Grasslands Explained
Savanna, steppe, prairie, or pampas: They're all grasslands, the globe's most agriculturally useful habitats.
Biology, Ecology, Conservation, Earth Science, Climatology
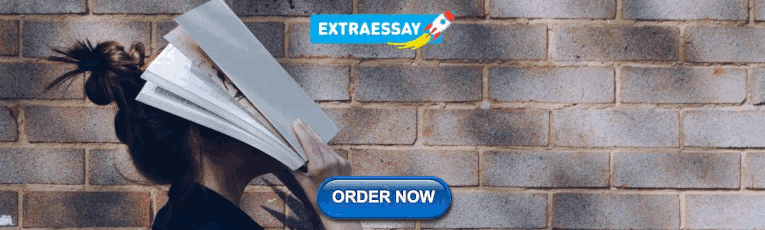
Little Missouri National Grassland
Grasslands, like the Little Missouri National Grassland in the United States, fill the ecological niche between forests and deserts, often bordering the two.
Photograph by Phil Schermeister
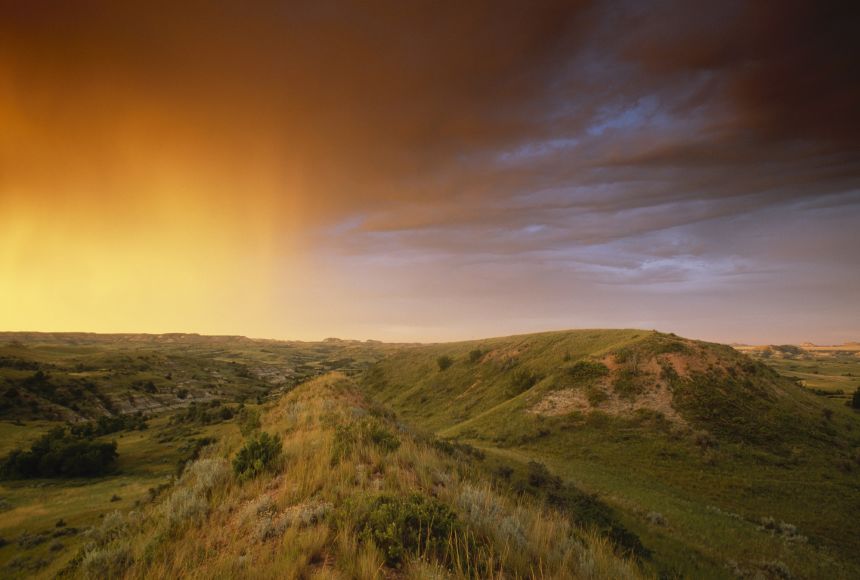
Grasslands go by many names. In the United States Midwest, they're often called prairies . In South America, they're known as pampas . Central Eurasian grasslands are referred to as steppes , while African grasslands are savannas . What they all have in common are grasses, their naturally dominant vegetation. Grasslands are found where there is not enough regular rainfall to support the growth of a forest, but not so little that a desert forms. In fact, grasslands often lie between forests and deserts. Depending on how they’re defined, grasslands account for between 20 and 40 percent of the world's land area. They are generally open and fairly flat, and they exist on every continent except Antarctica, which makes them vulnerable to pressure from human populations. Threats to natural grasslands, as well as the wildlife that live on them, include farming, overgrazing, invasive species, illegal hunting, and climate change . At the same time, grasslands could help mitigate climate change: One study found California's grasslands and rangelands could store more carbon than forests because they are less susceptible to wildfires and drought. Still, only a small percentage—less than 10 percent—of the world's grassland is protected. Types of Grasslands There are two main kinds of grasslands: tropical and temperate . Examples of temperate grasslands include Eurasian steppes, North American prairies, and Argentine pampas. Tropical grasslands include the hot savannas of sub-Saharan Africa and northern Australia. Rainfall can vary across grasslands from season to season and year to year, ranging from 25.4 too 101.6 centimeters (10 to 40 inches) annually. Temperatures can go below freezing in temperate grasslands to above 32.2 degrees Celsius (90 degrees Fahrenheit). The height of vegetation on grasslands varies with the amount of rainfall. Some grasses might be under 0.3 meters (one foot) tall, while others can grow as high as 2.1 meters (seven feet). Their roots can extend 0.9 to 1.8 meters (three to six feet) deep into the soil. The combination of underground biomass with moderate rainfall—heavy rain can wash away nutrients—tends to make grassland soils very fertile and appealing for agricultural use. Much of the North American prairielands have been converted into land for crops, posing threats to species that depend on those habitats, as well as drinking water sources for people who live nearby. Grassland Plants and Animals Grasslands support a variety of species. Vegetation on the African savannas, for example, feeds animals including zebras, wildebeest, gazelles, and giraffes. On temperate grasslands, you might find prairie dogs, badgers, coyotes, swift foxes, and a variety of birds. There can be up to 25 species of large plant-eaters in a given grassland habitat, comprising a sort of buffet where different grasses appeal to different species. Some grass species in these habitats include red oat grass ( Themeda triandra ) and Rhodes grass ( Chloris gayana ) in tropical savannas, and purple needlegrass ( Nassella pulchra ) and galleta in temperate areas. When rainy season arrives, many grasslands become coated with wildflowers such as yarrow ( Achiella millefolium ), hyssop, and milkweed. The plants on grasslands have adapted to the drought, fires, and grazing common to that habitat. Fires, both natural and human-caused, are important factors shaping grasslands. In the U.S. Midwest, for example, Native Americans set fires to help maintain grasslands for game species, such as bison. Fire can also help prevent fire-intolerant trees and shrubs from taking over while increasing the diversity of wildflowers that support pollinators.
Media Credits
The audio, illustrations, photos, and videos are credited beneath the media asset, except for promotional images, which generally link to another page that contains the media credit. The Rights Holder for media is the person or group credited.
Production Managers
Program specialists, last updated.
October 19, 2023
User Permissions
For information on user permissions, please read our Terms of Service. If you have questions about how to cite anything on our website in your project or classroom presentation, please contact your teacher. They will best know the preferred format. When you reach out to them, you will need the page title, URL, and the date you accessed the resource.
If a media asset is downloadable, a download button appears in the corner of the media viewer. If no button appears, you cannot download or save the media.
Text on this page is printable and can be used according to our Terms of Service .
Interactives
Any interactives on this page can only be played while you are visiting our website. You cannot download interactives.
Related Resources
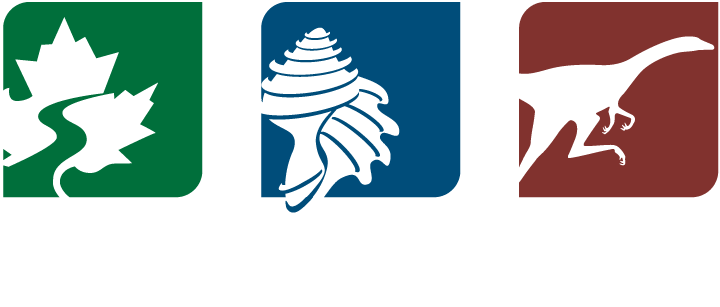
- Introduction
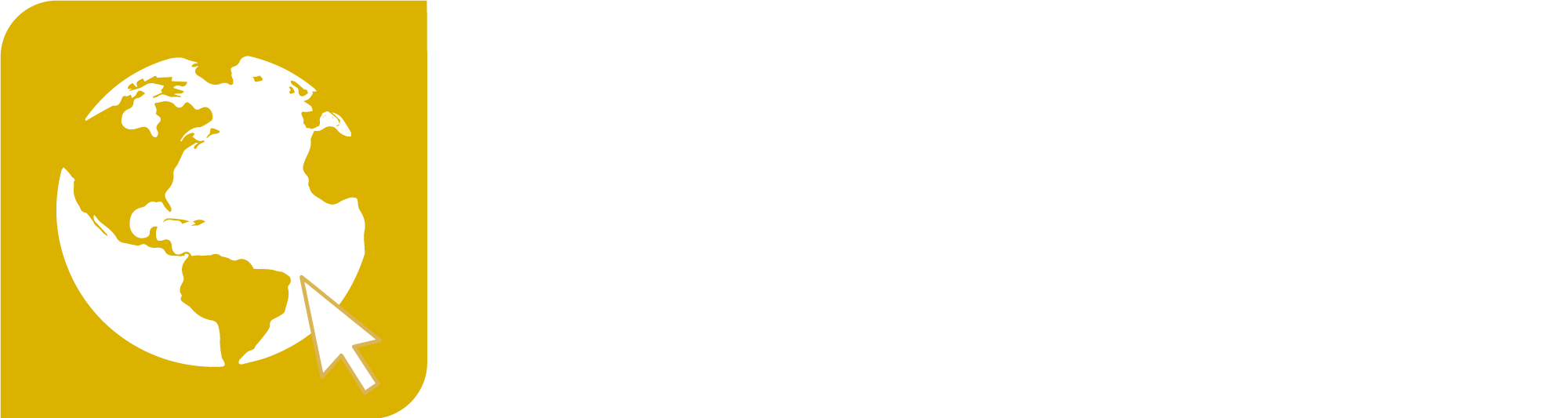
Overview of global grassland ecosystems
Page snapshot: An introduction to temperate and tropical grass-dominated biomes and the threats that they currently face.
Topics covered on this page: What are grasslands? ; Why are grasslands located where they are? ; What are the threats to grasslands? ; Resources .
Credits: Funded by the National Science Foundation. Any opinions, findings, and conclusions or recommendations expressed in this material are those of the author(s) and do not necessarily reflect the views of the National Science Foundation. Page by Naomi Schulberg (2023).
Updates: Page last updated August 31, 2023.
Image above: Savanna in the LUMO Community Wildlife Sanctuary, Kenya. Photo by Christopher T Cooper (Wikimedia Commons , Creative Commons Attribution 3.0 Unported license ).
What are grasslands?
Ecosystems in which grass is the dominant vegetation ( grasslands ) are found on every continent besides Antarctica. They are found in areas that are both too dry to support forests and too wet to support deserts. They are maintained by various environmental factors, including grazing, fires, and seasonal drought.
Different names are used to describe grasslands in different regions. In North America, grasslands are called prairies . In South America, grasslands are referred to as pampas and cerrados. In Africa, they are savannas and veldts ; in Australia, they are downs ; and in Asia and Europe, they are steppes . Different grasslands support different amounts of non-grass vegetation. For example, savannas generally have scattered trees, whereas trees are rarer in steppes and prairies.
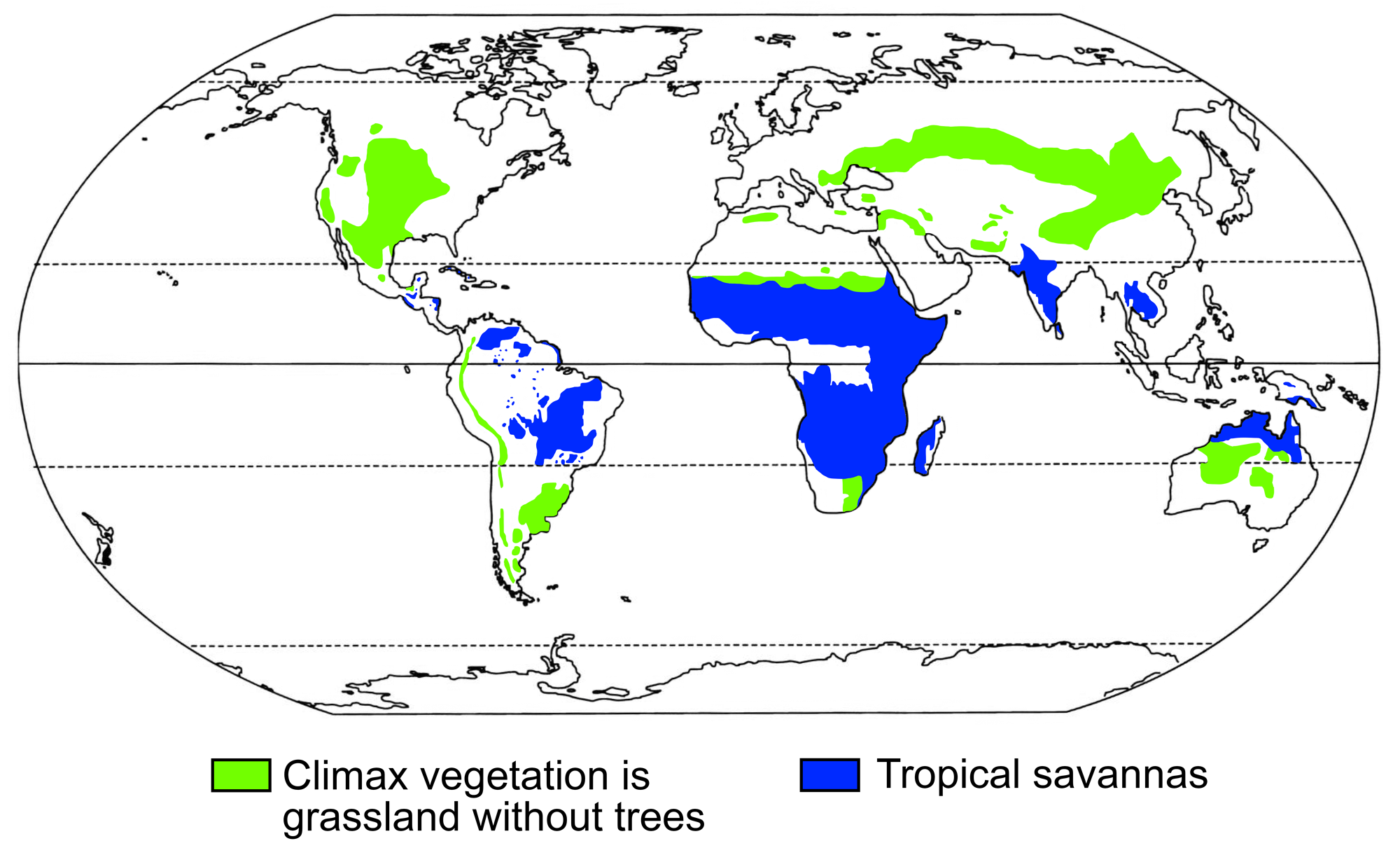
Modern distribution of grasslands (meaning biomes in which the climax vegetation is grass-dominated and lacks tress) and tropical savannas. Modified from figure 1 in Jacobs et al. (1999) Annals of the Missouri Botanical Garden 86: 590-643 (Biodiversity Heritage Library , Creative Commons-NonCommercial-ShareAlike 3.0 Unported license , image cropped, resized, reshaded and relabeled).
Grasslands can broadly be classified as temperate grasslands and tropical grasslands. Tropical grasslands are found between 30ºN and 30ºS of the equator. In tropical grasslands, temperatures do not drop below freezing, and there are distinct wet and dry seasons. Levels of rainfall range between 50 to 130 centimeters (about 20 to 51 inches) per year. Tropical grasslands include African and Australian tropical savannas, Indian savannas and grasslands, and South American cerrado.
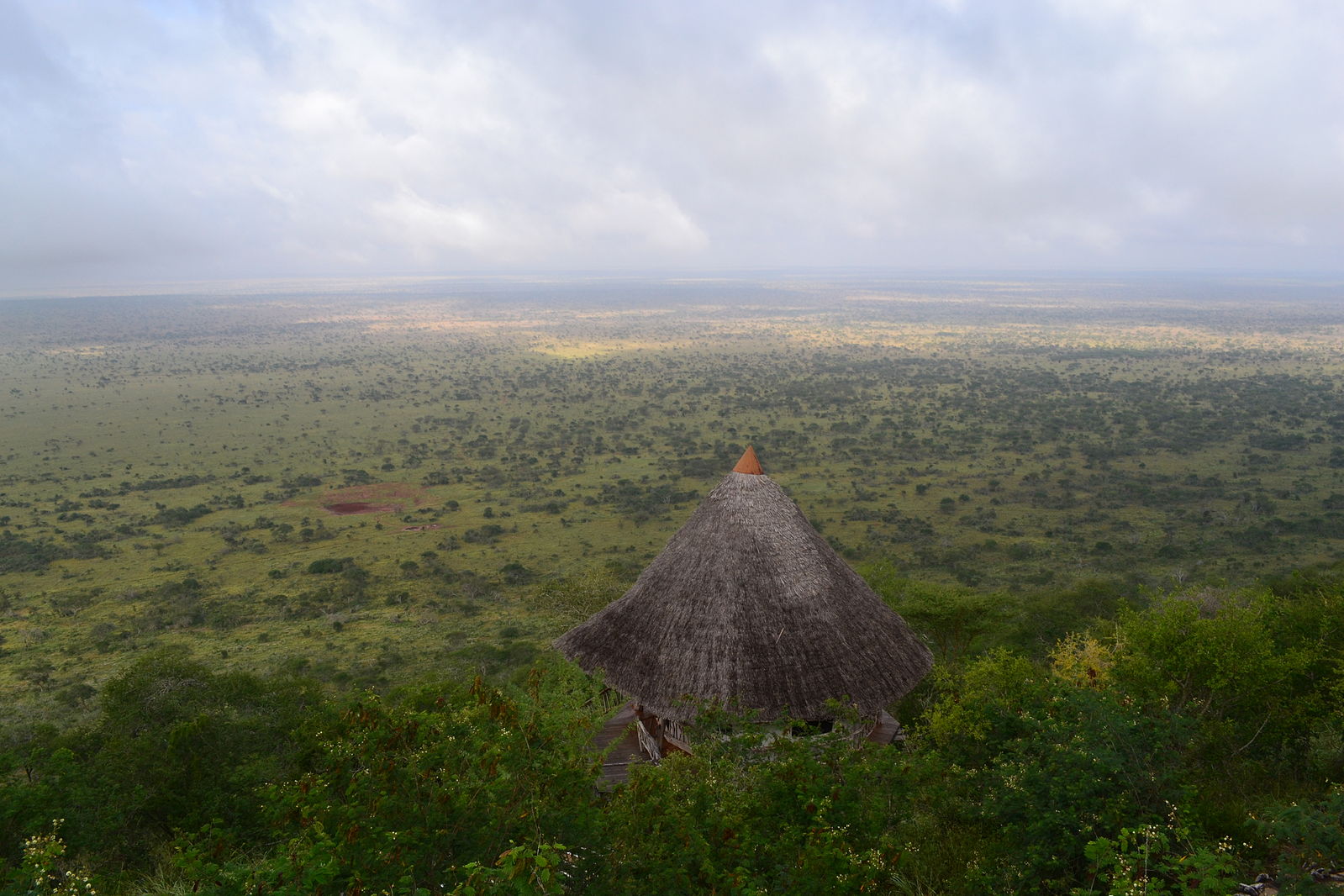
A hut in the LUMO Community Wildlife Sanctuary, a community-owned area of tropical savanna, in Kenya. Photo by Christopher T Cooper (Wikimedia Commons , Creative Commons Attribution 3.0 Unported license ).
Temperate grasslands are found at mid-latitudes and are generally located within continental interiors. They have distinct warm and cold seasons, with temperatures dropping below freezing in the winter. Precipitation ranges between 25 to 75 centimeters (about 10 to 29.5 inches) per year. Temperate grasslands include North American prairies, Eurasian steppes, South American pampas and punas, South African veldts, Australian temperate savannas, and New Zealand tussocks.
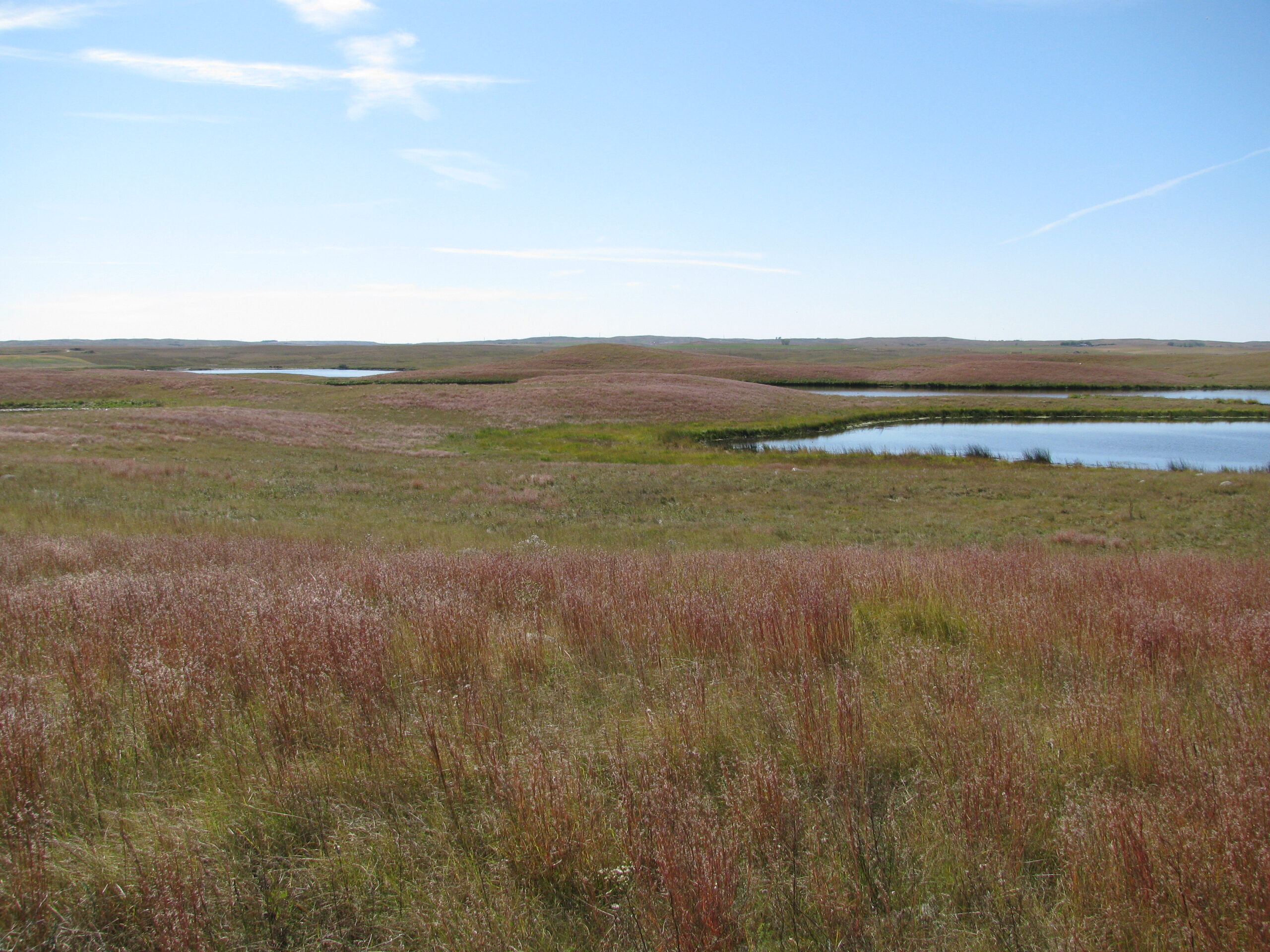
Mixed-grass prairie in the United States. Photo by the United States Forest Service Mountain-Prairie (flickr , Creative Commons Attribution 2.0 Generic license ).
Grasslands support a unique variety of plants and animals and provide other ecosystem services. For example, grassland soils have a high capacity for absorbing water, and are therefore helpful in flood management. Additionally, grasses and other vegetation in grasslands stabilize the soil with their roots, preventing erosion and desertification. For humans, grasslands are also the source of economic revenue, typically when they are used for grazing or they are converted to raising crops.
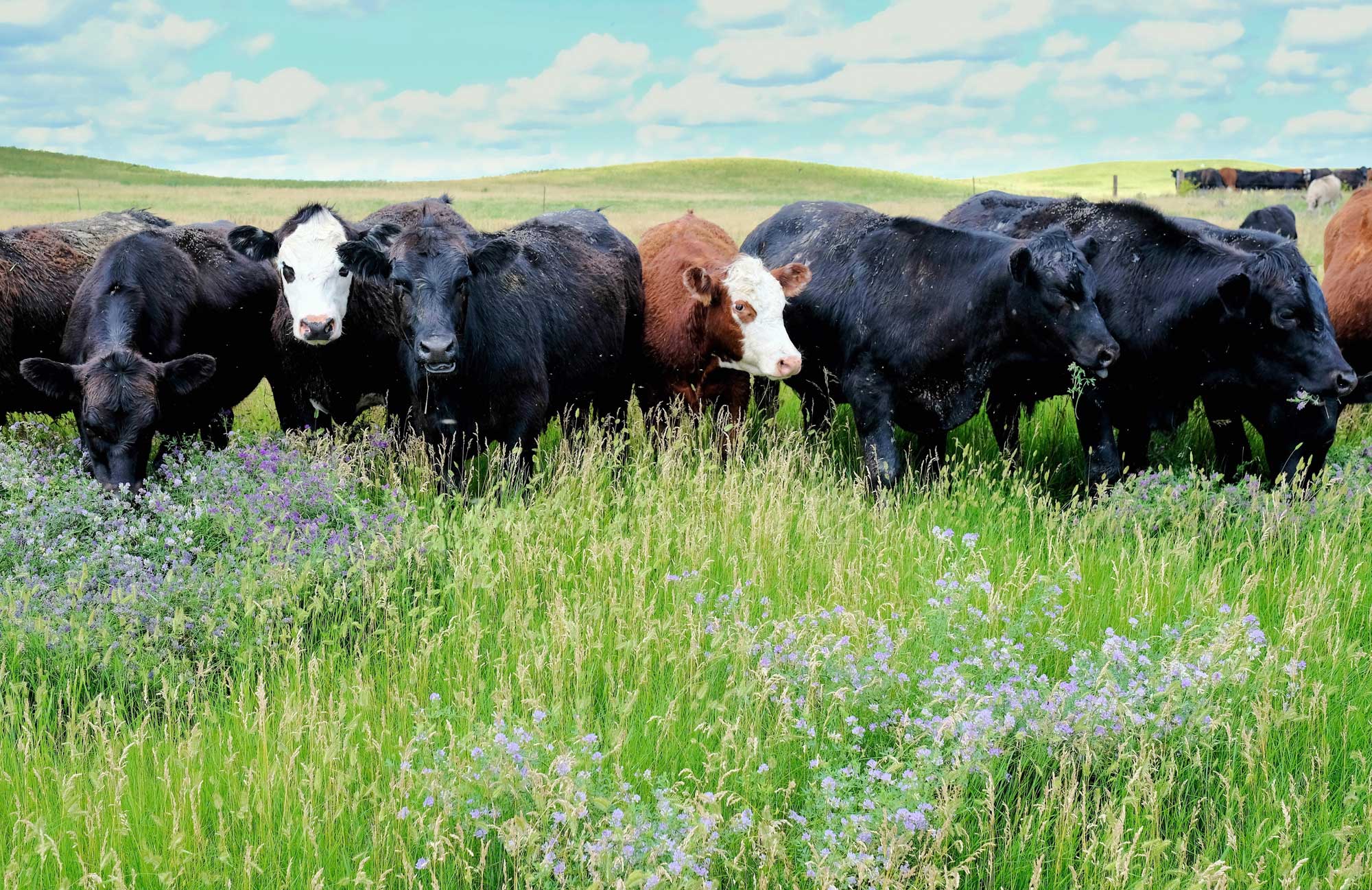
Cattle grazing on a prairie, South Dakota, U.S.A., 2018. Photo by Lars Plougmann (flickr , Creative Commons Attribution-ShareAlike 2.0 Generic license , image resized).
Why are grasslands located where they are?
Temperate grasslands .
Temperate grasslands are located at mid-latitudes. They are found on every continent besides Antarctica. Temperate grasslands are often found in continental interiors and in other locations relatively far from a body of water (like an ocean) that can serve as a source of rain. Mountain ranges are also a major factor determining the locations of temperate grasslands. Many of the world’s temperate grasslands are located on the leeward side of mountain ranges and are formed by the rain shadow effect .
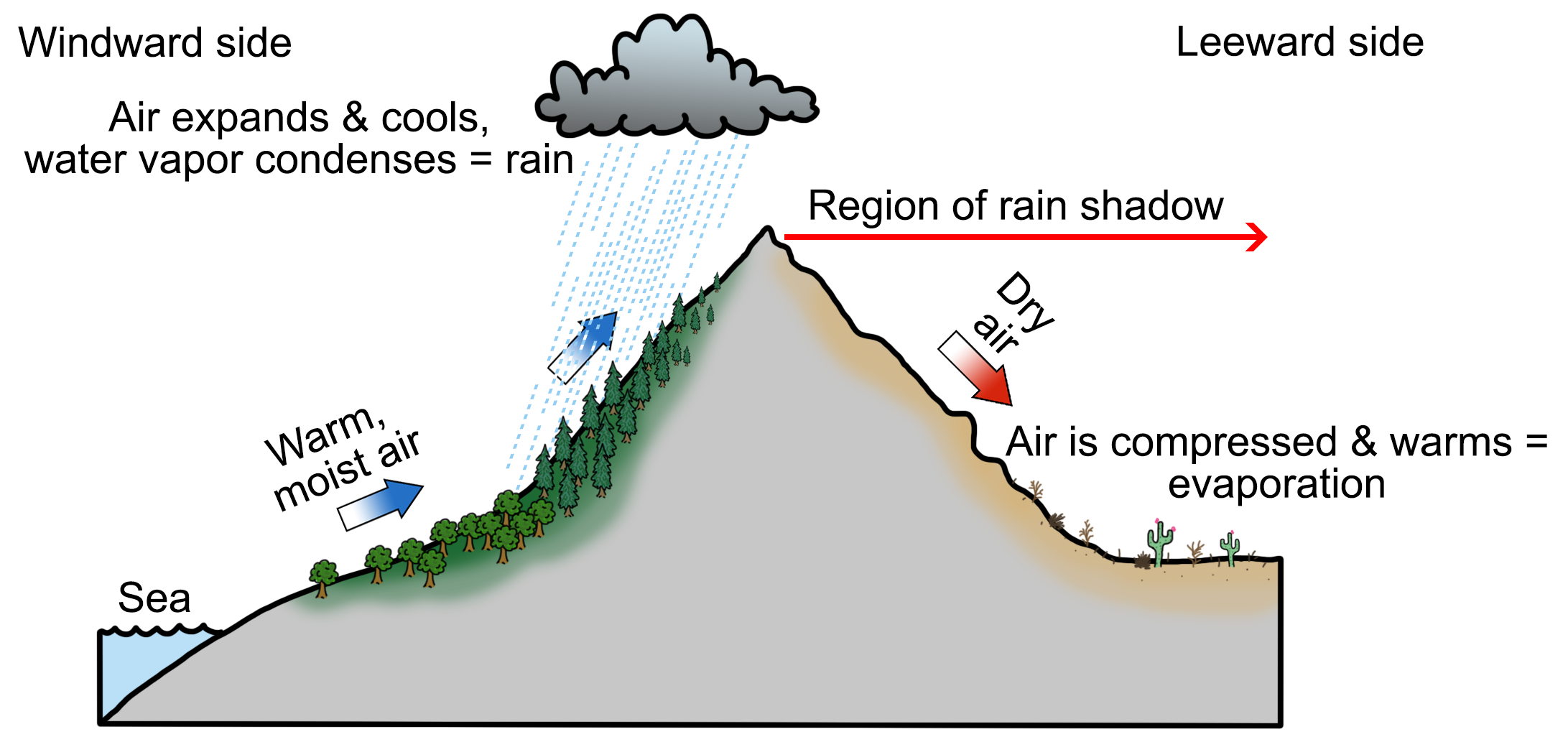
The rain shadow effect occurs when moisture-laden air rises up the windward side of a mountain, only to release this moisture as precipitation due to cooling and condensation. Once the air reaches the leeward side, it warms due to compression, promoting evaporation and a lack of precipitation. Diagram by Wade Greenberg-Brand, originally published in the Teacher Friendly Guide to Earth Science series, modified for Earth@Home.
Tropical grasslands
Tropical grasslands are located between 30ºN and 30ºS of the equator. They are found in South America, Africa, Asia, and Australia. The location of tropical grasslands, like other biomes in the tropics, are constrained and controlled by the interaction of trade winds at the intertropical convergence zone (ITCZ, located near the equator) and the effects of Hadley cells.
Hadley Cells are a global-scale tropical overturning circulation patterns that result in increasingly dry ecosystems moving from the equator to 30ºN and 30ºS. Solar heating causes air to rise at the ITCZ, where it condenses, causing precipitation (rainfall) to occur. Drier air then moves towards the poles and sinks at about 30ºN and 30ºS, creating deserts. The precipitation patterns created by Hadley Cells allow for tropical rainforests to occur at the equator and for deserts to occur at 30ºN and 30ºS. Tropical grasslands occur in the areas between the rainforests and deserts.
Differences in solar heating throughout the year cause the ITCZ to shift northwards and southwards seasonally. The ITCZ shifts northwards during the northern hemisphere summer and southwards during the northern hemisphere winter. The movement of the ITCZ results in seasonal wet and dry seasons in tropical grasslands. The wet season occurs when the area in question is close to the ITCZ, whereas the dry season occurs when the descending leg of the Hadley cell is overhead.
