Oyinlola et al., 2017 ).
Microbiological analysis can also provide insight on the microbial ecology (population density and types of bacteria and fungi) of Veggie-grown plants. The likelihood of contamination and growth of potential human pathogens on plants grown in Veggie is low since sterilization/sanitization of seeds, plant growth medium, irrigation water, and hardware can be controlled. However, if a contamination event were to occur, the risks to the crew of exposure to food borne pathogens could be increased considering the association of immune dysregulation to spaceflight ( Borchers et al., 2002 ). Heterotrophic bacterial counts and the presence of Enterobacteriaceae that serve as an indicator of contamination of processed foods, irrigation water, and food processing surfaces are frequently present in raw foods like fresh produce making those tests irrelevant as risk indicators for fruits and vegetables ( Heaton and Jones, 2008 ; Leff and Fierer, 2013 ). The microbial load on crops intended for consumption by astronauts presumably should fall within an acceptable range of microbiological standards set for food. Currently, however, there are no standards in place for microbial levels in fresh produce grown in space. The closest related NASA standards are for non-thermostabilized food items including any of the freeze dried foods consumed in orbit, e.g., shrimp cocktail, chocolate candy, granola bars. For example, the current limit for aerobic bacteria on a non-thermostabilized food item is <2 × 10 4 colony-forming units (CFU) per gram on one sample or <1 × 10 4 on two out of five samples ( Perchonok et al., 2012 ) however, these standards apply to prepackaged food sent from Earth. For fresh produce grown on ISS, the microbiological acceptability is assessed on a case-by-case basis relying on microbiological analysis of crops grown in ground studies analogous to Veggie grown crops. Microbiological analysis has been performed previously on edible plants grown in the Russian Lada chamber on ISS ( Hummerick et al., 2010 , 2011 ). Mizuna, barley and radish from Lada were stored at −80°C after harvest until analysis was performed at Kennedy Space Center, Merritt Island, FL, United States. A range of microbial densities as indicated by aerobic bacterial and fungal plate counts was found depending on plant type and location. The four samples of mizuna, the only leafy green tested, were grown in different Lada root modules at different times and counts ranged from 3.1 × 10 3 – 8.7 × 10 5 . When compared to field grown or market produce of similar types these numbers are well within the expected range of microbial densities or even lower ( Holvoet et al., 2015 ; Wood et al., 2015 ; Fröhling et al., 2018 ; Hummerick et al., unpublished data).
However, not all microorganisms can be cultured in the laboratory and alternate methods of community characterization are needed ( Byrd et al., 1991 ; Schaule et al., 1993 ; Kalmbach et al., 1997 ). Genomic DNA analysis using whole genome or amplicon sequencing provides the capability of identifying 98–99% of the microbes present in a complex, heterogeneous microbial community. With the current advances in technology, the Illumina MiSeq provided a platform for capturing the community structure of the leaf and root material returned from the ISS and for the ground control samples. Identifying the community makeup is the first step in understanding the complex structure and interactions between mutualistic or symbiotic partners in plant growth systems.
The purpose of this study was to determine the effects of ISS and Veggie environmental conditions on the microbiological and nutritional quality of Veggie grown lettuce intended for crew consumption.
Materials and Methods
Plant growth and harvest.
The Veggie production system on ISS is a small plant growth chamber designed and built by ORBITEC (Madison, WI, United States) to grow crops in space ( Morrow et al., 2005 ; Morrow and Remiker, 2009 ). The Veggie production system, equipped with LED lighting and a passive watering system, launched to the ISS in 2014 aboard Space X’s third Commercial Resupply (CRS-3) mission ( Figure 1 ). Red romaine lettuce Lactuca sativa cv. ‘Outredgeous’ was grown in Veggie rooting pillows as described by Massa et al. (2017a) . Briefly, plants were grown in Veggie pillows containing solid, porous, arcillite (calcined clay) substrate in two different blends, either 100% sized to 600 μ–1 mm or a 1:1 ratio of that size to 1–2 mm (Turface Proleague, Profile Products, LLC) and controlled release fertilizer (Nutricote 18-6-8, type 180, Florikan, Sarasota, FL, United States). Procedures for detailed substrate and pillow preparation are outlined in Massa et al. (2017b) . Two surface sanitized seeds were attached with guar in each plant pillow between paired germination wicks. All plant rooting pillows with attached seeds were kept dry in heat sealed, gas-impermeable Tedlar bags until test initiation on the ISS. Seeds for three separate, independent plantings (biological replicates) were germinated and grown for 33–56 days on ISS and ground controls were run in controlled environment chambers under ISS environmental conditions with a 24 h delay for VEG-01A and B and a 72 h delay for VEG-03A. Each experiment began with six rooting pillows. Germinated seeds were thinned to one plant per pillow at day 7. Each independent planting was grown at different times aboard ISS with parallel ground experiments for comparison ( Table 1 ).
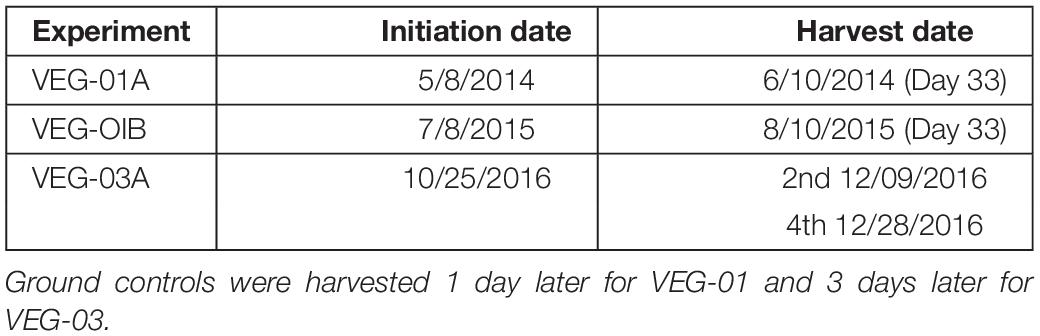
Table 1. Initiation and harvest schedule of red romaine lettuce crop in Veggie aboard ISS.
VEG-01A was grown from May 8 to June 10, 2014; VEG-01B was grown from July 8 to August 10, 2015, and VEG-03A was grown from October 25 to December 28, 2016. VEG-01A and B were harvested after 33 days of growth using sanitized scissors to remove the leaves ( Table 1 ). VEG-03A leaves were harvested sequentially starting at 33 days followed by 3 weekly harvests. Harvested leaves from each plant were wrapped in foil and placed into a −80°C freezer. The first, third, and half of the fourth harvests were consumed by the crew. Only the 2nd harvest and the remaining leaves (approximately half of the total leaves) of the final harvest from VEG-03A were stored for sample return. After completion of the final harvest for all three plantings, two rooting pillows from each independent experiment were removed from Veggie hardware and placed into bags and frozen at −80°C. Ground control samples were processed and stored the same as flight samples. The Veggie baseplate was positioned 30.5–31.75 cm below the LED light array to provide approximately 200 μmol⋅m 2 ⋅s –1 of photosynthetically active radiation at the surface of the pillows in the center of the Veggie unit at a ratio of 12 red (630 nm): 3 Blue (455 nm): 1 green (530 nm). A photoperiod was set to 16 h light/8 h dark. Environmental conditions from the three experiments are summarized in Supplementary Table S1 . Both the VEG-01A and part of the VEG-03A experiment on ISS had data collected inside the Veggie chamber using a temperature and relative humidity data logger.
Sample Processing
After return to Kennedy Space Center, Merritt Island, FL, United States samples from all three experiments were maintained in a −80°C freezer until analysis. Plant samples were removed from the freezer and processed immediately while pillows were thawed at 4°C overnight. Leaf biomass was divided for microbiological and chemical analysis. The samples intended for chemical analysis were subsequently frozen at −80°C, freeze dried, then ground up using an IKA Tekmar A10 Analytical Grinding Mill. Roots were removed from each pillow and divided for microbiological and molecular analysis.
Microbiological Analysis
Leaf and pillow root samples were placed into sterile 50 ml centrifuge tubes with sterile phosphate buffered saline (PBS) with glass beads, weighed then shaken vigorously for 2 min. Sample extracts were diluted into PBS and appropriate dilutions were plated in duplicate onto trypticase soy agar (TSA) and inhibitory mold agar (IMA). Plates were incubated at 30°C for 48 h for TSA and 72–120 h for IMA before enumeration of colonies. Individual colony phenotypes were selected for each sample and streaked for isolation. Isolated bacterial colonies were identified using the Micro Id System (Biolog, Hayward, CA, United States) or MicroSEQ 16S rDNA sequencing kit for bacteria (Thermo Fisher Scientific, Waltham, MA, United States). Fungal colonies were identified using the MicroSEQ D2 LSU rDNA kit for fungi (Thermo Fisher Scientific, Waltham, MA, United States). Sequencing was completed on the ABI 3130 Genetic Analyzer (Thermo Fisher Scientific, Waltham, MA, United States). Bacterial and fungal DNA sequences were identified using MicroSEQ ID Software V2.0 (Bacterial Library, 2009; Fungal Library, 2011, Applied Biosystems, Life Technologies, Foster City, CA, United States) and/or NCBI Basic Local Alignment Search Tool (BLAST).
For microbial food safety screening, sample extracts were plated onto E. coli /coliform and Staph Express Petrifilm (3M, St. Paul, MN, United States) according to manufacturer’s instructions. Petrifilms were incubated at 35°C for 24 h and colonies positive for E. coli and S. aureus were enumerated. Coliform colonies, if present, were re-isolated and further identified using Biolog GEN III plates. Screening for Salmonella was done by inoculating buffered peptone water (BPW) with 1 ml of sample extract followed by incubation at 35°C for 24 h. For selective enrichment, 1 ml of the incubated BPW was transferred into 5 ml of Rappaport-Vassiliadis (RV) broth or Tetrathionate broth (Thermo Fisher Scientific, Waltham, MA, United States) and incubated for 24 h at 35°C. Broths were then streaked onto selective media for Salmonella and incubated at 35°C for 24–48 h. These methods were adapted from the FDA bacteriological analytical manual ( FDA U.S. Food and Drug Administration, 2018 ).
Microbial DNA Isolation, 16S and ITS PCR, and Sequencing
DNA was isolated from sample material processed for microbiological analysis described above. Each sample was centrifuged at 13,000 rpm to pellet microbial cells, suspended in RNA later and stored at −80°C until processing for DNA isolation was completed. DNA was extracted from plant leaf and root material of the Veggie pillows using the Microbial Cell DNA Isolation Kit (Qiagen, Inc, Carlsbad, CA, United States) then quantified using the Qubit 2.0 double stranded DNA assay (Invitrogen, Grand Island, NY, United States). The variable 4 region of the 16S ribosomal RNA gene (rRNA) was selected as a phylogenetic marker to identify microorganisms taxonomically to the species level. A dual indexing system for multiplexing was adapted for these communities and polymerase chain reaction (PCR) was optimized for reagent concentrations and volume. Each community was labeled with two barcoded primers for identification at completion of sequencing. Amplicons were created in triplicate using 1 ng of template DNA, and final reagent concentrations of 1X buffer, 200 μM dNTP’s, 25 mM MgCl 2 , and 300 nM each barcoded 16S rRNA gene primer for bacterial identification or barcoded ITS primers for fungal identification ( Bokulich and Mills, 2013 ; Kozich et al., 2013 ). The PCR cycling conditions were 95°C for 5 min to denature the Taq polymerase enzyme, followed by 30 cycles of 95°C for 1 min, 58°C for annealing and 72°C for 2 min for extension. A final 10 min extension of 72°C completed the PCR run on a Bio-Rad C-1000 thermocycler. After PCR all amplicons were purified using the Min-Elute System (Qiagen, Carlsbad, CA, United States) and quantified with the Qubit 2.0 high sensitivity ds DNA assay (Invitrogen, Grand Island, NY, United States). The purified amplicons were then pooled in an equimolar concentration to create the library following Illumina protocols. The sample library was combined with a 10% Phi-X control library to create diversity and sequenced on the Illumina MiSeq using a V2 500-cycle kit (Illumina, Inc, San Diego, CA, United States).
Sequence Analysis
All 16S and ITS FASTQ sequences were obtained using the Illumina MiSeq Control Software and default settings for demultiplexing all samples. Sequence analyses for 16S sequences were completed using RDP GreenGenes ( DeSantis et al., 2006 ) to obtain the taxonomic reference. ITS FASTQ results were analyzed with the UNITE database ( Kõljalg et al., 2013 ) to analyze the reference sequences and for taxonomic assignment. The Shannon Index was calculated for alpha diversity for each sample with MiSeq control Software (Illumina, Inc. San Diego, CA, United States) while the Bray–Curtis ( Anderson, 2001 ) statistics methods calculated beta diversity between samples.
Total Anthocyanins
Approximately 100 mg of each dry sample powder was placed in an ASE350 cell of the Dionex Automated Solvent Extraction System (Dionex Corporation, Sunnyvale, CA, United States) and extracted with a solvent mixture of methanol:water:acetic acid (MWA) 85:14.5:0.5 (V/V/V) (methanol and acetic acid; Sigma-Aldrich, St. Louis, MO, United States). Parameters for the ASE 350 were: 100°C with 5 min static time; 70% flush; 90 s purge; 1 cycle; and 1500 psi. Extracts were analyzed immediately after extraction for total anthocyanin content by reading their absorbance at 530 and 650 nm (Beckman DU 700). A standard curve was created using Cyandin-3-Glycoside (Sigma-Aldrich, St. Louis, MO, United States).