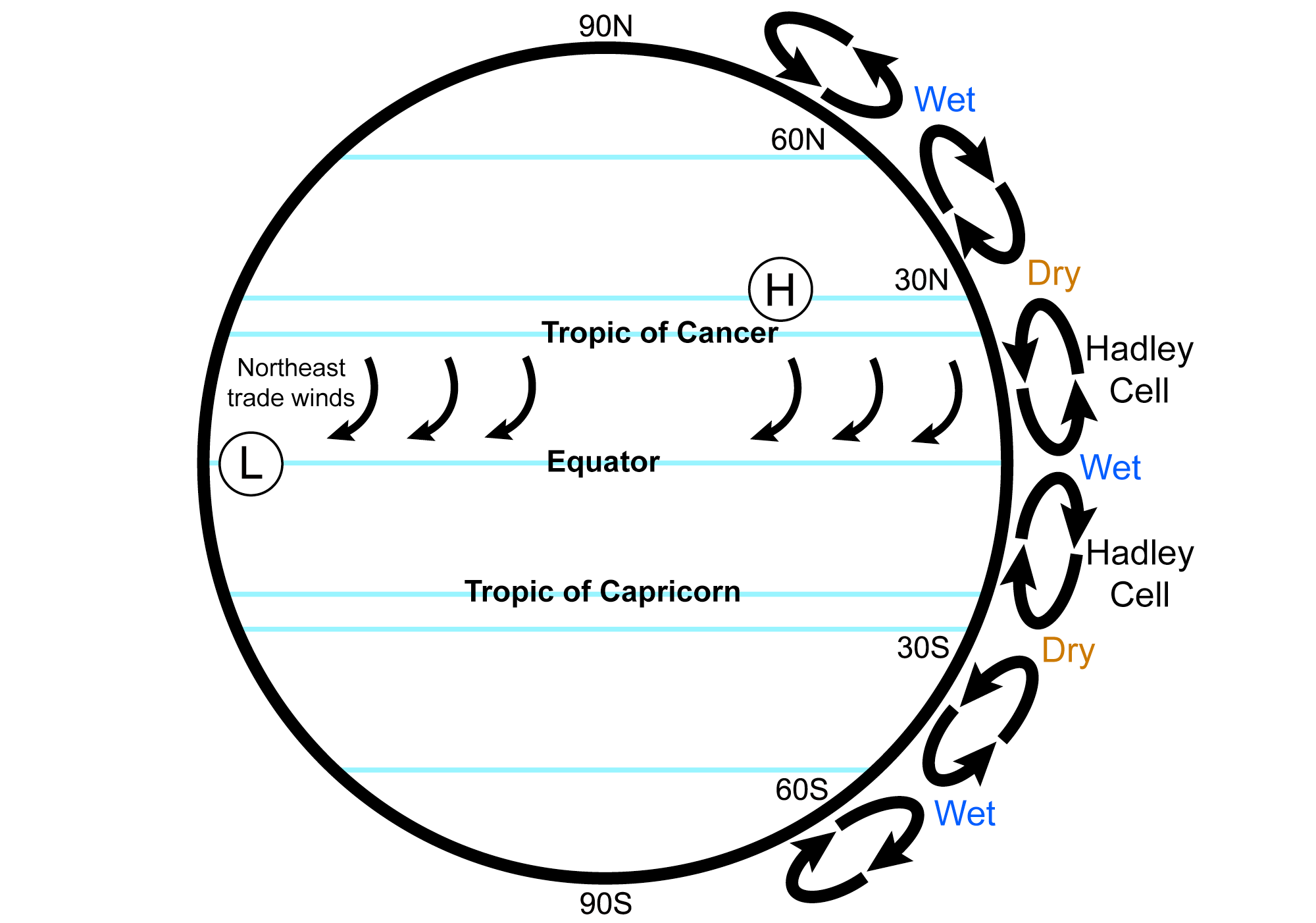
Hadley cells. Diagram redrawn by E. J. Hermsen from a diagram by Alexandra Moore that was originally published in The Teacher-Friendly Guide to the Earth Science of the Western US.
What are the threats to grasslands?
Grassland ecosystems currently face several threats, mostly due to human activities that alter grasslands directly, as well as from activities not directly altering grasslands (like burning fossil fuels), but that impact them nonetheless. Grasslands are often impacted by more than one threat simultaneously.
Agriculture
Both temperate and tropical grasslands are often used for agriculture, due to their fertile soils and the climates under which they thrive, which are suitable for farming or raising livestock. Cultivating crops requires removing native grasses from ecosystems. The crops that replace grasslands—which are often monocultures (fields of one species or even one variety of crop)—deplete the soils of nutrients. Pesticides may kill native insects, which can have negative impacts on native plants. Grazing agriculture is also common in both temperate and tropical grasslands, and overgrazing is an activity that threatens native grasses. Because grass roots absorb water and stabilize the soil, removing native grasses for planting crops or due to overgrazing can cause soil erosion and desertification.
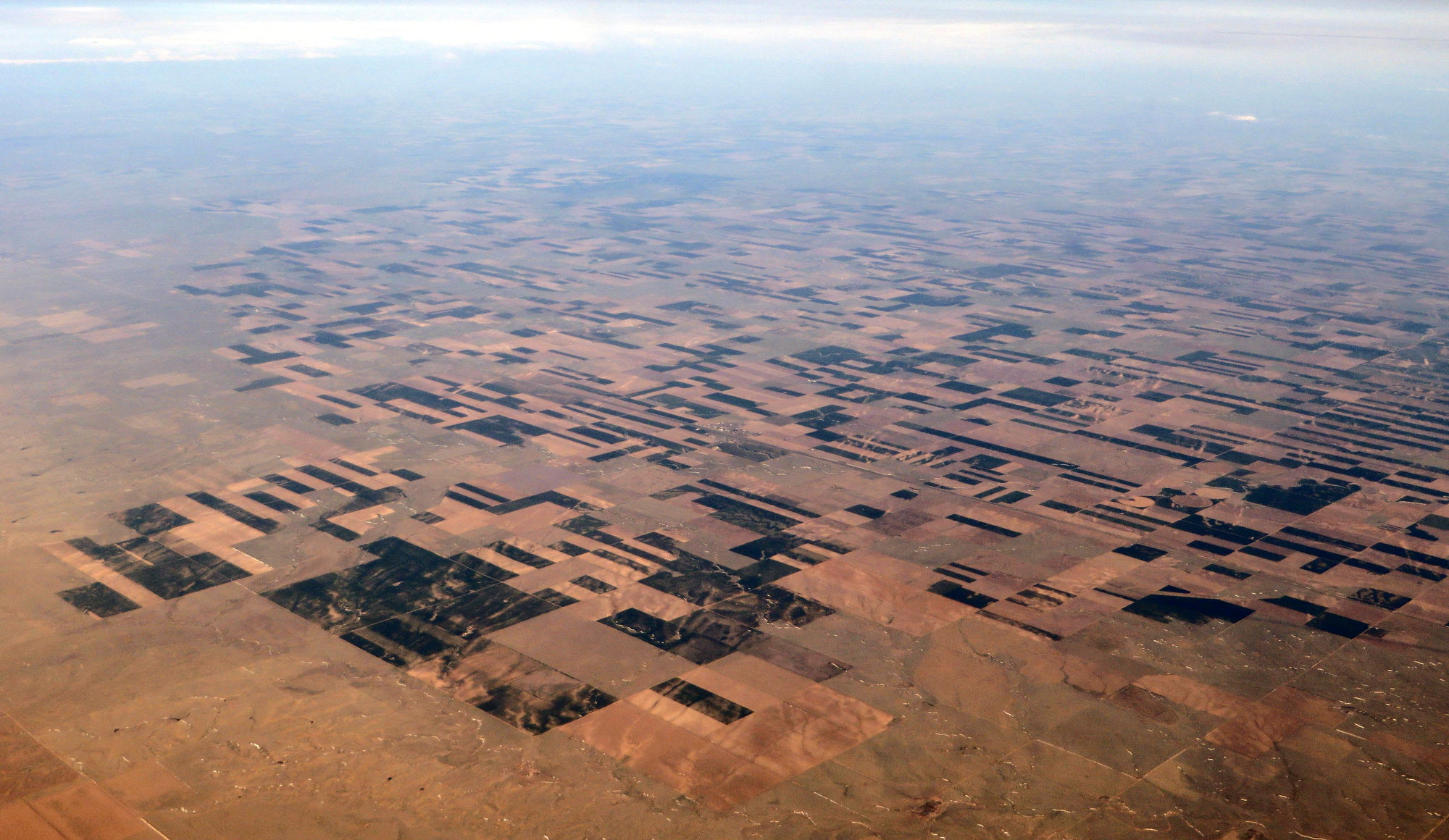
An aerial photograph of the region near Arriba, Colorado, Great Plains of the United States, 2014. Much of the prairie in the United States has been converted to agricultural uses, as shown in this photo. Photo by Ken Lund (flickr , Creative Commons Attribution-ShareAlike 2.0 Generic license , image cropped and resized).
Invasive species
Invasive species are species of plants or animals that are not native to a region and that outcompete native species, with negative or harmful impacts. Invasive species of plants may damage grassland ecosystems. Many invasive species, such as buffelgrass ( Cenchrus ciliaris ) and cogongrass ( Imperata cylindrica ) in North America and prosopis ( Prosopis juliflora , a type of shrub in the legume family/Fabaceae ) in Africa and Asia, can outcompete and displace native grasses. Some invasive plants that occur in grasslands can also consume large quantities of groundwater, like prosopis and tamarisk ( Tamarix , a shrub). Invasive plants that are fire adapted, like cogongrass, may fuel destructive wildfires, which can create a feedback mechanism that reinforces their dominance.
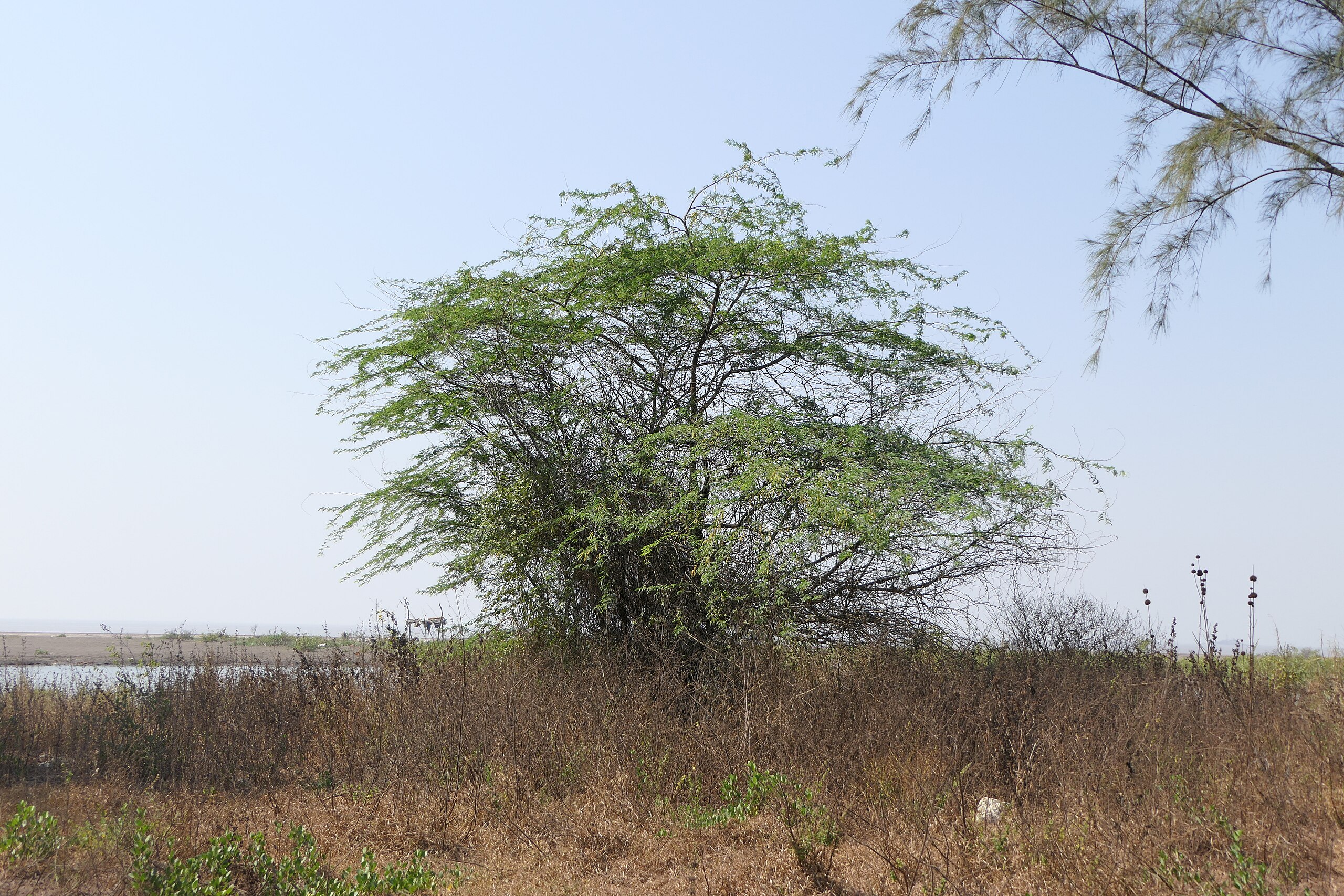
Prosopis ( Prosopis juliflora ), a shrub that is native to the Americas and invasive in Asia. This prosopis was photographed in Thane, India. Photo by Dinesh Valke (flickr , Creative Commons Attribution-Share Alike 2.0 Generic license ).
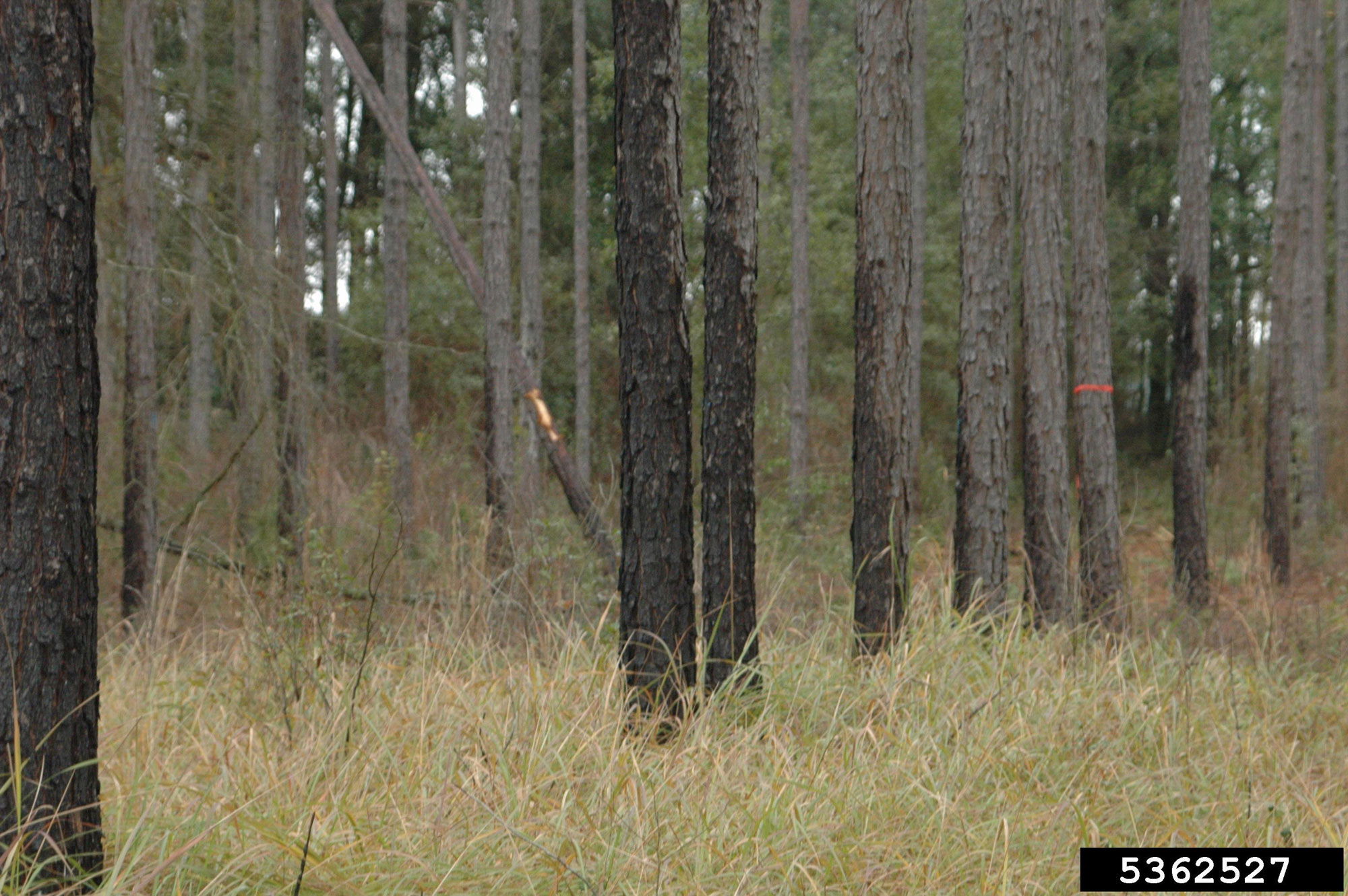
Cogongrass infestation. Original caption: "Burn marks on trees from extreme heat produced by flames fueled by cogongrass." Photo by Carey Minteer, University of Florida (Bugwood.org photo UGA536527 , Creative Commons Attribution 3.0 United States license).
Fire suppression
Fire suppression is another activity which can damage grassland ecosystems. Grasslands are maintained by periodic fires, and without fires, forests or shrublands may grow in their place. Fire suppression due to human activity is thus reducing grassland habitats.
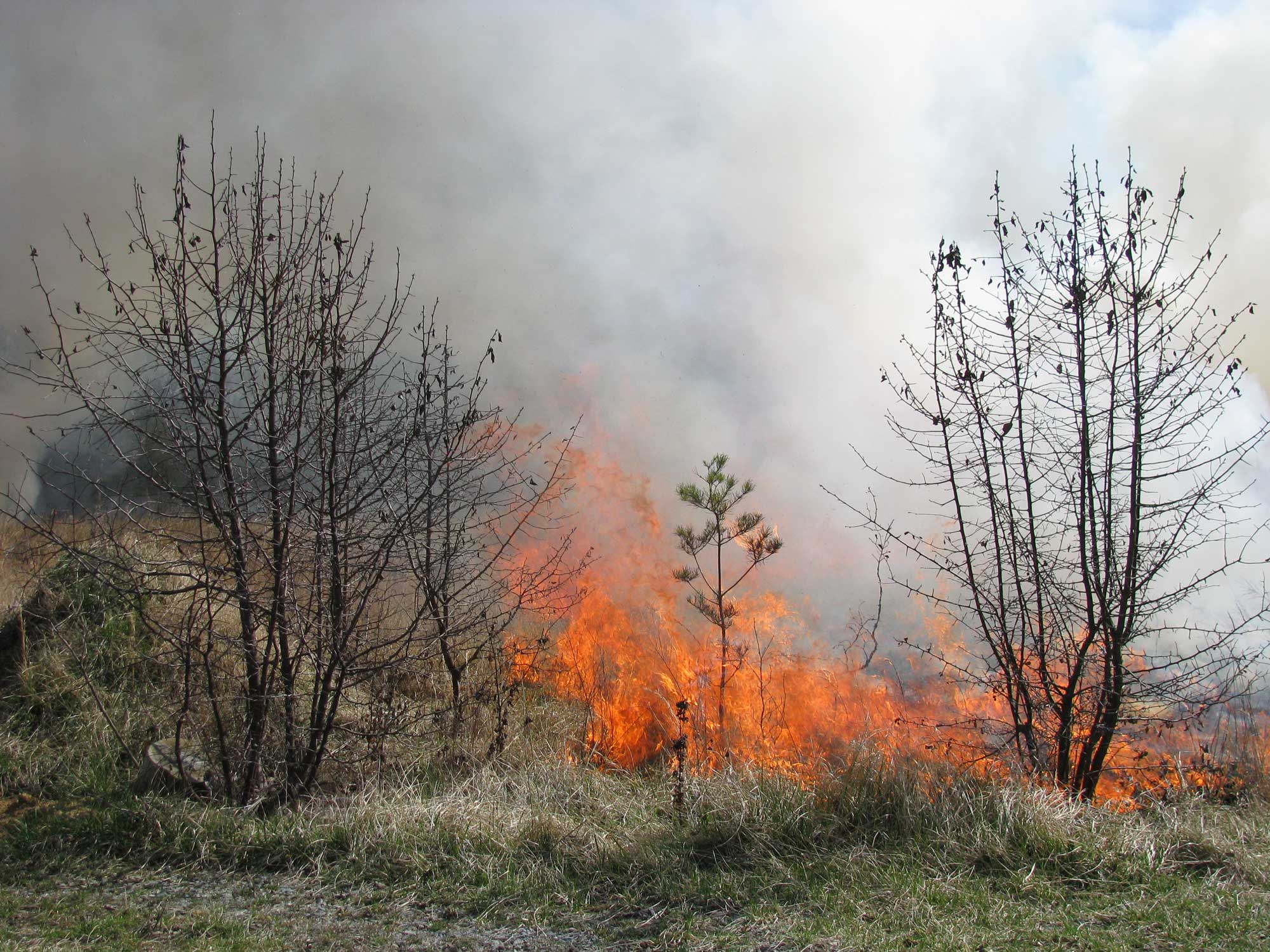
Original caption: "A controlled burn consumes small trees and shrubs encroaching on a grassland field. The burn, at Patuxent Research Refuge, was to maintain native grasslands, remove woody vegetation, and control invasive species such as Korean Lespedeza." Maryland, U.S.A., 2012. Photo by Sandy Spencer/USFWS (U.S. Fish and Wildlife Service on flickr , public domain ).
Climate change
Grasslands are threatened by climate change. Most projections indicate that extended periods of drought are likely to become more common in many of the world’s grassland ecosystems, which will likely threaten native species of plants and animals. Desertification is also a likely effect of climate change in some grasslands. The subsaharan savannas in Africa, for example, are at risk of desertification as the areas around the Sahara Desert continue to become drier.
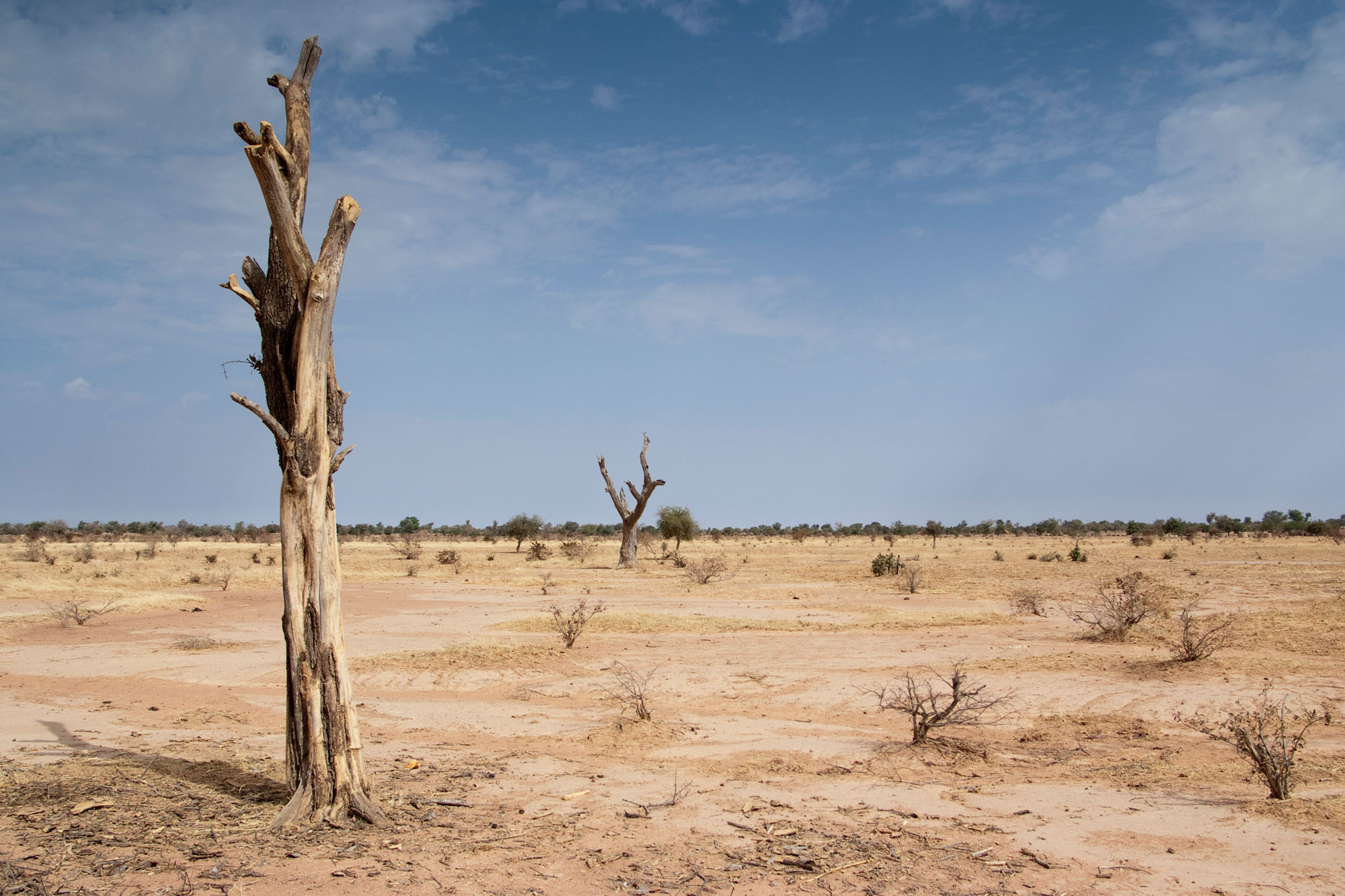
Desertification near the southwestern margin of the Sahara Desert in Burkina Faso, 2011. In this case, cutting of trees probably influenced drying of the landscape; often, desertification may have multiple drivers. Photo by TREEAID (flickr , Creative Commons Attribution 2.0 Generic license , image resized).
Ecosystem services from national grasslands (U.S. Forest Service): https://www.fs.usda.gov/managing-land/national-forests-grasslands/national-grasslands/ecoservices
Grassland (NASA Earth Observatory): https://earthobservatory.nasa.gov/biome/biograssland.php
Rain shadow (National Geographic Education): https://education.nationalgeographic.org/resource/rain-shadow/
Ten reasons for preserving grasslands (Swedish University of Agricultural Sciences News): https://www.slu.se/en/ew-news/2019/2/grasmarker/
Articles & reports
Carr, K. 2018. What are the impacts of humans on grassland biomes? Sciencing, 13 March 2018. https://sciencing.com/impacts-humans-grassland-biomes-2594.html
Nunez, C. 2022. Grasslands explained. National Geographic Education, 20 May 2022. https://education.nationalgeographic.org/resource/grasslands-explained/
Howari, F. M., M. Sharma, Y. Nazzal, A. El-Keblawy, S. Mir, C. M. Xavier, I. Ben Salem, A. A. Al-Taani, and F. Alaydaroos. 2022. Changes in the invasion rate of Prosopis juliflora and its impact on depletion of groundwater in the northern part of the United Arab Emirates. Plants 11(5): 682. https://doi.org/10.3390/plants11050682
Levin, S. A. 2013. Grassland Ecosystems. In Encyclopedia of biodiversity (2nd ed.). Elsevier Inc.
Thank you for visiting nature.com. You are using a browser version with limited support for CSS. To obtain the best experience, we recommend you use a more up to date browser (or turn off compatibility mode in Internet Explorer). In the meantime, to ensure continued support, we are displaying the site without styles and JavaScript.
- View all journals
- Explore content
- About the journal
- Publish with us
- Sign up for alerts
- Perspective
- Published: 07 September 2021
Combatting global grassland degradation
- Richard D. Bardgett ORCID: orcid.org/0000-0002-5131-0127 1 ,
- James M. Bullock 2 ,
- Sandra Lavorel 3 ,
- Peter Manning ORCID: orcid.org/0000-0002-7940-2023 4 ,
- Urs Schaffner ORCID: orcid.org/0000-0002-3504-3014 5 ,
- Nicholas Ostle 6 ,
- Mathilde Chomel 1 ,
- Giselda Durigan 7 ,
- Ellen L. Fry ORCID: orcid.org/0000-0001-7513-2006 1 ,
- David Johnson ORCID: orcid.org/0000-0003-2299-2525 1 ,
- Jocelyn M. Lavallee 1 ,
- Gaëtane Le Provost ORCID: orcid.org/0000-0002-1643-6023 4 ,
- Shan Luo 6 ,
- Kenny Png ORCID: orcid.org/0000-0003-2374-2595 1 ,
- Mahesh Sankaran ORCID: orcid.org/0000-0002-1661-6542 8 , 9 ,
- Xiangyang Hou 10 ,
- Huakun Zhou 11 ,
- Li Ma 11 , 12 ,
- Weibo Ren 13 ,
- Xiliang Li 10 ,
- Yong Ding 10 ,
- Yuanheng Li 10 &
- Hongxiao Shi 10
Nature Reviews Earth & Environment volume 2 , pages 720–735 ( 2021 ) Cite this article
7314 Accesses
375 Citations
266 Altmetric
Metrics details
- Ecosystem services
- Sustainability
Grasslands are under severe threat from ongoing degradation, undermining their capacity to support biodiversity, ecosystem services and human well-being. Yet, grasslands are largely ignored in sustainable development agendas. In this Perspective, we examine the current state of global grasslands and explore the extent and dominant drivers of their degradation. Socio-ecological solutions are needed to combat degradation and promote restoration. Important strategies include: increasing recognition of grasslands in global policy; developing standardized indicators of degradation; using scientific innovation for effective restoration at regional and landscape scales; and enhancing knowledge transfer and data sharing on restoration experiences. Stakeholder needs can be balanced through standardized assessment and shared understanding of the potential ecosystem service trade-offs in degraded and restored grasslands. The integration of these actions into sustainability policy will aid in halting degradation and enhancing restoration success, and protect the socio-economic, cultural and ecological benefits that grasslands provide.
This is a preview of subscription content, access via your institution
Access options
Access Nature and 54 other Nature Portfolio journals
Get Nature+, our best-value online-access subscription
24,99 € / 30 days
cancel any time
Subscribe to this journal
Receive 12 digital issues and online access to articles
92,52 € per year
only 7,71 € per issue
Buy this article
- Purchase on Springer Link
- Instant access to full article PDF
Prices may be subject to local taxes which are calculated during checkout
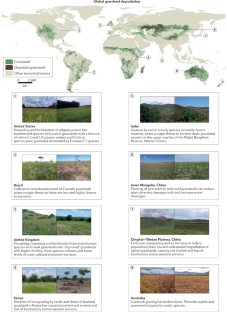
Similar content being viewed by others
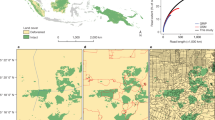
Ghost roads and the destruction of Asia-Pacific tropical forests
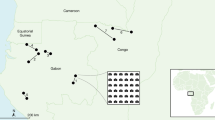
FSC-certified forest management benefits large mammals compared to non-FSC
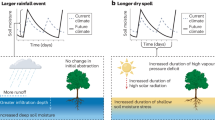
Plant responses to changing rainfall frequency and intensity
Suttie, J. M. Reynolds, S. G. & Batello, C. Grasslands of the World (FAO, 2005).
O’Mara, F. P. The role of grasslands in food security and climate change. Ann. Bot. 110 , 1263–1270 (2012).
Article Google Scholar
Wilsey, B. J. The Biology of Grasslands (Oxford Univ. Press, 2018).
White, R. P. Murray, S., Rohweder, M., Prince, S. D. & Thompson, K. M. Grassland Ecosystems (World Resources Institute, 2000).
Gibbs, H. K. & Salmon, J. M. Mapping the world’s degraded lands. Appl. Geogr. 57 , 12–21 (2015).
Lark, T. J., Spawn, S. A., Bougie, M. & Gibbs, H. K. Cropland expansion in the United States produces marginal yields at high costs to wildlife. Nat. Commun. 11 , 4295 (2020).
Abberton, M., Conant, R. & Batello, C. (eds) Grassland Carbon Sequestration: Management, Policy and Economics (FAO, 2010).
Gang, C. et al. Quantitative assessment of the contributions of climate change and human activities on global grassland degradation. Environ. Earth Sci. 72 , 4273–4282 (2014).
Dong, S., Kassam, K.-A. S., Tourrand, J. F. & Boone, R. B. (eds) Building Resilience of Human-Natural Systems of Pastoralism in the Developing World (Springer, 2016).
Bengtsson, J. et al. Grasslands — more important for ecosystem services than you might think. Ecosphere 10 , e02582 (2019).
Kwon, H. Y. et al. in Economics of Land Degradation and Improvement – A Global Assessment for Sustainable Development (eds Nkonya, E., Mirzabaev, A. & von Braun, J.) 197–214 (Springer, 2015).