ORAC and Total Phenolic Content
A separate extract was prepared for the determination, firstly, of the antioxidant capacity using the hydrophilic Oxygen Radical Adsorption Capacity (ORAC-FL) assay and secondly, the total phenolics in the sample. Twenty five milligrams (25 mg) of the freeze dried sample was placed in an ASE 350 cell and extracted with acetone:water:acetic acid (AWA) at the ratio of 70%:29.5%:0.5%. Parameters for the ASE 350 were: 80°C with 5 min static time; 60% flush; 60 s purge; 1 cycle; and 1500 psi. Aliquots of 0.2 ml were subsequently reacted with Folin-Ciocalteu phenol reagent (Sigma Aldrich, St. Louis, MO, United States) for the determination of total phenolics by the modified Folin-Ciocalteu assay ( Prior et al., 2005 ). A series of known concentrations of gallic acid (Sigma Aldrich, St. Louis, MO, United States) was prepared and reacted with the same reagent to create a calibration curve that was in turn used to determine the concentration in the sample extracts. Consequently, the total phenolics were expressed as gallic acid (GA) equivalents. A diluted (1/50) AWA extract was subjected to the ORAC-FL assay as described by Wu et al. (2004) and Prior et al. (2005) . Specifically, dilutions of the AWA extracted samples were prepared in a 75 mM phosphate buffer solution (pH 7.0). Aliquots of 20 μL of the diluted sample were placed (in triplicate) in a 96 well transparent flat bottom microplate (Thermo Fisher Scientific, Waltham, MA, United States), along with appropriately diluted Trolox [(±)-6-Hydroxy-2,5,7,8-tetramethylchromane- 2-carboxylic acid; Sigma-Aldrich, St Louis, MO, United States] standards (in triplicate), under subdued light. A “forward-then-reverse” pattern was used to place samples in the microplates, and edge wells were not used for standards or samples due to possible plate effects. Samples were analyzed on a BioTek Synergy Hybrid plate reader (BioTek, Winooski, VT, United States) which automatically added 200 μL of diluted fluorescein (Sigma-Aldrich, St. Louis, MO, United States) into each well, followed by 20 μL of the peroxyl radical generator (AAPH [2′2′azobis (2-amidinopropane)] Sigma-Aldrich, St. Louis, MO, United States). The assay was monitored every few minutes for 2 h, at 37°C. The ORAC-FL value was calculated from the area under the decay curve, and was reported as μmol Trolox equivalents (TE)/g dry weight.
Elemental Analysis
Dried and ground plant material (0.2–0.5 g) was digested in an open vessel system using a graphite-heating block (Mod Block, PN 4370-010007, CPI International). The plant material was digested at 95°C using a modification of the Environmental Protection Agency, (EPA) Method 3050B, as described below. A 5 ml aliquot of 70% nitric acid (70% Trace Metal Grade, Fisher Scientific, Suwanee, GA, United States) was added to the samples and then boiled for approximately 2 h (or until sample was completely clear). After cooling 2.5 ml of 30% hydrogen peroxide (Fisher Scientific, Suwannee, GA, United States) was applied. When the peroxide reaction ceased, samples were reheated for an additional 50 m in covered vials. Samples were cooled overnight, diluted to 50 ml with ultra-pure DI water and then passed through a 25 mm 0.45 μm syringe filter (GE Whatman, Pittsburgh, PA, United States).
Samples were analyzed via Inductively Coupled Optical Emission Spectrometry. (iCAP 7000 Series, Thermo Fisher Scientific, Waltham, MA, United States) A multi-element standard (Environmental Express) was diluted to the same acid concentration as the samples and quantification was done by external calibration.
Statistical Analysis
Data from microbiological counts (log transformed) and chemistry values were compared using a one-way ANOVA followed by Tukey’s multiple comparisons test using GraphPad Prism version 8.0.0 for Windows (GraphPad Software, San Diego, CA, United States). Alpha diversity was determined using the Shannon Diversity Index and was calculated by Illumina RDP Control Software (Illumina, San Diego, CA, United States). Beta diversity of community sequencing was determined with Bray–Curtis dissimilarity ( Anderson, 2001 ) using a one-way ANOVA (QIIME 2.0) ( Bolyen et al., 2018 ). A t -test (Microsoft Excel) was done to compare differences in the percent phyla identified.
Microbial Counts on Leaves and Roots
VEG-01A was the first time the Veggie facility had been used for plant growth on ISS ( Massa et al., 2017a , b ) so presumably it would have been the least likely of the three plantings described in this study to harbor microbial contamination. Aerobic plate counts for the leaves harvested from VEG-01B flight plants were significantly higher than the counts from both harvests of VEG-03A flight and the ground controls ( P < 0.05) ( Figure 2 ). With the exception of VEG-01B, the flight and ground control aerobic plate counts on leaves were not significantly different. The bacterial counts on ground control samples from VEG-01B were significantly lower than the flight leaves by orders of magnitude ( P < 0.0001) ( Figure 2 ). Previous studies ( Hummerick et al., 2010 , 2012 ) have shown an increase in microbial counts with a repeated harvest or “cut and come again” protocol as was done in VEG-03A. An increase between the 2nd and 4th harvests was not seen in bacterial counts on the leaves, however, fungal counts in the flight leaves were significantly higher in the 4th harvest when compared to the 2nd harvest ( P = 0.0002) ( Figure 3 ).
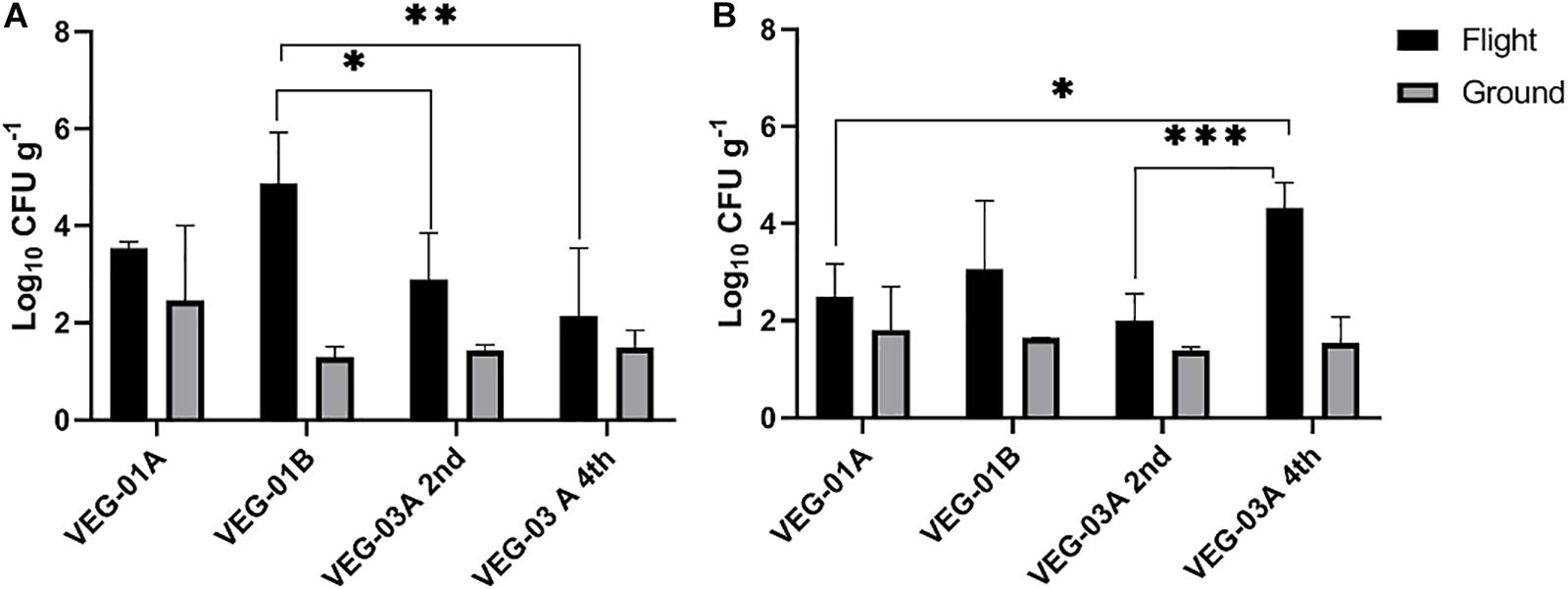
Figure 2. Mean bacterial (A) and fungal (B) counts on Outredgeous leaves from three Veggie experiments. Bacterial counts are CFU on TSA per gram of frozen tissue and fungal counts are CFU from IMA plates. Error bars represent standard error of the mean. Horizontal lines indicate significance between flight samples. * P < 0.05, ∗∗ P < 0.01, ∗∗∗ P < 0.001. Significant differences were determined using an ANOVA with Tukey’s post test to compare groups.
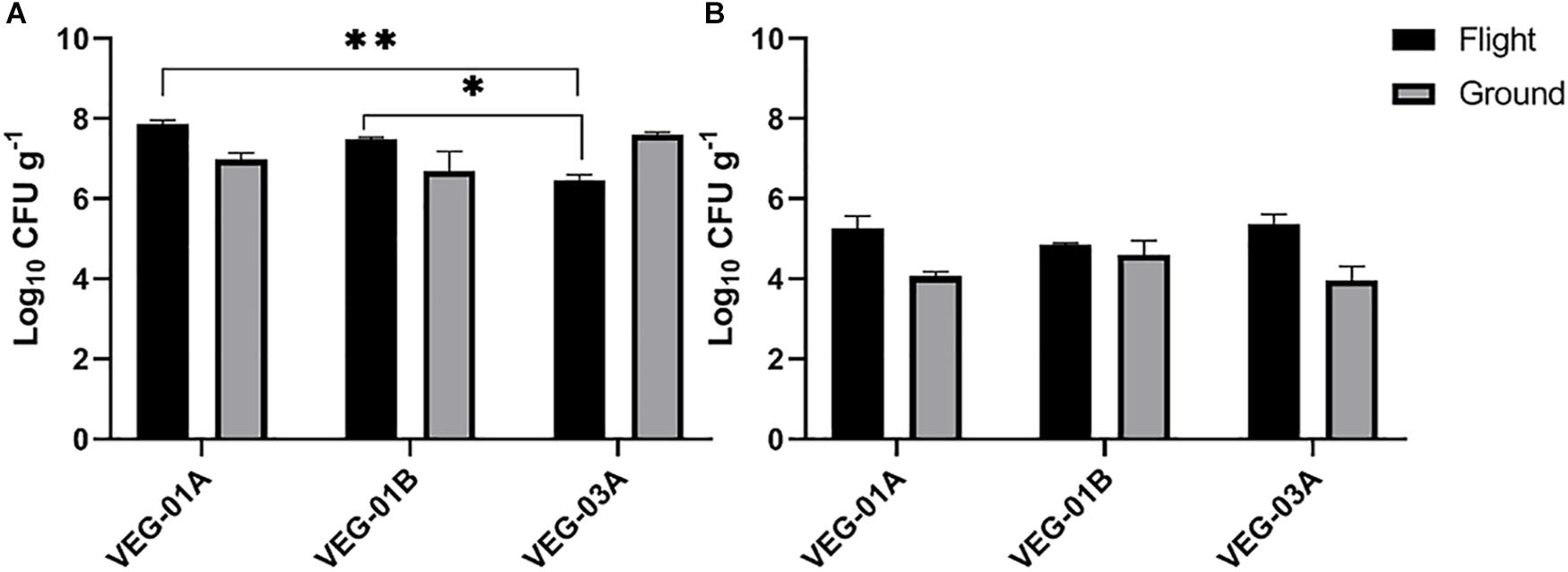
Figure 3. Mean bacterial (A) and fungal (B) counts on Outredgeous roots from three Veggie grow outs. Bacterial counts are CFU on TSA per gram of frozen tissue and fungal counts are CFU on IMA plates. Error bars represent standard error of the mean. Horizontal lines indicate significance between flight samples. ∗ P < 0.05, ∗∗ P < 0.01. Significant differences were determined using an ANOVA with Tukey’s post test to compare groups.
The bacterial counts on lettuce leaves grown on ISS in VEG-01A, B and both harvests from VEG-03A were relatively low on average, 3.53, 4.86, 2.89, and 2.14 log 10 CFU/g respectively ( Figure 2 ), when compared to farm or market produce counts of similar type ( Valentin-Bon and Jacobson, 2008 ; Rastogi et al., 2012 ; Jackson et al., 2013 ; Holvoet et al., 2015 ; Wood et al., 2015 ; Hagenmair and Baker, 2016 ; Oyinlola et al., 2017 ; Fröhling et al., 2018 ; Zhang et al., 2018 ). Fungal counts on Veggie grown lettuce ranging from 2.3 to 4.3 log 10 CFU/g were within the range of yeast and mold counts on lettuce reported in the literature ( Figure 3 ) ( Oliveira et al., 2010 ).
Bacterial counts from the roots in the VEG-03A grow out were significantly lower than the other two grow outs and lower than the corresponding ground control ( Figure 3 ) although these counts were in a range of 10 6 -10 8 per gram which is typical of lettuce root tissue ( Riser et al., 1984 ; Adesina et al., 2009 ).
Screening for potential food borne pathogens, E. coli, Salmonella sp. , and S. aureus on the leaf tissue yielded negative results. These screening results were corroborated by NGS data.
Cultivated Bacterial and Fungal Isolate Identification
Cultivation of individual bacterial colony phenotypes on TSA from VEG-01 and VEG-03 samples yielded 14 genera identified from the leaf samples ( Table 2 ) and 19 from the root samples ( Table 3 ). It is important to note that culture based isolation utilized in this study is limited to the selection of the mostly dominant colony phenotypes that grew under aerobic conditions on one type of general growth media. A majority of the bacterial taxa isolated from the Veggie grown leaves are known endophytic and/or epiphytic phyllosphere commensals or symbionts including Arthrobacter ( Scheublin and Leveau, 2013 ), Methylobacterium ( Peredo and Simmons, 2018 ), Sphingomonas ( Kim et al., 1998 ), Pantoea ( Whipps et al., 2008 ), Burkholderia ( Eberl and Vandamme, 2016 ), and Curtobacterium ( Chase et al., 2016 ). Paenibacillus is ubiquitous in soil and is associated with plants predominately as a rhizosphere bacterium exhibiting plant growth promoting characteristics ( Grady et al., 2016 ). A few of the leaf isolates are known to be transients on the surface of the leaf and are not typically part of the normal phyllosphere microbial community including Bacillus and Staphylococcus ( Maduell et al., 2008 ; Dees et al., 2015 ). Human associated bacteria of the genus Staphylococcus were found on the leaves of both flight and ground samples from VEG-03A. These are not natural inhabitants of the plant phyllosphere, however certain strains can be pathogenic to humans and cause food borne illness under optimal growth conditions ( Kadariya et al., 2014 ).