Murphy, B. P., Andersen, A. N. & Parr, C. L. The underestimated biodiversity of tropical grassy biomes. Philos. Trans. R. Soc. B 371 , 20150319 (2016).
Smith, P. et al. Greenhouse gas mitigation in agriculture. Philos. Trans. R. Soc. B 363 , 789–813 (2008).
Mermoz, S., Bouvet, A., Toan, T. L. & Herold, M. Impacts of the forest definitions adopted by African countries on carbon conservation. Environ. Res. Lett. 13 , 104014 (2018).
Erdős, L. et al. The edge of two worlds: A new review and synthesis on Eurasian forest-steppes. Appl. Veg. Sci. 21 , 345–362 (2018).
Dengler, J., Janišová, M., Török, P. & Wellstein, C. Biodiversity of Palaearctic grasslands: a synthesis. Agric. Ecosyst. Environ. 182 , 1–14 (2014).
Bullock, J. M. et al. in The UK National Ecosystem Assessment Technical Report (UNEP-WCMC, 2011).
Parr, C. L., Lehmann, C. E. R., Bond, W. J., Hoffmann, W. A. & Andersen, A. N. Tropical grassy biomes: misunderstood, neglected, and under threat. Trends Ecol. Evol. 29 , 205–213 (2014).
Venter, Z. S., Cramer, M. D. & Hawkins, H. J. Drivers of woody plant encroachment over Africa. Nat. Commun. 9 , 2272 (2018).
Palchan, D. & Torfstein, A. A drop in Sahara dust fluxes records the northern limits of the African Humid Period. Nat. Commun. 10 , 3803 (2019).
Wilson, J. B., Peet, R. K., Dengler, J. & Pärtel, M. Plant species richness: the world records. J. Veg. Sci. 23 , 796–802 (2012).
Eriksson, O. & Cousins, S. A. Historical landscape perspectives on grasslands in Sweden and the Baltic region. Land 3 , 300–321 (2014).
Bråthen, K., Pugnaire. F. I. & Bardgett, R. D. The paradox of forbs in grasslands and their legacy of the Mammoth steppe. Front. Ecol. Environ . (in the press).
Shava, S. & Masuku, S. Living currency: The multiple roles of livestock in livelihood sustenance and exchange in the context of rural indigenous communities in southern Africa. South. Afr. J. Environ. Educ. https://doi.org/10.4314/sajee.v35i1.16 (2019).
FAO. Livestock Keepers – Guardians of Biodiversity (FAO, 2009).
Bond, W. J. Ancient grasslands at risk. Science 351 , 120–122 (2016).
Ripple, W. J. et al. Collapse of the world’s largest herbivores. Sci. Adv. 1 , e1400103 (2015).
Arbieu, U., Grünewald, C., Martín-López, B., Schleuning, M. & Böhning-Gaese, K. Large mammal diversity matters for wildlife tourism in Southern African Protected Areas: Insights for management. Ecosyst. Serv. 31 , 481–490 (2018).
Lavorel, S. et al. Historical trajectories in land use pattern and grassland ecosystem services in two European alpine landscapes. Reg. Environ. Change 17 , 2251–2264 (2017).
Scurlock, J. M. O. & Hall, D. O. The global carbon sink: a grassland perspective. Glob. Change Biol. 4 , 229–233 (1998).
Chang, J. et al. Climate warming from managed grasslands cancels the cooling effect of carbon sinks in sparsely grazed and natural grasslands. Nat. Commun. 12 , 118 (2021).
Goldstein, A. Protecting irrecoverable carbon in Earth’s ecosystems. Nat. Clim. Change 10 , 287–295 (2020).
Conant, R. T., Cerri, C. E., Osborne, B. B. & Paustian, K. Grassland management impacts on soil carbon stocks: a new synthesis. Ecol. Appl. 27 , 662–668 (2017).
IPBES. The IPBES Assessment Report on Land Degradation and Restoration (IPBES, 2018).
Cao, J. et al. Grassland degradation on the Qinghai-Tibetan Plateau: reevaluation of causative factors. Rangel. Ecol. Manag. 72 , 988–995 (2019).
Andrade, B. O. et al. Grassland degradation and restoration: a conceptual framework of stages and thresholds illustrated by southern Brazilian grasslands. Nat. Conserv. 13 , 95–104 (2015).
Okpara, U. T. et al. A social-ecological systems approach is necessary to achieve land degradation neutrality. Environ. Sci. Policy 89 , 59–66 (2018).
Castro, A. J. et al. Ecosystem service trade-offs from supply to social demand: A landscape-scale spatial analysis. Landsc. Urban Plan. 132 , 102–110 (2014).
Felipe-Lucia, M. R. et al. Ecosystem services flows: why stakeholders’ power relationships matter. PLoS One 10 , e0132232 (2015).
Manning, P. et al. Redefining ecosystem multifunctionality. Nat. Ecol. Evol. 2 , 427–436 (2018).
Wang, S. et al. Management and land use change effects on soil carbon in northern China’s grasslands: a synthesis. Agric. Ecosyst. Environ. 142 , 329–340 (2011).
Allan, E. et al. Land use intensification alters ecosystem multifunctionality via loss of biodiversity and changes to functional composition. Ecol. Lett. 18 , 834–843 (2015).
Bullock, J. M., Aronson, J., Newton, A. C., Pywell, R. F. & Rey-Benayas, J. M. Restoration of ecosystem services and biodiversity: conflicts and opportunities. Trends Ecol. Evol. 26 , 541–549 (2011).
Ridding, L. E., Watson, S. C. L., Newton, A. C., Rowland, C. S. & Bullock, J. M. Ongoing, but slowing, habitat loss in a rural landscape over 85 years. Landsc. Ecol. 35 , 257–273 (2020).
Hilker, T., Natsagdorj, E., Waring, R. H., Lyapustin, A. & Wang, Y. J. Satellite observed widespread decline in Mongolian grasslands largely due to overgrazing. Glob. Chang. Biol. 20 , 418–428 (2014).
Poschlod, P. & WallisDeVries, M. F. The historical and socioeconomic perspective of calcareous grasslands - lessons from the distant and recent past. Biol. Conserv. 104 , 361–376 (2002).
Stevens, C. J., Dise, N. B., Mountford, J. O. & Gowing, D. J. Impact of nitrogen deposition on the species richness of grasslands. Science 303 , 1876–1879 (2004).
Aune, S., Bryn, A. & Hovstad, K. A. Loss of semi-natural grassland in a boreal landscape: impacts of agricultural intensification and abandonment. J. Land Use Sci. 13 , 375–390 (2018).
Veldman, J. W. et al. Where tree planting and forest expansion are bad for biodiversity and ecosystem services. Bioscience 65 , 1011–1018 (2015).
Shukla, P. R. et al. (eds) Climate Change and Land: An IPCC Special Report on Climate Change, Desertification, Land Degradation, Sustainable Land Management, Food Security, and Greenhouse Gas Fluxes in Terrestrial Ecosystems (CGIAR, 2019).
Burrell, A. L., Evans, J. P. & De Kauwe, M. G. Anthropogenic climate change has driven over 5 million km 2 of drylands towards desertification. Nat. Commun. 11 , 3853 (2020).
Archer, S. R. et al. in Rangeland Systems: Processes, Management and Challenges (ed. Briske, D. D.) 25–84 (Springer, 2017).
Zhang, G. et al. Exacerbated grassland degradation and desertification in Central Asia during 2000–2014. Ecol. Appl. 28 , 442–456 (2018).
Dudley, N. et al. Grassland and Savannah Ecosystems: An Urgent Need for Conservation and Sustainable Management (WWF Deutschland, 2020).
Henderson, K. A. et al. Landowner perceptions of the value of natural forest and natural grassland in a mosaic ecosystem in southern Brazil. Sustain. Sci. 11 , 321–330 (2016).
Costanza, R. et al. Changes in the global value of ecosystem services. Glob. Environ. Change 26 , 152–158 (2014).
Durigan, G., Pilon, N. A. P., Assis, G. B., Souza, F. M. & Baitello, J. B. Plantas Pequenas do Cerrado: Biodiversidade Negligenciada . (Instituto Florestal, Secretaria do Meio Ambiente, 2018).
Assandri, G., Bogliani, G., Pedrini, P. & Brambilla, M. Toward the next Common Agricultural Policy reform: Determinants of avian communities in hay meadows reveal current policy’s inadequacy for biodiversity conservation in grassland ecosystems. J. Appl. Ecol. 56 , 604–617 (2019).
Liang, L., Chen, F., Shi, L. & Niu, S. NDVI-derived forest area change and its driving factors in China. PLoS One 13 , e0205885 (2018).
Cao, S. et al. Damage caused to the environment by reforestation policies in arid and semi-arid areas of China. Ambio 39 , 279–283 (2010).
Cao, S., Wang, G. & Chen, l Questionable value of planting thirsty trees in dry regions. Nature 465 , 31 (2010).
Zastrow, M. China’s tree-planting drive could falter in a warming world. Nature 573 , 474–475 (2019).
Landau, E., da Silva, G. A., Moura, L., Hirsch, A., & Guimaraes, D. Dinâmica da produção agropecuária e da paisagem natural no Brasil nas últimas décadas: sistemas agrícolas, paisagem natural e análise integrada do espaço rural (Embrapa Milho e Sorgo-Livro científico (ALICE), 2020).
Wolff, S., Schrammeijer, E. A., Schulp, C. J. & Verburg, P. H. Meeting global land restoration and protection targets: What would the world look like in 2050? Glob. Environ. Change 52 , 259–272 (2018).
Bastin, J. F. et al. The global tree restoration potential. Science 365 , 76–79 (2019).
Veldman, J. W. et al. Comment on “The global tree restoration potential”. Science 366 , eaay7976 (2019).
Dass, P., Houlton, B. Z., Wang, Y. & Warlind, D. Grasslands may be more reliable carbon sinks than forests in California. Environ. Res. Lett. 13 , 074027 (2018).
Jackson, R. B., Banner, J. L., Jobbágy, E. G., Pockman, W. T. & Wall, D. H. Ecosystem carbon loss with woody plant invasion of grasslands. Nature 418 , 623–626 (2002).
Jackson, R. B. et al. The ecology of soil carbon: pools, vulnerabilities, and biotic and abiotic controls. Annu. Rev. Ecol. Evol. Syst. 48 , 419–445 (2017).
Berthrong, S. T., Jobbágy, E. G. & Jackson, R. B. A global meta-analysis of soil exchangeable cations, pH, carbon, and nitrogen with afforestation. Ecol. Appl. 19 , 2228–2241 (2009).
Kirschbaum, M. U. F. et al. Implications of albedo changes following afforestation on the benefits of forests as carbon sinks. Biogeosciences 8 , 3687–3696 (2011).
Conant, R. T. Challenges and Opportunities for Carbon Sequestration in Grassland Systems. A Technical Report on Grassland Management and Climate Change Mitigation (FAO, 2010).
Wu, G. L. et al. Trade-off between vegetation type, soil erosion control and surface water in global semi-arid regions: A meta-analysis. J. Appl. Ecol. 57 , 875–885 (2020).
Veldman, J. W. et al. Tyranny of trees in grassy biomes. Science 347 , 484–485 (2015).
Burrascano, S. et al. Current European policies are unlikely to jointly foster carbon sequestration and protect biodiversity. Biol. Conserv. 201 , 370–376 (2016).
Vanak, A. T., Hiremath, A. & Rai, N. Wastelands of the mind: Identity crisis of India’s tropical savannas. Curr. Conserv. 7 , 16–23 (2014).
Google Scholar
Ratnam, J., Tomlinson, K. W., Rasquinha, D. N. & Sankaran, M. Savannahs of Asia: antiquity, biogeography, and an uncertain future. Philos. Trans. R. Soc. B Biol. Sci. 371 , 20150305 (2016).
Overbeck, G. E. et al. Conservation in Brazil needs to include non-forest ecosystems. Divers. Distrib. 21 , 1455–1460 (2015).
Kumar, D. et al. Misinterpretation of Asian savannas as degraded forest can mislead management and conservation policy under climate change. Biol. Conserv. 241 , 108293 (2020).
Kemp, D. R. et al. Innovative grassland management systems for environmental and livelihood benefits. Proc. Natl Acad. Sci. USA 110 , 8369–8374 (2013).
Scholes, R. et al. (eds) Summary for Policymakers of the Assessment Report on Land Degradation and Restoration of the Intergovernmental Science-Policy Platform on Biodiversity and Ecosystem Services (IPBES Secretariat, 2018).
Lamarque, P. et al. Stakeholder perceptions of grassland ecosystem services in relation to knowledge on soil fertility and biodiversity. Reg. Environ. Change 11 , 791–804 (2011).
Hauck, J., Schmidt, J. & Werner, A. Using social network analysis to identify key stakeholders in agricultural biodiversity governance and related land-use decisions at regional and local level. Ecol. Soc. 21 , 49 (2016).
Reid, R. S., Fernández-Giménez, M. E. & Galvin, K. A. Dynamics and resilience of rangelands and pastoral peoples around the globe. Annu. Rev. Environ. Resour. 39 , 217–242 (2014).
Quétier, F., Rivoal, F., Marty, P., De Chazal, J. & Lavorel, S. Social representations of an alpine grassland landscape and socio-political discourses on rural development. Reg. Environ. Change 10 , 119–130 (2010).
Linders, T. E. W. et al. Stakeholder priorities determine the impact of an alien tree invasion on ecosystem multifunctionality. People Nat. 3 , 658–672 (2021).
Gos, P. & Lavorel, S. Stakeholders’ expectations on ecosystem services affect the assessment of ecosystem services hotspots and their congruence with biodiversity. Int. J. Biodivers. Sci. Ecosyst. Serv. Manag. 8 , 93–106 (2012).
Fontana, V. et al. Comparing land-use alternatives: Using the ecosystem services concept to define a multi-criteria decision analysis. Ecol. Econ. 93 , 128–136 (2013).
Jellinek, S. et al. Integrating diverse social and ecological motivations to achieve landscape restoration. J. Appl. Ecol. 56 , 246–252 (2019).
Lavorel, S. & Grigulis, K. How fundamental plant functional trait relationships scale-up to trade-offs and synergies in ecosystem services. J. Ecol. 100 , 128–140 (2012).
Stürck, J. et al. Simulating and delineating future land change trajectories across Europe. Reg. Environ. Change 18 , 733–749 (2018).
Lavorel, S. in Grasslands and Climate Change (eds Gibson, D. J. & Newman, J. A.) 131–146) (Cambridge Univ. Press, 2018).
Ayanu, Y. et al. Ecosystem engineer unleashed: Prosopis juliflora threatening ecosystem services? Reg. Environ. Change 15 , 155–167 (2015).
Mbaabu, P. R. et al. Restoration of degraded grasslands, but not invasion by Prosopis juliflora , avoids trade-offs between climate change mitigation and other ecosystem services. Sci. Rep. 10 , 20391 (2020).
Sayer, J. A. et al. Ten principles for a landscape approach to reconciling agriculture, conservation, and other competing land uses. Proc. Natl Acad. Sci. USA 110 , 8349–8356 (2013).
Flintan, F. & Cullis, A. Introductory Guidelines to Participatory Rangeland Management in Pastoral Areas (Save the Children USA, 2010).
Robinson, L. W. et al. Participatory Rangeland Management Toolkit for Kenya (ILRI, 2018).
Roba, G. & David, J. Participatory Rangeland Management Planning: A Field Guide (IUCN, 2018).
Langemeyer, J., Gómez-Baggethun, E., Haase, D., Scheuer, S. & Elmqvist, T. Bridging the gap between ecosystem service assessments and land-use planning through Multi-Criteria Decision Analysis (MCDA). Environ. Sci. Policy 62 , 45–56 (2016).
Adem Esmail, B. & Geneletti, D. Multi-criteria decision analysis for nature conservation: A review of 20 years of applications. Methods Ecol. Evol. 9 , 42–53 (2018).
Martin-Lopez, B. et al. A novel tele-coupling framework to assess social relations across spatial scales for ecosystem services research. J. Environ. Manage. 241 , 251–263 (2019).
Joseph, L. N., Maloney, R. F. & Possingham, H. P. Optimal allocation of resources among threatened species: a project prioritization protocol. Conserv. Biol. 23 , 328–338 (2009).
Wortley, L., Hero, J. M. & Howes, M. Evaluating ecological restoration success: a review of the literature. Restor. Ecol. 21 , 537–543 (2013).
Cameron, A. Restoration of ecosystems and ecosystem services, in Ecosystem Services and Poverty Alleviation: Trade-offs and Governance (eds Schreckenberg, K., Mace, G. & Poudyal. M.) (Routledge, 2018).
Suding, K. N. Toward an era of restoration in ecology: successes, failures, and opportunities ahead. Annu. Rev. Ecol. Evol. Syst. 42 , 465–487 (2011).
Mekuria, W., Veldkamp, E., Corre, M. D. & Haile, M. Restoration of ecosystem carbon stocks following exclosure establishment in communal grazing lands in Tigray, Ethiopia. Soil Sci. Soc. Am. J. 75 , 246–256 (2011).
Mekuria, W. & Aynekulu, E. Exclosure land management for restoration of the soils in degraded communal grazing lands in northern Ethiopia. Land Degrad. Dev. 24 , 528–538 (2011).
Hu, Y. & Nacun, B. An analysis of land-use change and grassland degradation from a policy perspective in Inner Mongolia, China, 1990–2015. Sustainability 10 , 4048 (2018).
Nedessa, B., Ali, J. & Nyborg, I. Exploring Ecological and Socio-Economic Issues for the Improvement of Area Enclosure Management (Drylands Coordination Group, 2005).
Schweiger, A. K. et al. Plant spectral diversity integrates functional and phylogenetic components of biodiversity and predicts ecosystem function. Nat. Ecol. Evol. 2 , 976–982 (2018).
Vågen, T. G. & Winowiecki, L. A. Mapping of soil organic carbon stocks for spatially explicit assessments of climate change mitigation potential. Environ. Res. Lett. 8 , 015011 (2013).
Xia, J. et al. Spatio-temporal patterns and climate variables controlling of biomass carbon stock of global grassland ecosystems from 1982 to 2006. Remote Sens. 6 , 1783–1802 (2014).
Spawn, S. A. et al. Harmonized global maps of above and belowground biomass carbon density in the year 2010. Sci. Data 7 , 112 (2020).
Bellocchi, G. & Chabbi, A. Grassland management for sustainable agroecosystems. Agronomy 10 , 78 (2020).
Plas, F. et al. Towards the development of general rules describing landscape heterogeneity – multifunctionality relationships. J. Appl. Ecol. 56 , 168–179 (2019).
Kimberley, A. et al. Functional rather than structural connectivity explains grassland plant diversity patterns following landscape scale habitat loss. Landsc. Ecol. 36 , 265–280 (2021).
Gilarranz, L. J., Rayfield, B., Liñán-Cembrano, G., Bascompte, J. & Gonzalez, A. Effects of network modularity on the spread of perturbation impact in experimental metapopulations. Science 357 , 199–201 (2017).
Smith, F. P., Prober, S. M., House, A. P. N. & McIntyre, S. Maximizing retention of native biodiversity in Australian agricultural landscapes — The 10:20:40:30 guidelines. Agric. Ecosyst. Environ. 166 , 35–45 (2013).
Auffret, A. G. et al. Plant functional connectivity — integrating landscape structure and effective dispersal. J. Ecol. 105 , 1648–1656 (2017).
Isaac, N. J. B. et al. Defining and delivering resilient ecological networks: Nature conservation in England. J. Appl. Ecol. 55 , 2537–2543 (2018).
Vörösmarty, C. J. Global threats to human water security and river biodiversity. Nature 467 , 555–561 (2010).
Barbier, E. B. The economic linkages between rural poverty and land degradation: some evidence from Africa. Agric. Ecosyst. Environ. 82 , 355–370 (2000).
Kardol, P. & Wardle, D. A. How understanding aboveground–belowground linkages can assist restoration ecology. Trends Ecol. Evol. 25 , 670–679 (2010).
Bardgett, R. D. Plant trait-based approaches for interrogating belowground function. Biol. Environ. 117 , 1–13 (2017).
Isbell, F. et al. Benefits of increasing plant diversity in sustainable agroecosystems. J. Ecol. 105 , 871–879 (2017).
Manning, P. et al. Transferring biodiversity-ecosystem function research to the management of ‘real-world’ ecosystems. Adv. Ecol. Res. 61 , 323–356 (2019).
Jochum, M. et al. The results of biodiversity–ecosystem functioning experiments are realistic. Nat. Ecol. Evol. 4 , 1485–1494 (2020).
Cole et al. Grassland biodiversity restoration increase resistance of carbon fluxes to drought. J. Appl. Ecol. 56 , 1806–1816 (2019).
Yang, Y., Tilman, D., Furey, G. & Lehman, C. Soil carbon sequestration accelerated by restoration of grassland biodiversity. Nat. Commun. 10 , 718 (2018).
Fry, E. L. et al. Soil multifunctionality and drought resistance are determined by plant structural traits in restoring grassland. Ecology 99 , 2260–2271 (2018).
Gould, I. J., Quinton, J. N., Weigelt, A., De Deyn, G. B. & Bardgett, R. D. Plant diversity and root traits benefit physical properties key to soil function in grasslands. Ecol. Lett. 19 , 1140–1149 (2016).
Wubs, E. R., van der Putten, W. H., Bosch, M. & Bezemer, T. M. Soil inoculation steers restoration of terrestrial ecosystems. Nat. Plants 2 , 16107 (2016).
Pilon, N. A., Assis, G. B., Souza, F. M. & Durigan, G. Native remnants can be sources of plants and topsoil to restore dry and wet cerrado grasslands. Restor. Ecol. 27 , 569–580 (2019).
Wang, L. et al. Diversifying livestock promotes multidiversity and multifunctionality in managed grasslands. Proc. Natl Acad. Sci. USA 116 , 201807354 (2019).
Wang, X. et al. High ecosystem multifunctionality under moderate grazing is associated with high plant but low bacterial diversity in a semi-arid steppe grassland. Plant Soil 448 , 265–276 (2020).
Pocock, M. J. O., Evans, D. M. & Memmott, J. The robustness and restoration of a network of ecological networks. Science 335 , 973–977 (2012).
Buisson, E. et al. Resilience and restoration of tropical and subtropical grasslands, savannas, and grassy woodlands. Biol. Rev. 94 , 590–609 (2019).
Lee, M., Manning, P., Rist, J., Power, S. A. & Marsh, C. A global comparison of grassland biomass responses to CO 2 and nitrogen enrichment. Philos. Trans. R. Soc. B 365 , 2047–2056 (2010).
Craven, D. et al. Multiple facets of biodiversity drive the diversity–stability relationship. Nat. Ecol. Evol. 2 , 1579–1587 (2018).
Borer, E. T. et al. Finding generality in ecology: a model for globally distributed experiments. Methods Ecol. Evol. 5 , 65–73 (2014).
Fraser, L. H. et al. Worldwide evidence of a unimodal relationship between productivity and plant species richness. Science 349 , 302–305 (2015).
Spake, R. et al. An analytical framework for spatially targeted management of natural capital. Nat. Sustain. 2 , 90–97 (2019).
Dudley et al. Grasslands and savannahs in the UN Decade on Ecosystem Restoration. Restor. Ecol. 28 , 1313–1317 (2020).
Yengoh, G. T., Dent, D., Olsson, L., Tengberg, A. E. & Tucker, C. J. III. Use of the Normalized Difference Vegetation Index (NDVI) to Assess Land Degradation at Multiple Scales: Current Status, Future Trends, and Practical Considerations (Springer, 2015).
Buchhorn, M. et al. Copernicus Global Land Service: Land Cover 100m, epoch 2015, Globe (Version V2.0.2) [data set]. Zenodo https://doi.org/10.5281/zenodo.3243509 (2019).
Rossiter, J., Wondie Minale, M., Andarge, W. & Twomlow, S. A communities Eden–grazing Exclosure success in Ethiopia. Int. J. Agric. Sustain. 15 , 514–526 (2017).
Durigan, G. et al. Invasão por Pinus spp: Ecologia, Prevenção, Controle e Restauração (Instituto Florestal, 2020).
Wang, Z. et al. Effect of manipulating animal stocking rate on the carbon storage capacity in a degraded desert steppe. Ecol. Res. 32 , 1001–1009 (2017).
Wang, Z. et al. Effects of stocking rate on the variability of peak standing crop in a desert steppe of Eurasia grassland. Environ. Manag. 53 , 266–273 (2014).
Zhang, R. et al. Grazing induced changes in plant diversity is a critical factor controlling grassland productivity in the Desert Steppe, Northern China. Agric. Ecosyst. Environ. 265 , 73–83 (2018).
Wang, Z. et al. Impact of stocking rate and rainfall on sheep performance in a desert steppe. Rangel. Ecol. Manag. 64 , 249–256 (2011).
Li, Z. et al. Identifying management strategies to improve sustainability and household income for herders on the desert steppe in Inner Mongolia, China. Agric. Syst. 132 , 62–72 (2015).
Shao, Q., Cao, W., Fan, J., Huang, L. & Xu, X. Effects of an ecological conservation and restoration project in the Three-River Source Region, China. J. Geogr. Sci. 27 , 183–204 (2017).
Li, X. L. et al. Restoration prospects for Heitutan degraded grassland in the Sanjiangyuan. J. Mt. Sci. 10 , 687–698 (2013).
Xu, Y. et al. Trade-offs and cost-benefit of ecosystem services of revegetated degraded alpine meadows over time on the Qinghai-Tibetan Plateau. Agric. Ecosyst. Environ. 279 , 130–138 (2019).
Dong, S. K. et al. Farmer and professional attitudes to the large-scale ban on livestock grazing of grasslands in China. Environ. Conserv. 34 , 246–254 (2007).
Download references
Acknowledgements
R.D.B. and N.O. acknowledge support from BBSRC in the form of a Global Challenge Research Fund Impact Acceleration Account (GCRF-IAA) award (BB/GCRF-IAA/14) and a GCRF Foundation Award (BB/P022987/1) “Restoring soil function and resilience to degraded grasslands”, and the N8 via an AgriFood Programme pump priming grant. U.S. acknowledges support from the Swiss Programme for Research on Global Issues for Development (r4d) “Woody invasive alien species in East Africa: assessing and mitigating their negative impact on ecosystem services and rural livelihood” (grant number 400440_152085) and core financial support from CABI and its member countries ( http://www.cabi.org/about-cabi/who-we-work-with/key-donors/ ).
Author information
Authors and affiliations.
Department of Earth and Environmental Sciences, The University of Manchester, Manchester, UK
Richard D. Bardgett, Mathilde Chomel, Ellen L. Fry, David Johnson, Jocelyn M. Lavallee & Kenny Png
UK Centre for Ecology & Hydrology (UKCEH), Wallingford, UK
James M. Bullock
Laboratoire d’Ecologie Alpine (LECA), Centre National de Recherche Scientifique (CNRS), Université Grenoble Alpes, Université Savoie Mont-Blanc, Grenoble, France
Sandra Lavorel
Senckenberg Biodiversity and Climate Research Centre, Frankfurt, Germany
Peter Manning & Gaëtane Le Provost
CABI, Delémont, Switzerland
Urs Schaffner
Lancaster Environment Centre, Lancaster University, Lancaster, UK
Nicholas Ostle & Shan Luo
Instituto Florestal de São Paulo, São Paulo, Brazil
Giselda Durigan
National Centre for Biological Sciences, Tata Institute of Fundamental Research, Bangalore, India
Mahesh Sankaran
School of Biology, University of Leeds, Leeds, UK
Institute of Grassland Research, Chinese Academy of Agricultural Sciences, Hohhot, China
Xiangyang Hou, Xiliang Li, Yong Ding, Yuanheng Li & Hongxiao Shi
Key Laboratory of Restoration Ecology of Cold Area in Qinghai Province, Northwest Institute of Plateau Biology, Chinese Academy of Sciences, Xining, Qinghai, China
Huakun Zhou & Li Ma
University of the Chinese Academy of Sciences, Beijing, China
School of Ecology and Environment, Inner Mongolia University, Hohhot, China
You can also search for this author in PubMed Google Scholar
Contributions
R.D.B. conceived the idea and gained funding with input from N.O. R.D.B. wrote the paper, with significant input from J.M.B., P.M., U.S. and S. Lavorel. G.L.P. and P.M. designed the figures. All authors contributed to the development of ideas and writing of the paper.
Corresponding author
Correspondence to Richard D. Bardgett .
Ethics declarations
Competing interests.
The authors declare no competing interests.
Additional information
Peer review information.
Nature Reviews Earth & Environment thanks Peter Török, Johannes Isselstein and the other, anonymous, reviewer(s) for their contribution to the peer review of this work.
Publisher’s note
Springer Nature remains neutral with regard to jurisdictional claims in published maps and institutional affiliations.
Supplementary information
Supplementary information, rights and permissions.
Reprints and permissions
About this article
Cite this article.
Bardgett, R.D., Bullock, J.M., Lavorel, S. et al. Combatting global grassland degradation. Nat Rev Earth Environ 2 , 720–735 (2021). https://doi.org/10.1038/s43017-021-00207-2
Download citation
Accepted : 19 July 2021
Published : 07 September 2021
Issue Date : October 2021
DOI : https://doi.org/10.1038/s43017-021-00207-2
Share this article
Anyone you share the following link with will be able to read this content:
Sorry, a shareable link is not currently available for this article.
Provided by the Springer Nature SharedIt content-sharing initiative
This article is cited by
Light grazing alleviates aeolian erosion–deposition effects on microbial communities in a semi-arid grassland.
- Mingming Cui
- Junqiang Zheng
Ecological Processes (2024)
Historical impacts of grazing on carbon stocks and climate mitigation opportunities
- César Terrer
Nature Climate Change (2024)
Nature-based Solutions can help restore degraded grasslands and increase carbon sequestration in the Tibetan Plateau
- Yingxin Wang
Communications Earth & Environment (2024)
A 10-m annual grazing intensity dataset in 2015–2021 for the largest temperate meadow steppe in China
- Chuchen Chang
- Yingjun Zhang
Scientific Data (2024)
Facile green synthesis route for new ecofriendly photo catalyst for degradation acid red 8 dye and nitrogen recovery
- Nouf F. Al Harby
- H. A. Fetouh
- Mervette El-Batouti
Scientific Reports (2024)
Quick links
- Explore articles by subject
- Guide to authors
- Editorial policies
Sign up for the Nature Briefing newsletter — what matters in science, free to your inbox daily.

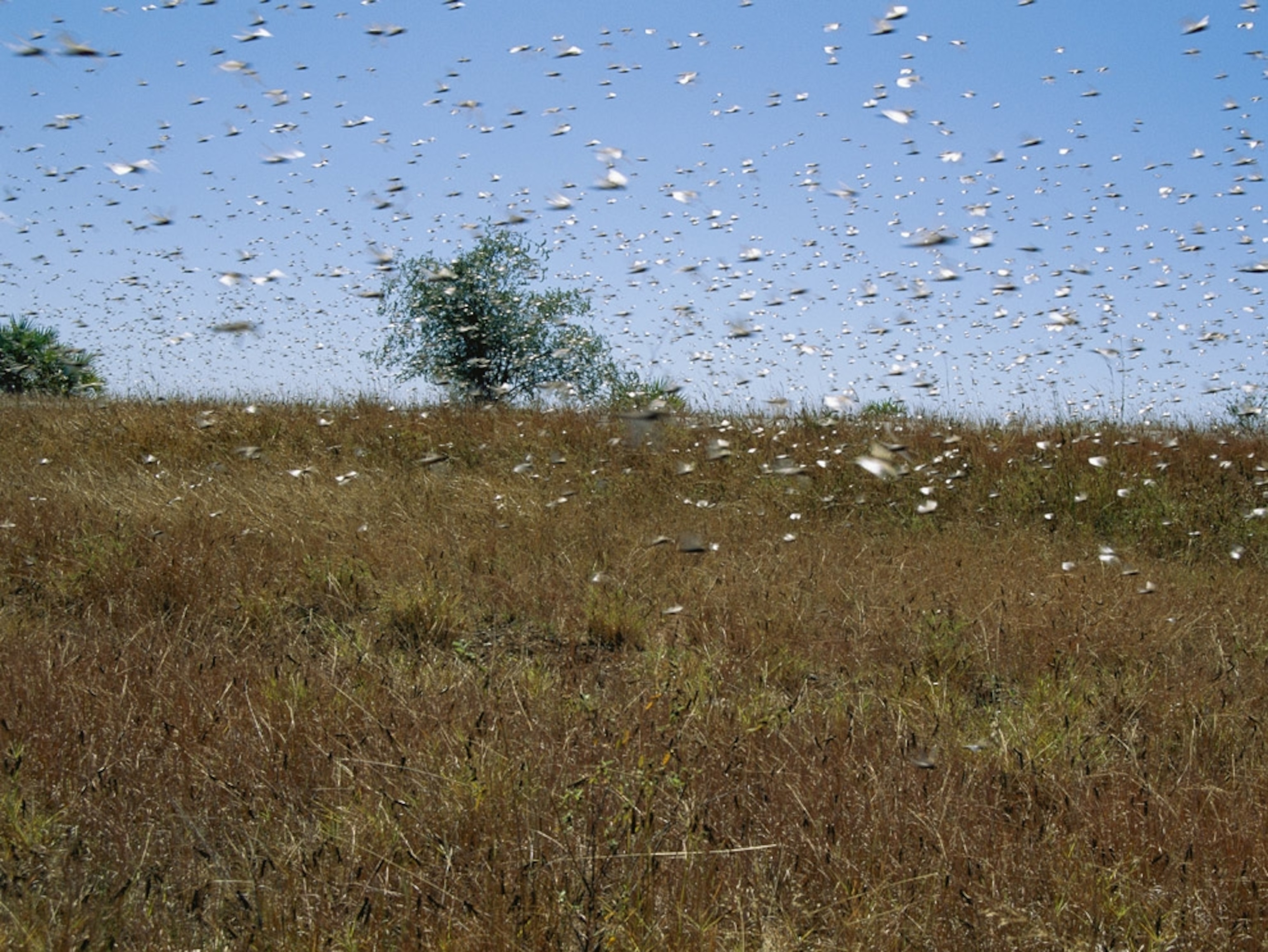
Insects swarm over the Madagascar savannah. Grasslands in different regions have different names: Africa has savannas; Asia has steppes; South America has pampas; and North America has prairies.