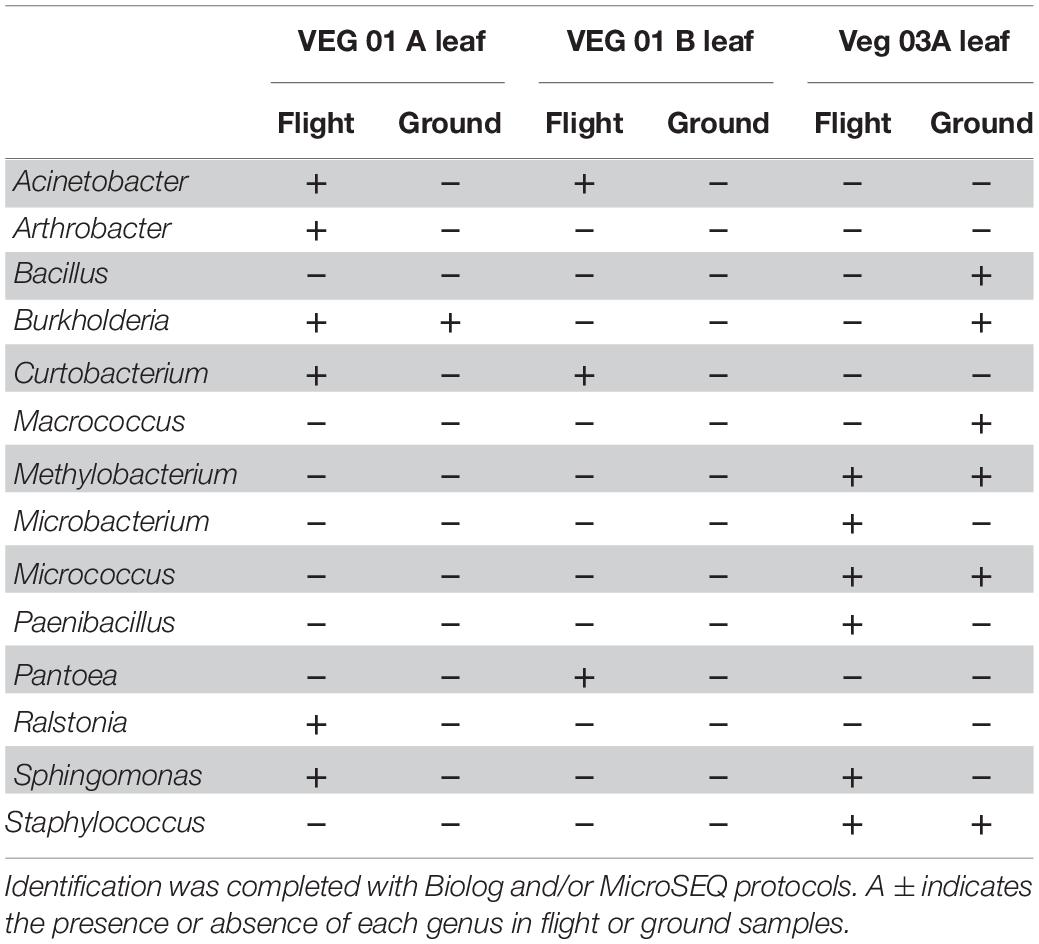
Table 2. Bacterial isolates recovered and identified from leaf tissue from two separate rooting pillows from three independent experiments grown aboard ISS with parallel ground studies for comparison.
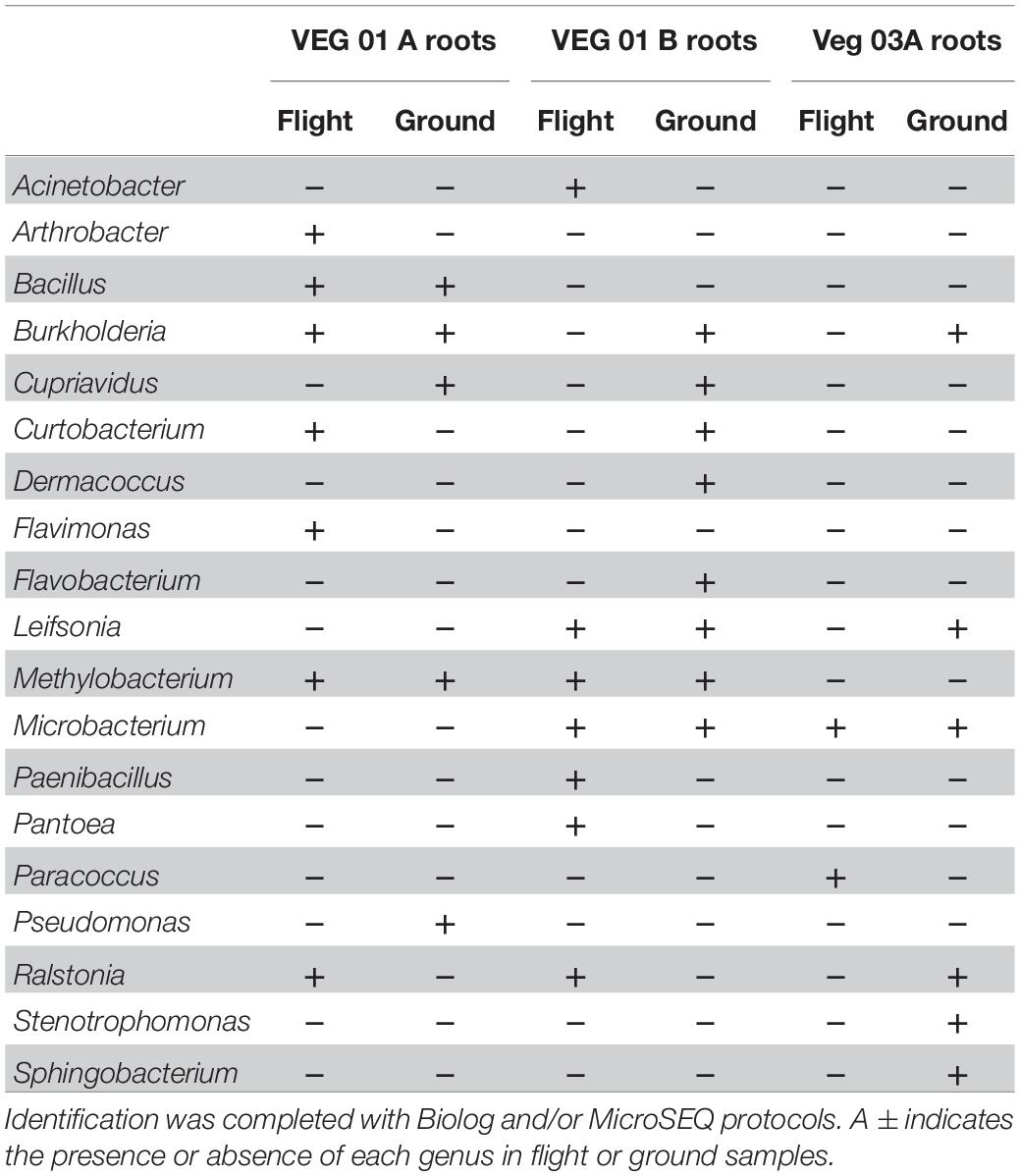
Table 3. Bacterial isolates recovered and identified from root tissue from two separate rooting pillows from three independent experiments grown aboard ISS with parallel ground studies for comparison.
The bacterial genera isolated from the roots ( Table 3 ) are predominately associated with soil and plant phyllosphere and rhizosphere. Ten of the 19 root isolates were also recovered from the leaf samples excluding the four genera Macrococcus, Micrococcus , Staphylococcus , and Sphingomonas .
Nine genera of fungi were isolated and identified from leaf tissue ( Table 4 ), while six were recovered from root ( Table 5 ). All the fungal genera present in the roots were also present on the leaves with the exception of Alternaria . The fungi Aspergillus, Penicillium , and Alternaria are ubiquitous, saprophytic fungi often isolated from soil and the environment including ISS surface and air samples ( Royer et al., 2004 ; Venkateswaran et al., 2017 ). Verma et al. (2011) found many of these genera of filamentous fungi as endophytes in the Indian Lily plant root. Among the fungi, the basidiomycete, Rhodotorula was the most common and in fact was isolated from every Veggie sample with the exception of the VEG-03 leaf tissue.
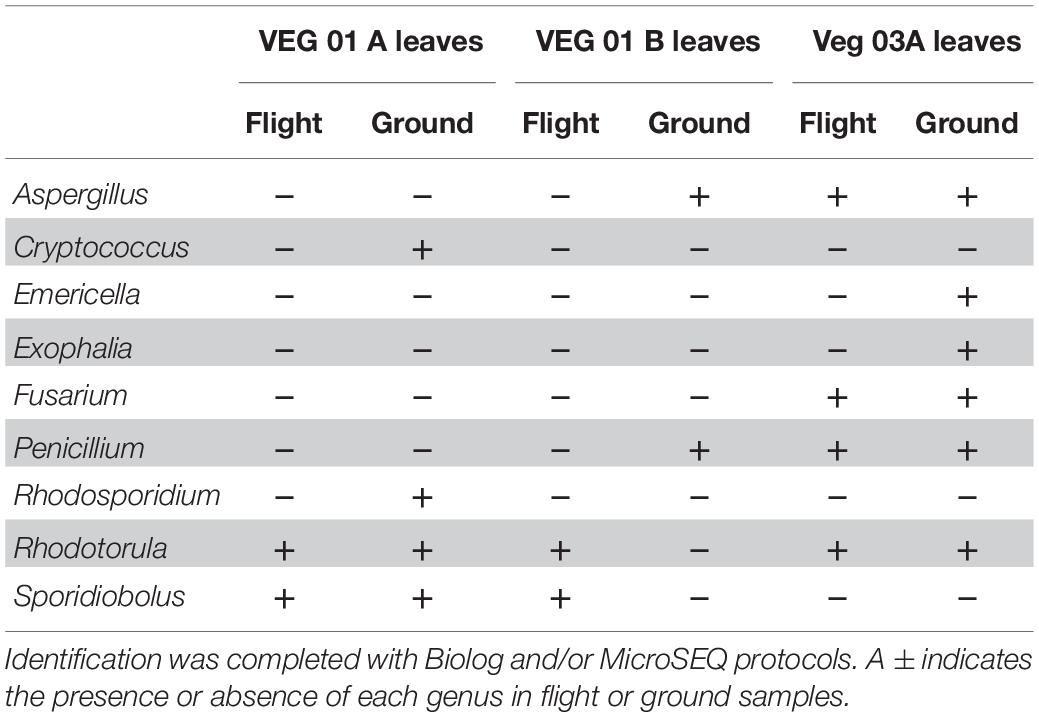
Table 4. Fungal isolates recovered and identified from leaf tissue from two separate rooting pillows from three independent experiments grown aboard ISS with parallel ground studies for comparison.
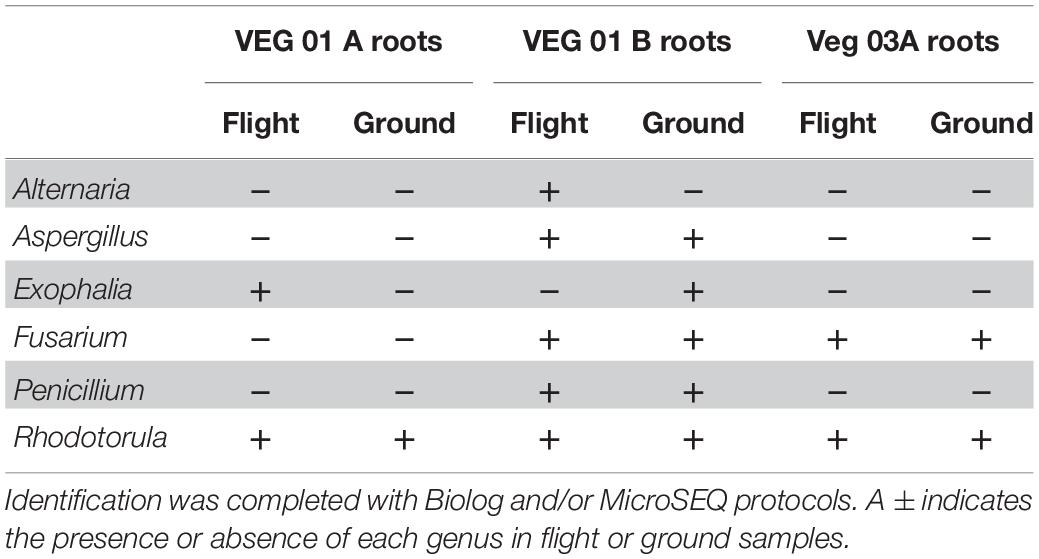
Table 5. Fungal isolates recovered and identified from root tissue from two separate rooting pillows from three independent experiments grown aboard ISS with parallel ground studies for comparison.
Community Sequencing
Sampling and sequencing of the flight and ground Veggie grown plants were completed on a minimum of two root pillows and up to five leaf samples per grow out. Sequencing profiles for each of the Veggie plantings were used to calculate and investigate the Shannon Species Diversity Index (Alpha Diversity) ( Table 6 ) and establish relative diversity within the bacterial communities in leaf and root and ground and flight samples. Leaf diversity was lower than root in each of the three lettuce crops and there was no significant difference between flight and ground samples ( Table 6 ). Although diversity was similar in all roots or leaves, there appeared to be variation in the dominant genera.