Grassland threats, explained
Much of Earth's grassland has been lost to agricultural development, threatening wildlife. But solutions are emerging.
Characterized by their flat, open pastures and abundance of nutrient-rich soil, more than a quarter of the world’s land—and about 70 percent of its agricultural land —is covered by grasslands.
This biome is home to a range of flora and fauna, which add to its resilience against natural disasters such as droughts or wildfires. In fact, native grassland plants have adapted to extreme weather conditions to such an extent that savannas, a subset of grasslands found in Africa, Australia, South America, and India, require seasonal droughts and wildfires to maintain biodiversity . (Read more about grasslands here.)
But this resiliency does not equate to immunity.
Grasslands are threatened by habitat loss , which can be caused by human actions, such as unsustainable agricultural practices, overgrazing, and crop clearing . Almost half of all temperate grasslands and 16 percent of tropical grasslands have been converted to agricultural or industrial uses and only one percent of the original tallgrass prairie exists today.
Specific threats to grasslands:
- Poor agricultural practices can ruin soil and strip grasslands of life. If crops are not rotated properly, the soil can become infertile and nothing can be grown for several years.
- Monocropping, or growing only one crop at a time (like corn) is an agricultural practice that depletes the soil’s nutrients. Further, because grasslands thrive off of biodiversity of plants and animals, monocropping that provides only a single type of plant tends to weaken the biome and increases vulnerability to natural disasters.
- Toxic pesticides used in agricultural croplands can be deadly for wild flora and fauna.
- Grazing livestock can consume, trample, and destroy grasses. Selective grazing can reduce the competitive nature of the entire ecosystem by weeding out some plants and allowing others to over-populate. Additionally, conventional agricultural croplands often provide few food sources and nesting areas for birds.
- Continued global warming could turn current marginal grasslands into deserts as rainfall patterns change.
- Development of urban areas is increasingly cutting into grassland habitat.
- Invasive species can displace native plants and reduce the quality of a grassland . Invasive plants may not be equipped to handle extreme weather, like droughts and wildfires, thus resulting in further habitat loss.
Solutions to grassland problems:
- Continue education efforts , particularly among farmers, on how to protect the soil and prevent soil erosion.
- Protect and restore wetlands , which are an important part of grassland ecology.
- Rotate agricultural crops to prevent the sapping of nutrients.
- Plant trees as windbreaks to reduce erosion on farmfields ( though make sure it is the right species for the area ).
- Conduct controlled dry season burning to stimulate fresh plant growth and to restore calcium to the soil that builds up in the dry grasses. Some species of native plants need occasional fire to thrive, while blazes can also help remove invasive species.
FREE BONUS ISSUE
Related topics.
- ENVIRONMENTAL CONCERNS
- HABITAT PRESERVATION
- AGRICULTURE
- SUSTAINABILITY
You May Also Like
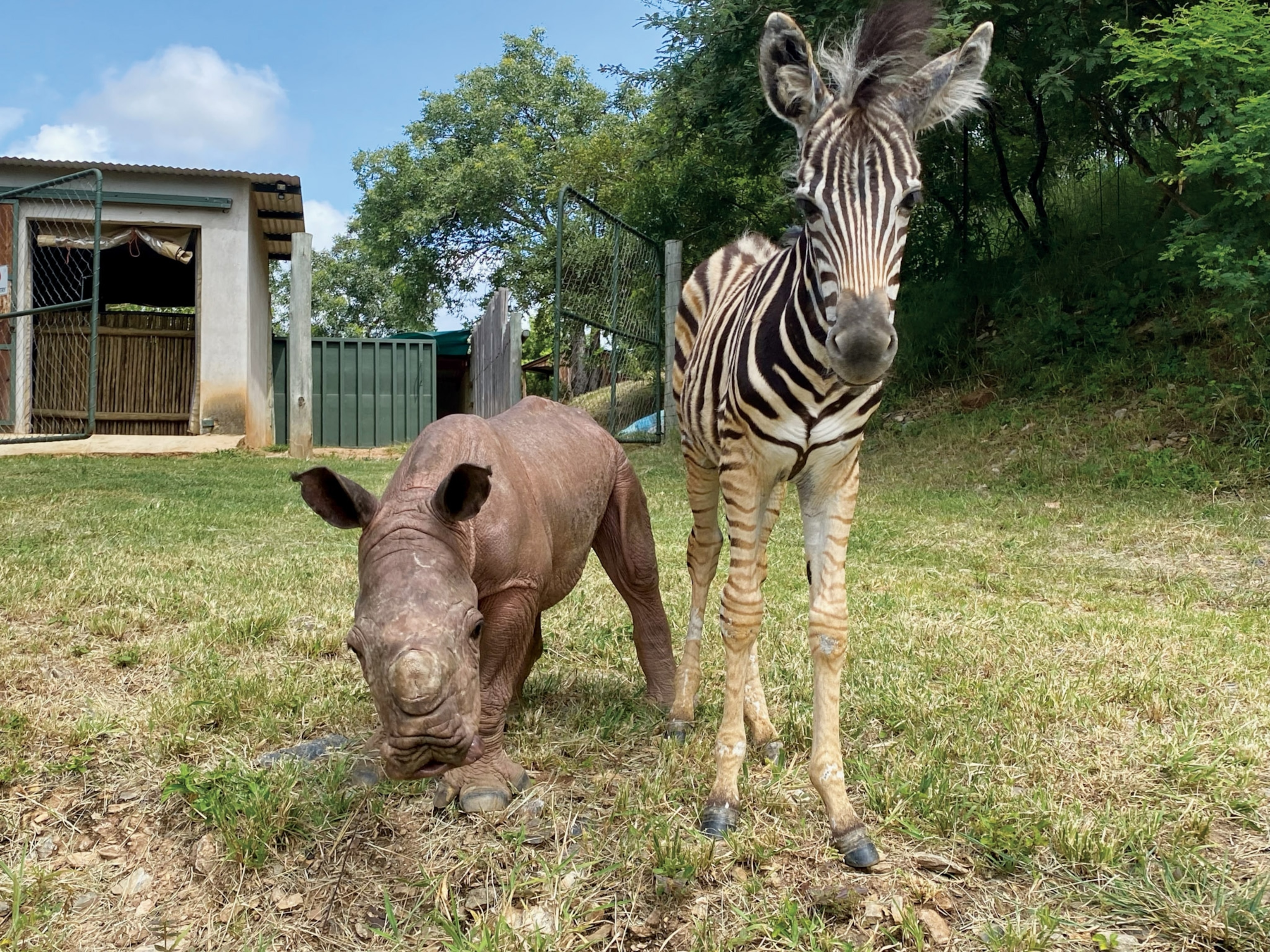
Why this rhino-zebra friendship makes perfect sense
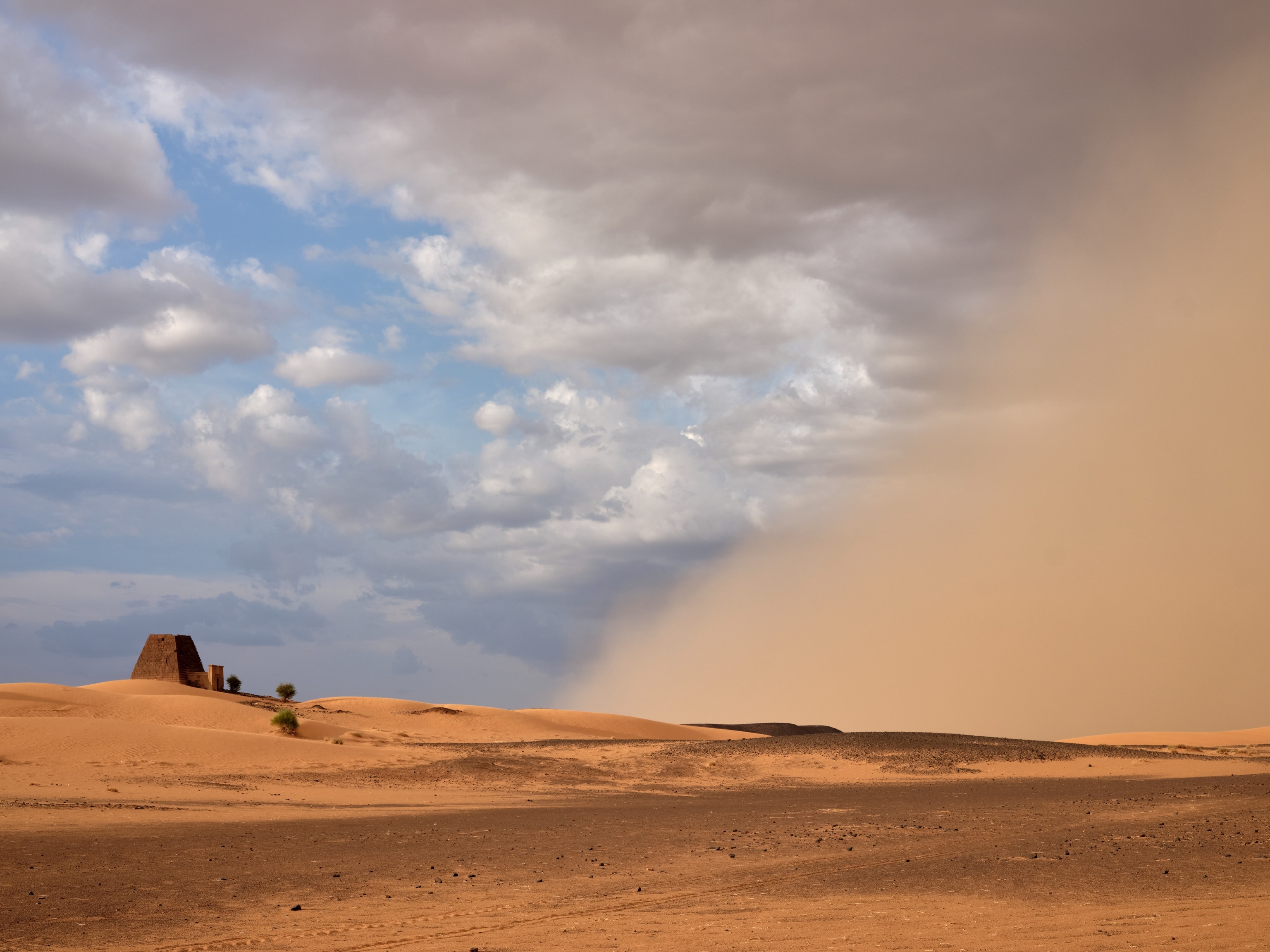
Are dust storms getting worse? Here’s why they’re so destructive.
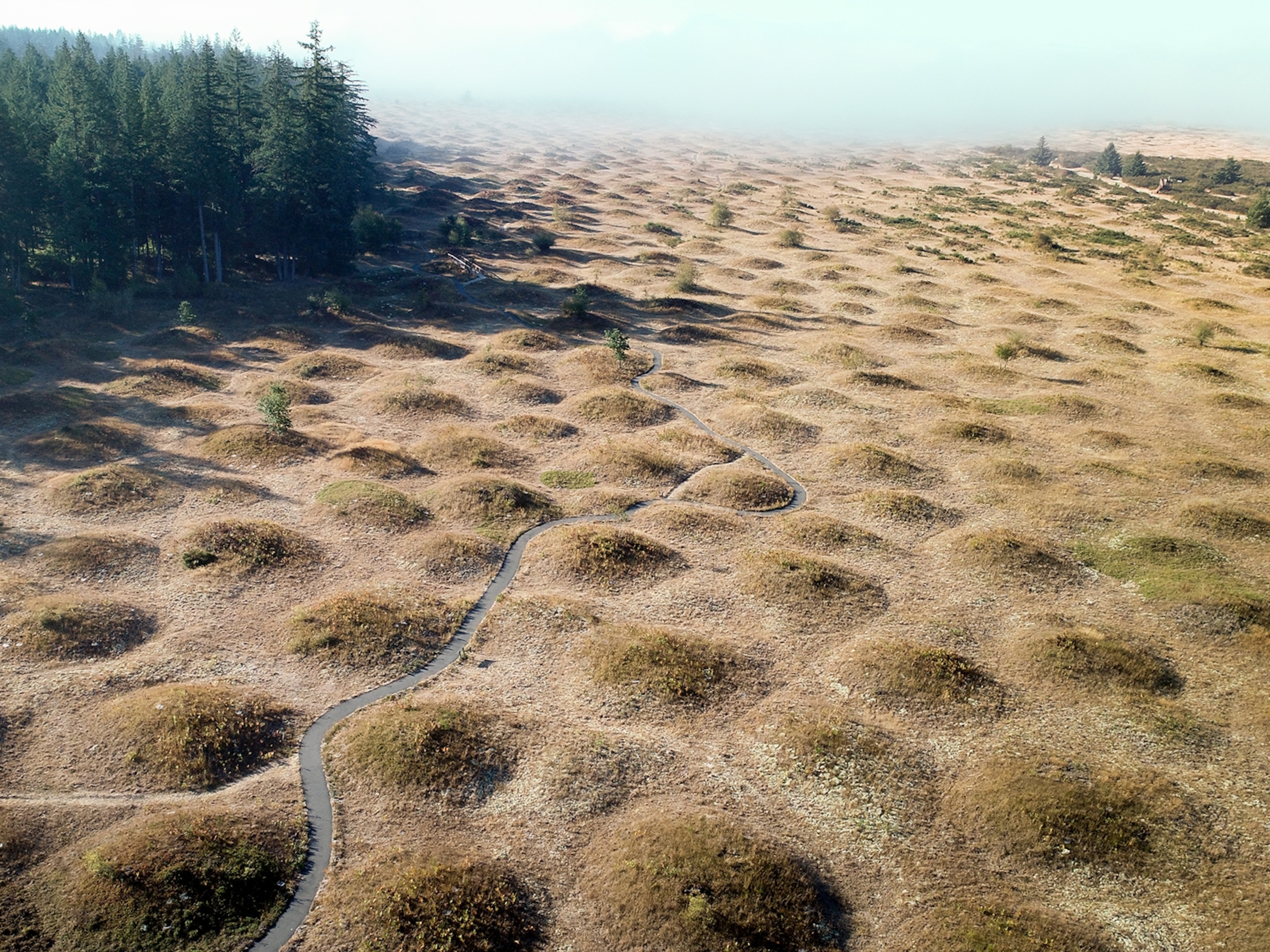
Will we ever solve the mystery of the Mima mounds?
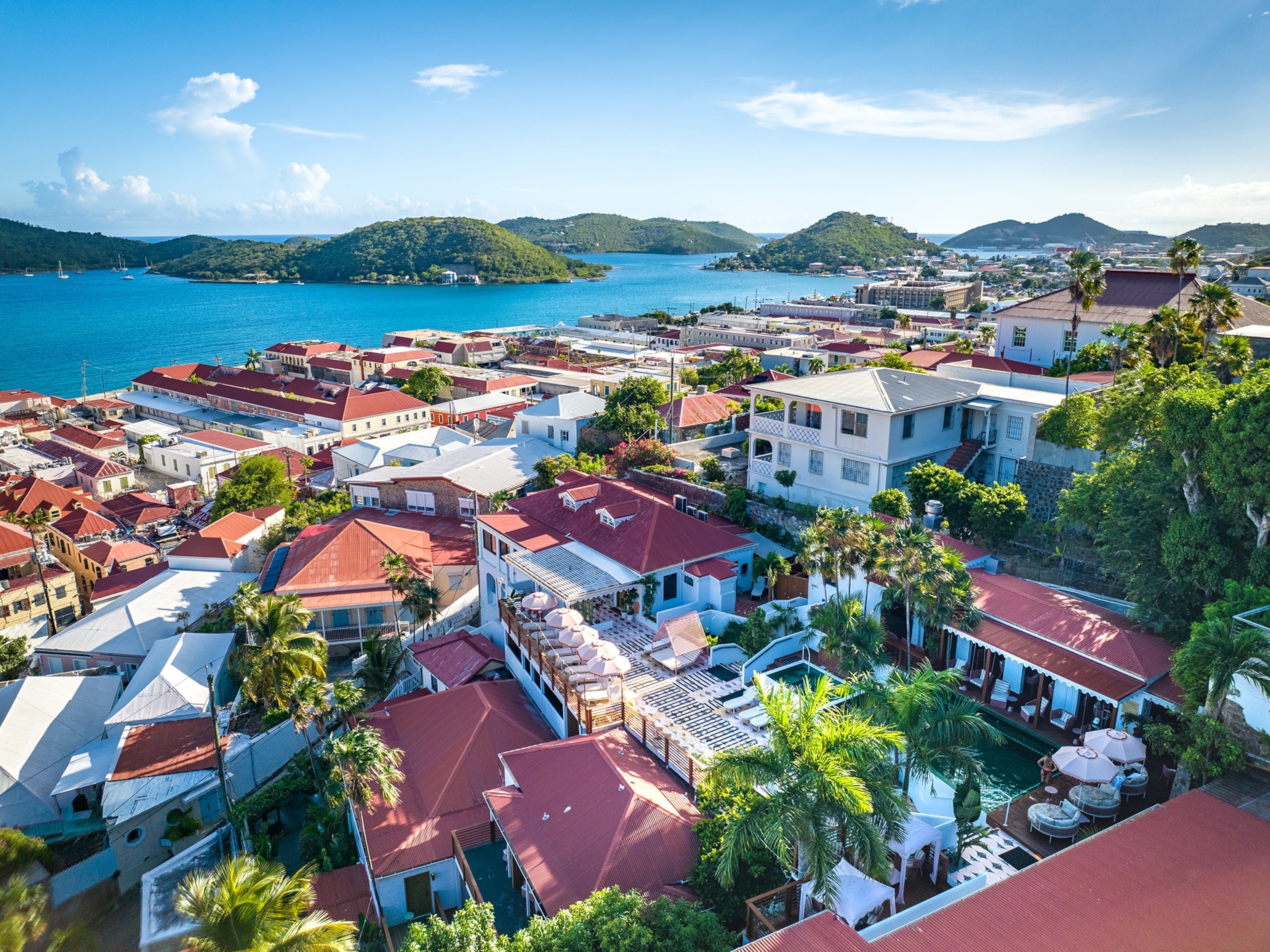
Here are the hotels we love for 2024
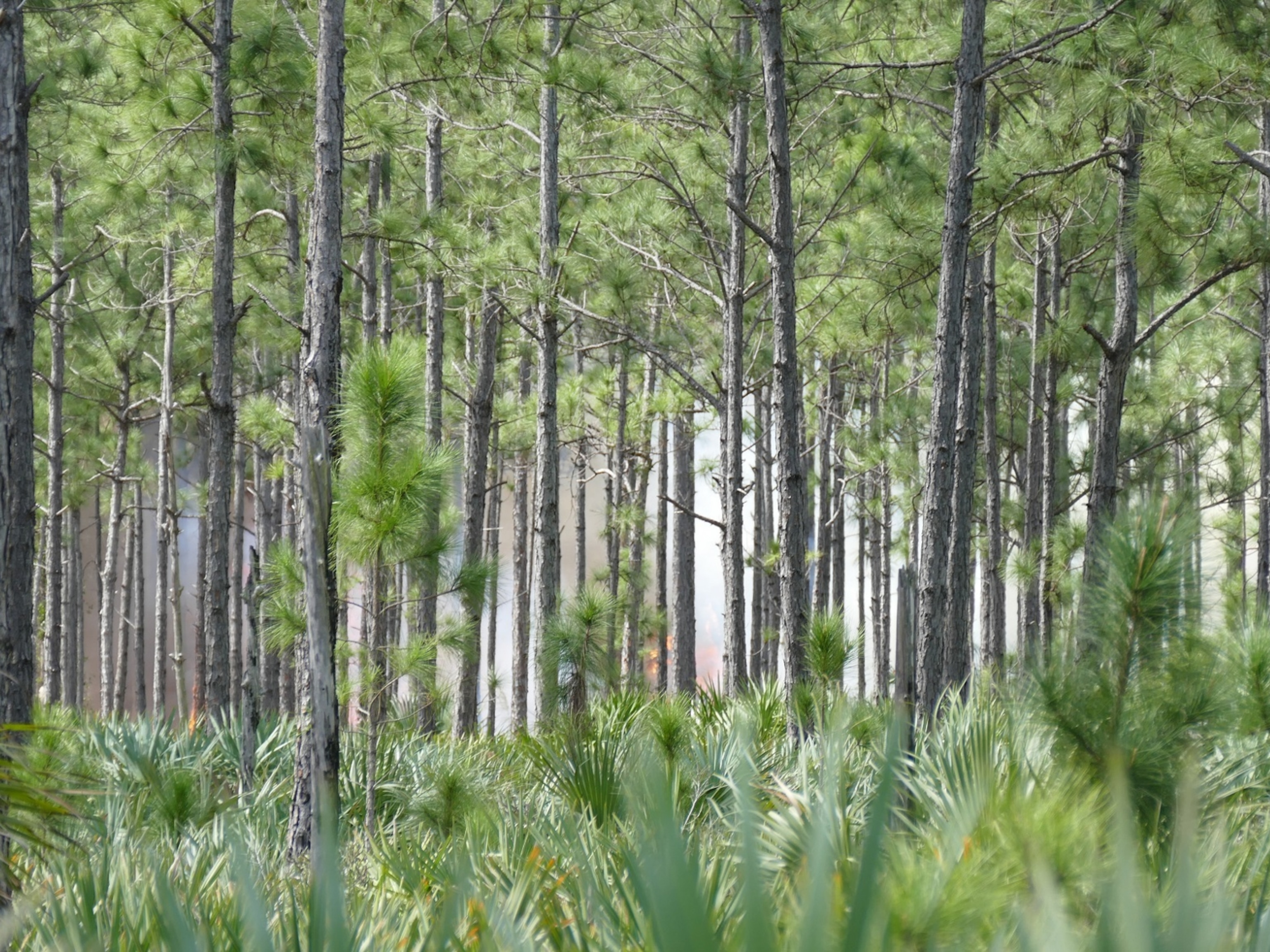
Is this paradise—or a parking lot? This Florida ecosystem is a battleground.
- Environment
- Perpetual Planet
History & Culture
- History & Culture
- History Magazine
- Mind, Body, Wonder
- Paid Content
- Terms of Use
- Privacy Policy
- Your US State Privacy Rights
- Children's Online Privacy Policy
- Interest-Based Ads
- About Nielsen Measurement
- Do Not Sell or Share My Personal Information
- Nat Geo Home
- Attend a Live Event
- Book a Trip
- Inspire Your Kids
- Shop Nat Geo
- Visit the D.C. Museum
- Learn About Our Impact
- Support Our Mission
- Advertise With Us
- Customer Service
- Renew Subscription
- Manage Your Subscription
- Work at Nat Geo
- Sign Up for Our Newsletters
- Contribute to Protect the Planet
Copyright © 1996-2015 National Geographic Society Copyright © 2015-2024 National Geographic Partners, LLC. All rights reserved

An official website of the United States government
The .gov means it’s official. Federal government websites often end in .gov or .mil. Before sharing sensitive information, make sure you’re on a federal government site.
The site is secure. The https:// ensures that you are connecting to the official website and that any information you provide is encrypted and transmitted securely.
- Publications
- Account settings
Preview improvements coming to the PMC website in October 2024. Learn More or Try it out now .
- Advanced Search
- Journal List
- Plants (Basel)

Research Progress of Grassland Ecosystem Structure and Stability and Inspiration for Improving Its Service Capacity in the Karst Desertification Control
1 School of Karst Science, Guizhou Normal University, Guiyang 550001, China
2 State Engineering Technology Institute for Karst Desertification Control of China, 116 Baoshan North Road, Guiyang 550001, China
Kangning Xiong
Shuzhen song, yongkuan chi, jinzhong fang, associated data.
Not applicable.
The structure and stability of grassland ecosystems have a significant impact on biodiversity, material cycling and productivity for ecosystem services. However, the issue of the structure and stability of grassland ecosystems has not been systematically reviewed. Based on the Web of Science (WOS) and China National Knowledge Infrastructure (CNKI) databases, we used the systematic-review method and screened 133 papers to describe and analyze the frontiers of research into the structure and stability of grassland ecosystems. The research results showed that: (1) The number of articles about the structure and stability of grassland ecosystems is gradually increasing, and the research themes are becoming increasingly diverse. (2) There is a high degree of consistency between the study area and the spatial distribution of grassland. (3) Based on the changes in ecosystem patterns and their interrelationships with ecosystem processes, we reviewed the research progress and landmark results on the structure, stability, structure–stability relationship and their influencing factors of grassland ecosystems; among them, the study of structure is the main research focus (51.12%), followed by the study of the influencing factors of structure and stability (37.57%). (4) Key scientific questions on structural optimization, stability enhancement and harmonizing the relationship between structure and stability are explored. (5) Based on the background of karst desertification control (KDC) and its geographical characteristics, three insights are proposed to optimize the spatial allocation, enhance the stability of grassland for rocky desertification control and coordinate the regulation mechanism of grassland structure and stability. This study provided some references for grassland managers and relevant policy makers to optimize the structure and enhance the stability of grassland ecosystems. It also provided important insights to enhance the service capacity of grassland ecosystems in KDC.
1. Introduction
Constituting almost 40% of the terrestrial biosphere, grasslands provide the habitat for a great number of diverse animals and plants and contribute to the livelihoods of more than 1 billion people worldwide [ 1 ]. Under the growing impact of global climate change and unreasonable human activities, grasslands are facing major problems that threaten the sustainable development of grassland ecosystems, such as a sharp decline in biodiversity, pasture degradation and reduced supply capacity [ 2 , 3 , 4 ]. Therefore, how to promote the healthy development of grassland ecosystems and enhance their service capacity has become an urgent issue [ 5 , 6 , 7 ]. Grassland ecosystem services are influenced by multiple factors such as their structure and stability. Furthermore, trade-offs and synergies between ecosystem services often depend on their structural and functional interactions [ 8 ]. A reasonable ecosystem structure can improve ecosystem productivity; promote material cycling, energy flow and information transfer; and increase the provision capacity of ecosystem service [ 9 ]. This plays a very important role in the healthy development of ecosystems and in human well-being. Rational allocation of grassland structure is one of the main measures to improve the stability and resilience of grassland ecosystems [ 10 ]. However, while the global concept of sustainability continues to spread, irrational structural configurations of grasslands (unreasonable cropping patterns and unscientific pasture management, such as planting density, species or grazing methods, etc.) continue to exist, which not only undermines the gains of ecological restoration and conservation, but also exacerbates the conflict between ecological conservation and economic development [ 11 ]. In particular, large-scale cultivation-based rangelands generally have a single planting structure, low biodiversity, high vulnerability and low stability, making it difficult to create a cascade of ecosystem service benefits [ 12 ]. As a result, grassland ecosystem structure and structural optimization are gradually becoming a research priority, with a focus on component structure, spatial and temporal structure, nutrient structure and their driving factors [ 13 , 14 ]. Optimizing the structure of grassland ecosystems is an important measure to improve and maintain the service capacity of grassland ecosystems [ 15 , 16 ], to balance grassland ecology and farmers’ livelihoods, and to resolve the contradictory issues of grassland ecology and sustainable economic development [ 17 ].
Structural changes drive changes in stability in grassland ecosystems [ 18 ], which, in turn, change their ecological functions and the provisioning capacity of an ecosystem [ 19 ]. At present, there are many studies about revealing the mechanisms of species diversity on grassland stability through controlled experiments in grassland [ 20 , 21 , 22 , 23 ]. The application of basic principles and methods of biochemistry to quantitatively study nutrient limitation and nutrient balance in forage, as well as the regulation mechanism of water–fertilizer coupling on forage quality, productivity and stability in grassland, which is the focus of the current research [ 24 , 25 , 26 ]. At the same time, studies on assessing the stability of grassland ecosystems are gradually emerging [ 27 ], such as those using remote sensing data; indicators characterizing ecosystem vitality, resilience and organization; and landscape pattern indices to evaluate ecosystem stability [ 28 , 29 ]. However, there is a lack of research regarding the mechanisms by which the complementarity and diversity of functional traits regulate the stability of grasslands, which results in a structure–process–function–service cascade [ 30 ]. What is more, there are different methods for quantifying ecosystem stability indicators, and the evaluation models are uneven; therefore, whether they are universally applicable remains to be verified [ 31 , 32 ]. Biodiversity and species diversity have an important influence on the productivity, stability and nutrient cycling of grasslands and their resistance and resilience to disturbance. Resilience, resistance and restoration are the main elements in determining whether an ecosystem is stable [ 33 ]. The study of the role of biodiversity, species diversity and functional traits on stability has been the focus of ecosystem research [ 34 , 35 , 36 , 37 ], and they are influenced by multiple factors on multiple scales [ 38 ]. It has been shown that extreme weather and irrational human activities are the main drivers of structural changes in grassland ecosystems [ 39 ], which indirectly alter ecosystem stability, increase the overall vulnerability of grassland ecosystems and reduce their capacity to provide ecosystem services [ 40 ]. Therefore, a deep understanding of the relationship between the structure and stability of grassland ecosystems is a major part of maintaining the stability of grassland ecosystems and enhancing their service capacity [ 41 , 42 , 43 ].
Due to their fragile attributes, ecologically fragile areas are prone to high ecosystem sensitivity and structural vulnerability under climate change and irrational human activities, which, in turn, reduces the stability of their ecosystems and changes their ability to supply services [ 44 ]. Therefore, the mutual interaction between ecosystem structure and stability should be deeply understood to enhance their resistance to human disturbances and environmental changes [ 45 , 46 ]. However, in ecologically fragile areas, irrational land use reduces the diversity of grassland species and productivity stability and affects the sustainable development of the region [ 47 ]. Especially in the environmentally fragile areas of karst, unreasonable human activities have led to the degradation of vegetation, increased soil erosion, gradual exposure of rocks and degradation of land productivity, with the surface showing a visual evolution similar to that of a desert landscape [ 48 , 49 ]. For this reason, the first task in the comprehensive control of rocky desertification is to restore and re-establish vegetation. In order to solve the ecological problem of karst desertification, the Chinese government has carried out a lot of work on the issue of karst desertification in southwest China since 1989, such as grain for green and closing the land for reforestation (grass), etc. The area of rocky desertification generally exhibits a trend of “continuous net reduction” [ 50 ], which provides a Chinese solution to global greening [ 51 , 52 , 53 , 54 ]. However, the KDC ecosystem still suffers from its simple structure, incomplete system function, high ecosystem sensitivity, lagging ecosystem service capacity and difficulty in maintaining the results of rocky-desertification management [ 55 ]. The rugged and fragmented surface in the karst areas, coupled with the constraints of rocky desertification, has affected the biodiversity of grassland ecosystems, resulting in a homogeneous structure and low productivity for the grassland [ 56 ]. Therefore, how to maintain ecological stability and optimize the structure of the system in the grassland ecosystems of KDC has become a key issue to consolidate the achievements of rocky-desertification management, ensure the smooth flow of service supply and demand, and enhance the well-being of local people [ 57 , 58 ]. Therefore, the structure and stability of grassland ecosystems and their interactions are not only a global concern [ 59 , 60 , 61 ], but also an important element that needs attention in KDC [ 62 , 63 ]. In the natural vegetation succession, grasses are the pioneer plants for vegetation restoration and ecological improvement [ 64 ]. Meanwhile, artificial grass breeding can enrich grassland species diversity, improve grassland ecosystem stability, provide high-quality forage, reduce the risk of surface erosion, improve soil nutrient composition and provide multiple ecosystem services for humans [ 51 , 65 , 66 ]. Optimizing the spatial configuration of systems is an important means of improving the stability and resilience of grassland ecosystems [ 67 ]. Therefore, optimizing the structure of grassland ecosystems for KDC and enhancing their stability are of great significance in enhancing the service capacity of grassland ecosystems and promoting the sustainable development of the regional ecological environment.