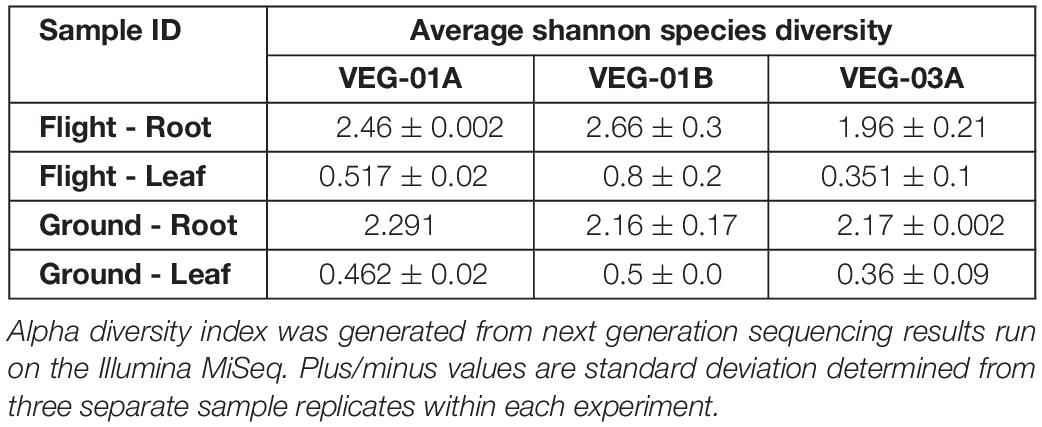
Table 6. Average Shannon Diversity Indices for three, independent Veggie experiments VEG-01A, VEG-01B, and VEG-03A, on the International Space Station and parallel ground studies.
Alpha diversity in leaf tissue of the three Veggie experiments showed a significant difference between ground and flight leaf samples of VEG-03A only ( P = 0.01). VEG-01A and B flight samples were not significantly different from the respective ground samples. There was also no significant difference in Beta diversity between VEG-01A, VEG-01B, and VEG-03A experiments. Bray Curtis dissimilarity calculations comparing all three experiments were not significantly different between any leaf or root samples of the three tests ( P > 0.05).
A majority of the bacterial community sequencing at the phyla level were assigned to phyla Proteobacteria, Bacteroidetes, Actinobacteria, and Chloroflexi. Comparison at the phyla taxonomic level indicated no significant difference between ground and flight root communities nor in the leaf communities. The dominant phyla for all plants, both ground and flight, was Proteobacteria. VEG-03A flight roots had the lowest percent of the Proteobacteria reads at 59% while the corresponding flight leaf sample was 76% of the total bacterial communities. All other plants from VEG-01A and B ranged from 80 to 96% Proteobacterial reads. However, this disparity was not significantly different. A closer look at the genera contained in the Veggie leaf and root samples showed that Burkholderia, Ralstonia , and Janthinobacterium dominated the Proteobacteria phyla ( Figure 4 ). Other Proteobacteria present in the samples were Azospirillum , and Herbaspirillum , which were detected in higher abundance in VEG-03A as well as Bradyrhizobium and Mesorhizobium , all of which may play a role in nitrogen fixation ( Okubo et al., 2012 ). The dominant genera representing the Chloroflexi was the gram positive, thermophilic, Thermogemmatispora , a soil microbe ( Komaki et al., 2016 ). These microbes were also transported to the leaf but were detected in much lower abundances (percentage), particularly in the flight and ground samples in VEG-03A. Thermogemmatispora was also detected in higher percentages than other microbes but was less than in VEG-03A. The dominant microbe representing the Bacteroidetes, which took second to the Proteobacteria in numerous samples was Chitinophaga. Chitinophaga was higher in abundance of reads in flight sample roots with only VEG-01B showing an elevated abundance in the ground samples ( Figure 4 ).
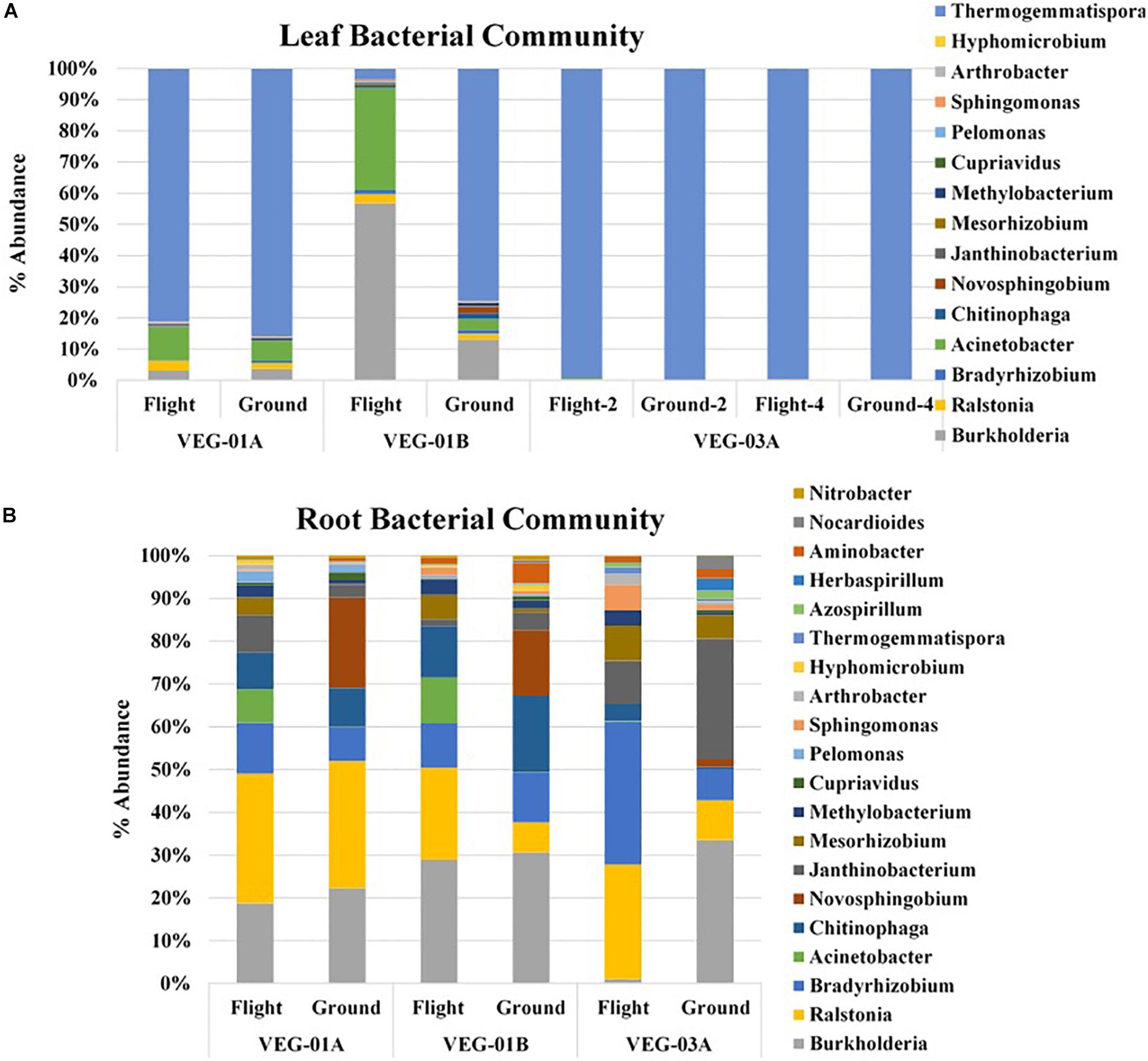
Figure 4. Bacterial community for lettuce leaf (A) and root (B) tissue for VEG-01A, VEG-01B, and VEG-03A experiments. Flight experiments were grown on the ISS while ground controls were grown in Environmental Growth Chambers at Kennedy Space Center, Merritt Island, FL, United States. Bacteria are the top genera obtained from next generation sequencing on the Illumina MiSeq.
Fungal communities from VEG-01A and VEG-01B were surveyed and it was determined that the Ascomycota phyla dominated both flight and ground communities as well as leaf and root communities (92–99%). Few Basidiomycota were detectable. VEG-01A flight roots presented the highest representation at 7% with VEG-01B flight root community containing 6%. All other communities contained 2% or less of total fungi detected ( Figure 5 ).
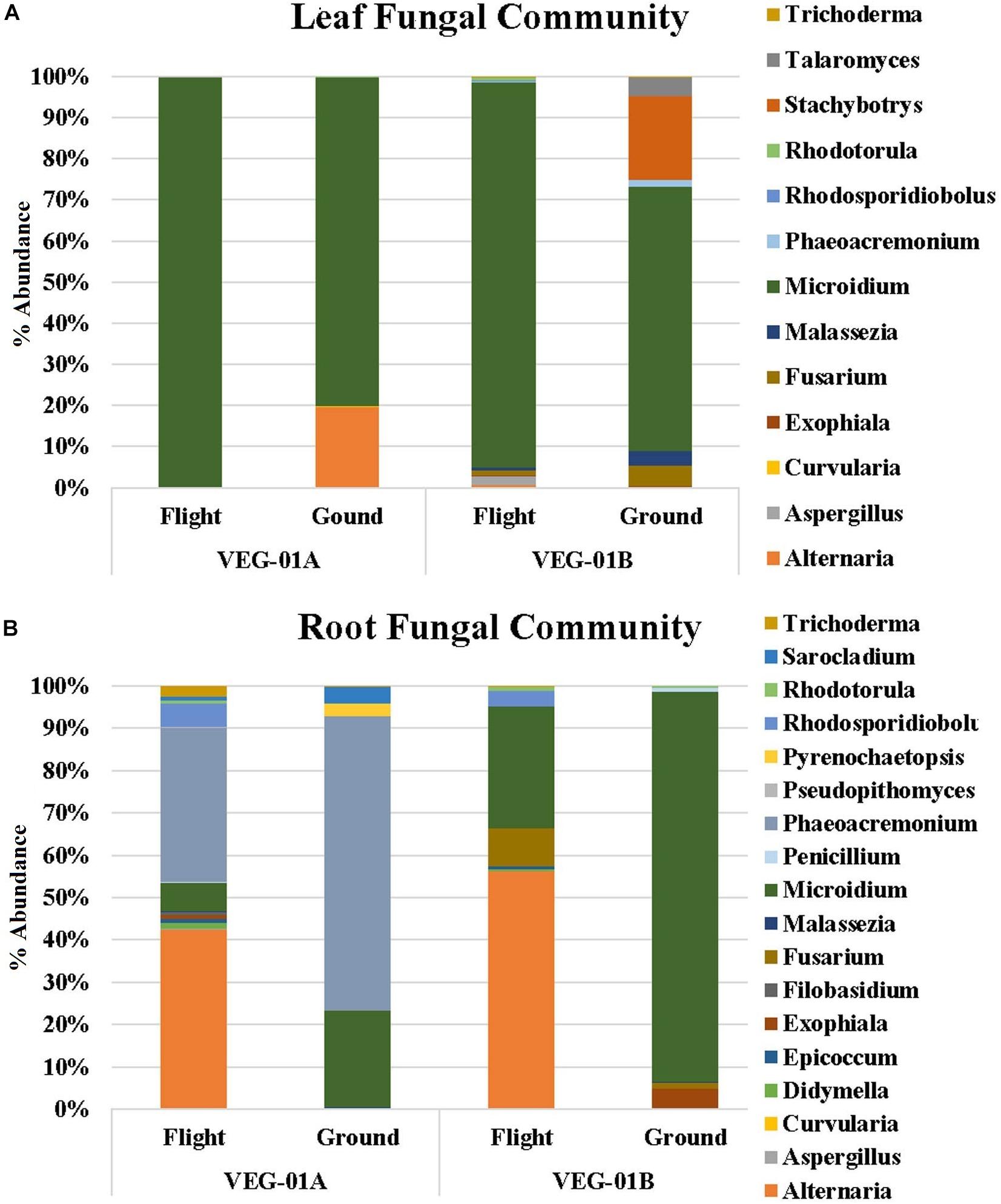
Figure 5. Fungal community for lettuce leaf (A) and root (B) tissue for VEG-01A and VEG-01B experiments. Flight experiments were grown on the ISS while ground controls were grown in Environmental Growth Chambers at Kennedy Space Center, Merritt Island, FL, United States. Fungi are the top genera obtained from next generation sequencing on the Illumina MiSeq.
Fungal diversity in the root samples for both flight and ground was significantly higher than leaf – an expected outcome ( P < 0.05). Among the dominating genera in flight and ground leaf samples was Microidium , an obligate biotroph ( Braun and Cook, 2012 ; Meeboon and Takamatsu, 2017 ). Leaf samples also housed Alternaria , Fusarium (VEG-01B only), and Malassezia , a yeast like fungi. Root samples from all three growth tests had increased levels of Alternaria, Phaeoacremonium , and Rhodosporidiobolus ( Figure 5 ). Alternaria was found in all samples with the exception of VEG-01A leaf tissue while Phaeoacremonium was present in increased abundance in VEG-01A root and in reduced numbers in VEG-01A leaf tissue ( Figure 5 ).
Comparison of culturable and non-culturable microbes in all samples provided corroborating evidence in that all culturable microbes were identified in the sequencing data. However, sequencing data revealed many additional genera present, increasing the species diversity.
Leaf tissue from flight and ground experiments were analyzed for changes in elemental composition ( Table 7 ). There was no significant differences between ground and flight samples within each experiment, however when comparing among all three experiments, Fe and K content were significantly lower in VEG-03A samples compared to VEG-01A and VEG-01B (Fe, P ≤ 0.001; K, P ≤ 0.0001). On the other hand, Na content was found to be significantly higher in VEG-01B compared to VEG-01A or VEG-03A ( P ≤ 0.0001). Phosphate (P) measured among all three experiments showed that VEG-01B had higher P contents than VEG-01A and VEG-03A ( P ≤ 0.05). Similarly, sulfate measured as S and Zn contents were statistically higher in VEG-01B compared to either VEG-01A or VEG-03A (S, P ≤ 0.05; Zn, P ≤ 0.0001). No significant differences were observed in either Ca, Mg, or Mn content.
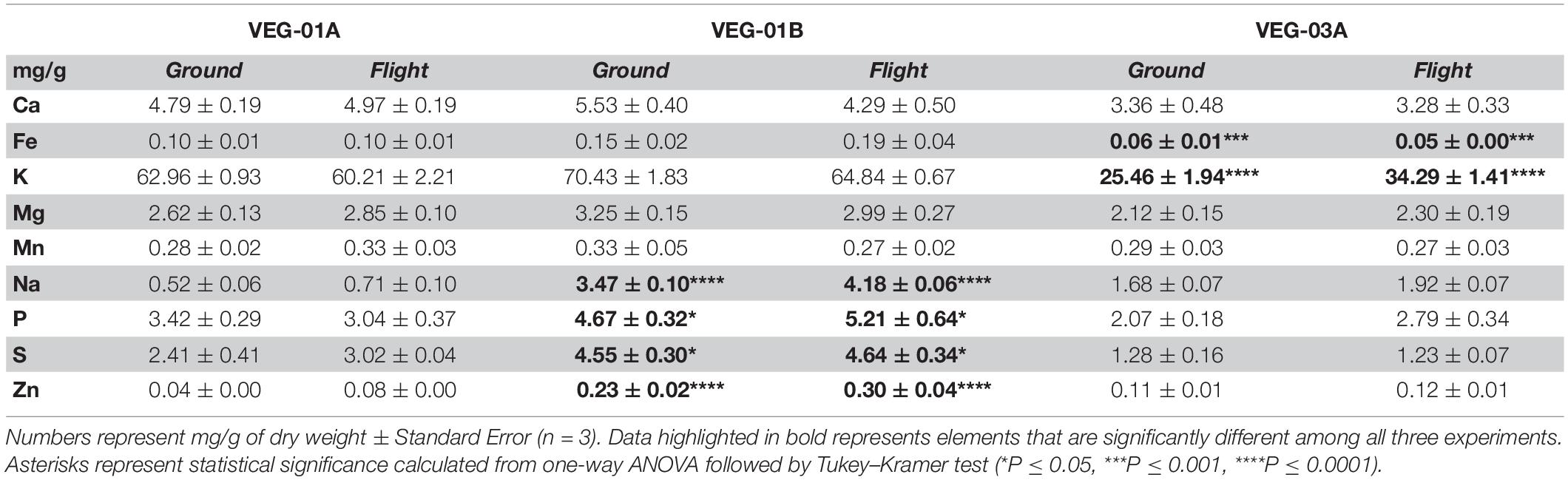
Table 7. Elemental content of lettuce leaf tissue from VEG-01A, VEG-01B, and VEG-03A ground and flight samples.
Total Phenolics, Anthocyanins and ORAC Analysis
As a measure of antioxidant capacity, total phenolics, anthocyanins and oxygen radical absorbance capacity (ORAC) were measured on lettuce leaf tissue, flight and ground samples from all three tests ( Table 8 ). Comparing total phenolics, VEG-03A with 15.0 (ground) and 19.6 (flight) mg/g of dry weight (gallic acid equivalents) had the statistically lowest amounts when compared to either VEG-01A or VEG-01B ( P ≤ 0.001). Anthocyanins measured as cyanidin-3-glucoside equivalents showed no difference among all three experiments compared. Similarly, ORAC units measured among all three experiments did not exhibit any observable variance.
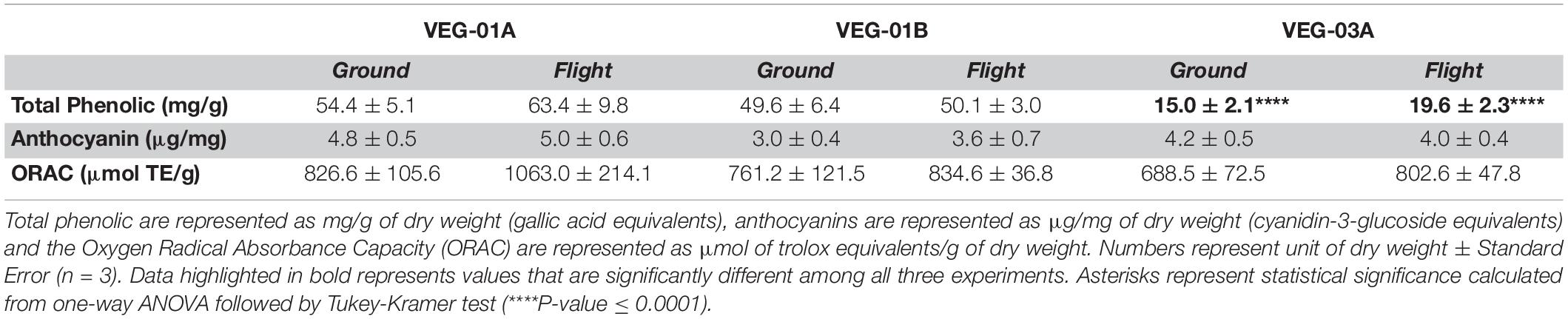
Table 8. Antioxidant capacity of lettuce leaf tissue from VEG-01A, VEG-01B, and VEG-03A flight and ground samples.
Differences in culturable bacterial and fungal density on the leaves in each of the Veggie plantings can not necessarily be traced to a particular source or environmental condition. However, it is reasonable to conclude that environmental conditions such as temperature and humidity, water levels provided, the microbiota in the air on ISS, and possible human interaction at the time of growth and harvest may contribute to the microbial community and density on the surface of the leaves. Terrestrially, airborne bacteria and fungal spores can land on the surface of leaves and become a part of the epiphytic microbial community albeit transient and dynamic depending on selective pressures ( Lindow and Brandl, 2003 ; Vokou et al., 2012 ; Maignien et al., 2014 ). Varying conditions such as humidity, leaf surface moisture and temperature can also effect the colonization and growth of bacteria and fungi on leaf surfaces. There is a positive correlation between fungal sporulation and the duration of leaf surface moisture ( Talley et al., 2002 ; Li et al., 2014 ). Poor air circulation can cause persistent droplets of moisture on the surface of a leaf due to transpiration producing a micro environment favorable for microbial proliferation. In the case of the three Veggie plantings on the ISS, environmental data collected by HOBO data recorders ( Supplementary Table S1 ) showed that temperature and humidity were slightly higher inside the Veggie chamber during the first 22 days of VEG-03A growth than the other two Veggie tests. Additionally, germinating seedlings in the VEG-03A test had excess moisture in the early period of growth, likely due to a fan malfunction, when compared to the VEG-01A and VEG-01B plants. It was during this experiment that our team identified a consistent anomaly with the Veggie hardware – specifically that when power is cycled to the hardware, upon restarting the fan will read “low” but it will actually be off. This problem also led to issues of excess moisture in an earlier Zinnia test but a repetition of this with lettuce confirmed the anomaly. In contrast, VEG-01A and VEG-01B tests had no fan issues, but instead the plants had some periods of low water early on (see Massa et al., 2017a ). Given that Veggie is a manually watered system and that fluid behavior in microgravity differs considerably from that on Earth, these early Veggie experiments involved considerable variation in fluid addition as both the ground teams and the astronauts struggled to effectively water the crops. Logged data from VEG-03A were not collected for the duration of the 64 days experiment so it is speculative to conclude any correlation between microbial counts and Veggie chamber conditions.
Key to this work is determining the microbiological food safety of Veggie grown crops. Bacterial and fungal counts on fresh plant material are not necessarily an indication of the food quality and microbiological safety of the item and vary depending on the type of plant ( Leff and Fierer, 2013 ; Qadri et al., 2015 ). Studies show that leafy greens grown in the field or greenhouse have a range of approximately 4–8 log 10 CFU bacterial counts per gram of sample while yeast and mold counts on lettuce are reported in the range of 2–5 log 10 ( Valentin-Bon and Jacobson, 2008 ; Rastogi et al., 2012 ; Jackson et al., 2013 ; Holvoet et al., 2015 ; Wood et al., 2015 ; Hagenmair and Baker, 2016 ; Oyinlola et al., 2017 ; Fröhling et al., 2018 ; Zhang et al., 2018 ). The microbial counts on lettuce leaves grown on ISS in VEG-01A, B and both harvests from VEG-03A were relatively low on average when compared to farm or market produce counts of similar type. In addition, none of the target human pathogens were detected in culture based testing and 16S community sequencing. These results demonstrate that from a microbiological perspective the Outredgeous variety of lettuce grown in Veggie was safe for human consumption.
Microbiological data collected from lettuce and reported in the literature provides evidence that different parts of the plant, i.e., leaves and roots support distinct microbial communities ( Ibekwe and Grieve, 2004 ; Toju et al., 2019 ). While our culture based isolation of bacteria recovered a few species unique to leaves many of the isolates present on the phyllosphere were also present in the roots. Previous work on the relationship of the phyllosphere bacterial community and airborne bacteria by Vokou et al. (2012) demonstrated that colonization of the phyllosphere is not always related to the aerial microbial community within the plants proximity and may include commensal and symbiotic taxa as well as environmentally indigenous transients. Staphylococcus has been isolated on the ISS on both surfaces and in the air ( Venkateswaran et al., 2014 , 2017 ) so it is not unlikely that plants in Veggie chambers would be exposed to these types of bacteria. Microbiological surveys done on a variety of surfaces and materials on the ISS reported many of the same bacteria and fungi that we isolated from plant material grown in Veggie. We compared bacterial and fungal isolates from this study of Veggie grown plants with isolates described in a study by Venkateswaran et al. (2017) which characterized the microbiology of ISS surfaces. More than 50% of the genera of bacteria and 100% of the fungi isolated and identified on ISS surfaces were also isolated from Veggie samples.
NASA reported monitoring results of space station samples including air surfaces and water from 1998 to 2012 using cultivation methods ( Castro et al., 2004 ; Yamaguchi et al., 2014 ). In these studies, the two most commonly isolated genera from water samples collected between 2009 and 2012 were Burkholderia sp. and Ralstonia sp. These are persistent and common in station potable water as well as terrestrial water sources and have been isolated and identified from Space Shuttle potable water ( Koenig and Pierson, 1997 ; Ryan et al., 2007 ) so it is not unexpected that these were also isolated in the roots and leaves of both flight and ground control plants.
Plants, regardless of growth conditions, harbor an indigenous population that may affect plant health and, if utilized as a food source, human health. One of the important findings in this study is the similarity and densities in the dominant leaf and root microbial community members as those reported in the literature grown under very different conditions ( Dees et al., 2015 ).
Culturable microbes include approximately 10% of the community microbial participants. Research on core rhizosphere and phyllosphere associated bacterial microbiota (symbiotic and pathogenic) have been described using culture-independent sequencing methods ( Panstruga and Kuhn, 2019 ). Next generation sequencing provides an alternative method to identify non-culturable microorganisms. It is important to identify these organisms as it may provide insight into the safety and health quality of the plants/crops being harvested and consumed. Each of the bacterial and fungal isolates identified in this study were confirmed with sequencing results, however, many additional genera were identified. Research studies indicate Proteobacteria, Bacteroidetes, and Actinobacteria phyla dominate communities, though differences may occur between host plant species at lower taxonomic levels ( Panstruga and Kuhn, 2019 ). Sequencing data from the three Veggie experiments followed these trends.
Lettuce leaf tissue in all three Veggie experiments was dominated with Thermogemmatispora , a gram positive, sporulating, soil organism that has been known to produce secondary metabolites provided to plants as a secondary resource ( Komaki et al., 2016 ). Thermogemmatispora has been found in lettuce seeds in high abundance but was reduced in root and leaf tissues (unpublished data, Khodadad et al). Bradyrhizobium and Mesorhizobium are also commonly found in soils as they may participate in nitrogen fixation ( Okubo et al., 2012 ). Bradyrhizobium has also been noted in higher abundance in low nutrient soils ( Okubo et al., 2012 ). Both microbes were present in higher abundance in flight root samples and were low or undetectable in leaf tissue with the highest abundance in VEG-03A roots. VEG-03A was grown for longer periods of time and may have depleted the nutrient levels in the substrate. In addition, microbes commonly associated with water were also detected in both leaf and root tissue. These microbes mentioned previously include Ralstonia , Burkholderia, Sphingomonas, Cupriavidus , and Pseudomonas were identified in all leaf and root samples of all three Veggie experiments. These have been identified as present on ISS as well as in the ISS water system ( Castro et al., 2004 ; Checinska et al., 2015 ; Venkateswaran et al., 2017 ).
It is important to note that several of the microbes identified may be potential endosymbionts with plants or fungi and be present as epiphytic or endophytic organisms. One example is Chitinophaga , found in all flight and ground root samples. Though it is unknown what the function of this bacteria may have been within these Veggie communities, it has been found to interact with fungal species present in plants by enhancing nutrient uptake and growth, altering plant water interactions or deterring potential pathogens ( Shaffer et al., 2017 ). These interactions have the capability of shaping plant health and productivity by accessing and making additional carbon sources available ( Kivlin et al., 2011 ; Shaffer et al., 2017 ). To determine their role and benefit to plants or fungi would require additional flight and ground studies. As fungi were present in each flight and ground sample community, it is important to note those in abundance and their persistence over time. Fungi have been detected on the ISS in various locations ( Venkateswaran et al., 2014 , 2017 ). Leaf and root systems of VEG-01A and B were surveyed for the presence of fungi and compared. Alternaria, Microidium , and Phaeoacremonium were the top three fungal genera identified with sequencing. Alternaria , an Ascomycota, has been described as a saprophyte that may decompose organic matter, and increase in communities with high humidity ( Patriarca, 2016 ). They may also associate with other fungal genera such as Fusarium or Stachybotrys . Though neither of these fungal genera were identified in VEG-01A, both were first detected in VEG-01B samples with higher incidence in ground samples therefore, they may have been introduced as a contaminant. Phaeoacremonium is a plant associated endophyte previously associated with lettuce and woody plants ( Mostert et al., 2005 ) while Microidium may be an opportunistic plant pathogen especially in high humidity environments ( Braun and Cook, 2012 ; Meeboon and Takamatsu, 2017 ).