Thus far, research on the structure and stability of grassland ecosystems is increasing and breakthroughs have been achieved. The structure and stability of grassland is an essential element to maintain the sustainable and healthy operation of grassland in KDC, which is an important part of its ecosystem. However, there is a lack of relevant studies on the structure and stability of grassland ecosystems in KDC. In view of this, based on the perspective of grassland-ecosystem pattern change and its relationship with ecosystem processes, and the systematic review method, this study systematically reviewed the main research progress and landmark results on the structure and stability of global grassland ecosystems, and summarized the key scientific issues on structure optimization, stability enhancement and the interaction between structure and stability, aiming to provide some insights into grassland-ecosystem structure optimization and stability enhancement in KDC. In this way, it can enhance the supply of grassland ecosystem services and promote the sustainable development of the regional ecological environment and social economy in KDC.
2. Results and Discussion
2.1. research characteristics.
As shown in Figure 1 , the number of studies on the structure and stability of grassland ecosystems showed an overall fluctuating upward trend. From 1995 to 2004, the average annual number of articles did not exceed two, and it was in the budding stage. The number of papers published from 2005–2014 was 26, and the number of papers published during this period increased rapidly with the UN Millennium Ecosystem Assessment (MEA) synthesis report [ 68 ], with large fluctuations; this is the development phase. The number of articles published from 2015 to present was 97, mainly due to the convening of the United Nations Sustainable Development Goals (SDGs) [ 69 ]; the number of studies on ecosystem structure and stability shows a rapid upward trend, and the degree of research on concepts and mechanisms is gradually expanding and entering a diversified development stage.

Trend in the annual distribution of literature related to the structure and stability of grassland ecosystems (MEA: Millennium Ecosystem Assessment; SDGs: Sustainable Development Goals).
2.1.1. Stage Characteristics
Budding stage.
Research during the budding stage was mainly focused on theoretical studies of ecosystem structure and stability, and mechanisms of impact. The aim is mainly to improve the yield and quality of grassland forage through these studies and to meet the needs of human food security. The relationship between biodiversity and ecosystem function and productivity and the mechanisms that influence them have long been debated and have been shown to be positively correlated in national and international studies [ 70 ]. For example, through field experiments controlling for plant species diversity, functional diversity and functional composition, Tilman et al. concluded that these three factors are the main determinants of plant productivity, total plant nitrogen and light infiltration capacity, which, in turn, have a significant impact on ecosystems [ 71 ]. Spehn et al. monitored range biomass production, resource use (space, light and nitrogen) and decomposition processes on eight European pastures with different plant species richness, showing that higher species and functional group diversity also resulted in higher yields and more efficient use of resources [ 72 ]. These scholars have mainly observed changes in grassland biodiversity and productivity from the field scale, which is only a single-sided influence process, and have not addressed the interaction mechanisms between grassland species diversity, and biodiversity, etc., and stability. Moreover, the scale of the study is limited to the field scale, which does not involve the regional or global scale. Therefore, it remained uncertain at this stage how the findings would be extended to the landscape and regional level, and how they would be extended across different ecosystem types and processes [ 73 ].
Development Stage
Facing the threat of increased environmental pollution and the endangerment of cherished flora and fauna, the United Nations hosted the MEA, which motivated the conservation and sustainable development of ecosystems and was a phase of development in the study of the structure and stability of grassland ecosystems.
The study of factors that influence the structure and stability of grassland ecosystems was becoming progressively more advanced. How to manage pastures scientifically so that they can maintain healthy grassland ecology while balancing pasture production were the main issue of research during this period. For example, Isbell et al. conducted a long-term N enrichment experiment on grasslands to analyze the effects of N enrichment on productivity, plant diversity and the species composition of natural grasslands. The results showed that in the early stages, nitrogen enrichment increased grassland productivity, but over time, nitrogen enrichment was negatively correlated with species diversity and productivity [ 74 ]. Thus, scientific anthropogenic fertilization, generalizable results derived from experimental fertilization, is an important factor driving grassland biodiversity and species composition. Facing the problem that the productivity of natural grasslands cannot meet the current demand for livestock feed, the improvement of natural grasslands and the optimization of the planting structure of artificial grasslands became the focus of research at this stage [ 75 ]. For example, Albayrak et al. conducted experiments with seed mixes of oats and vetch on pastures in the highlands of Madagascar. The experiments showed that the mixed planting not only enhanced forage yield and quality, but also improved resource utilization [ 76 ]. Meanwhile, methods for evaluating the stability of grassland ecosystems are gradually arising. Zheng et al. used the fuzzy comprehensive evaluation method to evaluate the stability of mixed-seeded grassland in terms of community components, function and resistance to invasion. The results showed that the mixed seeding species and the proportion of mixed seeding could influence the community stability; however, this did not play a decisive role [ 77 ]. Therefore, how to scientifically quantify the stability of legume–grass mixed grassland communities, considering the temporal scale, spatial scale and their corresponding sensitive indicators, etc., is one of the issues that needed to be urgently explored at this stage.
In summary, research on the structure and stability of grassland ecosystems has gone through a budding stage and a development stage, which have developed towards diversification. In the budding stage, qualitative theoretical studies and influence mechanisms are the focus; in the development stage, with the aim of maintaining the stability of grassland ecosystems and protecting and promoting their sustainable development, a series of studies on the structure, function, stability influence mechanisms and stability evaluation of grassland ecosystems were carried out. Since then, the research directions and themes of ecosystem structure and stability have gradually developed in a diversified manner.
Diversification Stage
In order to thoroughly solve the development problems in the social, economic and environmental dimensions and shift to a sustainable development path, the United Nations Sustainable Development Summit put forward the SDGs, and many countries and regions have responded to the goals of poverty eradication, hunger eradication and climate change, etc. How to optimize the structure and stability of grassland ecosystems to promote sustainable development of ecosystems has become an issue that needs to be solved at the stage of diversified development [ 78 , 79 ].
With the gradual progress of research, the research at the stage of diversified development is mainly focused on the control experiments of grassland, to reveal the influence mechanism of species diversity and stability [ 80 ], and to quantitatively study the nutrients and productivity of pasture, and the regulatory mechanism of stability [ 24 ]. In addition, with the development of technology, data disclosure and the rise of multiple research methods (GIS technology, etc.), different spatial and temporal comparative studies have been widely performed.
The exploration of the mechanisms influencing species configuration and productivity and stability is a prerequisite for exploring the scientific management of grasslands and improving their productivity and stability. For example, Prieto et al. studied the effects of grassland productivity and sustainable supply capacity through species and genetic diversity. The results show that the complementary effects of taxonomy and genetic diversity can increase productivity under conditions of multi-grass species configurations, which, in turn, increases the productivity and resilience of grasslands in the face of environmental hazards [ 81 ]. Quantitative studies of nutrient limitation and balance and water–fertilizer coupling in forages are important processes to improve forage quality, productivity, and resistance to invasion [ 23 ]. Niu et al. assessed the effect of nitrogen enrichment on the stability of semi-arid grassland ecosystems in northern China and its potential mechanisms by simulating atmospheric nitrogen enrichment. The results showed that community stability was non-linearly related to nitrogen enrichment and that this relationship was positively correlated with species asynchrony, species richness and species diversity, as well as the stability of dominant species and the stability of grassland functional groups [ 82 ]. Therefore, it is important to re-evaluate the mechanisms by which multiple levels of nitrogen deposition affect the stability of natural ecosystems to gain a deeper understanding of the multiple nutrient inputs to grasslands and their stability response mechanisms.
In summary, the optimization and stabilization of grassland ecosystem structure can provide important basic research to elucidate the mechanisms of the complementarity and functional diversity of functional traits of forage grasses, as well as the synergy and trade-off between productive and ecological functions of grassland, thus promoting the healthy and sustainable development of grassland. We believe that, based on the idea of cascading benefits of grassland ecosystem pattern, process, function, and services, we should explore the mechanisms of multi-species configurations or natural grassland improvement and stability maintenance, and clarify how the species configuration of grassland (leguminous–grass, annual–perennial, and deep-rooted–shallow-rooted, etc.) affects the nutrient flow of grassland, which, in turn, affects community stability, and ultimately promotes the synergistic development of grassland-ecosystem productivity and stability.
2.1.2. Stage Characteristics
Table 1 illustrates the global distribution of studies related to the structure and stability of grassland ecosystems. China and the USA have the highest number of published literature, with over 20 articles, which shows the concern for global issues such as the sustainable development of grasslands and food security in those countries [ 5 , 83 ]. Developed countries such as Europe and Oceania also published a relatively significant amount of literature. In addition, countries such as Brazil and South Africa account for a relatively small number of publications.
Distribution of the number of publications issued by countries or region.
Due to regional differences in natural economic and social conditions, research on grassland ecosystems has developed unevenly and has prominent regional characteristics. In terms of the number of publications, Asia accounts for 47.8%, which is related to national policy support and the attention of research institutions [ 84 ]. In the Asian region, China is the main publication country, with most of the research focus on large-scale natural grasslands in the provinces of Tibet, Inner Mongolia and Xinjiang [ 85 ], where the restoration of these grasslands is of great importance to China in realizing the “two mountains theory” of “Lucid waters and lush mountains are invaluable assets.” [ 86 ]. The emergence of global issues (global warming, land degradation and resource crises) has led to sustainable and green development being a crucial issue in the 21st century, as exemplified by the United Nations SDGs. As a result, publications focused on the restoration of grassland ecosystems are gradually increasing, with particular attention being paid by countries in North America. In addition, with a large proportion of grasslands in North and South America, particularly in the Pampas, which has always been an export area for beef cattle, the sustainability of grassland ecosystems determines the economic lifeblood of the entire region. Similarly, although Europe does not have the same area of grassland as Asia or the Americas, food mainly comes from pastureland due to dietary habits, etc. As a result, the stable output of pastureland is vital to Europe’s survival [ 87 ]. Oceania is the region with the highest exports of wool and dairy products, and the quality of forage is related to the value of trade in export commodities and is equally important to the economy of the region as a whole.
The low number of publications in Africa is mainly related to the economic development of Africa, where most of the countries are still developing countries and the primary concern is to solve the problem of food and maintain national security and stability. Thus, although the area of grassland in Africa is large, and the sustainable development of savannah, in particular, is of great importance for maintaining the global climate, it is mostly studied by developed countries, such as the USA and Denmark [ 88 , 89 , 90 ]. South Africa is one of the few African countries with a significant volume of publications due to its better economic conditions.
2.2. Research Progress and Major Landmark Results
Based on the titles, keywords, abstracts and previous studies of the literature [ 91 ], drawing on the studies of Wang et al. and Fu et al. and the changes in grassland ecosystem patterns and their relationship with ecological processes [ 92 , 93 ], the 133 papers were divided into five aspects: structural studies (component structure, trophic structure, spatio-temporal structure), structural optimization, stability studies, structure–stability relationships and influencing factors ( Figure 2 ), according to structural composition, ecological processes (replaced by stability) [ 94 , 95 ], the relationship between structure and stability, and factors influenced by the environment. The largest portion of literature focused on the factors influencing ecosystem structure and stability, at 31.5%, followed by structure studies, which accounted for 30% of this type of literature. In addition, studies on ecosystem stability accounted for 17.2% of the literature, while studies on ecosystem structure optimization and structure–stability relationships accounted for 12.7% and 8%, respectively.

Grassland ecosystem structure and stability research content division. (From left to right, there is the legend of structure studies; structure optimization; stability studies; relationship between structure and stability; and influencing factors of structure and stability).
Grasslands include the degraded, natural, and artificial, which determine the type of ecosystem structure and species composition of their habitats. This alters the process of ecosystem material cycling and information transfer through the component structure, spatial and temporal structure and nutrient structure [ 96 ], influencing the degree of ecosystem stability and, thus, the supply of ecosystem service capacity [ 97 ]. It also combines with artificial capital to form multiple forms of animal husbandry, which enter the market and, further, form an industrial chain which provides ecosystem services and benefits to humans. Therefore, attention to the structural composition and stability of grassland ecosystems is a prerequisite for interpreting the processes that shape grassland-ecosystem services.
2.2.1. Structure Research
Grassland ecosystem structure refers to the relatively ordered and stable state of the various components of an ecosystem in space and time [ 98 ], including component structure, nutrient structure and spatial and temporal structure [ 99 ].
Component Structure
The structure of grassland ecosystems consists of biotic (plants, animals, micro-organisms) and abiotic (water, air, soil) factors, and a certain hierarchy and structural pattern is maintained between the components of each system. The composition of grasslands is the basis of all grassland research, and the analysis of changes in species composition and their response to grassland has always been an important part of grassland research [ 100 , 101 , 102 ]. Silva Mota et al. evaluated changes in floristic composition, structure, diversity, and life-forms spectra along an altitudinal gradient in the rupestrian grasslands in the south-eastern Espinhaço Mountains Range, and the results showed that differences in vegetation structure, diversity, species composition, frequency and the richness of each life form in relation to soil attributes and elevation. Plant height, species richness, diversity and evenness, frequency and richness of phanerophytes and chamaephytes decreased with elevation. The related results indicated that soil is an important driver of community change [ 103 ]. At the same time, human activities affect the species composition of grassland, and, thus, changing the cascading benefits of research into ecosystem services has become an emerging research direction. However, it is difficult to solve the various problems of grassland composition alone at the present stage. Therefore, the above studies were conducted in combination with spatial and temporal changes [ 104 ].
Spatial and Temporal Structure
The characteristics of ecosystems depend on biodiversity, that is, the functional characteristics of organisms present in the ecosystem, and the distribution and abundance of these organisms in space and time [ 105 ]. Due to species evolution and turnover, and a lack of scientific grassland management measures, the species diversity of grassland is gradually homogenizing, the soils are gradually being degraded, and the productivity is gradually decreasing [ 106 ]. Therefore, study on the spatial and temporal structure of grassland ecosystem provides a good reference for improving the service capacity of grassland ecosystem in the KDC area.
Time Structure
Quantitative assessment of the interannual variability in grassland landscape patterns in response to their ecosystem service values is a current research hot spot [ 107 ]. Monitoring the spatial and temporal dynamics of grassland areas can help decision makers to plan the use of grassland in a rational manner and achieve the sustainable development of grassland [ 108 ]. Landscape changes directly affect changes in the demand and supply of ecosystem services. Compared to the spatial scale of ecosystem services, little attention has been paid to how interannual changes in land use affects ecosystem service trade-offs, but this is necessary to facilitate the rationalization of decisions, especially when those decisions aim to re-establish diverse services and restore biodiversity [ 109 ]. Therefore, it is important to analyze the spatial and temporal evolution characteristics of grasslands and elucidate the main drivers of grassland-ecosystem service capacity to develop reasonable ecological restoration measures for grasslands. Recent studies have shown that current research has focused too much on global, national, and provincial spatial and temporal dynamics at large scales, neglecting research on the mechanisms of trade-off synergy and value transfer of grassland-ecosystem service functions at small scales in counties and sample sites [ 107 ]. Therefore, it is important to conduct overall monitoring of grassland landscape patterns in different periods in a specific region, analyze the temporal variability and heterogeneity of grassland-ecosystem landscapes at spatial scales, and grasp the structural-change characteristics of grassland ecosystems, to promote the optimization of the overall ecosystem structure and stability enhancement.
Seasonal changes in grasslands are also a current research hotspot in ecological restoration. The physical characteristics of plant growth in grassland determine its seasonal variation, which can lead to changes in ecosystem structure in the short term [ 110 ]. Thus, the changes in the species composition of grasslands in the short term can affect not only the above- and below-ground productivity supply of grasslands, but also the quality of forage and, in turn, the supply of multiple ecosystem functions. Rodriguez Barrera et al. compared the effects of groundhog and grassland use types and their seasonal changes on grassland vegetation structure and diversity in the semi-arid grassland in northern Mexico. The results of this study showed that grassland use type and its seasonal variation were the main determinants of grassland vegetation structure and cover [ 111 ]. However, current research on ecosystem services is mainly focused on inter-annual variability, with less research on monthly and seasonal variability [ 112 ]. Therefore, the interannual and seasonal changes in grassland-ecosystem structure should be strengthened in future studies.
Spatial Structure
Some studies have specifically classified grassland structure into vertical canopy structure and horizontal planting structure [ 113 , 114 ]. Therefore, the spatial structure of grassland ecosystems is discussed in terms of vertical and horizontal structure.
In terms of vertical structure, the three-dimensional structure of forest grassland is globally recognized as a stable state of ecosystems [ 115 , 116 ]. Studies have shown that a reasonable three-dimensional structure can effectively use light, heat, water, air and other environmental factors to improve productivity and maintain the stability of ecosystems [ 117 ], and that a composite ecosystem structure has a better soil retention effect [ 118 ]. The ecosystems of economic fruit forests and agroforestry are typical composite ecosystems, of which grassland is an essential and important component. The shallow roots of grassland combined with the deep roots of trees provide effective use of natural elements such as light and hot water and air, and intercept water through the multi-layered canopy, thus preventing flooding and providing resistance to erosion and, thus, having a good soil and water conservation effect [ 119 ].
In terms of horizontal structure, the optimal combination of multiple species can improve the productivity of grassland ecosystems and promote the efficient recovery of degraded grasslands, thus enhancing the stability and service functions of grassland ecosystems [ 120 ]. It has been proven that the persistence and stability of forage production in grassland ecosystems is generally higher than mixed planting with perennial grass species and single-forage cultivation [ 121 , 122 ]. Mixing planting with leguminous forages and replanting natural grasslands with leguminous forages can increase the productivity of grasslands, enhance their resilience and biodiversity, maintain the stability of grassland ecosystems and improve their service capacity [ 123 ]. By monitoring light cut-offs in the canopy of grassland communities with high species diversity, researchers found a positive correlation between plant species richness and multiple ecosystem functions, at which time grassland resource utilization was high. The relationship between grassland biodiversity-productivity and management intensity was also positively correlated when supplemented by sound grassland management [ 124 ]. Thus, a rational vertical canopy structure and a scientific horizontal structure not only maintain the biodiversity of the grassland, but also ensure its productivity, thus providing the basis for multi-functional grassland management [ 125 ]. In a protected area in central Italy (“Laghi di Suviana e Brasimone” regional park), Cervasio et al. showed that mixed seeding had a significant contribution to improving grassland quality and had a positive impact on grassland ecosystem biodiversity by changing the vertical and horizontal characteristics of grassland ecosystem species [ 126 ].
In addition, ecological corridors with a predominantly grassland landscape structure are also an important part of current spatial-structure research [ 127 , 128 ]. Ecological corridors are important links between the structure and function of a system, and grasslands can be used as buffer zones to improve the connectivity of ecological functions, allowing species to use corridors for long-distance dispersal or to provide shelter for some animal movements [ 129 ], thus maintaining and protecting biodiversity and improving the quality of their habitats [ 130 , 131 ]. At the same time, the spatial pattern of the landscape influences the magnitude of ecosystem service provision at the landscape scale through grassland use patterns and connectivity [ 132 , 133 , 134 , 135 ]. By quantifying inter-annual and seasonal changes in grassland landscape patterns in northern China, Hao et al. showed that degraded vegetation can influence changes in ecosystem service functions by changing land use patterns, increasing or dividing patch sizes of grassland and agricultural land, and increasing the size of forest areas where appropriate [ 136 ]. This is a very good reference for the grassland ecological protection of KDC. At this stage, however, most studies are still at the stage of qualitative description, and these mainly explore the factors influencing ecological functions (such as biomass production and other ecosystem services) and plant community characteristics [ 137 ]. There is also an urgent need to use these insights to develop combinations of grass species for high-yielding, high-quality communities [ 138 ], as the research subjects and geographical environments change and whether regional research results can be adapted to global environments or specific geographical areas, such as karst desertification areas and alpine grassland regions.
The study of the spatial structure of grassland ecosystems should focus on the vertical and horizontal cropping structure of grasslands and the distribution of grassland landscape patterns. This not only helps researchers to monitor the dynamics of grasslands comprehensively from a micro to macro level, but also helps grasslands (as an ecological linkage corridor) to provide better connectivity to other ecosystems, guaranteeing the healthy development of the whole terrestrial ecosystem and providing more and better service functions for humans [ 139 ]. Studies have found that increasing the abundance, evenness and diversity of dominant species not only effectively improves the productivity of the system [ 140 , 141 ], but also ensures the harmonious development of the “production-living-ecological function” of grassland ecosystems [ 142 , 143 ], maintaining the stability of their productive functions and the diversity of biological species. In summary, the vertical and horizontal structural characteristics of grasslands change the species composition and biodiversity of grasslands, affecting the quality of grassland habitats and their ability to provide services.
Trophic Structure
The study of trophic structure, food webs and ecological networks in ecosystems is the theoretical basis for understanding the composition, structure and dynamics of ecosystems, and provides indispensable scientific support for biodiversity conservation, ecosystem management and restoration, as well as response to global change [ 99 ]. The trophic structure of an ecosystem refers to the food chains and food webs formed by producers, consumers and decomposers in a biome with food as the link, which constitutes the main pathway for material cycling and energy flow [ 144 ]. Food webs are based on the interactions between species at different trophic levels, forming upstream and downstream regulatory mechanisms to maintain their structural stability [ 145 ].
Species interactions or alterations are one of the most important areas in the study of food webs. Food webs, which depict networks of trophic relationships in ecosystems, provide complex yet tractable depictions of biodiversity, species interactions, and ecosystem structure and function [ 146 ]. Nearly all ecosystems have been altered by human activities; these changes may modify species interactions and food-web stability [ 147 ]. De Castro et al. started from the classic soil food-web model of Hunt to formulate a plausible topology of soil food-web models and then compare the effect of this topology with those of random topologies [ 148 , 149 ]. The results showed that the stronger the species interactions in the soil, the more stable the food web, and that disrupting the functional-group assemblages and strength of interactions in the soil food web inevitably has a significant impact on species and their relative abundance. Duchardt et al. applied structural equation models (SEM) to disentangle direct and indirect effects of prairie dogs on multiple trophic levels (vegetation, arthropods, and birds) in the Thunder Basin National Grassland, and the results indicated that prairie dogs directly or indirectly influence associated vegetation, arthropods, and avifauna [ 150 ].
In summary, changes in key traits that sustain interactions are one of the most important factors in determining the stability of food webs [ 151 ]. Therefore, the study of species interactions is important for clarifying inter-species relationships and food-web driving mechanisms, which is one of the priorities that should be focused on in the future.
2.2.2. Structure Optimization
Structural-optimization measures can change the species composition of grasslands, thus affecting their ecosystem biodiversity and altering their capacity to supply ecosystem functions. There are many ways to optimize structure, but this study will only discuss biological measures (optimal allocation of species structure) and engineering measures (pasture management systems).
Scientific and rational species allocation is the key to the high productivity and stability of grassland ecosystems [ 152 ]. Optimal species structure allocation includes the optimal vertical and horizontal allocation of grassland species. The three-dimensional structure of composite ecosystems has proven to be an important strategy for reconciling environmental protection and economic development in ecologically fragile areas [ 153 ], with grasslands being the most crucial aspect, and their strong renewal rate making them a large surface area within the composite ecosystem. The resulting three-dimensional ecosystem structure not only plays an important role in maintaining and providing ecosystem services, but its multiple ecosystem services also provide an effective way to promote the restoration of degraded ecosystems, which is an important biological measure to make full use of resources [ 117 , 154 ]. A diverse three-dimensional and horizontal structure is an effective measure to restore grassland ecosystems, that is, through a combination of different species of grasses from different families (in intercropping, crop rotation, and mixed sowing, etc.; see Figure 3 ) [ 155 ]. The most studied of these is the mixed cropping pattern of legumes and grasses. This mixed cropping pattern makes full use of sunlight, heat, water and air, creates complementarity between species, and promotes the uptake of soil nutrients by forage grasses, which, in turn, improves their annual growth and nitrogen use efficiency [ 156 ]. Multi-species planting with different habits is an effective means of restoring grassland ecosystems, and it can effectively improve the degradation of grasslands, protect and improve soil quality, enrich inter-species and intra-species species diversity, and effectively increase the resilience and recovery of degraded grassland ecosystems [ 157 ]. Scientific seeding is an effective measure to restore the dynamic balance of grass species [ 158 ]. Under the same circumstances, mixed-seeded grasslands have higher forage density and species richness, and it has more competitive and better grassland vegetation restoration compared to single-seeded grasslands. However, it has been demonstrated that species diversity and stability in grassland ecosystems with different rates of mixed seeding are positively correlated, and that seeding rates are negatively correlated with resistance to invasion [ 159 ]. Therefore, understanding forage seeding rates and their effective mix relationships is an important area of vegetation-restoration research [ 160 ]. Meanwhile, the mixing and intercropping of annual and perennial forages are also efficient management practices that take advantage of the temporal structure of grassland ecosystems, reducing inter-specific competition and increasing the persistence of forage production [ 161 ]. Mixing annual and perennial legume-grass is recognized as a highly productive and robust management practice [ 162 ], which can maintain a diverse supply of ecosystem services, and the leguminous forage grasses provide a more adequate supply of nitrogen to other grass species, thus maintaining high grass biomass and meeting the production requirements of farmers [ 163 ].

Artificial grassland planting structure. ( a ) Forage mono sowing: Grass forage, Lolium perenne L., ( b ) Pasture intercropping: Grasses-Leguminosae Forage, Lolium perenne L. + Medicago sativa L., Trifolium repens L.+ Lolium perenne L., ( c ) Pasture mix sowing: Grasses-Leguminosae Forage, Lolium perenne L. + Medicago sativa L., ( d ) Forage high and low mix sowing: Lolium perenne L. + Medicago sativa L. + Trifolium repens L. + Lolium perenne L. (The grass species of ( b – d ) are the same, but the arrangement of planting order is different. ( b , c ) are planted in a horizontal way, but the density and proportion of planting are different. ( b ) is good for personnel to manage, and ( c ) is good for the nutrient complementation of grass species. ( d ) is planted in a vertical way, which can fully utilize light, heat, water and air).
A scientific system of pasture management can effectively enhance the sustainable supply of grassland resources. Pasture management not only includes grass seed cultivation and fertilization, but also includes the full use of time and space, such as rotational grazing, rest grazing and ban grazing. In the early days, pasture management was mainly aimed at improving pasture production. In the new context of global climate change and increased environmental pollution, balancing ecological protection and green economic development has become a necessary path to improve the overall ecological quality of regions [ 164 ]. The general system of rotational and rest grazing is only an artificial strategic rest and tactical grazing of grasslands [ 165 ]. Therefore, some studies have proposed models such as the sustainable grazing system (SGS), DairyMod, GRAZPLAN, GrassGro, DairyNZ whole-farm model and EverGraze [ 166 , 167 , 168 , 169 , 170 , 171 , 172 ]. The above systems encourage the sustainable development of grassland ecosystems, and are key to preventing soil erosion and promoting soil improvement, as well as being important measures to conserve grassland plant biodiversity. Michalk et al. detailed sustainable and permanent grazing systems and answer the key questions currently facing Australia: (1) whether increasing the number of paddocks and implementing rotational grazing results in higher grazing rates, higher yields per hectare and better economic benefits; (2) which combination of grazing methods and grazing rates is most appropriate to create higher and more stable perennial grasslands to improve yields and environmental benefits in different parts of the landscape; and (3) can landscape variability be identified, mapped and effectively managed on native grasslands in high-rainfall areas [ 173 ] ? These can provide some inspiration for grassland grazing and farmers’ livelihoods in KDC.
Therefore, optimizing the structural configuration of grasslands in terms of both biological and engineering measures is currently an unavoidable and important issue for improving the sustainable development of grasslands and economic development. It will not only solve the shortage of supply capacity for ecosystem services and promote regional economic development, but also issues related to the global supply of food.
2.2.3. Stability Studies
Stability refers primarily to the ability of a community or ecosystem to maintain its original structural and functional state and resist disturbance after a disturbance, known as resistance stability, and the ability of a community or ecosystem to return to its original state after a disturbance, known as resilience stability.
Exploring the causes that affect the stability of grassland ecosystems and suggesting targeted restoration strategies for degraded outcomes is a major focus of current research [ 83 ]. Community stability increases species diversity, species heterogeneity and population size, so maintaining the long-term stability of communities is crucial to maintaining ecosystem function. Researchers have explored the potential drivers and mechanisms of ecosystem stability by studying changes in the relationship between biodiversity and ecosystem service functions [ 174 , 175 , 176 ]. Biodiversity is a major driving factor of ecosystem stability [ 177 ]. Biodiversity consists mainly of above- and below-ground components. In terms of above-ground biodiversity, an increasing number of studies have shown that the more above-ground biodiversity (plant diversity) there is, the more stable the ecosystem is [ 178 ]. The results of Garcia-Palacios et al. showed that the diversity of leaf traits may promote the stability of an ecosystem under low drought conditions, while the species richness may play a greater role in the stability of an ecosystem under high drought conditions [ 179 ]. Similarly, below-ground biodiversity (soil biodiversity) altered grassland-ecosystem resistance and resilience through direct and indirect effects on plant diversity, net ecosystem productivity and plant species interactions, and, thus, changed grassland ecosystem stability [ 180 ]. In addition, recent studies have shown that phenological variation can also reconcile plant diversity with the seasonal stability of ecosystems, and, thus, affect the stability of the whole ecosystem [ 181 ].
Evaluating grassland-ecosystem stability has also become an effective means of detecting changes in grassland landscapes or the effectiveness of grassland restoration [ 182 ]. When evaluating the stability of grassland ecosystems, timescale variation is a factor that must be considered. By comparing the changes (years, seasons, or months) in grassland landscape patterns in different periods combined with evaluation models and methods, researchers have selected indicators of ecosystem vitality, resistance, resilience and variability to evaluate ecosystem stability [ 32 ], revealing the processes of change in grassland ecosystems under extreme weather conditions and their driver factors, quantifying the correlation between grassland ecosystem stability and biodiversity and their spatial patterns, and providing important prerequisites for improving sustainable ecosystem services [ 183 , 184 ]. The traditional evaluation methods of ecosystem stability are mainly qualitative analysis methods, including empirical methods and expert consultation methods, which are, generally, using expert consultation methods to quantify measures. The other is mainly quantitative evaluation including the comprehensive-evaluation method, gray-information analysis, ecological models and other methods, among which the most common methods of comprehensive analysis are hierarchical analysis, the entropy weighting method, the weighted comprehensive average method, and the comprehensive index evaluation method, etc. New kinds of ecosystem stability evaluation are diverse, and an increasing number of methods are becoming more credible and scientific in their assessment of ecological stability. These methods for evaluating the stability of ecosystems can provide good theoretical references for the ecosystem evaluation of KDC, and they can provide insights into the establishment of stability evaluation index systems for karst grassland ecosystems.
2.2.4. The Relationship between Structure and Stability
A good system structure maintains its relative stability, and ecosystems with high stability generally have a more rational structure. Tilman et al. argued that diversity leads to higher community productivity, ecosystem stability and resistance to invasion [ 74 ]. Before the 1970s, ecologists believed that communities with higher diversity had more stable ecosystems [ 185 ]. Since then, ecologists have focused more on the relationship between species diversity and ecosystem stability [ 186 , 187 ].
Biodiversity affects ecosystem services by altering ecosystem function and stability [ 188 ]. Biodiversity–stability relationships showed that above-ground productivity and temporal stability increased significantly with increasing species richness, while biodiversity was largely influenced by ecosystem structure [ 85 , 189 ]. Therefore, understanding the relationship between grassland-ecosystem structure and stability and its influencing factors is essential for the sustainable development of grassland ecosystems [ 190 ]. However, how plant species diversity regulates the stability of ecosystems (such as biomass reproduction and nutrient cycling) has become one of the challenging questions in ecology [ 191 , 192 ]. Experiments have demonstrated that the higher the complexity of the ecological network of grasslands, the higher the ecological stability, by influencing plant physiological conditions, as well as species generation, diversity and variation [ 193 , 194 ]. Of course, grassland-ecosystem stability is also vulnerable to the influence of ecosystem components (ecosystem species diversity, composition), climate change and anthropogenic activities, mainly in the form of lowering the productivity of grassland, weakening biodiversity and declining service functions, which, in turn, can affect grassland-ecosystem services [ 195 ]. Therefore, studying the structure of grassland ecosystems and their material and energy flows and cycles among different components, and exploring the relationship between grassland-ecosystem structure, function, and stability is one of the research areas that should be focused on in the future [ 196 ]. Recent studies have shown that the positive relationship between biodiversity and stability is also influenced by spatial scale [ 197 ]. Therefore, the study of the relationship between structure and stability should also consider different spatial scales and timescales.
2.2.5. Factors Affecting Structure and Stability
The structure and stability of grassland ecosystems are influenced by multiple factors, such as the natural environment and human activities. Therefore, the heterogeneity of spatial environments inevitably leads to variability in the degree of stability. In the case of grassland ecosystems, their structure and stability are mainly influenced by climate change, nitrogen deposition or nutrient addition, grazing and grassland management, plant invasion and natural disasters ( Figure 4 ).

The structural components of grassland ecosystems and the factors that influence them combine to influence grassland ecosystem stability.
Global climate change is affecting ecosystem function and stability, indirectly altering species diversity, species composition, and functional plant traits [ 198 , 199 ], thus reducing the capacity of ecosystem services and reducing the various benefits that humans derive from them [ 200 ]. The impact of climate change on ecosystem function and biodiversity is highly dependent on grazing history and natural conditions [ 190 ]. By comparing changes in the spatial pattern of grasslands and simulating different climatic conditions on grassland ecosystem resistance, recovery times and rates, White et al. concluded that future climate change will have a significant impact on the resistance and recovery of disturbed ecosystems; however, the spatial pattern of impacts varies widely [ 201 ].
Key limiting elements can alter species interactions [ 202 ], change the spatial and temporal patterns of terrestrial plant communities [ 203 ], and influence ecosystem function [ 204 ]. Atmospheric nitrogen deposition and the application of nutrients (phosphorus, potassium, etc.) can affect species diversity in grassland communities [ 205 ]. The results of related studies show that the addition of nitrogen or phosphorus individually can increase the productivity of grasslands by 0–20%, while the addition of both nitrogen and phosphorus can increase the productivity of grasslands by 60% [ 206 , 207 ]. Nitrogen is an essential nutrient for plant growth, and an appropriate addition of nitrogen deposition will directly promote rapid plant growth and increase the net primary productivity of terrestrial ecosystems. However, excessive nitrogen deposition can also lead to soil acidification, altering the effectiveness of soil mineral nutrients and the structure and function of microbial communities, which, in turn, affects plant growth and changes plant diversity [ 208 , 209 , 210 ]. Plant diversity is closely related to ecosystem stability and productivity [ 211 ], and ultimately drives changes in ecosystem structure and function [ 212 ]. Therefore, paying attention to the differences in supply between different nutrient elements and the resulting differences in the resistance and resilience of plant diversity that result is extremely important for both productivity stability and the temporal stability of grasslands [ 213 ].
Plant invasion and vegetation succession are also among the factors that alter the species composition of grasslands and directly change the structure of grassland ecosystems [ 214 , 215 ]. Since the early 20th century, the invasion of woody plants into grasslands and their impact on the carrying capacity of grasslands has become a serious problem for savannas [ 216 ]. As reported in savanna ecosystems, the large-scale invasion of shrubs, trees, and other plants has led to significant changes in the functioning of global ecosystems, which will have profound impacts on the biodiversity, carbon storage capacity, and supply of these ecosystems [ 217 ]. Invasions of poisonous weeds has been considered one of the most important causes of economic losses by inhibiting forage production and killing livestock. However, a recent study concluded that toxic weeds can also have some potential positive ecological impacts on grasslands, such as promoting soil and water conservation, improving nutrient cycling and biodiversity, and protecting rangelands from excessive livestock damage [ 218 ]. Therefore, appropriate actions are needed by policy makers, managers and stakeholders to assess the ecological functions of invasive toxic weeds and to reconcile the long-term trade offs between livestock development and maintaining the ecological services provided by grasslands.
Animal husbandry plays an important role in eradicating hunger and improving malnutrition [ 219 ]. Protein and energy from livestock products are the main sources of human nutrition. Therefore, improving and sustaining livestock development is critical to advancing the United Nations SDGs, especially in addressing zero hunger and mitigating climate change [ 220 ]. Rational livestock management is a major initiative to promote healthy ecosystem development. Therefore, rangeland management, as a determinant of maintaining biodiversity, ecosystem services and landscape [ 221 ], is more important to focus on exploring its driving mechanisms on grassland species structure and ecosystem stability [ 222 ]. In addition, natural hazards are of great importance to our understanding of global biogenic burning and its emissions, carbon cycling, biodiversity, conservation and land management, especially as ecosystem succession and biodiversity changes associated with grassland fires are critical to the patterns and dynamics of ecosystem functions and services [ 223 ].
In summary, sustainable management of grasslands requires a deep understanding of the functional relationships among these factors due to the spatial heterogeneity of environmental conditions, production potential and flora composition [ 224 ]. It is more important to jointly explore the similarities and differences in the structural composition of grassland ecosystems in multiple factors and scales, extract the key factors affecting the service capacity of grassland ecosystems, and apply them to the restoration of the grassland vegetation of KDC.
3. Materials and Methods
Based on the research of Khan et al. and Chapman et al. [ 225 , 226 ], we performed a systematic literature search and review following the protocol from Preferred Reporting Items for Systematic Reviews and Meta-analyses (PRISMA) including quantitative statistics and qualitative content analysis. Systematic reviews have an advantage over traditional reviews and commentaries in that they cover studies by following an explicitly formulated procedure, which can help readers to understand the whole protocol followed for the literature review [ 227 ].
Literature Search and Selection
The first step was identifying records. We conducted a systematic search of peer-reviewed literature (articles, reviews) using the key words in WOS and CNKI databases ( Table 2 ). A total of 99 repeated references were excluded; 3821 references were selected for review. The search timeframe for both databases was the maximum timeframe of the databases, and the search deadline was 1 November 2022.
Literature search terms and results.
The second step was screening. We screened the titles and abstracts of each article to select articles that assessed, depicted, and quantified or mapped grassland ecosystem structure and stability which were eligible for full-text reading ( n = 278). The third step was reading the full text of each of the selected publications, and, finally, a total of 133 references were chosen as case studies. To summarize the information about main structural characteristics, stability, structure–stability relationship and influencing factors and suggest future directions of ecosystem service-capacity enhancement from case studies, we recorded the annual distribution of the literature, distribution of countries of publication, types of grassland structure, disturbance factors of grassland stability, and research methods ( Figure 5 ).

The flow diagram showing the steps of the methodology and selection process used for the systematic literature review on the left and the literature review process on the right.
4. Prospects
4.1. key scientific issues that need to be addressed.
Research on the structure and stability of grassland ecosystems has achieved great success, but there are still many scientific questions that need to be addressed. Based on the previous systematic analysis, this paper categorizes the key scientific problems to be solved in the structure and stability of grassland ecosystem into three aspects: structure optimization and stability improvement and their relationship.
4.1.1. Structure Optimization
To address the problems of ecological imbalance, food-chain disruption, and multiple functions in grassland ecosystems, we investigate the interactions between population dynamics and community properties through synergistic intra- and inter-species differences and spatial landscape-scale differences, determine the mechanisms and effects of biodiversity on the stability of ecosystem functions, and clarify the overall operation of ecosystems [ 27 ].
To address the problem of the relatively homogeneous grassland-ecosystem structure, this study suggests exploring the maintenance mechanisms of grassland-species allocation and stability; combining the heterogeneity of grassland functions and spatial patterns; performing a comprehensive assessment of grasslands in terms of local stand conditions, climatic habitats, plant functional traits, and other conditions; and targeting the selection of grass species with high adaptability and resistance to stress for mixed seeding [ 228 ].
To address the problem of the optimal configuration of grassland ecological structure, different grassland management measures can be combined to form an efficient and integrated management system by organically combining biological, engineering and management aspects to promote the sustainable development of grassland ecological animal husbandry. For example, scientific methods such as rotational grazing, rest grazing and limited-term enclosure, and the use of artificially planted forage instead of grazing. Sustainable grazing management in adaptive multiple paddocks (AMP) can be employed to incorporate forage and ruminants into regeneration-management planting systems to strengthen the productivity, stability and resilience of agroecosystems and, thus, improve ecosystem service capacity.
4.1.2. Stability Improvement
The issue of the low stability of grassland ecosystems, can be explored through the mechanisms regulating the stability of grassland ecosystems, identify and quantify the factors affecting the stability of grassland ecosystems, select grass species suitable for local conditions, increase the diversity of grassland species, improve the resilience of grassland ecosystems, enhance ecological stability, and further promote the process of grassland ecological restoration, so as to reduce the ecological vulnerability of grassland and enhance the resilience and stability of grassland ecosystems [ 229 ].
To deal with the problem that the ecological stability index system and evaluation model have not been unified, it is necessary to establish and standardize the selection criteria of ecological stability evaluation indexes based on the analysis and summary of ecological stability research results, to select methods according to the basic attributes of grassland ecosystems, especially the key variables of ecosystem processes and functions, and to consider the validity, sensitivity and operability of alternative indexes. The evaluation indexes are selected using the expert consultation method, the evaluation index weights are determined using the hierarchical analysis method, and the mathematical model is constructed to specify them and quantify the factor percentages [ 230 ], so as to build a scientific grassland-ecosystem stability evaluation index system and promote the sustainable development of the system [ 231 ].
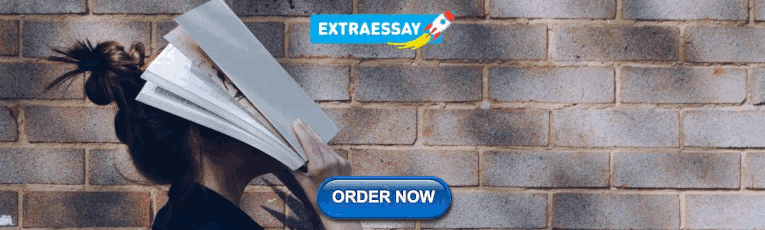
4.1.3. Interaction between Structure and Stability
To address the problem of unclear relationships between the structure and stability of grassland ecosystems, study is based on the idea of “structure-process-function-services”, to strengthen the material cycle, energy flow and information transfer process of producer-consumer-decomposer in grassland ecosystems, to clarify the relationship between grassland species allocation and productivity and stability, and to clarify the response mode of structure and stability. It has been suggested that structure can directly alter the biodiversity of grassland ecosystems and further alter the stability of grassland ecosystems [ 232 ]. Therefore, the relationship between structure and stability can be explored through the medium of biodiversity.
To address the problem of single research methods (e.g., field surveys, field experiments) or models (statistics, modeling) for the structure and stability of grassland ecosystems, remote-sensing research into the process of interannual or monthly changes in grassland landscape patterns can be employed, combined with indoor experiments, field surveys, and field trials for comparative assessment [ 233 , 234 ].
4.2. The Current Status of Grassland Ecosystem for KDC and Inspiration for Improving the Ecosystem Service Capacity of Grasslands for KDC
4.2.1. the current status of grassland ecosystem for kdc.
Karst areas are very fragile and prone to ecological degradation due to their unique binary three-dimensional geological structure, of which karst desertification is an extreme manifestation of ecological degradation [ 229 ]. In the past 30 years, the KDC in southern China has achieved remarkable results in ecological environment and ecological restoration, but there is an urgent need to improve the stability of the KDC ecosystem and ecosystem service function [ 52 , 235 ]. Grasslands of the KDC are dominated by artificial grasslands and supplemented by improved grasslands, which constitute an important ecosystem in karst areas [ 229 ]. The artificial grasses in the KDC areas mainly include monoculture Dactylis glomerata L., Lolium perenne L. and Medicago sativa L. Grasslands have a monoculture planting structure and weak disease resistance, and the resulting artificial grass ecosystem is extremely fragile. This is mainly due to farmers’ pursuit of high forage yields. Thus, while the artificial grassland has brought high economic income to farmers for a short period of time, its ability to provide ecosystem services is gradually diminishing. In addition, the degraded grassland is dominated by replanting white clover by government aircrafts. However, due to the overgrazing behavior of farmers in recent years, the replanted degraded grassland is difficult to recover, and only some grass species that are resistant to gnawing and trampling are propagated, resulting in the difficult recovery of the grass species structure of degraded grassland and low stability of the ecosystem.
In the process of KDC and ecological restoration, grass is the pioneer plant for vegetation restoration and ecological environment improvement [ 64 ]. Therefore, optimizing the structure and enhancing the stability of grassland ecosystems for KDC is of great significance for the sustainable development of regional ecological environments and promoting the virtuous cycle of regional eco-industry.
4.2.2. Inspiration for Improving the Ecosystem Service Capacity of Grasslands for the KDC
Due to its inherent sensitivity and fragility, the ecosystem structure and function of the grassland ecosystem in the KDC area are improper and the stability of the ecosystem is poor [ 236 , 237 ]. In the context of promoting the world’s desertification management and global sustainable greening, how to consolidate and stabilize the effectiveness of KDC and, at the same time, optimize the structure of desertification-control grassland ecosystems, guaranteeing the stability of the ecosystem and enhancing the ecosystem service capacity is a key issue that needs to be solved in the KDC area [ 238 ]. In view of this, based on the premise of sorting out the structure and stability of global grassland ecosystems, this paper drew on the above key scientific issues of structure optimization, stability enhancement and the interaction between structure and stability and, combined with the regional characteristics of karst desertification and the grassland status of KDC, proposes three insights for the enhancement of the ecosystem service capacity of grassland in the KDC area.
Adequate Understanding of Ecosystem Structure Optimization Is a Necessary Prerequisite for the Improvement of Ecosystem Services in Grasslands for the KDC
Although existing pasture cultivation has greatly increased forage production in the short term [ 239 ], it has made an outstanding contribution to KDC. However, the factors influencing the improvement of ecosystem services are not only determined by a single factor of production stability, and there is an urgent need to improve grassland ecosystem services through a variety of specific ways. Grassland ecosystem structure directly determines grassland ecosystem function and indirectly influences grassland ecosystem services. Research on multi-component mixes of legume grasses with different growth habits or morphological characteristics has concluded that the greater the number of components in the mix, the greater the likelihood of containing key species, and the greater the potential for community stability, thus achieving a seasonal balance in grassland output throughout the year and lasting stability in the number of years of use [ 240 ]. Similar experiments have been conducted in KDC areas, for example, the state replanted degraded grasslands with Trifolium repens L. and encouraged farmers to plant Trifolium repens L.+ Lolium perenne L. or Dactylis glomerata L. + Medicago sativa L. and other measures, which improved the ecosystem structure of grasslands of KDC to some extent. However, with the unique geographical environment of karst, seasonal drought and unreasonable human management, the ecological benefits of degraded and improved grasslands have gradually decreased [ 241 ]. Therefore, the following two points should be considered to enhance the service capacity of grassland ecosystems: (1) to optimize the planting structure of grassland ecosystems for rocky desertification management and enrich the spatial species composition of grassland, and to select stony, drought-tolerant and calcium-loving grasses for planting in response to the characteristics of karst desertification such as thin soil layers, easy soil erosion and calcareous lithology; and (2) strengthening research on the benefits of the structure–process–function–services cascade, so as to clarify the process of maintaining ecosystem productivity and stability [ 95 ].
Enhancing Grassland Ecosystem Stability Is an Important Guarantee for Enhancing Grassland Ecosystem Services in KDC
The more complex the structure of grassland ecosystem, the higher the degree of its ecological stability, which further affects the multifunctional functioning of grassland ecosystem and eventually changes the ecosystem service capacity. The existing grassland ecosystem structure of rocky desertification control is single and the grassland planting is mainly based on single high-yielding forage grasses, for example, Pennisetum sinese Roxb L. and Lolium perenne L., which have weak stability, low disease resistance and short yield supply time, which seriously hinders the sustainable development of local ecosystems and lacks effective measures to consolidate the achievement of rocky desertification control. Grassland ecosystem stability is an important factor that limits the sustainable development of grassland ecosystem productivity. Therefore, we should combine the needs of local farmers, and select suitable grass species for planting; conduct experiments on grass planting under different rocky desertification habitats; explore the best planting density of grass species and legume-grass planting ratio; and then improve the plant diversity of grassland ecosystems and enhance their stability and resilience. At the same time, we should combine various monitoring means (such as field sampling, drone aerial photography and remote sensing, etc.), and strengthen the research on the regulation mechanism of grassland ecosystem stability with the guidance of enhancing the sustainability of KDC, so as to improve the service capacity of the grassland ecosystems of KDC, further enhance its ecological recovery quality and maintain the sustainable development of the karst ecological environment.
Identifying the Interactions between Grassland Ecosystem Structure and Stability Is an Important Part of Enhancing Grassland Ecosystem Services
This paper showed that most of the literature focuses on multiple factors of the structure and stability of grassland ecosystems, but these studies have mainly focused on unidirectional effects, such as Gschwend et al. and Hu et al. showing that biodiversity can stabilize productivity through different mechanisms, such as the asynchronous responses of species to environmental change and stability [ 242 , 243 ]. What is more, exploring the mutual response mechanisms between grassland planting structures and their productive and ecological stability is an important element in clarifying the effects of current grass seed mixes in KDC and local sustainable development. Xu et al. explored the water–fertilizer coupling mechanism between soil and forage through intercropping in the southern karst region. The experiments proved that a reasonable intercropping system could coordinate the relationship between crops and environment and improve soil nutrients, which made a certain contribution to exploring soil–plant relationships in the grasslands of karst areas and improving the structure and stability of stone desertification grassland ecosystems [ 244 ]. However, from the perspective of the interaction mechanism of grassland ecosystem structure and stability, exploration into the changes in the supply of ecosystem service capacity of grassland managed by karst rock desertification is still lacking. Therefore, through field control experiments in the context of KDC, the interaction mechanisms of grassland-ecosystem structure and stability should be clarified in the context of KDC, and the exploration of above-ground-subsurface material flow and nutrient circulation fluency should provide important insights to elucidate the trade offs and synergistic relationships among service flows ( Figure 6 ).

Processes of energy and service flows in grassland ecosystems (structure affects stability and thus changes the level of function and ultimately service provision).
5. Conclusions
Based on the WOS and CNKI databases, this study systematically reviewed the research progress of grassland-ecosystem structure and stability, and concluded the following: (1) There is a significant increase in the number of annual publications on the structure and stability of grassland ecosystems, and the research directions and themes are becoming increasingly diverse. The research mainly focuses on revealing the mechanisms influencing species diversity and stability, quantitatively studying the nutrient and productivity of forage grasses, and the regulatory mechanisms of stability. (2) The spatial pattern of the study countries is highly consistent with the spatial distribution characteristics of grassland ecosystems, mainly dominated by countries with wider grassland areas such as China and the United States. However, the number of national publications in Africa contradicts the distribution pattern of grasslands. (3) The research on the structure and stability of grassland ecosystems focuses on the structural characteristics of grassland ecosystems, structural optimization, ecosystem stability, and the structure–stability relationship and its influencing factors, in which the grassland-ecosystem structure and structure and stability influencing factors are the main research directions (4) The key scientific issues that need to be addressed nowadays were grouped according to whether they addressed structure optimization, stability enhancement, and structure–stability relationship. We should strengthen the multi-perspective exploration of grasslands under different spatial and temporal conditions, to enhance the functional output of grassland ecosystems from optimizing the structure of grassland ecosystems, to enhance the stability of grassland ecosystems, and to form a structure–process–function–services cascade benefit study. Based on the above conclusions, three insights can be provided for the enhancement of grassland ecosystem services in the KDC: (i) fully understanding ecosystem structure optimization is a necessary prerequisite for karst grassland stability; (ii) enhancing grassland ecosystem stability is an important guarantee for enhancing grassland ecosystem services in KDC; and (iii) clarifying the interaction between grassland ecosystem structure and stability is an important link to enhance grassland ecosystem services.
Acknowledgments
We would like to thank all the editors for their contributions to this paper and the anonymous reviewers for their thoughtful comments, which enriched the paper.
Funding Statement
This research was funded by the Key Project of Science and Technology Program of Guizhou Province (No. 5411 2017 Qiankehe Pingtai Rencai), the China Overseas Expertise Introduction Program for Discipline Innovation (No. D17016) and Natural Science Research Project of Education Department of Guizhou Province [Qianjiaohe KY Zi (2022) 157].
Author Contributions
Conceptualization, S.H.; methodology, S.H.; software, S.H.; validation, S.H.; formal analysis, S.H.; investigation, S.H.; resources, S.H.; data curation, S.H.; writing—original draft preparation, S.H.; writing—review and editing, S.H., K.X., Y.C., S.S., J.F. and C.H.; visualization, S.H.; supervision, K.X.; project administration, K.X. and Y.C.; funding acquisition, K.X. and Y.C. All authors have read and agreed to the published version of the manuscript.
Data Availability Statement
Conflicts of interest.
The authors declare no conflict of interest.
Disclaimer/Publisher’s Note: The statements, opinions and data contained in all publications are solely those of the individual author(s) and contributor(s) and not of MDPI and/or the editor(s). MDPI and/or the editor(s) disclaim responsibility for any injury to people or property resulting from any ideas, methods, instructions or products referred to in the content.
Advertisement
Grassland ecosystem services: a systematic review of research advances and future directions
- Research Article
- Published: 15 February 2020
- Volume 35 , pages 793–814, ( 2020 )
Cite this article
- Yuanyuan Zhao 1 , 2 ,
- Zhifeng Liu ORCID: orcid.org/0000-0002-4087-0743 3 , 4 &
- Jianguo Wu 3 , 5
9777 Accesses
166 Citations
12 Altmetric
Explore all metrics
Grasslands provide a variety of ecosystem services (ESs) for humans. While much ES research has focused on forests and wetlands, synthesizing the currently somewhat sporadic studies of grassland ecosystem services (GESs) is much needed.
We aimed to review the scope, major methods, and key findings of GESs, and identify knowledge gaps and future directions.
We conducted a systematic review of articles published during 1970–2018 (including 380 peer-reviewed articles from Web of Science and 32 book chapters from Google Scholar).
The number of GES studies has accelerated in recent decades, with China (31%) and the United States (18%) together accounting for almost half of them. A total of 33 GESs were mentioned in the searched articles, of which carbon sequestration, forage production, and water erosion control had the highest frequencies. Methods for evaluating GESs include field survey, field experiments, and statistical and process-based modeling. Grasslands are the primary source of meat and dairy products, account for about one-third of the total carbon of all terrestrial ecosystems, and provide numerous other ESs, such as night cooling, soil erosion control, and flood mitigation.
Conclusions
This review presents the state-of-the-science of GESs, and identifies several future research directions. To move forward, we propose a framework with a 3-M methodology: (1) “Multi-scales”—understanding GESs from various spatiotemporal scales; (2) “Multi-methods”—evaluating GESs with multiple statistical and modeling techniques using multiple data sources; and (3) “Multi-perspectives”—assessing GESs from ecological, social, and economic perspectives for sustainability.
This is a preview of subscription content, log in via an institution to check access.
Access this article
Price includes VAT (Russian Federation)
Instant access to the full article PDF.
Rent this article via DeepDyve
Institutional subscriptions
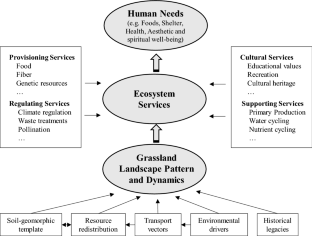
(Modified from Campbell et al. 1996 , White et al. 2000 and Havstad et al. 2007 )
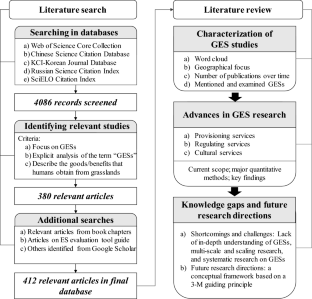
Similar content being viewed by others
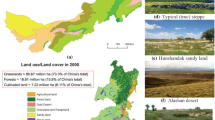
Ecology and sustainability of the Inner Mongolian Grassland: Looking back and moving forward
Development and Future of Grassland Ecosystems: Do We Need a Paradigm Shift?
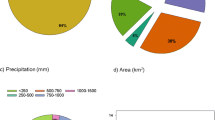
Sustainable grazing land management to protect ecosystem services
Abulizi A, Yang Y, Mamat Z, Luo J, Abdulslam D, Xu Z, Zayiti A, Ahat A, Halik W (2017) Land-use change and its effects in Charchan Oasis, Xinjiang, China. Land Degrad Dev 28(1):106–115
Google Scholar
Affek AN (2018) Indicators of ecosystem potential for pollination and honey production. Ecol Indic 94:33–45
Akhtar N, Rashid A, Murad W, Bergmeier E (2013) Diversity and use of ethno-medicinal plants in the region of Swat. North Pak J Ethnobiol Ethnomed 9:25
Allan E, Manning P, Alt F, Binkenstein J, Blaser S, Bluethgen N, Boehm S, Grassein F, Hoelzel N, Klaus VH, Kleinebecker T, Morris EK, Oelmann Y, Prati D, Renner SC, Rillig MC, Schaefer M, Schloter M, Schmitt B, Schoening I, Schrumpf M, Solly E, Sorkau E, Steckel J, Steffen-Dewenter I, Stempfhuber B, Tschapka M, Weiner CN, Weisser WW, Werner M, Westphal C, Wilcke W, Fischer M (2015) Land use intensification alters ecosystem multifunctionality via loss of biodiversity and changes to functional composition. Ecol Lett 18(8):834–843
PubMed PubMed Central Google Scholar
Anadon JD, Sala OE, Turner BL II, Bennett EM (2014) Effect of woody-plant encroachment on livestock production in North and South America. Proc Natl Acad Sci USA 111(35):12948–12953
CAS PubMed Google Scholar
Angelstam P, Munoz-Rojas J, Pinto-Correia T (2019) Landscape concepts and approaches foster learning about ecosystem services. Landsc Ecol 34(7):1445–1460
Archer SR, Predick KI (2014) An ecosystem services perspective on brush management: research priorities for competing land-use objectives. J Ecol 102:1394–1407
Atjay GL, Ketner P, Duvigneaud P (1979) Terrestrial primary production and phytomass. In: Bolin B, Degens ET, Kempe S, Ketner P (eds) The global carbon cycle. Wiley, Chichester, pp 129–181
Auffret AG, Cousins SAO (2018) Land uplift creates important meadow habitat and a potential original niche for grassland species. Proc R Soc B 285:20172349
PubMed Google Scholar
Bagstad K, Villa F, Johnson G, Voigt B (2011) ARIES–ARtificial Intelligence for Ecosystem Services: a guide to models and data, version 1.0. ARIES report series n.1
Bai Y, Wang Y (2017) Long-term ecological research and demonstrations support protection and sustainable management of grassland ecosystems. Bull Chin Acad Sci 32(8):910–916 (in Chinese with English abstract)
Barnes RF, Nelson CJ (2003) Forages and grasslands in a changing world. In: Barnes RF, Nelson CJ, Collins M, Moore KJ (eds) Forages: an introduction to grassland agriculture. Iowa State University Press, Ames
Bastian O, Grunewald K, Syrbe R-U, Walz U, Wende W (2014) Landscape services: the concept and its practical relevance. Landsc Ecol 29(9):1463–1479
Bennett AB, Isaacs R (2014) Landscape composition influences pollinators and pollination services in perennial biofuel plantings. Agric Ecosyst Environ 193:1–8
Brown JR, MacLeod ND (2017) An ecosystem services filter for rangeland restoration. Rangeland J 39(5–6):451–459
Byrd KB, Flint LE, Alvarez P, Casey CF, Sleeter BM, Soulard CE, Flint AL, Sohl TL (2015) Integrated climate and land use change scenarios for California rangeland ecosystem services: wildlife habitat, soil carbon, and water supply. Landsc Ecol 30(4):729–750
Campbell B, Frost P, Byron N (1996) Miombo woodlands and their use: overview and key issues. In: Campbell B (ed) The miombo in transition: woodlands and welfare in Africa. Center for International Forestry Research (CIFOR), Bogor, pp 1–10
Cao Q, Yu D, Georgescu M, Han Z, Wu J (2015) Impacts of land use and land cover change on regional climate: a case study in the agro-pastoral transitional zone of China. Environ Res Lett 10(12):124025
Cardinale BJ, Duffy JE, Gonzalez A, Hooper DU, Perrings C, Venail P, Narwani A, Mace GM, Tilman D, Wardle DA, Kinzig AP, Daily GC, Loreau M, Grace JB, Larigauderie A, Srivastava DS, Naeem S (2012) Biodiversity loss and its impact on humanity. Nature 486:59
Cardinale BJ, Gross K, Fritschie K, Flombaum P, Fox JW, Rixen C, van Ruijven J, Reich PB, Scherer-Lorenzen M, Wilsey BJ (2013) Biodiversity simultaneously enhances the production and stability of community biomass, but the effects are independent. Ecology 94(8):1697–1707
Cardinale BJ, Wright JP, Cadotte MW, Carroll IT, Hector A, Srivastava DS, Loreau M, Weis JJ (2007) Impacts of plant diversity on biomass production increase through time because of species complementarity. Proc Natl Acad Sci USA 104(46):18123–18128
Ceotto E (2008) Grasslands for bioenergy production. A review. Agron Sustain Dev 28:47–55
Chapman S, Watson JEM, Salazar A, Thatcher M, McAlpine CA (2017) The impact of urbanization and climate change on urban temperatures: a systematic review. Landsc Ecol 32(10):1921–1935
Chen L, Gao J, Ji Y, Bai Z, Shi M, Liu H (2014) Effects of particulate matter of various sizes derived from suburban farmland, woodland and grassland on air quality of the central district in Tianjin, China. Aerosol Air Qual Res 14(3):829–839
Chi W, Zhao Y, Kuang W, He H (2019) Impacts of anthropogenic land use/cover changes on soil wind erosion in China. Sci Total Environ 668:204–215
Chytry M, Jarosik V, Pysek P, Hajek O, Knollova I, Tichy L, Danihelka J (2008) Separating habitat invisibility by alien plants from the actual level of invasion. Ecology 89(6):1541–1553
Conant RT (2010) Challenges and opportunities for carbon sequestration in grassland systems: a technical report on grassland management and climate change mitigation. Food and Agriculture Organization of the United Nations, Rome
Conkling TJ, Belant JL, DeVault TL, Martin JA (2017) Effects of crop type and harvest on nest survival and productivity of dickcissels in semi-natural grasslands. Agric Ecosyst Environ 240:224–232
Costanza R, d’Arge R, de Groot R, Farber S, Grasso M, Hannon B, Limburg K, Naeem S, O’Neill RV, Paruelo J, Raskin RG, Sutton P, van den Belt M (1997) The value of the world’s ecosystem services and natural capital. Nature 387(6630):253–260
CAS Google Scholar
Costanza R, de Groot R, Sutton P, van der Ploeg S, Anderson SJ, Kubiszewski I, Farber S, Turner RK (2014) Changes in the global value of ecosystem services. Glob Environ Change 26:152–158