Plants require macronutrients (N, P, K, Ca, Mg, S) and micronutrients (Fe, B, Mn, Cu, Zn, Mo) for their growth and development ( Epstein, 1972 ). These elements are essential and play critical roles in multiple plant processes. Comparing all three experiments, VEG-01B had higher elemental contents for Na, P, S, and Zn, whereas VEG-03A had the lowest amounts for Fe and K. Considering the chronological order of the experiments onboard the ISS, VEG-01B was grown a year later than VEG-01A, which raises the possibility of a change in the ISS water, but that may not be the only variable as plants grown in microgravity experience stresses from environmental conditions, so it would be impractical to draw any conclusions from these differences. VEG-03A, which was grown 1.5 years after the VEG-01B, was the sequential harvest experiment. As described in section “Materials and Methods,” there were four repeated harvests (38, 45, 59, and 64 days after initiation) of this experiment and only two (day 45 and day 64) were returned to earth. Romaine lettuce in field settings can take 75 days to fully mature, however, controlled environment growth is generally faster. By the final harvest photos indicated that some of the plants seemed to be transitioning to a reproductive growth phase, so it is possible that stresses from space flight may have pushed the plants to initiate senescence and that could provide a possible explanation for slightly lower elemental levels of some elements in VEG-03A samples ( Maillard et al., 2015 ).
Similarly, differences in the levels of total phenolics among VEG-01A, VEG-01B, and VEG-03A samples could be attributed to the environmental conditions. There have been known challenges associated with irrigation of plants in the Veggie hardware and VEG-01 showed evidence of insufficient and excess (in case of zinnia, VEG-01C) water in the root zone ( Massa et al., 2017a ). Studies have shown that either insufficient watering (drought-like, Sarker and Oba, 2018 ) or over-watering leading to hypoxia ( Rajapakse et al., 2009 ) can induce production of phenolic compounds and free-radical scavenging activities. Again, there are not enough data to make any conclusions about the effects of the sequential harvesting method on plant ionome or phenolic content and more robust experiments are needed.
Numerous Veggie tests have been conducted on the ISS, the plant growth evaluated, and in some cases the leaves consumed. Three plantings of red romaine lettuce were considered here (VEG-01A, VEG-01B, and VEG-03A). Through culturable and non-culturable methods of microbial analysis, the Veggie tests demonstrated diverse microbial communities with no potential human pathogens detected and therefore could provide a safe supplement to the astronauts’ diet. Chemical analysis provided evidence of significant changes in elemental and antioxidant content which may be an important factor to consider for nutritional value in future, long duration, exploration missions.
Data Availability Statement
The datasets generated for this study can be found in GeneLab accessions: VEG01A: GLDS-267, VEG01B: GLDS-268, and VEG03A: GLDS-269.
Author Contributions
GM, RW, MR, and TS contributed to the design of the work with MH, LS, JR, and CK completing research and data analysis. AD contributed to the data analysis. All authors contributed to the development of the manuscript and final version to be published.
Funding for the Veggie hardware technology validation tests was from NASA’s Space Life and Physical Sciences (SLPS) Space Biology Program.
Conflict of Interest
CK, MH, LS, AD, and JR are employed by AECOM Management Services, Inc., under the Laboratory Services and Support Contract at NASA, Kennedy Space Center, Merritt Island, FL, United States.
The remaining authors declare that the research was conducted in the absence of any commercial or financial relationships that could be construed as a potential conflict of interest.
Acknowledgments
We are very grateful to astronauts Steve Swanson, Scott Kelly, Kjell Lindgren, and Shane Kimbrough for their care of the plants in these growth tests. We would like to acknowledge the tremendous efforts of the many members of the VEG and Veggie teams at Kennedy Space Center, Marshall Space Flight Center, and Sierra Nevada Corp-ORBITEC in preparing, flying, operating and returning these experiments. Special thanks go to J. C. Carver, Chuck Spern, and Nicole Dufour for their excellent management of this project. We also wish to thank science team members Bob Morrow and Gerard Newsham for planning, preparation, and implementation of these crop tests. We also acknowledge the valuable assistance of interns Eric Barash and Jessica Scotten for assistance in the laboratory during sample processing and data analysis.
Supplementary Material
The Supplementary Material for this article can be found online at: https://www.frontiersin.org/articles/10.3389/fpls.2020.00199/full#supplementary-material
Adesina, M. F., Grosch, R., Lembke, A., Vatchev, T. D., and Smalla, K. (2009). In vitro antagonists of Rhizoctonia solani tested on lettuce: rhizosphere competence, biocontrol efficiency and rhizosphere microbial community response: research article. FEMS Microbiol. Ecol. 69, 62–74. doi: 10.1111/j.1574-6941.2009.00685.x
PubMed Abstract | CrossRef Full Text | Google Scholar
Anderson, M. J. (2001). A new method for non-parametric multivariate analysis of variance. Austral Ecol. 26, 32–46. doi: 10.1111/j.1442-9993.2001.01070.pp.x
CrossRef Full Text | Google Scholar
Bokulich, N. A., and Mills, D. A. (2013). Improved selection of internal transcribed spacer-specific primers enables quantitative, ultra-high-throughput profiling of fungal communities. Appl. Environ. Microbiol. 79, 2519–2526. doi: 10.1128/AEM.03870-12
Bolyen, E., Rideout, J. R., Dillion, M. R., Bokulich, N. A., Abnet, C., Al-Ghalith, G. A., et al. (2018). QIIME 2: Reproducible, interactive, scalable, and extensible microbiome data science. PeerJ Prepr. 6:e27295v2. doi: 10.7287/peerj.preprints.27295v2
Borchers, A. T., Keen, C. L., and Gershwin, M. E. (2002). Microgravity and immune responsiveness: implications for space travel. Nutrition 18, 890–898.
PubMed Abstract | Google Scholar
Braun, U., and Cook, R. T. A. (2012). Taxonomic manual of the erysiphales (Powdery Mildew). CBS Biodivers. Ser. 11, 1–707.
Google Scholar
Byrd, J. J., Xu, H., and Colwell, R. R. (1991). Viable but nonculturable bacteria in drinking water. Appl. Environ. Microbiol. 57, 875–878. doi: 10.1128/aem.57.3.875-878.1991
Castro, V. A., Thrasher, A. N., Healy, M., Ott, C. M., and Pierson, D. L. (2004). Microbial characterization during the early habitation of the international space station. Microb. Ecol. 47, 119–126. doi: 10.1007/s00248-003-1030-y
Chase, A. B., Arevalo, P., Polz, M. F., Berlemont, R., and Martiny, J. B. H. (2016). Evidence for ecological flexibility in the cosmopolitan genus Curtobacterium . Front. Microbiol. 7:1874. doi: 10.3389/fmicb.2016.01874
Checinska, A., Probst, A. J., Vaishampayan, P., White, J. R., Kumar, D., Stepanov, V. G., et al. (2015). Microbiomes of the dust particles collected from the international space station and spacecraft assembly facilities. Microbiome 3:50.
Cooper, M. R. (2013). Initial Nutritional Quality of ISS Space Food Provisions. Galveston, TX: NASA HRP Investigator’s Workshop.
Dees, M. W., Lysøe, E., Nordskog, B., and Brurberg, M. B. (2015). Bacterial communities associated with surfaces of leafy greens: shift in composition and decrease in richness over time. Appl. Environ. Microbiol. 81, 1530–1539. doi: 10.1128/AEM.03470-14
DeSantis, T. Z., Hugenholtz, P., Larsen, N., Rojas, M., Brodie, E. L., Keller, K., et al. (2006). Greengenes, a chimera-checked 16S rRNA gene database and workbench compatible with ARB. Appl. Environ. Microbiol. 72, 5069–5072. doi: 10.1128/aem.03006-05
Eberl, L., and Vandamme, P. (2016). Members of the genus Burkholderia : good and bad guys. F1000Res. 5:1007. doi: 10.12688/f1000research.8221.1
Epstein, E. (1972). Mineral Nutrition of Plants: Principles and Perspectives. New York: John Wiley and Sons, Inc.
FDA U.S. Food and Drug Administration (2018). FDA Bacteriological Analytical Manual FDA Bacteriological Anal. Man. Online with Update. Available online at: https://www.fda.gov/Food/FoodScienceResearch/LaboratoryMethods/ucm071363.htm (accessed September 18, 2018).
Fröhling, A., Rademacher, A., Rumpold, B., Klocke, M., and Schlüter, O. (2018). Screening of microbial communities associated with endive lettuce during postharvest processing on industrial scale. Heliyon 4:e00671. doi: 10.1016/j.heliyon.2018.e00671
Gislerød, H. R., and Mortensen, L. M. (1990). Relative humidity and nutrient concentration affect nutrient uptake and growth of Begonia× hiemalis . Hortscience 25, 524–526. doi: 10.21273/hortsci.25.5.524
Grady, E. N., MacDonald, J., Liu, L., Richman, A., and Yuan, Z. C. (2016). Current knowledge and perspectives of Paenibacillus : a review. Microb. Cell Fact. 15:203.
Hagenmair, R. D., and Baker, R. A. (2016). A survey of the microbial population and ethanol content of bagged salad. J. Food Prot. 61, 357–359. doi: 10.4315/0362-028x-61.3.357
Heaton, J. C., and Jones, K. (2008). Microbial contamination of fruit and vegetables and the behaviour of enteropathogens in the phyllosphere: a review. J. Appl. Microbiol. 104, 613–626. doi: 10.1111/j.1365-2672.2007.03587.x
Holvoet, K., Sampers, I., Seynnaeve, M., Jacxsens, L., and Uyttendaele, M. (2015). Agricultural and management practices and bacterial contamination in greenhouse versus open field lettuce production. Int. J. Environ. Res. Public Health 12, 32–63. doi: 10.3390/ijerph120100032
Hummerick, M., Garland, J., Bingham, G., Sychev, V., and Podolsky, I. (2010). “Microbiological analysis of Lada Vegetable Production Units (VPU) to define critical control points and procedures to ensure the safety of space grown vegetables,” in Proceedings of the International Conference on Environmental Systems , Boston, MA, 5277. doi: 10.2514/6.2010-6255
Hummerick, M., Garland, J., Bingham, G., Wheeler, R., Topham, S., Sychev, V., et al. (2011). A Hazard Analysis Critical Control Point Plan Applied to the Lada Vegetable production units (VPU) to Ensure the Safety of Space Grown Vegetables. Reston, VA: American Institute of Aeronautics and Astronautics.
Hummerick, M., Gates, J., Nguyen, B.-T., Massa, G., and Wheeler, R. (2012). “The effect of plant cultivar, growth media, harvest method and post harvest treatment on the microbiology of edible crops,” in Proceedings of the 42nd International Conference on Environmental Systems , Boston, MA, 1–10. doi: 10.2514/6.2012-3506
Ibekwe, A. M., and Grieve, C. M. (2004). Changes in developing plant microbial community structure as affected by contaminated water. FEMS Microbiol. Ecol. 48, 239–248. doi: 10.1016/j.femsec.2004.01.012
Jackson, C. R., Randolph, K. C., Osborn, S. L., and Tyler, H. L. (2013). Culture dependent and independent analysis of bacterial communities associated with commercial salad leaf vegetables. BMC Microbiol. 13:274. doi: 10.1186/1471-2180-13-274
Kadariya, J., Smith, T. C., and Thapaliya, D. (2014). Staphylococcus aureus and staphylococcal food-borne disease: an ongoing challenge in public health. Biomed Res. Int. 2014:827965. doi: 10.1155/2014/827965
Kalmbach, S., Manz, W., and Szewzyk, U. (1997). Isolation of new bacterial species from drinking water biofilms and proof of their in situ dominance with highly specific 16S rRNA probes. Appl. Environ. Microbiol. 63, 4164–4170. doi: 10.1128/aem.63.11.4164-4170.1997
Kim, H., Nishiyama, M., Kunito, T., Senoo, K., Kawahara, K., Murakami, K., et al. (1998). High population of Sphingomonas species on plant surface. J. Appl. Microbiol. 85, 731–736. doi: 10.1111/j.1365-2672.1998.00586.x
Kivlin, S. N., Hawkes, C. V., and Treseder, K. K. (2011). Global diversity and distribution of Arbuscular Mycorrhizal fungi. Soil Biol. Biochem. 43, 2294–2303. doi: 10.1016/j.soilbio.2011.07.012
Kliss, M., Hevenga, G., Hoehn, A., and Stodieck, L. (2000). Toward the Development of a “Salad Machine ”. SAE Technical Paper 2006–2308. Warrendale, PA: SAE International.