Cui F, Tang H, Zhang Q, Wang B, Dai L (2019) Integrating ecosystem services supply and demand into optimized management at different scales: a case study in Hulunbuir,China. Ecosyst Serv 39:100984
Daily G (1997) Nature’s services: societal dependence on natural ecosystems. Island Press, Washington, DC
de Groot RS, Wilson MA, Boumans RMJ (2002) A typology for the classification, description and valuation of ecosystem functions, goods and services. Ecol Econ 41:393–408
Deng L, Shangguan Z, Sweeney S (2014) “Grain for Green” driven land use change and carbon sequestration on the Loess Plateau, China. Sci Rep 4:7039
CAS PubMed PubMed Central Google Scholar
Dirks L, Dirks G, Wu J (2012) Evolving perspectives on biofuels in the United States. Front Energy 6(4):379–393
Dixon AP, Faber-Langendoen D, Josse C, Morrison J, Loucks CJ (2014) Distribution mapping of world grassland types. J Biogeogr 41(11):2003–2019
Duarte GT, Santos PM, Cornelissen TG, Ribeiro MC, Paglia AP (2018) The effects of landscape patterns on ecosystem services: meta-analyses of landscape services. Landsc Ecol 33(8):1247–1257
Egoh B, Reyers B, Rouget M, Bode M, Richardson DM (2009) Spatial congruence between biodiversity and ecosystem services in South Africa. Biol Conserv 142:553–562
Egoh B, Reyers B, Rouget M, Richardson DM, Le Maitre DC, van Jaarsveld AS (2008) Mapping ecosystem services for planning and management. Agric Ecosyst Environ 127:135–140
Egoh BN, Reyers B, Rouget M, Richardson DM (2011) Identifying priority areas for ecosystem service management in South African grasslands. J Environ Manag 92(6):1642–1650
Enri SR, Probo M, Farruggia A, Lanore L, Blanchetete A, Dumont B (2017) A biodiversity-friendly rotational grazing system enhancing flower-visiting insect assemblages while maintaining animal and grassland productivity. Agric Ecosyst Environ 241:1–10
Eze S, Palmer SM, Chapman PJ (2018) Soil organic carbon stock in grasslands: effects of inorganic fertilizers, liming and grazing in different climate settings. J Environ Manag 223:74–84
Farley KA, Bremer LL, Harden CP, Hartsig J (2013) Changes in carbon storage under alternative land uses in biodiverse Andean grasslands: implications for payment for ecosystem services. Conserv Lett 6(1):21–27
Farley KA, Kelly EF, Hofstede RGM (2004) Soil organic carbon and water retention following conversion of grasslands to pine plantations in the Ecuadoran Andes. Ecosystems 7(7):729–739
Favretto N, Stringer LC, Dougill AJ, Dallimer M, Perkins JS, Reed MS, Atlhopheng JR, Mulale K (2016) Multi-criteria decision analysis to identify dryland ecosystem service trade-offs under different rangeland land uses. Ecosyst Serv 17:142–151
Feng X, Fu B, Lu N, Zeng Y, Wu B (2013) How ecological restoration alters ecosystem services: an analysis of carbon sequestration in China’s Loess Plateau. Sci Rep 3:2846
Ferner J, Schmidtlein S, Guuroh RT, Lopatin J, Linstaedter AA (2018) Disentangling effects of climate and land-use change on West African drylands’ forage supply. Glob Environ Change Hum Policy Dimens 53:24–38
Fisher JA, Patenaude G, Meir P, Nightingale AJ, Rounsevell MDA, Williams M, Woodhouse IH (2013) Strengthening conceptual foundations: analysing frameworks for ecosystem services and poverty alleviation research. Glob Environ Change Hum Policy Dimens 23(5):1098–1111
Fu B, Liu Y, Lu Y, He C, Zeng Y, Wu B (2011) Assessing the soil erosion control service of ecosystems change in the Loess Plateau of China. Ecol Complex 8(4):284–293
Fu B, Wang Y, Xu P, Yan K (2013) Mapping the flood mitigation services of ecosystems—a case study in the Upper Yangtze River Basin. Ecol Eng 52:238–246
Garrido P, Elbakidze M, Angelstam P (2017) Stakeholders’ perceptions on ecosystem services in Ostergotland’s (Sweden) threatened oak wood-pasture landscapes. Landsc Urban Plan 158:96–104
Gong L, Shao Q, Zhai J (2014) Sand-fixing function under the change of vegetation coverage in a wind erosion area in northern China. J Resour Ecol 5(2):105–114
Gray EF, Bond WJ (2013) Will woody plant encroachment impact the visitor experience and economy of conservation areas? Koedoe. https://doi.org/10.4102/koedoe.v55i1.1106
Article Google Scholar
Han G, Hao X, Zhao M, Wang M, Ellert BH, Willms W, Wang M (2008) Effect of grazing intensity on carbon and nitrogen in soil and vegetation in a meadow steppe in Inner Mongolia. Agric Ecosyst Environ 125:21–32
Hao R, Yu D, Liu Y, Liu Y, Qiao J, Wang X, Du J (2017a) Impacts of changes in climate and landscape pattern on ecosystem services. Sci Total Environ 579:718–728
Hao R, Yu D, Wu J (2017b) Relationship between paired ecosystem services in the grassland and agro-pastoral transitional zone of China using the constraint line method. Agric Ecosyst Environ 240:171–181
Havstad KM, Peters DPC, Skaggs R, Brown J, Bestelmeyer B, Fredrickson E, Herrick J, Wright J (2007) Ecological services to and from rangelands of the United States. Ecol Econ 64(2):261–268
Hegland SJ, Boeke L (2006) Relationships between the density and diversity of floral resources and flower visitor activity in a temperate grassland community. Ecol Entomol 31(5):532–538
Hein L, van Koppen K, de Groot RS, van Ierland EC (2006) Spatial scales, stakeholders and the valuation of ecosystem services. Ecol Econ 57:209–228
Helm A, Oja T, Saar L, Takkis K, Talve T, Partel M (2009) Human influence lowers plant genetic diversity in communities with extinction debt. J Ecol 97:1329–1336
Holland JM, Douma JC, Crowley L, James L, Kor L, Stevenson DRW, Smith BM (2017) Semi-natural habitats support biological control, pollination and soil conservation in Europe. A review. Agron Sustain Dev 37(4):31
Honigova I, Vackar D, Lorencova E, Melichar J, Gotzl M, Sonderegger G, Ouskova V, Hosek M, Chobot K (2012) Survey on grassland ecosystem services. Report to the EEA—European Topic Centre on Biological Diversity. Nature Conservation Agency of the Czech Republic, Prague, p 78
Horrocks CA, Dungait JAJ, Heal KV, Cardenas LM (2015) Comparing N 2 O fluxes from recently created extensive grasslands and sites remaining under intensive agricultural management. Agric Ecosyst Environ 199:77–84
Huntsinger L, Oviedo JL (2014) Ecosystem services are social-ecological services in a traditional pastoral system: the case of California’s mediterranean rangelands. Ecol Soc 19(1):8
Isbell F, Adler PR, Eisenhauer N, Fornara D, Kimmel K, Kremen C, Letourneau DK, Liebman M, Polley HW, Quijas S, Scherer-Lorenzen M (2017) Benefits of increasing plant diversity in sustainable agroecosystems. J Ecol 105(4):871–879
Jenkins WA, Murray BC, Kramer RA, Faulkner SP (2010) Valuing ecosystem services from wetlands restoration in the Mississippi Alluvial Valley. Ecol Econ 69:1051–1061
Jiang C, Zhang H, Zhang Z (2018) Spatially explicit assessment of ecosystem services in China’s Loess Plateau: patterns, interactions, drivers, and implications. Glob Planet Change 161:41–52
Jiang C, Zhang H, Zhang Z, Wang D (2019) Model-based assessment soil loss by wind and water erosion in China’s Loess Plateau: dynamic change, conservation effectiveness, and strategies for sustainable restoration. Glob Planet Change 172:396–413
Kemp DR, Han G, Hou X, Michalk DL, Hou F, Wu J, Zhang Y (2013) Innovative grassland management systems for environmental and livelihood benefits. Proc Natl Acad Sci USA 110(21):8369–8374
Khan KS, Kunz R, Kleijnen J, Antes G (2003) Five steps to conducting a systematic review. J R Soc Med 96(3):118–121
Lal R (2011) Sequestering carbon in soils of agro-ecosystems. Food Policy 36:S33–S39
Lamarque P, Meyfroidt P, Nettier B, Lavorel S (2014) How ecosystem services knowledge and values influence farmers’ decision-making. PLoS ONE 9(9):e107572
Lamarque P, Tappeiner U, Turner C, Steinbacher M, Bardgett RD, Szukics U, Schermer M, Lavorel S (2011) Stakeholder perceptions of grassland ecosystem services in relation to knowledge on soil fertility and biodiversity. Reg Environ Change 11(4):791–804
Laura VV, Bert R, Steven B, Dirk R, Kris V (2018) Assessing the impact of grassland management extensification in temperate areas on multiple ecosystem services and biodiversity. Agric Ecosyst Environ 267:201–212
Lavorel S, Grigulis K (2012) How fundamental plant functional trait relationships scale-up to trade-offs and synergies in ecosystem services. J Ecol 100(1):128–140
Lavorel S, Grigulis K, Leitinger G, Kohler M, Schirpke U, Tappeiner U (2017) Historical trajectories in land use pattern and grassland ecosystem services in two European alpine landscapes. Reg Environ Change 17(8):2251–2264
Li X, Wang Z, Wang D, Wang L, Pan D, Li J, De K, Seastedt TR (2019) Livestock grazing impacts on plateau pika (Ochotona curzoniae) vary by species identity. Agric Ecosyst Environ 275:23–31
Lian Z, Xu W, Yang W, Blank D, Huang Y (2014) Effects of livestock grazing on soil seed bank: a review. Pratacult Sci 31(12):2301–2307
Lindgren J, Lindborg R, Cousins SAO (2018) Local conditions in small habitats and surrounding landscape are important for pollination services, biological pest control and seed predation. Agric Ecosyst Environ 251:107–113
Liu J, Wu J, Liu F, Han X (2012) Quantitative assessment of bioenergy from crop stalk resources in Inner Mongolia, China. Appl Energy 93:305–318
Lu D, Mao W, Yang D, Zhao J, Xu J (2018) Effects of land use and landscape pattern on PM2.5 in Yangtze River Delta, China. Atmos Pollut Res 9(4):705–713
Machovina B, Feeley KJ (2017) Restoring low-input high-diversity grasslands as a potential global resource for biofuels. Sci Total Environ 609:205–214
Macleod CJA, Ferrier RC (2011) Temperate grasslands in catchment systems: the role of scale, connectivity and thresholds in the provision and regulation of water quality and quantity. In: Lemaire G, Hodgson J, Chabbi A (eds) Grassland productivity and ecosystem services. CABI International, Oxfordshire, pp 229–238
Manning P, de Vries FT, Tallowin JRB, Smith R, Mortimer SR, Pilgrim ES, Harrison KA, Wright DG, Quirk H, Benson J, Shipley B, Cornelissen JHC, Kattge J, Boenisch G, Wirth C, Bardgett RD (2015) Simple measures of climate, soil properties and plant traits predict national-scale grassland soil carbon stocks. J Appl Ecol 52(5):1188–1196
Martinez Pastur G, Peri PL, Lencinas MV, Garcia-Llorente M, Martin-Lopez B (2016) Spatial patterns of cultural ecosystem services provision in Southern Patagonia. Landsc Ecol 31(2):383–399
Martinez-Estevez L, Balvanera P, Pacheco J, Ceballos G (2013) Prairie dog decline reduces the supply of ecosystem services and leads to desertification of semiarid grasslands. PLoS ONE 8(10):e75229
MEA (2005) Ecosystems and human well-being: current state and trends. Island Press, Washington, DC
Modernel P, Rossing WAH, Corbeels M, Dogliotti S, Picasso V, Tittonell P (2016) Land use change and ecosystem service provision in Pampas and Campos grasslands of southern South America. Environ Res Lett 11(11):113002
Monteiro MV, Doick KJ, Handley P, Peace A (2016) The impact of greenspace size on the extent of local nocturnal air temperature cooling in London. Urban For Urban Green 16:160–169
Mu J, Zeng Y, Wu Q, Niklas KJ, Niu K (2016) Traditional grazing regimes promote biodiversity and increase nectar production in Tibetan alpine meadows. Agric Ecosyst Environ 233:336–342
Nearing M, Foster GR, Lane LJ, Finkner SC (1989) A process-based soil erosion model for USDA-water erosion prediction project technology. Trans ASAE 32(5):1587–1593
Nearing MA, Jetten V, Baffaut C, Cerdan O, Couturier A, Hernandez M, Le Bissonnais Y, Nichols MH, Nunes JP, Renschler CS, Souchère V, van Oost K (2005) Modeling response of soil erosion and runoff to changes in precipitation and cover. CATENA 61(2–3):131–154
Nearing M, Pierson F, Hernandez M, Al-Hamdan O, Weltz M, Spaeth K, Wei H, Stone J (2011) A rangeland hydrology and erosion model. Trans ASABE 54:901–908
Ni J (2002) Carbon storage in grasslands of China. J Arid Environ 50(2):205–218
OECD, FAO (2011) OECD-FAO Agricultural Outlook 2011–2020. OECD Publishing and FAO, Paris. https://doi.org/10.1787/agr_outlook-2011-en
Book Google Scholar
Olson JS, Watts JA, Allison LJ (1983) Carbon in live vegetation of major world ecosystems. Report ORNL-5862. Oak Ridge National Laboratory, Tennessee
O’Mara FP (2012) The role of grasslands in food security and climate change. Ann Bot 110(6):1263–1270
Onatibia GR, Aguiar MR, Semmartin M (2015) Are there any trade-offs between forage provision and the ecosystem service of C and N storage in arid rangelands? Ecol Eng 77:26–32
Orford KA, Murray PJ, Vaughan IP, Memmott J (2016) Modest enhancements to conventional grassland diversity improve the provision of pollination services. J Appl Ecol 53(3):906–915
Oteros-Rozas E, Martin-Lopez B, Fagerholm N, Bieling C, Plieninger T (2018) Using social media photos to explore the relation between cultural ecosystem services and landscape features across five European sites. Ecol Indic 94:74–86
Oteros-Rozas E, Martin-Lopez B, Lopez CA, Palomo I, Gonzalez JA (2013) Envisioning the future of transhumant pastoralism through participatory scenario planning: a case study in Spain. Rangel J 35(3):251–272
Pan Y, Wu J, Xu Z (2014) Analysis of the tradeoffs between provisioning and regulating services from the perspective of varied share of net primary production in an alpine grassland ecosystem. Ecol Complex 17:79–86
Papanastasis VP, Bautista S, Chouvardas D, Mantzanas K, Papadimitriou M, Mayor AG, Koukioumi P, Papaioannou A, Vallejo RV (2017) Comparative assessment of goods and services provided by grazing regulation and reforestation in degraded mediterranean rangelands. Land Degrad Dev 28(4):1178–1187
Parton W, Scurlock J, Ojima D, Schimel D, Hall D (1995) Impact of climate change on grassland production and soil carbon worldwide. Glob Change Biol 1(1):13–22
Petz K, Alkemade R, Bakkenes M, Schulp CJE, van der Velde M, Leemans R (2014) Mapping and modelling trade-offs and synergies between grazing intensity and ecosystem services in rangelands using global-scale datasets and models. Glob Environ Change Human Policy Dimens 29:223–234
Piao S, Fang J, Zhou L, Tan K, Tao S (2007) Changes in biomass carbon stocks in China’s grasslands between 1982 and 1999. Glob Biogeochem Cycles. https://doi.org/10.1029/2005gb002634
Pogue SJ, Krobel R, Janzen HH, Beauchemin KA, Legesse G, de Souza DM, Iravani M, Selin C, Byrne J, McAllister TA (2018) Beef production and ecosystem services in Canada’s prairie provinces: a review. Agric Syst 166:152–172
Prochnow A, Heiermann M, Plochl M, Linke B, Idler C, Amon T, Hobbs PJ (2009) Bioenergy from permanent grassland—a review: 1. Biogas Bioresour Technol 100:4931–4944
Qiu J, Carpenter SR, Booth EG, Motew M, Zipper SC, Kucharik CJ, Loheide SP II, Turner AG (2018) Understanding relationships among ecosystem services across spatial scales and over time. Environ Res Lett 13(5):054020
Reed MS, Stringer LC, Dougill AJ, Perkins JS, Atlhopheng JR, Mulale K, Favretto N (2015) Reorienting land degradation towards sustainable land management: linking sustainable livelihoods with ecosystem services in rangeland systems. J Environ Manag 151:472–485
Ren Y, Lu Y, Fu B (2016) Quantifying the impacts of grassland restoration on biodiversity and ecosystem services in China: a meta-analysis. Ecol Eng 95:542–550
Renard KG, Foster GR, Weesies GA, Porter JP (1991) RUSLE: revised universal soil loss equation. J Soil Water Conserv 46(1):30–33
Renard K, Yoder D, Lightle D, Dabney S (2011) Universal soil loss equation and revised universal soil loss equation. Handbook of erosion modelling. Blackwell Publ, Oxford, pp 137–167
Robledo-Abad C, Althaus HJ, Berndes G, Bolwig S, Corbera E, Creutzig F, Garcia-Ulloa J, Geddes A, Gregg JS, Haberl H, Hanger S, Harper RJ, Hunsberger C, Larsen RK, Lauk C, Leitner S, Lilliestam J, Lotze-Campen H, Muys B, Nordborg M, Olund M, Orlowsky B, Popp A, Portugal-Pereira J, Reinhard J, Scheiffle L, Smith P (2017) Bioenergy production and sustainable development: science base for policymaking remains limited. GCB Bioenergy 9(3):541–556
Rodriguez JP, Beard TD, Bennett EM, Cumming GS, Cork SJ, Agard J, Dobson AP, Peterson GD (2006) Trade-offs across space, time, and ecosystem services. Ecol Soc 11(1):28
Saha D, Kukal SS (2015) Soil structural stability and water retention characteristics under different land uses of degranded lower himalayas of North-west India. Land Degrad Dev 26(3):263–271
Sala OE, Paruelo JM (1997) Ecosystem services in grasslands. In: Daily GC (ed) Nature’s services: societal dependence on natural ecosystems. Island Press, Washington, DC, pp 237–251
Sala OE, Yahdjian L, Havstad K, Aguiar MR (2017) Rangeland ecosystem services: nature’s supply and humans’ demand. Rangeland Systems. Springer Series on Environmental Management. Springer, Berlin, pp 467–489
Sanaei A, Ali A, Chahouki MAZ (2018) The positive relationships between plant coverage, species richness, and aboveground biomass are ubiquitous across plant growth forms in semi-steppe rangelands. J Environ Manag 205:308–318
Sannigrahi S, Bhatt S, Rahmat S, Paul SK, Sen S (2018) Estimating global ecosystem service values and its response to land surface dynamics during 1995-2015. J Environ Manag 223:115–131
Schaich H, Bieling C, Plieninger T (2010) Linking ecosystem services with cultural landscape research. GAIA 19(4):269–277
Sherrouse BC, Semmens DJ (2015) Social values for ecosystem services, version 3.0 (SolVES 3.0): documentation and user manual. U.S. Geological Survey Open-File Report 2015-1008. http://dx.doi.org/10.3133/ofr20151008
Silvertown J (2015) Have ecosystem services been oversold? Trends Ecol Evol 30:641–648
Silvestri S, Zaibet L, Said MY, Kifugo SC (2013) Valuing ecosystem services for conservation and development purposes: a case study from Kenya. Environ Sci Policy 31:23–33
Sirimarco X, Paula Barral M, Horacio Villarino S, Laterra P (2017) Water regulation by grasslands: a global meta-analysis. Ecohydrology. https://doi.org/10.1002/eco.1934
Smit HJ, Metzger MJ, Ewert F (2008) Spatial distribution of grassland productivity and land use in Europe. Agric Syst 98:208–219
Stahlheber KA, Watson B, Dickson TL, Disney R, Gross KL (2016) Balancing biofuel production and biodiversity: harvesting frequency effects on production and community composition in planted tallgrass prairie. Biomass Bioenergy 92:98–105
Sun R, Chen L (2017) Effects of green space dynamics on urban heat islands: mitigation and diversification. Ecosyst Serv 23:38–46
Sutton PC, Costanza R (2002) Global estimates of market and non-market values derived from nighttime satellite imagery, land cover, and ecosystem service valuation. Ecol Econ 41:509–527
Tallis H, Ricketts T, Guerry A, Wood S, Sharp R, Nelson E, Ennaanay D, Wolny S, Olwero N, Vigerstol K, Pennington D, Mendoza G, Aukema J, Forster J, Forrest J, Cameron D, Arkema K, Lonsdorf E, Kennedy C, Verutes G, Kim C, Guannel G, Papenfus M, Toft J, Marsik M, Bernhardt J, Griffin R, Glowinski K, Chaumont N, Perelman A, Lacayo M (2013) InVEST 2.5. 6 User’s Guide. The Natural Capital Project, Stanford
Tao S, Fang J, Zhao X, Zhao S, Shen H, Hu H, Tang Z, Wang Z, Guo Q (2015) Rapid loss of lakes on the Mongolian Plateau. Natl Acad Sci USA 112(7):2281–2286
Tilman D, Hill J, Lehman C (2006) Carbon-negative biofuels from low-input high-diversity grassland biomass. Science 314:1598–1600
Trolliet F, Serckx A, Forget P-M, Beudels-Jamar RC, Huynen M-C, Hambuckers A (2016) Ecosystem services provided by a large endangered primate in a forest-savanna mosaic landscape. Biol Conserv 203:55–66
van Eekeren N, de Boer H, Hanegraaf M, Bokhorst J, Nierop D, Bloem J, Schouten T, de Goede R, Brussaard L (2010) Ecosystem services in grassland associated with biotic and abiotic soil parameters. Soil Biol Biochem 42(9):1491–1504
Vukomanovic J, Steelman T (2019) A systematic review of relationships between mountain wildfire and ecosystem services. Landsc Ecol 34:1179–1194
Wang L, Delgado-Baquerizo M, Wang D, Isbell F, Liu J, Feng C, Liu J, Zhong Z, Zhu H, Yuan X, Chang Q, Liu C (2019) Diversifying livestock promotes multidiversity and multifunctionality in managed grasslands. Proc Natl Acad Sci USA 116(13):6187–6192
Wang Z, Han X, Li L (2008) Effects of grassland conversion to croplands on soil organic carbon in the temperate Inner Mongolia. J Environ Manag 86(3):529–534
Wang Z, Song Y, Gulledge J, Yu Q, Liu H, Han X (2009) China’s grazed temperate grasslands are a net source of atmospheric methane. Atmos Environ 43(13):2148–2153
Wang B, Tang H, Xu Y (2017a) Integrating ecosystem services and human well-being into management practices: insights from a mountain-basin area, China. Ecosyst Serv 27:58–69
Wang M, Wagner M, Miguez-Macho G, Kamarianakis Y, Mahalov A, Moustaoui M, Miller J, VanLoocke A, Bagley JE, Bernacchi CJ, Georgescu M (2017b) On the long-term hydroclimatic sustainability of perennial bioenergy crop expansion over the United States. J Clim 30(7):2535–2557
Wehn S, Hovstad KA, Johansen L (2018) The relationships between biodiversity and ecosystem services and the effects of grazing cessation in semi-natural grasslands. Web Ecol 18(1):55–65
Wen L, Dong S, Li Y, Li X, Shi J, Wang Y, Liu D, Ma Y (2013) Effect of degradation intensity on grassland ecosystem services in the alpine region of Qinghai–Tibetan Plateau, China. PLoS ONE. https://doi.org/10.1371/journal.pone.0058432
Article PubMed PubMed Central Google Scholar
Westman WE (1977) How much are nature’s services worth? Science 197(4307):960–964
Weyland F, Laterra P (2014) Recreation potential assessment at large spatial scales: a method based in the ecosystem services approach and landscape metrics. Ecol Indic 39:34–43
White R, Murray S, Rohweder M (2000) Pilot analysis of global ecosystems: grassland ecosystems. World Resources Institute, Washington, DC
Whittaker RH, Likens E (1975) The biosphere and man. In: Lieth H, Whittaker RH (eds) Primary productivity of the biosphere, ecological studies no. 14, vol 306. Springer, Berlin Table 15-1
Winfree R, Fox JW, Williams NM, Reilly JR, Cariveau DP (2015) Abundance of common species, not species richness, drives delivery of a real-world ecosystem service. Ecol Lett 18(7):626–635
Wu JG (2013) Landscape sustainability science: ecosystem services and human well-being in changing landscapes. Landsc Ecol 28:999–1023
Wu JG, Li H (2006a) Concepts of scale and scaling. In: Wu J, Jones KB, Li H, Loucks OL (eds) Scaling and uncertainty analysis in ecology: methods and applications. Springer, Dordrecht, pp 3–15
Wu JG, Li H (2006b) Perspectives and methods of scaling. In: Wu J, Jones KB, Li H, Loucks OL (eds) Scaling and uncertainty analysis in ecology: methods and applications. Springer, Dordrecht, pp 17–44
Wu JG, Naeem S, Elser J, Bai Y, Huang J, Kang L, Pan Q, Wang Q, Hao S, Han X (2015) Testing biodiversity-ecosystem functioning relationship in the world’s largest grassland: overview of the IMGRE project. Landsc Ecol 30:1723–1736
Wu JX, Zhao Y, Yu C, Luo L, Pan Y (2017) Land management influences trade-offs and the total supply of ecosystem services in alpine grassland in Tibet, China. J Environ Manag 193:70–78
Xia J, Liu S, Liang S, Chen Y, Xu W, Yuan W (2014) Spatio-temporal patterns and climate variables controlling of biomass carbon stock of global grassland ecosystems from 1982 to 2006. Remote Sens 6(3):1783–1802
Yahdjian L, Sala OE, Havstad KM (2015) Rangeland ecosystem services: shifting focus from supply to reconciling supply and demand. Front Ecol Environ 13(1):44–51
Yan Y, Xu X, Xin X, Yang G, Wang X, Yan R, Chen B (2011) Effect of vegetation coverage on aeolian dust accumulation in a semiarid steppe of northern China. CATENA 87(3):351–356
Zhang MA, Borjigin E, Zhang HP (2007) Mongolian nomadic culture and ecological culture: on the ecological reconstruction in the agro-pastoral mosaic zone in Northern China. Ecol Econ 62(1):19–26
Zhang H, Fan J, Cao W, Harris W, Li Y, Chi W, Wang S (2018a) Response of wind erosion dynamics to climate change and human activity in Inner Mongolia, China during 1990 to 2015. Sci Total Environ 639:1038–1050
Zhang H, Fan J, Cao W, Zhong H, Harris W, Gong G, Zhang Y (2018b) Changes in multiple ecosystem services between 2000 and 2013 and their driving factors in the Grazing Withdrawal Program, China. Ecol Eng 116:67–79
Zhang X, Niu J, Buyantuev A, Zhang Q, Dong J, Kang S, Zhang J (2016) Understanding grassland degradation and restoration from the perspective of ecosystem services: a case study of the Xilin River Basin in Inner Mongolia, China. Sustainability 8(7):594
Zhao Y, Wu J, He C, Ding G (2017) Linking wind erosion to ecosystem services in drylands: a landscape ecological approach. Landsc Ecol 32(12):2399–2417
Zobeck TM, Parker NC, Haskell S, Guoding K (2000) Scaling up from field to region for wind erosion prediction using a field-scale wind erosion model and GIS. Agric Ecosyst Environ 82(1–3):247–259
Zobeck TM, Sterk G, Funk R, Rajot JL, Stout JE, Van Pelt RS (2003) Measurement and data analysis methods for field-scale wind erosion studies and model validation. Earth Surf Proc Land 28(11):1163–1188
Download references
Acknowledgements
We thank Yingbo Qu and Mobeen Akhtar for technical assistance with literature search and anonymous reviewers for their valuable comments. This work was supported by the Second Tibetan Plateau Scientific Expedition and Research Program of China (2019QZKK0405), National Natural Science Foundation of China (41971130 and 41871185) and the National Basic Research Program of China (2014CB954303).
Author information
Authors and affiliations.
Yanchi Research Station, School of Soil and Water Conservation, Beijing Forestry University, Beijing, 100083, China
Yuanyuan Zhao
Key Laboratory of State Forestry Administration on Soil and Water Conservation, Beijing Forestry University, Beijing, 100083, China
Center for Human–Environment System Sustainability (CHESS), State Key Laboratory of Earth Surface Processes and Resource Ecology (ESPRE), Beijing Normal University, 19 Xinjiekouwai Street, Beijing, 100875, China
Zhifeng Liu & Jianguo Wu
School of Natural Resources, Faculty of Geographical Science, Beijing Normal University, Beijing, 100875, China
Zhifeng Liu
School of Life Sciences and School of Sustainability, Arizona State University, Tempe, AZ, 85287, USA
You can also search for this author in PubMed Google Scholar
Corresponding author
Correspondence to Zhifeng Liu .
Additional information
Publisher's note.
Springer Nature remains neutral with regard to jurisdictional claims in published maps and institutional affiliations.
Rights and permissions
Reprints and permissions
About this article
Zhao, Y., Liu, Z. & Wu, J. Grassland ecosystem services: a systematic review of research advances and future directions. Landscape Ecol 35 , 793–814 (2020). https://doi.org/10.1007/s10980-020-00980-3
Download citation
Received : 07 November 2019
Accepted : 03 February 2020
Published : 15 February 2020
Issue Date : April 2020
DOI : https://doi.org/10.1007/s10980-020-00980-3
Share this article
Anyone you share the following link with will be able to read this content:
Sorry, a shareable link is not currently available for this article.
Provided by the Springer Nature SharedIt content-sharing initiative
- Grassland ecosystem services
- Systematic review
- Provisioning services
- Regulating services
- Cultural services
- Grassland sustainability
- Find a journal
- Publish with us
- Track your research
Grassland Ecosystem and the Energy Flow in the Ecosystem Case Study
1. The primary producers in an ecosystem refer to those organisms in the ecological unit that are capable of synthesising inorganic compounds to produce bio-mass.
The primary producers in grassland ecosystem are the plants consumed by animals (Miller & Spoolman 2012, p. 62). The main three primary producers in the ecosystem include grass, trees and flowers. Among the three, grass is the most common primary producer in ecosystems.
2. A primary consumer in an ecosystem is an animal that consumes and obtains its energy from plants (Miller & Spoolman 2012, p. 62).
In the grass land ecosystem, the main types of primary consumers are wild beasts, which feed on the grass, gazelles, which feed on leaves, and giraffes, which feed on foliage. Apart from the leaves and foliages, the primary consumers in the grass land ecosystem can also feed on the roots and backs of trees.
3. A secondary consumer in an ecosystem is an animal that feeds on and obtains its energy from other animals (Primary consumers). The secondary consumers do not get as much energy the primary consumers (Miller & Spoolman 2012, p. 64). In the grassland ecosystem, the main types of secondary consumers are hyenas, which consume gazelles, wild dogs, which feed on the rabbits and leopards that feed on the wild beasts.
4. A tertiary consumer is the animal at the topmost level in an ecosystem that only feeds on the secondary consumers (Miller & Spoolman 2012, p. 65). In the grassland ecosystem, pythons that feed on the wild dogs are examples of tertiary consumers.
The tertiary consumers are always fewer in any ecosystem. There is a loss of energy from one level to another in an ecosystem. Therefore, tertiary consumers have to be fewer in number to survive on the energy reduction (Keller & Botkin 2008, p. 56).
A Simple Food Chain in Grass Land Ecosystem
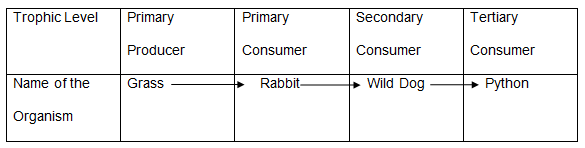
Chemical Cycling in Grassland Ecosystem
1. In the grassland ecosystem, dead organic materials are mainly obtained from the primary producers and primary consumers that happen to die in the ecosystem (Sharma 2005, p. 161).
The non-living things, plant and animal material come from the dead grass, trees, leaves, and branches of the trees, which fall off the remains of primary consumers that are killed by the secondary consumers. The secondary consumers grow old or become sick and die on their own (Miller & Spoolman 2012, p. 72).
2. The dead organic materials in the grassland ecosystem are decomposed by various micro-organisms. There is a continuous breakdown of these materials to become nutrients that the living primary producers obtain from the soil.
The bio-products of the dead organisms in the ecosystem include carbon dioxide, water, phosphates. The rate at which the animals are decomposed depend, among other factors, on the temperature of the area (Sharma 2005, 163).
3. The main micro-organisms that are involved in decomposing the dead materials are fungi and bacteria (Miller & Spoolman 2012, p. 74).
These organisms are called reducers; they reduce the dead organic materials to smaller quantities that can be absorbed by the primary consumers. The decomposers accomplish their functions in the ecosystem through the help of the abiotic components such moisture, solar radiation and temperature (Sharma 2005, p. 167).
Keller, E. A, & Botkin, DB 2008, Essential environment science , John Wiley, Hoboken, NJ.
Miller, G. T., & Spoolman, S 2012, Living in the environment: principles, connection, and solutions , Brooks, Belmont, CA.
Sharma, P. D 2005, Ecology and environment , Rastogi Publications, Meerut.
- Chicago (A-D)
- Chicago (N-B)
IvyPanda. (2019, April 11). Grassland Ecosystem and the Energy Flow in the Ecosystem. https://ivypanda.com/essays/grassland-ecosystem-and-the-energy-flow-in-the-ecosystem/
"Grassland Ecosystem and the Energy Flow in the Ecosystem." IvyPanda , 11 Apr. 2019, ivypanda.com/essays/grassland-ecosystem-and-the-energy-flow-in-the-ecosystem/.
IvyPanda . (2019) 'Grassland Ecosystem and the Energy Flow in the Ecosystem'. 11 April.
IvyPanda . 2019. "Grassland Ecosystem and the Energy Flow in the Ecosystem." April 11, 2019. https://ivypanda.com/essays/grassland-ecosystem-and-the-energy-flow-in-the-ecosystem/.
1. IvyPanda . "Grassland Ecosystem and the Energy Flow in the Ecosystem." April 11, 2019. https://ivypanda.com/essays/grassland-ecosystem-and-the-energy-flow-in-the-ecosystem/.
Bibliography
IvyPanda . "Grassland Ecosystem and the Energy Flow in the Ecosystem." April 11, 2019. https://ivypanda.com/essays/grassland-ecosystem-and-the-energy-flow-in-the-ecosystem/.
- Grassland Genomes Adaptation to Environmental Change
- Theoretical Perspective on the Giraffe's Adaptation
- Evolution of Predator and Prey Pairings
- Burmese Pythons
- Water Yield Re-Estimation From the Catchment Due to Bushfire
- Maasai Mara Trip and Preparations
- Greenhouse Gases Produced by Grasslands
- Electricity Generation from Decomposing Food
- Overview of Computer Languages - Python
- Recovery Plans in Australia
- Wildlife Tourism Essay
- The Impact of Logging and Deforestations on an Ecosystem
- Environmental Issue raised in the “Weather Extremes Leave Parts of U.S. Grid Buckling”
- Rolston and Callicott into the transforming concepts
- Ecological Imperialism
Grassland Habitat
A herd of antelope moves slowly through the tall grass. Suddenly a cheetah leaps from its hiding place, and the animals zig and zag across the savanna. They make it to a small grove of scrubby trees, but they can’t let their guard down yet. It’s the end of the rainy season and the antelopes—like many animals on the grasslands—must leave their protected place and migrate across open grasslands to find water.
Between the Forest and the Desert
Grassland habitats are places that receive more rain than deserts but less precipitation than forests. Most of the plants here are grasses, which don’t need as much water as forest vegetation.
Every continent except Antarctica has grasslands, but they have different names in different places: In Africa, they’re called savannas and veldts; pampas in South America; steppes in in Europe and Asia; prairies or grasslands in North America; and rangelands in Australia . One-fourth of the planet is covered by grasslands.
Grasslands are usually found in the dry interior of continents, between the mountains and deserts. Many grasslands were formed tens of thousands of years ago after the last ice age, when the Earth began to warm up and the climate became drier.
Wildfires can be bad business, but sometimes they help the habitat stay healthy. Nutrients are released into the soil by microbes that become more active when the soil temperature rises. And because the stems and buds of grasses grow underground, they aren’t damaged by fire and therefore grow back quickly.
Grass isn’t just … grass. Lots of different kinds of grasses grow in the habitat. Wild oats, foxtail, purple coneflowers, buffalo grass, goldenrod, and sunflowers are just a few of the types of grasses that grow and flower on grasslands.
Oh, Give Me a Home …
The Serengeti in northern Tanzania and southwestern Kenya , countries in Africa, is nearly as big as Maryland and is home to animals such as zebras , gazelles, wildebeests, cheetahs , lions , and leopards . One of the largest grasslands in the world is the Eurasian Steppe. It stretches from Hungary to China —almost one-fifth of the way around the world—and has animals like saiga antelope and vultures.
Kangaroo and flightless birds like emu and ostriches live throughout Australia’s rangeland, which covers almost the entire continent. In North America, deer, antelope, rabbits , and prairie dogs roam the grassland. As well as buffalo (usually called bison ) … just like the song says!
Watch "Destination World"
North america, south america, read this next, nature boom time videos, u.s. states and territories facts and photos, save the earth tips.
- Terms of Use
- Privacy Policy
- Your California Privacy Rights
- Children's Online Privacy Policy
- Interest-Based Ads
- About Nielsen Measurement
- Do Not Sell My Info
- National Geographic
- National Geographic Education
- Shop Nat Geo
- Customer Service
- Manage Your Subscription
Copyright © 1996-2015 National Geographic Society Copyright © 2015-2024 National Geographic Partners, LLC. All rights reserved
Your Article Library
Grassland Ecosystem : Essay on Grassland Ecosystem!
ADVERTISEMENTS:
Grasslands (also called Greenswards) are areas where the vegetation is dominated by grasses and other herbaceous (non-woody) plants. Grasslands occupy about 24% of the earth’s surface. They occur in regions too dry for forests and too moist for deserts.
The annual rainfall ranges between 25- 75 cm, usually seasonal. The principal grasslands includes Prairies (Canada, USA), Pampas (South America), Steppes (Europe and Asia), and Veldts (Africa).
The highest abundance and greatest diversity of large mammals are found in these ecosystems. The dominant animal species include wild horses, asses and antelope of Eurasia, herds of Bison of America; and the antelope and other large herbivores of Africa.
Biotic Components:
1. Producer Organisms:
In grassland, producers are mainly grasses; though, a few herbs and shrubs also contribute to primary production of biomass. Some of the most common species of grasses are: Brachiaria sp., Cynodon sp., Desmodium sp., Digitaria sp.
2. Consumers:
In grassland, consumers are of three main types:
(a) Primary Consumers:
The primary consumers are herbivores feeding directly on grasses. These are grazing animals such as Cows, Buffaloes, Sheep, Goats, Deer, and Rabbits etc. Besides them, numerous species of insects, termites, etc. are also present.
(b) Secondary Consumers:
These are carnivores that feed on primary consumers (Herbivores). These include;-Frogs, Snakes, Lizards, Birds, Foxes, Jackals etc.
(c) Tertiary Consumers:
These include hawks etc. which feed on secondary consumers.
3. Decomposers:
These include wide variety of saprotrophic microorganism like: Bacteria; Fungi; Actinomycetes.
A-biotic Components:
These include basic inorganic and organic compounds present in the soil and aerial environment. The essential elements like C, H, N, O, P, S etc. are supplied by water, nitrogen, nitrates, sulphates, phosphates present in soil and atmosphere.
Related Articles:
- Forest Ecosystem (Terrestrial Ecosystem)
- Types of Ecosystem: Grassland, Forest, Desert and Cropland Ecosystem
Grassland Ecosystem
No comments yet.
Leave a reply click here to cancel reply..
You must be logged in to post a comment.
- IAS Preparation
- UPSC Preparation Strategy
- Grassland Ecosystem Components Structure Economic Importance
Grassland Ecosystem
Grasslands are areas dominated by grasses. They occupy about 20% of the land on the earth surface. Grasslands occur in both in tropical and temperate regions where rainfall is not enough to support the growth of trees.
Grasslands are found in areas having well-defined hot and dry, warm and rainy seasons.
This article will provide information about the Grassland Ecosystem, the classification of grasslands, other different names of grasslands, in the context of the IAS Exam.
Explore The Ultimate Guide to IAS Exam Preparation
Download The E-Book Now!
This is a part of the Geography and Environment & Ecology section of the UPSC Syllabus.
The candidates can read more relevant information from the links provided below:
Types of Natural Ecosystem
A natural ecosystem is a setup of animals and plants which functions as a unit and is capable of maintaining its identity. A natural ecosystem is totally dependent on solar energy. There are two main categories of ecosystems. They are:
- Terrestrial ecosystem – Ecosystems found on land e.g. forest, grasslands, deserts, tundra.
- Freshwater ecosystems, such as rivers, lakes and ponds.
- Marine ecosystems, such as oceans, estuaries.
Grassland Ecosystems – An Introduction
- Grasslands are one of the intermediate stages in ecological succession and cover a part of the land on all the altitudes and latitudes at which climatic and soil conditions do not allow the growth of trees.
- Grasslands make up almost a quarter of the total land surface. The types of plants that grow here greatly depend on what the climate and soil are like.
- Grasslands cover areas where rainfall is usually low and/or the soil depth and quality is poor.
- The low rainfall prevents the growth of numerous trees and shrubs but is sufficient to support the growth of grass cover during the monsoon.
Different Names of Grasslands
Grasslands are known by various names in different parts of the world.
Tropical grasslands are commonly called Savannas. They occur in eastern Africa, South America, Australia and India. Savannas form a complex ecosystem with scattered medium-size trees in grasslands.
Structure/Components of Grassland Ecosystem
The different structural components of the grassland ecosystem can be classified as abiotic and biotic components.
Biotic Components
- Producers – In grassland, producers are mainly grasses; though, a few herbs & shrubs also contribute to the primary production of biomass.
- Primary Consumers – The primary consumers are herbivores feeding directly on grasses. Herbivores such as grazing mammals (e.g., cows, sheep, deer, rabbit, buffaloes, etc), insects (e.g., Dysdercus, Coccinella, Leptocorisa, etc), some termites and millipedes are the primary consumers.
- Secondary Consumers – These are carnivores that feed on primary consumers (Herbivores). The animals like foxes, jackals, snakes, frogs, lizards, birds etc., are the carnivores feeding on the herbivores. These are the secondary consumers of the grassland ecosystem.
- Tertiary Consumers – These include hawks etc. which feed on secondary consumers.
- Decomposers – These include bacteria of death and decay, moulds and fungi (e.g., Mucor, Penicillium, Aspergillus, Rhizopus, etc). These bring the minerals back to the soil to be available to the producers again.
Abiotic Components
- These include the nutrients present in the soil and the aerial environment.
- The elements required by plants are hydrogen, oxygen, nitrogen, phosphorus and sulphur.
- These are supplied by the soil and air in the form of CO2, water, nitrates, phosphates and sulphates.
- In addition to these, some trace elements are also present in the soil.
Flora and Fauna of Grassland Ecosystem
- Grasses are the dominating plants, with scattered drought resistant thorny trees in the tropical grasslands.
- Badgers, fox, ass, zebra, antelope are found grazing on grasslands that support the dairy and leather industries.
- Grasslands also support the large population of rodents, reptiles and insects.
Functions of Grassland Ecosystem
They perform certain functions. These are:
- Energy flow through the food chain
- Nutrient cycling (biogeochemical cycles)
- Ecological succession or ecosystem development
- Homeostasis (or cybernetic) or feedback control mechanisms
- To increase the fertility of the soil and to regulate the productivity of the ecosystem.
- To reduce the leaching of minerals due to low rainfall.
Economic Importance of Grasslands
- Grasslands are the grazing areas of many rural communities.
- Farmers who keep cattle or goats, as well as shepherds who keep sheep, are highly dependent on grasslands.
- Domestic animals are grazed in the ‘common’ land of the village.
- Fodder is collected and stored to feed cattle when there is no grass left for them to graze in summer.
- The grass is also used to thatch houses and farm sheds.
- The thorny bushes and branches of the few trees that are seen in grasslands are used as a major source of fuelwood.
- Overgrazing by huge herds of domestic livestock has degraded many grasslands.
- Grasslands have diverse species of insects that pollinate crops.
- There are also predators of these insects such as small mammals like shrews, reptiles like lizards, birds of prey, and amphibia such as frogs and toads.
- All these carnivorous animals help to control insect pests in adjoining agricultural lands.
Kickstart your IAS preparation now and complement it with the links given below:
- Daily Current Affairs
- IAS Environment Questions
- UPSC Mains GS-I Strategy, Structure & Syllabus
- Topic-wise IAS Prelims Questions with Solutions
- FAQ on UPSC IAS 2021 for Beginners
- 100 Difference Between Articles
Classification of Grasslands
As climate plays an important role in the formation of grasslands, it is generally used as a basis to divide the world’s grasslands into two broad categories: those that occur in the temperate region and those that occur in the tropical regions.
Tropical Grasslands
- These occur on either side of the equator and extend to the tropics.
- This vegetation grows in areas of moderate to a low amount of rainfall.
- The grass can grow very tall, about 3 to 4 metres in height.
- Savannah grasslands of Africa are of this type.
- Elephants, zebras, giraffes, deer, leopards are common in tropical grasslands
Temperate Grasslands
- These are found in the mid latitudinal zones and in the interior part of the continents.
- Usually, the grass here is short and nutritious.
- Wild buffaloes, bison, antelopes are common in the temperate region.
Grasslands in India
- In India, grasslands are found as village grazing grounds (Gauchar) and extensive low pastures of dry regions of the western part of the country and also in Alpine Himalayas.
- Perennial grasses are the dominant plant community.
- In the Himalayan mountains, there are high, cold Himalayan pastures.
- There are tracts of tall elephant grass in the low-lying Terai belt south of the Himalayan foothills.
- There are semi-arid grasslands in Western India, parts of Central India, and the Deccan Plateau.
- Patches of shola grasslands that occur on hill slopes alongside the extremely moist evergreen forests in South India.
- In some regions, grasslands also support a variety of other herbaceous plants like sedges, legumes and members of the sunflower family.
- Grasslands support numerous herbivores, from minute insects to very large mammals.
- Rats, mice, rodents, deer, elephants, dogs, buffalo, tigers, lions, ferrets are some common mammals of grasslands.
- In northeast India, the one-horned rhinoceros is amongst the threatened animal of grassland in this region.
- A large number of avian fauna makes the grassland colourful.
Interested candidates may like to know more about the natural vegetation of India . Click the linked article to learn about it.
UPSC 2021 Banner:

Other related links:
Leave a Comment Cancel reply
Your Mobile number and Email id will not be published. Required fields are marked *
Request OTP on Voice Call
Post My Comment

IAS 2024 - Your dream can come true!
Download the ultimate guide to upsc cse preparation.
- Share Share
Register with BYJU'S & Download Free PDFs
Register with byju's & watch live videos.
Sciencing_Icons_Science SCIENCE
Sciencing_icons_biology biology, sciencing_icons_cells cells, sciencing_icons_molecular molecular, sciencing_icons_microorganisms microorganisms, sciencing_icons_genetics genetics, sciencing_icons_human body human body, sciencing_icons_ecology ecology, sciencing_icons_chemistry chemistry, sciencing_icons_atomic & molecular structure atomic & molecular structure, sciencing_icons_bonds bonds, sciencing_icons_reactions reactions, sciencing_icons_stoichiometry stoichiometry, sciencing_icons_solutions solutions, sciencing_icons_acids & bases acids & bases, sciencing_icons_thermodynamics thermodynamics, sciencing_icons_organic chemistry organic chemistry, sciencing_icons_physics physics, sciencing_icons_fundamentals-physics fundamentals, sciencing_icons_electronics electronics, sciencing_icons_waves waves, sciencing_icons_energy energy, sciencing_icons_fluid fluid, sciencing_icons_astronomy astronomy, sciencing_icons_geology geology, sciencing_icons_fundamentals-geology fundamentals, sciencing_icons_minerals & rocks minerals & rocks, sciencing_icons_earth scructure earth structure, sciencing_icons_fossils fossils, sciencing_icons_natural disasters natural disasters, sciencing_icons_nature nature, sciencing_icons_ecosystems ecosystems, sciencing_icons_environment environment, sciencing_icons_insects insects, sciencing_icons_plants & mushrooms plants & mushrooms, sciencing_icons_animals animals, sciencing_icons_math math, sciencing_icons_arithmetic arithmetic, sciencing_icons_addition & subtraction addition & subtraction, sciencing_icons_multiplication & division multiplication & division, sciencing_icons_decimals decimals, sciencing_icons_fractions fractions, sciencing_icons_conversions conversions, sciencing_icons_algebra algebra, sciencing_icons_working with units working with units, sciencing_icons_equations & expressions equations & expressions, sciencing_icons_ratios & proportions ratios & proportions, sciencing_icons_inequalities inequalities, sciencing_icons_exponents & logarithms exponents & logarithms, sciencing_icons_factorization factorization, sciencing_icons_functions functions, sciencing_icons_linear equations linear equations, sciencing_icons_graphs graphs, sciencing_icons_quadratics quadratics, sciencing_icons_polynomials polynomials, sciencing_icons_geometry geometry, sciencing_icons_fundamentals-geometry fundamentals, sciencing_icons_cartesian cartesian, sciencing_icons_circles circles, sciencing_icons_solids solids, sciencing_icons_trigonometry trigonometry, sciencing_icons_probability-statistics probability & statistics, sciencing_icons_mean-median-mode mean/median/mode, sciencing_icons_independent-dependent variables independent/dependent variables, sciencing_icons_deviation deviation, sciencing_icons_correlation correlation, sciencing_icons_sampling sampling, sciencing_icons_distributions distributions, sciencing_icons_probability probability, sciencing_icons_calculus calculus, sciencing_icons_differentiation-integration differentiation/integration, sciencing_icons_application application, sciencing_icons_projects projects, sciencing_icons_news news.
- Share Tweet Email Print
- Home ⋅
- Science ⋅
- Nature ⋅
What Are the Impacts of Humans on Grassland Biomes?
Grassland Biome Dangers
Population growth among humans negatively impacts biomes around the world. Expanding human civilization affects grassland biomes – characterized by large areas of land where grasses are the primary form of plant life – in specific ways. The grazing land for many species of animals, which in turn provide a food source for larger predators, is often at risk because of human expansion into these areas.
Urban Development
The biggest impact that humans have on grasslands is by developing open areas for farming or urban development. Such development is prevalent because grasslands are generally level areas with little need for major work to develop the land. Land development drives animals away from populated areas and changes the conditions of the environment.
Agriculture and Farming
Grasslands covered to cropland or farms reduces the food source for many wild animals. In this case, the animals are considered pests by the farmers when they feed on the crops, or attack domestic herds. This can lead to migration or possibly the wildlife starvation.
Not only does the conversion of land into crops change the ecosystem, but so does the farming of livestock. If livestock are allowed to graze in areas where wild animals live, they compete for the food source and can deplete it. This overgrazing is a problem especially in the drier grassland regions, where the grass resources can be depleted. Over-plowed land strips rich nutrients from oil. Salts from irrigation waters damage soil, resulting in dust bowls, similar to what happened in the 1930s American West.
Hunting to Extinction
Hunting presents a serious impact on grassland biomes. European settlers devastated the American bison population which almost became extinct because of over hunting for the fur and meat. Poachers likewise kill rhinoceroses for their tusks, and elephants for their ivory on Africa savannas without any regard to protection of the species.
Global Warming
As the Earth’s climate changes in response to human involvement, the grasslands become vulnerable. Climate change causes ecological succession, in which the ecosystem of an area develops into another. Changing temperatures, weather patterns and water availability can throw an area of grassland out of balance and change it forever.
Drier Climates and Fires
Because grasslands are typically found in drier climates, the plant life is susceptible to fire. Wildfires occur as a natural process within an ecosystem and play a critical role in replenishing the land. But fires tend to originate more frequently near human populations, particularly in drier months.
Positive Impacts
Humans do not have only a negative impact on grasslands. Some humans do their part to preserve the land and restore it. National parks have been developed around grasslands, and some organizations replant depleted areas. Governments have enacted laws against the hunting of endangered animals. In particular, the U.S. National Parks Service has preserved land to foster the American bison population. While poaching still exists in many areas, there are efforts to stop it.
Related Articles
The kinds of human activities that have destroyed ecosystems, desert biome environmental problems, what are the causes of the destruction of ecosystem, why are jaguars endangered animals, human influences on the temperate rainforest, what ecological problems and hazards does the desert..., what are environmental problems in temperate shrublands, negative effects of pollution, dangers to the savanna ecosystem, what is the human impact on the tundra, cambodia's environmental problems, how is a biome formed, great plains of north america animals & plants, the effects of human intervention on the environment, what type of ecosystem do lions live in, facts on overpopulation and deforestation in the amazon..., landforms of the grasslands biome, four consequences of deforestation.
- University of California Museaum of Paleontology: The World's Biomes
- University of California Museaum of Paleontology: The Grassland Biome
- Oregon State University: Important Issues Affecting Grasslands and Their Forages
About the Author
Kevin Carr has been writing for a variety of outlets and companies since 1991. He has contributed to McGraw-Hill textbooks for middle school and high school, written for the Newspaper Network of Central Ohio and has been a featured film critic for online publications including 7M Pictures and Film School Rejects. Carr holds a Bachelor of Science in education.
Find Your Next Great Science Fair Project! GO
Home — Essay Samples — Environment — Environmental Issues — The Human Impact on Grassland Biomes: Causes and Consequences

The Human Impact on Grassland Biomes: Causes and Consequences
- Categories: Environmental Issues
About this sample

Words: 629 |
Published: Mar 8, 2024
Words: 629 | Page: 1 | 4 min read
Table of contents
Conversion into agricultural lands, urbanization, climate change, economic activities, recreational activities.
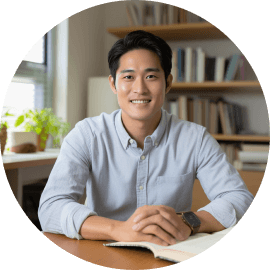
Cite this Essay
Let us write you an essay from scratch
- 450+ experts on 30 subjects ready to help
- Custom essay delivered in as few as 3 hours
Get high-quality help
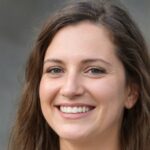
Verified writer
- Expert in: Environment
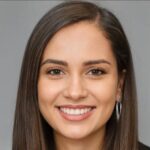
+ 120 experts online
By clicking “Check Writers’ Offers”, you agree to our terms of service and privacy policy . We’ll occasionally send you promo and account related email
No need to pay just yet!
Related Essays
3 pages / 1169 words
3 pages / 1312 words
2 pages / 1102 words
2 pages / 668 words
Remember! This is just a sample.
You can get your custom paper by one of our expert writers.
121 writers online
Still can’t find what you need?
Browse our vast selection of original essay samples, each expertly formatted and styled
Related Essays on Environmental Issues
ABC News. (n.d.). 'Irreversible Climate Change.' Retrieved from 19.
Biochemistry, the study of chemical processes within living organisms, plays a crucial role in addressing environmental challenges and advancing sustainability efforts. Understanding the biochemical processes of organisms, [...]
The natural environment plays a vital role in sustaining life on Earth, providing oxygen and water that are essential for all living beings.However, the movie The Lorax depicts a walled city called Thneedville where destructive [...]
The environment is the foundation of life on earth and ensures that humans and other living organisms can thrive. However, human activities have created significant environmental challenges in the modern world. This essay will [...]
Littering and pollution is a major issue around the world today. It affects all of our lives, and will affect us for years to come. Littering and pollution play a major part in our lives. Everywhere we walk and drive there is [...]
“Men occasionally stumble over the truth, but most of them pick themselves up and hurry off as if nothing ever happened.” (Winston S. Churchill). Humans tend to act ignorant when it comes to the painful truth for they believe [...]
Related Topics
By clicking “Send”, you agree to our Terms of service and Privacy statement . We will occasionally send you account related emails.
Where do you want us to send this sample?
By clicking “Continue”, you agree to our terms of service and privacy policy.
Be careful. This essay is not unique
This essay was donated by a student and is likely to have been used and submitted before
Download this Sample
Free samples may contain mistakes and not unique parts
Sorry, we could not paraphrase this essay. Our professional writers can rewrite it and get you a unique paper.
Please check your inbox.
We can write you a custom essay that will follow your exact instructions and meet the deadlines. Let's fix your grades together!
Get Your Personalized Essay in 3 Hours or Less!
We use cookies to personalyze your web-site experience. By continuing we’ll assume you board with our cookie policy .
- Instructions Followed To The Letter
- Deadlines Met At Every Stage
- Unique And Plagiarism Free
- Share full article
Advertisement
Supported by
Guest Essay
An Octopus Took My Camera, and the Images Changed the Way I See the World

By Craig Foster
Mr. Foster’s film, “My Octopus Teacher,” won the Academy Award for best documentary feature in 2021. He wrote from Simon’s Town, South Africa.
I was gifted with a new way of seeing the day I got mugged underwater. I had been filming creatures living in the Great African Sea Forest off the coast of South Africa about a year ago when my camera was grabbed straight out of my hands by a young octopus thief. Wrapping her arms around her bounty, she zoomed backward across the ocean floor.
This was not the first time I’d found myself at the mercy of an eight-armed robber. A couple of years earlier, another curious octopus stole the wedding ring off my wife’s finger, never to be recovered. Octopuses love novel shiny things. Peering into their dens, I’ve found earrings, bracelets, spark plugs, sunglasses and a toy car with a revolving cylinder that the octopus spun round and round with its suckers.
As I wondered how to get my camera back without alarming my young friend, something surprising happened. She turned the camera around and began to film my diving partner and me.
The intriguing images she captured — videos of her own arms draped over the camera lens with our bodies in the background — had a profound effect on me. After many years filming octopuses and hundreds of other animals that call the Sea Forest home, for the first time I was seeing the world — and myself — from her perspective.
We must have looked strange to her in our masks and with our underwater flashlights. But in that moment I remembered that despite all our technology, we are not so different from our animal kin. Every breath of air, every drop of water, every bite of food comes from the living planet we share.
Monday is Earth Day, and I am tempted to ask myself how humanity can save our wild planet and undo the devastation we have unleashed upon the natural world. Where I live, in the Cape of Good Hope, I am privileged to be surrounded by nature, but we are grappling with pollution and dwindling numbers of shellfish, fish, raptors and insect species. Worldwide, we are at a tipping point with an estimated 69 percent decline in wildlife populations.
When I consider the vast network of living creatures on earth, it’s clear that “saving the planet” is the wrong goal. Unless earth gets obliterated by an asteroid or experiences some similar catastrophic event, the planet could go on for several billion years. But without the biosphere that makes it possible for us to eat and breathe, humanity could not survive.
The question we should be asking is what caused the precipitous increase in species loss and what can we do to reverse it. To me, it all started when we disconnected from our wild origins. While agricultural and technological revolutions have enabled massive population growth and innovation , they have also instilled the belief that we can control nature, that our planet is an infinite resource to be mined for our advancement, comfort and entertainment.
Today 56 percent of the world population lives in urban areas, a percentage expected to grow to nearly 70 percent by 2050 . That means that more than half of us are cut off from reminders that we are still part of nature and utterly dependent on its health. It’s only when something truly devastating happens, like the recent flooding in Dubai, that we remember that even the greatest human advancements can be brought to a standstill by nature’s power.
I am not calling for us to leave all modern comforts behind, just pleading for us to get to know nature better, rather than try to “save” her.
In the past decade I have taken more than 4,000 dives in the Sea Forest. My encounters with mollusks, sharks and jellyfish there have convinced me that there is much we will lose if we do not value the tremendous abundance of life on earth.
We do this first by protecting biodiversity hot spots and by restoring degraded ecosystems; the enormous regenerative power I see every day in nature is what gives me hope for the future. It also means learning from and supporting Indigenous people who protect 80 percent of the world’s biodiversity and who have, over millenniums, developed many innovative ways to live with the land and sea. One promising example of partnership is a recent grant from the National Science Foundation to support collaboration between Indigenous ecological knowledge and Western science.
Activities that cause long-term destruction of the sea and earth, such as strip mining , deep sea mining and industrial trawling, need to be halted immediately. Farming methods have to change, with greater emphasis on soil recovery and regeneration . We must continue to find alternatives to fossil fuels and push for a worldwide reduction in the production and use of plastics.
But each of us has a role to play, too; it starts with challenging ourselves to reconnect with the wild. So much of our modern world seems designed to tame us: to dull our minds, to separate us from the natural world, to convince us that what will help us survive is more consumption.
Like my octopus friends, we fill our houses with shiny new things. But our piles of stuff are much bigger and the cost of acquisition much greater.
We can break free of this tame conditioning. When we dedicate even just a few minutes per day to observing wild creatures on their own terms, in their own homes, regardless of where we live, we connect with the concept of biodiversity not simply on an intellectual level but also on an emotional level. We see the world differently — and ourselves, too.
How strange it is that one silly primate can see itself as separate from all those it shares this world with. What might happen if we remembered we are a part of this wild world — and let that understanding and humility guide every choice we make?
Craig Foster is a co-founder of the Sea Change Project and the author of the forthcoming “Amphibious Soul: Finding the Wild in a Tame World.” His film “My Octopus Teacher” won the Academy Award for best documentary feature in 2021.
The Times is committed to publishing a diversity of letters to the editor. We’d like to hear what you think about this or any of our articles. Here are some tips . And here’s our email: [email protected] .
Follow the New York Times Opinion section on Facebook , Instagram , TikTok , WhatsApp , X and Threads .
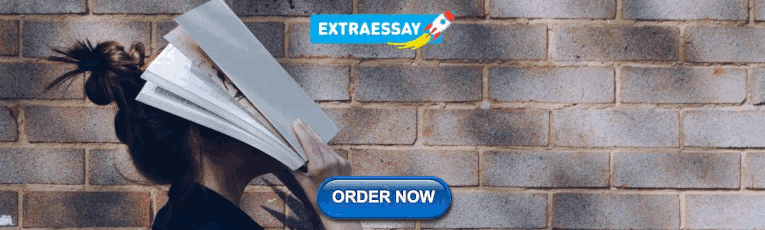
IMAGES
VIDEO
COMMENTS
The grassland ecosystem itself influences soil formation, and this causes grassland soils to differ from other soils. The nature of grass litter and its pattern of decomposition commonly result in the development of a dark, organically rich upper soil layer that can reach 300 millimetres below the surface.
Grassland biomes consist of large open areas of grass. Trees can be present, but they are infrequent. The animals found in grasslands range from African elephants (Loxodonta africana) to various species of prairie dogs (Cynomys spp.).. Low rainfall, wildland fires, and grazing by animals are three factors that maintain grasslands.In grassland regions, the climate is ideal for the growth of ...
A Grassland Ecosystem is a mixture of small herbs, weeds, grass, trefoil, dicotyledonous, shrubs and other leguminous species, contributing to a high degree of preservation. The economic importance of the grassland ecosystem is that it serves in the maintenance of the crop of many domesticated and wild herbivores such as cattle, sheep, goats ...
Tropical grasslands include the hot savannas of sub-Saharan Africa and northern Australia. Rainfall can vary across grasslands from season to season and year to year, ranging from 25.4 too 101.6 centimeters (10 to 40 inches) annually. Temperatures can go below freezing in temperate grasslands to above 32.2 degrees Celsius (90 degrees Fahrenheit).
Ecosystems in which grass is the dominant vegetation (grasslands) are found on every continent besides Antarctica. They are found in areas that are both too dry to support forests and too wet to support deserts. They are maintained by various environmental factors, including grazing, fires, and seasonal drought.
Introduction. Grasslands are one of the major ecosystems of the world, covering close to one-third of the Earth's terrestrial surface (Suttie et al. 2005, Lemaire et al. 2011).Extensively managed grasslands are recognized globally for their high biodiversity (Habel et al. 2013), and together with other rangelands, they often contribute to agricultural production through livestock grazing on ...
The global cost of grassland degradation on livestock production has been estimated at $6.8 billion over the period 2001-2011 (ref. 11 ), with the resulting impact on human welfare being ...
Further, because grasslands thrive off of biodiversity of plants and animals, monocropping that provides only a single type of plant tends to weaken the biome and increases vulnerability to ...
1. Introduction. Constituting almost 40% of the terrestrial biosphere, grasslands provide the habitat for a great number of diverse animals and plants and contribute to the livelihoods of more than 1 billion people worldwide [].Under the growing impact of global climate change and unreasonable human activities, grasslands are facing major problems that threaten the sustainable development of ...
The multitude of terms for grasslands presents barriers to consistent deinitions, data collection, and recognition of their true extent and value.5 The varied terminology relects the heterogeneity of grassland types and their plant compositions worldwide. This makes it hard to perceive grasslands as a single, uniied ecosystem type. In contrast,
Context Grasslands provide a variety of ecosystem services (ESs) for humans. While much ES research has focused on forests and wetlands, synthesizing the currently somewhat sporadic studies of grassland ecosystem services (GESs) is much needed. Objectives We aimed to review the scope, major methods, and key findings of GESs, and identify knowledge gaps and future directions. Methods We ...
1. The primary producers in an ecosystem refer to those organisms in the ecological unit that are capable of synthesising inorganic compounds to produce bio-mass. The primary producers in grassland ecosystem are the plants consumed by animals (Miller & Spoolman 2012, p. 62). The main three primary producers in the ecosystem include grass, trees ...
Nachusa Grasslands, spring 2016 Setaria pumila, a species of Poaceae (the dominant plant family in grasslands). A grassland is an area where the vegetation is dominated by grasses ().However, sedge and rush can also be found along with variable proportions of legumes, like clover, and other herbs.Grasslands occur naturally on all continents except Antarctica and are found in most ecoregions of ...
Understanding the grassland ecosystem is crucial for improving grassland ecosystem functions and services such as climate regulation, water and soil conservation, carbon sequestration, and biodiversity and gene pool maintenance. However, a systematic and comprehensive review of the relevant literature is still unclear and lacking. The VOSviewer software and cluster analysis were used to ...
Grassland Habitat. A herd of antelope moves slowly through the tall grass. Suddenly a cheetah leaps from its hiding place, and the animals zig and zag across the savanna. They make it to a small grove of scrubby trees, but they can't let their guard down yet. It's the end of the rainy season and the antelopes—like many animals on the ...
ADVERTISEMENTS: Grassland Ecosystem : Essay on Grassland Ecosystem! Grasslands (also called Greenswards) are areas where the vegetation is dominated by grasses and other herbaceous (non-woody) plants. Grasslands occupy about 24% of the earth's surface. They occur in regions too dry for forests and too moist for deserts. ADVERTISEMENTS: The annual rainfall ranges between 25- 75 […]
Flora and Fauna of Grassland Ecosystem. Grasses are the dominating plants, with scattered drought resistant thorny trees in the tropical grasslands. Badgers, fox, ass, zebra, antelope are found grazing on grasslands that support the dairy and leather industries. Grasslands also support the large population of rodents, reptiles and insects.
Essay On Grassland Biomes. 943 Words4 Pages. Introduction. Grassland biomes is a large area of land consisting of grasses, and flowers with trees, less or no trees depending on the type of grassland. There are major types of grassland biomes which are hot, mild and cold grasslands. Grasslands have adequate precipitation which supports large ...
Urban Development. The biggest impact that humans have on grasslands is by developing open areas for farming or urban development. Such development is prevalent because grasslands are generally level areas with little need for major work to develop the land. Land development drives animals away from populated areas and changes the conditions of ...
In conclusion, human activities have a significant impact on grassland biomes. The rate and nature of human impact have resulted in significant degradation of grassland ecosystems. Agriculture, urbanization, climate change, economic activities, and recreational activities are among the key ways in which humans are changing grasslands. It is ...
In the fight to curb climate change, grasslands are an important complement to forests and other high carbon-storage ecosystems (Yang et al., 2019).For example, grasslands contribute to cooling through solar radiation albedo effects (Temperton et al., 2019) and are notably resilient and resistant to changing disturbance regimes, even in the face of extreme events (Dass et al., 2018).
Biome Ecosystem Essay When it comes to a biome and an ecosystem, they're very different and some people do not realize that or simply do not understand the difference between the two. When it comes to a biome it is the specific geographical area were the species live, whereas an ecosystem is the interaction between everything living and non ...
It showed a total reduction of 20% of the grassland vegetation in the biome from 1985-2022, including 9.1 million hectares (22.5 million acres) of native grassland.
Agricultural land in Germany is covered by 28% by grassland [], of which approximately 20% is situated within the influence of ascending groundwater [].For national assessments of agricultural production and related ecosystem services and disservices, the consideration of grasslands beside cropland is therefore essential, especially because a large fraction of the wet grasslands have been ...
Reactive nitrogen (N) can significantly influence the richness and abundance of a myriad of organisms; however, it remains unclear how the addition of N affects soil nematodes and their associated ecosystem functions in natural grasslands on a global scale. For this study, we synthesized data on the responses of soil nematode taxon richness and abundance to N addition based on 72 published ...
Mr. Foster's film, "My Octopus Teacher," won the Academy Award for best documentary feature in 2021. He wrote from Simon's Town, South Africa. I was gifted with a new way of seeing the day ...