Koenig, D. W., and Pierson, D. L. (1997). Microbiology of the Space Shuttle water system. Water Sci. Tech. 35, 59–64. doi: 10.2166/wst.1997.0710
Kõljalg, U., Bahram, M., Kohout, P., Oja, J., Peterson, M., Põldmaa, K., et al. (2013). Towards a unified paradigm for sequence-based identification of fungi. Mol. Ecol. 22, 5271–5277. doi: 10.1111/mec.12481
Komaki, H., Hosoyama, A., Yabe, S., Yokota, A., Uchino, Y., and Takano, H. (2016). Draft genome sequence of Thermogemmatispora onikobensis NBRC 111776 T, an aerial mycelium- and spore-forming thermophilic bacterium belonging to the class ktedonobacteria. Genome Announc. 4:e01156-16. doi: 10.1128/genomeA.01156-16
Kozich, J. J., Westcott, S. L., Baxter, N. T., Highlander, S. K., and Schloss, P. D. (2013). Development of a dual-index sequencing strategy and curation pipeline for analyzing amplicon sequence data on the MiSeq illumina sequencing platform. Appl. Environ. Microbiol. 79, 5112–5120. doi: 10.1128/AEM.01043-13
Leff, J. W., and Fierer, N. (2013). Bacterial communities associated with the surfaces of fresh fruits and vegetables. PLoS One 8:e59310. doi: 10.1371/journal.pone.0059310
Li, Y., Uddin, W., and Kaminski, J. E. (2014). Effects of relative humidity on infection, colonization and conidiation of Magnaporthe orzyae on perennial ryegrass. Plant Pathol. 63, 590–597. doi: 10.1111/ppa.12127
Lindow, S. E., and Brandl, M. T. (2003). Microbiology of the phyllosphere MINIREVIEW microbiology of the phyllosphere. Appl. Environ. Microbiol. 69, 1875–1883. doi: 10.1128/AEM.69.4.1875
MacElroy, R. D., Kliss, M., and Straight, C. (1992). Life support systems for Mars transit. Adv. Space Res. 12, 159–166. doi: 10.1016/0273-1177(92)90022-p
Maduell, P., Armengol, G., Llagostera, M., Orduz, S., and Lindow, S. (2008). B. thuringiensis is a poor colonist of leaf surfaces. Microb. Ecol. 55, 212–219. doi: 10.1007/s00248-007-9268-4
Maignien, L., DeForce, E. A., Chafee, M. E., Eren, A. M., and Simmons, S. L. (2014). Ecological succession and stochastic variation in the assembly of Arabidopsis thaliana phyllosphere communities. mBio 5:e00682-13. doi: 10.1128/mBio.00682-13
Maillard, A., Diquelou, S., Billard, V., Laine, P., Garnica, M., Prudent, M., et al. (2015). Leaf mineral nutrient remobilization during leaf senescence and modulation by nutrient deficiency. Front. Plant Sci. 6:317. doi: 10.3389/fpls.2015.00317
Massa, G. D., Dufour, N. F., Carver, J. A., Hummerick, M. E., Wheeler, R. M., Morrow, R. C., et al. (2017a). Veggie hardware validation testing on the international space station. Open Agric. 2, 33–41.
Massa, G. D., Newsham, G., Hummerick, M. E., Morrow, R. C., and Wheeler, R. M. (2017b). Preparation for the veggie plant growth system on the international space station. Gravit. Space Res. 5, 24–34.
Massa, G. D., Wheeler, R. M., Morrow, R. C., and Levine, H. G. (2016). Growth chambers on the international space station for large plants. Acta Hortic. 1134, 215–222. doi: 10.17660/actahortic.2016.1134.29
McKeehen, J. D., Smart, D. J., Mackowiack, C. L., Wheeler, R. M., and Nielsen, S. S. (1996). Effect of CO2 levels on nutrient content of lettuce and radish. Adv. Space Res. 18, 85–92. doi: 10.1016/0273-1177(95)00864-b
Meeboon, J., and Takamatsu, S. (2017). Microidium phyllanthi-reticulati sp. nov. on Phyllanthus reticulatus . Mycotaxon 132, 289–297. doi: 10.5248/132.289
Morrow, R. C., and Remiker, R. W. (2009). A Deployable Salad Crop Production System for Lunar Habitats 2009-01-2382. SAE Technical Paper 2009-01-2382. Warrendale, PA: SAE International.
Morrow, R. C., Remiker, R. W., Mischnick, M. J., Tuominen, L. K., Lee, M. C., and Crabb, T. M. (2005). A Low Equivalent System Mass Plant Growth Unit for Space Exploration. SAE Tech. Pap. 2005-01–28. Warrendale, PA: SAE International.
Mostert, L., Groenewald, J. Z., Summerbell, R. C., Robert, V., Sutton, D. A., Padhye, A. A., et al. (2005). Species of Phaeoacremonium associated with infections in humans and environmental reservoirs in infected woody plants. J. Clin. Microbiol. 43, 1752–1767. doi: 10.1128/JCM.43.4.1752-1767.2005
Okubo, T., Tsukui, T., Maita, H., Okamoto, S., Oshima, K., Fujisawa, T., et al. (2012). Complete genome sequence of Bradyrhizobium sp. s23321: insights into symbiosis evolution in soil oligotrophs. Microbes Environ. 27, 306–315. doi: 10.1264/jsme2.me11321
Oliveira, M., Usall, J., Viñas, I., Anguera, M., Gatius, F., and Abadias, M. (2010). Microbiological quality of fresh lettuce from organic and conventional production. Food Microbiol. 27, 679–684. doi: 10.1016/j.fm.2010.03.008
Oyinlola, L. A., Obadina, A. O., Omemu, A. M., and Oyewole, O. B. (2017). Prevention of microbial hazard on fresh-cut lettuce through adoption of food safety and hygienic practices by lettuce farmers. Food Sci. Nutr. 5, 67–75. doi: 10.1002/fsn3.365
Panstruga, R., and Kuhn, H. (2019). Mutual interplay between phytopathogenic powdery mildew fungi and other microorganisms. Mol. Plant Pathol. 20, 463–470. doi: 10.1111/mpp.12771
Patriarca, A. (2016). Alternaria in food products. Curr. Opin. Food Sci. 11, 1–9. doi: 10.1016/j.cofs.2016.08.007
Perchonok, M., Douglas, G., and Cooper, M. (2012). Risk of Performance Decrement and Crew Illness Due to an Inadequate Food System. HRP Evidence Book. NASA Human Research Program. Available online at: http://humanresearchroadmap.nasa.gov/Evidence/reports/Food.pdf
Peredo, E. L., and Simmons, S. L. (2018). Leaf-FISH: microscale imaging of bacterial taxa on phyllosphere. Front. Microbiol. 8:2669. doi: 10.3389/fmicb.2017.02669
Prior, R. L., Wu, X., and Schaich, K. (2005). Standard methods for the determination of antioxidant capacity and phenolics in food and dietary supplements. J. Agric. Food Chem. 53, 4619–4626.
Qadri, O. S., Yousuf, B., and Srivastava, A. K. (2015). Fresh-cut fruits and vegetables: critical factors influencing microbiology and novel approaches to prevent microbial risks. A review. Cogent Food Agric. 1:1121606. doi: 10.1080/23311932.2015.1121606
Rajapakse, N. C., He, C., Cisneros-Zevallos, L., and Davies, F. T. (2009). Hypobaria and hypoxia affects growth and phytochemical contents of lettuce. Sci. Horticul. 122, 171–178. doi: 10.1016/j.scienta.2009.05.002
Rastogi, G., Tech, J. J., Coaker, G. L., Leveau, J. H. J., Sbodio, A., and Suslow, T. V. (2012). Leaf microbiota in an agroecosystem: spatiotemporal variation in bacterial community composition on field-grown lettuce. ISME J. 6, 1812–1822. doi: 10.1038/ismej.2012.32
Richards, J. T., Edney, S. L., Yorio, N. C., Stutte, G. W., Cranston, N., Wheeler, R. M., et al. (2004). “Effects of lighting intensity and supplemental CO2 on yield of potential salad crops for ISS,” in Proceedings of the International Conference on Environmental Systems , Barcelona.
Richards, J. T., Edney, S. L., Yorio, N. C., Stutte, G. W., and Wheeler, R. M. (2006). “Yields of salad crops grown under potential lunar or Mars habitat environments: effect of temperature and lighting intensities,” in Proceedings of the International Conference on Environmental Systems , Boston, MA.
Riser, E. C., Grabowski, J., and Glenn, E. P. (1984). Microbiology of hydroponically-grown lettuce. J. Food Prot. 47, 765–769. doi: 10.4315/0362-028X-47.10.765
Royer, J. C., Madden, K. T., Norman, T. C., and Lobuglio, K. F. (2004). Applied mycology & biotechnology an international series. Fungal Genomics 4:9.
Ryan, M. P., Pembroke, J. T., and Adley, C. C. (2007). Ralstonia pickettii in environmental biotechnology: potential and applications. J. Appl. Microbiol. 103, 754–764. doi: 10.1111/j.1365-2672.2007.03361.x
Sarker, U., and Oba, S. (2018). Drought stress enhances nutritional and bioactive compounds, phenolic acids and antioxidant capacity of Amaranthus leafy vegetable. BMC Plant Biol. 18:258. doi: 10.1186/s12870-018-1484-1
Schaule, G., Flemming, H. C., and Ridgway, H. F. (1993). Use of 5-cyano-2,3-ditolyl tetrazolium chloride for quantifying planktonic and sessile respiring bacteria in drinking water. Appl. Environ. Microbiol. 59, 3850–3857. doi: 10.1128/aem.59.11.3850-3857.1993
Scheublin, T. R., and Leveau, J. H. J. (2013). Isolation of Arthrobacter species from the phyllosphere and demonstration of their epiphytic fitness. Microbiologyopen 2, 205–213. doi: 10.1002/mbo3.59
Shaffer, J. P., U’Ren, J. M., Gallery, R. E., Baltrus, D. A., and Arnold, A. E. (2017). An endohyphal bacterium (Chitinophaga, Bacteroidetes) alters carbon source use by Fusarium keratoplasticum ( F. solani species complex, Nectriaceae). Front. Microbiol. 8:350. doi: 10.3389/fmicb.2017.00350
Sublett, W., Barickman, T., and Sams, C. (2018). The Effect of environment and nutrients on hydroponic lettuce yield, quality, and phytonutrients. Horticulturae 4:48. doi: 10.3390/horticulturae4040048
Talley, S. M., Coley, P. D., and Kursar, T. A. (2002). The effects of weather on fungal abundance and richness among 25 communities in the Intermountain West. BMC Ecol. 2:7. doi: 10.1186/1472-6785-2-7
Tibbitts, T. W. (1979). Humidity and plants. Bioscience 29, 358–363. doi: 10.2307/1307692
Toju, H., Kurokawa, H., and Kenta, T. (2019). Factors influencing leaf- and root-associated communities of bacteria and fungi across 33 plant orders in a grassland. Front. Microbiol. 10:241. doi: 10.3389/fmicb.2019.00241
Valentin-Bon, I., and Jacobson, A. (2008). Microbiological quality of bagged cut spinach and lettuce mixes. Appl. Environ. Microbiol. 74, 1240–1242. doi: 10.1128/aem.02258-07
Venkateswaran, K., Checinska, A., Singh, N., Mohan, G. B., Urbaniak, C., Blachowicz, A., et al. (2017). “Microbial characteristics of ISS environment surfaces,” in Proceedings of the 47th International Conference on Environmental Systems , Charleston, 131.
Venkateswaran, K., Vaishampayan, P., Cisneros, J., Pierson, D. L., Rogers, S. O., and Perry, J. (2014). International space station environmental microbiome - microbial inventories of ISS filter debris. Appl. Microbiol. Biotechnol. 98, 6453–6466. doi: 10.1007/s00253-014-5650-6
Verma, V. C., Gond, S. K., Kumar, A., Kharwar, R. N., Boulanger, L., and Strobel, G. A. (2011). Endophytic fungal flora from roots and fruits of an Indian neem plant Azadirachta indica A. Juss., and impact of culture media on their isolation. Indian J. Microbiol. 51, 469–476. doi: 10.1007/s12088-011-0121-6
Vokou, D., Vareli, K., Zarali, E., Karamanoli, K., Constantinidou, H. I. A., Monokrousos, N., et al. (2012). Exploring biodiversity in the bacterial community of the Mediterranean phyllosphere and its relationship with airborne bacteria. Microb. Ecol. 64, 714–724. doi: 10.1007/s00248-012-0053-7
Wheeler, R. M., Mackowiack, C. L., Stutte, G. W., Yorio, N. C., and Berry, W. L. (1997). Effect of elevated carbon dioxide on nutritional quality of tomato. Adv. Space Res. 20, 1975–1978. doi: 10.1016/s0273-1177(97)00263-9
Wheeler, R. M., Mackowiak, C. L., Sager, J. C., and Knott, W. M. (1996). Proximate composition of CELSS crops grown in NASA’s biomass production chamber. Adv. Space Res. 18, 43–47. doi: 10.1016/0273-1177(95)00860-h
Whipps, J. M., Hand, P., Pink, D., and Bending, G. D. (2008). Phyllosphere microbiology with special reference to diversity and plant genotype. J. Appl. Microbiol. 105, 1744–1755. doi: 10.1111/j.1365-2672.2008.03906.x
Wood, J. L., Chen, J. C., Friesen, E., Delaquis, P., and Allen, K. J. (2015). Microbiological survey of locally grown lettuce sold at farmers’ markets in Vancouver, British Columbia. J. Food Prot. 78, 203–208. doi: 10.4315/0362-028X.JFP-14-199
Wu, X., Gu, L., Prior, L., and McKay, S. (2004). Characterization of anthocyanins and proanthocyanidins in some cultivars of Ribes, Aronia, and Sambucus and their antioxidant capacity. J. Agric. Food Chem. 52, 7846–7856. doi: 10.1021/jf0486850
Yamaguchi, N., Roberts, M., Castro, S., Oubre, C., Makimura, K., Leys, N., et al. (2014). Microbial monitoring of crewed habitats in space-current status and future perspectives. Microbes Environ. 29, 250–260. doi: 10.1264/jsme2.ME14031
Zhang, G., Chen, Y., Hu, L., Melka, D., Wang, H., Laasri, A., et al. (2018). Survey of foodborne pathogens, aerobic plate counts, total coliform counts, and Escherichia coli counts in leafy greens, sprouts, and melons marketed in the United States. J. Food Prot. 81, 400–411. doi: 10.4315/0362-028X.JFP-17-253
Keywords : lettuce, microgravity, ISS, phyllosphere, rhizosphere, space, microbes
Citation: Khodadad CLM, Hummerick ME, Spencer LE, Dixit AR, Richards JT, Romeyn MW, Smith TM, Wheeler RM and Massa GD (2020) Microbiological and Nutritional Analysis of Lettuce Crops Grown on the International Space Station. Front. Plant Sci. 11:199. doi: 10.3389/fpls.2020.00199
Received: 17 October 2019; Accepted: 11 February 2020; Published: 06 March 2020.
Reviewed by:
Copyright © 2020 Khodadad, Hummerick, Spencer, Dixit, Richards, Romeyn, Smith, Wheeler and Massa. This is an open-access article distributed under the terms of the Creative Commons Attribution License (CC BY) . The use, distribution or reproduction in other forums is permitted, provided the original author(s) and the copyright owner(s) are credited and that the original publication in this journal is cited, in accordance with accepted academic practice. No use, distribution or reproduction is permitted which does not comply with these terms.
*Correspondence: Gioia D. Massa, [email protected]
Disclaimer: All claims expressed in this article are solely those of the authors and do not necessarily represent those of their affiliated organizations, or those of the publisher, the editors and the reviewers. Any product that may be evaluated in this article or claim that may be made by its manufacturer is not guaranteed or endorsed by the publisher.
- Share full article
Advertisement
Supported by
Guest Essay
Liz Truss Is Coming for America

By Tanya Gold
Ms. Gold is a British journalist who has written for Harper’s Magazine, The Spectator and UnHerd.
Liz Truss was the prime minister of Britain for 49 days in 2022, an interregnum between Boris Johnson and Rishi Sunak that was so short, it was outlasted by a lettuce . In the annals of British decline, she will be remembered for having been in office just three days when Queen Elizabeth II died and for her plan for an enormous and apparently unfunded tax cut, which she abruptly dropped after a run on the pound.
If this were the 19th century, Ms. Truss would have perhaps exiled herself to a country estate where peacocks roamed the grounds or fought her enemies with pistols. (In 1809 the foreign secretary, George Canning, was wounded in a duel with the war minister.) But this is not a time of penance or honor. Instead she has reinvented herself as a populist and has a new book, “ Ten Years to Save the West : Leading the Revolution Against Globalism, Socialism, and the Liberal Establishment,” which is part memoir, part pitch to the American right: She has seen the deep state up close and knows what needs to be done.
This is not Ms. Truss’s first political transformation. She began her career as an anti-monarchy member of the centrist Liberal Democrats, before transmogrifying into an uneasy Margaret Thatcher tribute act. She voted to remain in the European Union and then remade herself as a champion of Brexit. She survived every government from 2012 until her own. As environment secretary, she got memorably angry about cheese — “We import two-thirds of our cheese. That. Is. A. Disgrace” — but was never really considered a likely leader of the Conservative Party until her predecessor Mr. Johnson almost burned the party down .
When she did get her turn and tried to execute her vision of a low-tax, low-regulation, high-growth Britain, it did not go well. After she announced her economic program, the pound sank, interest rates shot up, and the Bank of England had to intervene . Abandoning a central plank of the plan was not enough to mollify her critics, and she resigned soon after. (Even Mr. Canning, who survived his wounds and eventually became prime minister, lasted longer. He died of pneumonia after 119 days.)
People deal with public failure in different ways. For Ms. Truss, the method seems to be twofold. First, to insist that she was and is right but was foiled by the deep state. Second, to see if America might buy what she’s selling.
Last April she gave the Margaret Thatcher Freedom Lecture at the Heritage Foundation in Washington, D.C., where she sketched out how she was foiled by the establishment. “I simply underestimated the scale and depth of this resistance and the scale and depth to which it reached into the media and into the broader establishment,” she said. The anti-growth movement — in which she seems to include President Biden, the I.M.F., the British Treasury and the Bank of England, among others — is “focused on redistributionism, on stagnation and on the imbuing of woke culture into our businesses.”
This year, in February, she told the Conservative Political Action Conference in Maryland that “the West has been run by the left for too long, and we’ve seen that it’s been a complete disaster.” (The Conservatives have governed Britain for 14 years.) Real conservatives, she said, “are now operating in what is a hostile environment. We essentially need a bigger bazooka in order to be able to deliver.” While at CPAC, she also spoke to Steve Bannon, whom she invited to “come over to Britain and sort out Britain,” and told Nigel Farage that she “felt safer for the West” when Donald Trump was president.
And now here is “Ten Years to Save the West,” which in title seems squarely aimed at America but in content often feels oddly parochial. Ms. Truss writes of traveling to Balmoral to accept Elizabeth II’s invitation to form a government. Here, Elizabeth II is a soothsayer. “She warned me that being prime minister is incredibly aging. She also gave me two words of advice: ‘Pace yourself.’ Maybe I should have listened.”
She is not sure that the flat above Downing Street “would be rated well on Airbnb.” It felt “a bit soulless,” she writes. It was apparently infested with fleas. And she couldn’t sleep because of the noise, including the clock at nearby Horse Guards, which chimed every quarter-hour. Picturing her flea-bitten and exhausted made me think of a line from Thackeray’s “Vanity Fair” — “Which of us has his desire? or, having it, is satisfied ?”
By the time she got to her resignation, she writes, it “seemed like just another dramatic moment in a very strange film in which I had somehow been cast,” which, to me, felt like truth.
It’s unclear whether Ms. Truss will be able to read an American room any better than a British one. In the book, she describes America as Britain’s “proudest creation, albeit an unintentional one,” and she is critical of Mr. Biden, who called her tax cuts for the wealthy a “ mistake .” “This was utter hypocrisy and ignorance,” she writes. She notes, on the other hand, that she was an early fan of “The Apprentice” and enjoyed Mr. Trump’s “catchphrases and sassy business advice.”
But Americans who fear the deep state aren’t necessarily the ones who want a small one, and Ms. Truss is a poor public speaker. I’d expect conservative Americans to see her as a curio and move on to more familiar and charismatic icons. But one never knows.
Who is to blame for Liz Truss? Maybe it was the winds of history. Or a political system that rewards risk takers and narcissism. Or it was 14 years of one party in power, at the end of which are the people who hung on long enough.
Or it was Mr. Johnson, who made her foreign secretary in his government. (He is now a tabloid columnist writing about his late-night chorizo binges and how much he loves his lawn mower , so he has nothing to laugh about, either.)
The Conservative Party is packing for the wilderness. Many lawmakers are not even standing in the forthcoming election, which must be held by January. And Ms. Truss herself may lose her seat, in Norfolk, to James Bagge, who is standing as an independent. Mr. Bagge is part of a local cohort concerned about issues like the National Health Service and the cost of living and unimpressed by Ms. Truss’s globe-trotting. “Truss says she has 10 years to save the West,” he recently told The London Times. “Well, we have six months to save Norfolk.”
I wonder whether Ms. Truss is coming for America because her enemies in Norfolk are coming for her. The Conservative endgame is here. The land of opportunity beckons.
Tanya Gold is a British journalist.
Photographs by Jose Luis Magana/Associated Press and RunPhoto, Issaraway Tattong, Cathering Fall Commerical, Flashpop, posteriori, and Carl Court/Getty Images.
The Times is committed to publishing a diversity of letters to the editor. We’d like to hear what you think about this or any of our articles. Here are some tips . And here’s our email: [email protected] .
Follow the New York Times Opinion section on Facebook , Instagram , TikTok , WhatsApp , X and Threads .
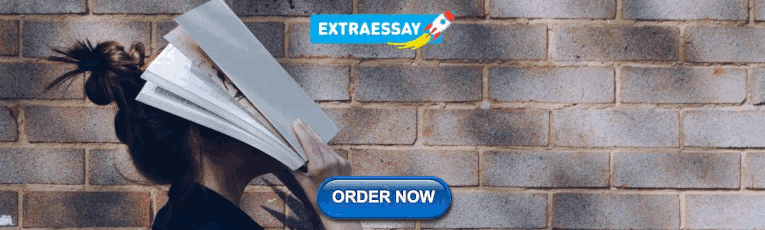
IMAGES
VIDEO
COMMENTS
The lettuce grown in soil has shown the lowest mass (108.8g/plant) of all the. growing media. Lettuce grown in coco coir had the greatest yield in terms of growth. Lettuce grown in peat potting ...
PDF | On Jun 1, 2017, M. R. Hasan and others published Growth and Yield of Lettuce (Lactuca Sativa L.) Influenced As Nitrogen Fertilizer and Plant Spacing | Find, read and cite all the research ...
Production of safe food in the densely populated areas of the developing countries is the most challenging issue due to the speedy urbanization, fragile food transportation facilities, and reduced farmlands. Given this background, a study was conducted to evaluate the agronomic properties and economic viability of lettuce grown vertically in the wall of building in Dhaka city, Bangladesh. Two ...
Lettuce is the second most popular vegetable crop in the U.S., behind only potatoes (USDA-ERS 2017b) In 2016, the USDA reports that 281,700 acres of head, leaf, and romaine type lettuce was harvested in the U.S. (USDA-NASS 2016), Greenhouse lettuce production is less expansive than field lettuce production with nearly 40 acres of greenhouse
thesis was to determine the effects of environmental conditions and horticultural management strategies on romaine lettuce grown hydroponically in a controlled environment, in order to optimize yield, morphological and nutritional outcomes. Controlled Environment Agriculture Overview
PDF | On Jun 1, 2018, Z.C. Gonzaga and others published Growth and yield of lettuce ( Lactuca sativa L.) grown under two types of cultivation systems as influenced by different organic soil ...
Lettuce is a cool-season vegetable, and they can grow vigorously at temperature from 7 to 24 °C (Sublett et al., 2018). According to Cornell hydroponic lettuce handbook, the suggested pH range is from 5.6-6.0 for CEA lettuce production and 5.8 is recommended as the optimal pH level for lettuce growth (Brechner et al., 2013), and
Lettuce (Lactuca sativa L.) belongs to the Asteraceae family and is rich in fiber, vitamins, minerals, and phenolic compounds [].In 2019, lettuce production reached 29,134,653 tons in the world from which about 547,590 tons were produced in Iran [].Considering the important and valuable nutritional role of lettuce due to its daily consumption and, on the other hand, the excessive application ...
Plant Material and Growth Condition. Lettuce (Lactuca sativa cv. 'Tiberius') seeds were sown in sponge blocks filled in plastic trays.Seedlings were developed under fluorescent lamps (TL-D56W, Osram, Munich, Germany) with a light intensity of 150 μmol m −2 s −1 and a photoperiod of 16 h day −1.Upon emerging three true leaves (15 days after sowing), seedlings were transplanted in a ...
Key words : Growth medium, lettuce, planting density, soilless culture INTRODUCTION Lettuce (Lactuca sativa) is one among the most consumed vegetables across the world. China remains the top producer with 9,189,523.81 tonnes of lettuce in the year 2015 (FAO, 2015). Lettuce is a widely consumed vegetable in Europe and North America.
Lettuce physiological disorders (tipburn and bolting) Tipburn reduces marketability and in some cases renders lettuce unsellable. It is a common physiological disorder of lettuce, possibly caused by various environmental factors, in field production and greenhouse hydroponics and aeroponics (Holmes et al., 2019). Tipburn is induced by a calcium ion
Lettuce (Lactuca sativa L.) is a major fresh vegetable and its leaves are commonly found in salad mixtures and sandwiches. In some eastern countries like China and Egypt, stems instead of leaves of lettuce are consumed, either cooked, raw, pickled, dried, or as a sauce. Some less common uses for lettuce include a cigarette without
The materials used were seeds of lettuce (Lettuce sativa L) on Oxisol soil types, with the total N content of 0.18 %, P (18 mg. g-1), K (23 mg. g-1) and C (1.52 %) were utilized. The organic fertilizers applied were cow-, sheep-, and guano manures, and compost and green manure (Leucaena leucocephala).
BS Thesis, Benguet State University, La Trinidad, Benguet. Pp. 1-5. [3] ... In BC/SB mixes, GI of lettuce grown in mixes with BC was similar to that of plants in 100% SB, and plants grown in mixes ...
Whole-genome resequencing of 445 Lactuca accessions, including major lettuce crop types and wild relative species, provides a comprehensive map of lettuce genome variations and sheds light on the ...
1 INTRODUCTION Background of the study Lettuce (Lactuca sativa L.) is the most widely grown of all green leaf salad vegetables and it is important vegetable according to the highest consumption rate and economics importance through the world. It is an important leafy vegetable crop and it is considered as an excellent nutritive source of minerals and vitamins since it is consume as fresh green ...
Lettuce (Lactuca sativa L.) is a domestic annual species in the family Asteraceae (Compositae) cultivated mainly for its fleshy leaves.It forms within the genus Lactuca a somewhat isolated genetic compatibility group with three wild species that also have nine pairs of chromosomes, L. serriola, L. saligna and L. virosa (Thompson et al. 1941; Thompson 1943).
Keywords - Guano char, Lettuce, Residual Effect, Pot experiment INTRODUCTION Lettuce is commonly known as lechugas which is native to Southern Europe and Western Asia. In the Philippines lettuce is considered as one of the most important salad crops and also used as garnishing for other food preparations. It is rich in vitamins B-
Lettuce ( Lactuca sativa L.) is a member of the fam-. ily Asteraceae (Compositae), a succ essful and diverse. group of plants with a global distribution (Funk et al. 2005). The Asteraceae are ...
Lettuce above- and below-ground growth were significantly reduced across replicates treated with a pre-existing aquaponic system inoculum when compared to replicates treated with a commercial inoculum. Reduced productivity was associated with enrichment in specific bacterial genera in plant roots, including Pseudomonas, following inoculum ...
Introduction. Crop production in space may be a necessary and desirable component of future exploration systems (MacElroy et al., 1992; Kliss et al., 2000).Fresh produce can be grown in situ to supplement a stored, packaged diet, and crops may provide beneficial nutrients as well as dietary variety. Veggie is a small plant growth chamber designed and built by Orbital Technologies Corporation ...
Growth and physiological parameters of lettuce were measured in this experiment. The maximum number of leaves per plant (21.44) and the highest fresh weight (92.49 g plant-1) were recorded from M1 ...
Liz Truss was the prime minister of Britain for 49 days in 2022, an interregnum between Boris Johnson and Rishi Sunak that was so short it was outlasted by a lettuce.In the annals of British ...
Red Oak cultivar of lettuce (Lactuca sativa L.) was planted in hydroponics with nutrient film technique (NFT) to study the effects of different color shaded net and no shaded net on growth and yield.