- Reference Manager
- Simple TEXT file
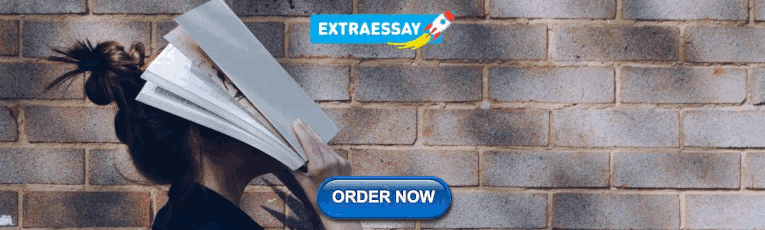
People also looked at
Review article, obesity or bmi paradox beneath the tip of the iceberg.
- Department of Experimental Medicine, Sapienza University, Rome, Italy
The obesity paradox refers to extant evidence showing that obesity in older subjects or in patients with several chronic diseases may be protective and associated with decreased mortality. A number of mechanisms have been postulated to support the existence of obesity paradox; however, marked heterogeneity was found across studies and this has cast doubt on the actual presence of this phenomenon. The aim of the present narrative review is to summarize evidence underlying the concept of obesity paradox, focusing on limitations and bias related to this phenomenon, with emphasis on the use of body mass index (BMI). A major cause of the discrepancy between studies may be related to the use of BMI in the definition of obesity, that should consider, instead, excess body fat as the main characteristic of this disease and as the unique determinant of its complications. In addition, the adjustment for potential confounders (e.g., stage and grade of diseases, smoking habit, inability to capture the presence of signs of undernutrition in the normal-weight comparative group, consideration of body composition) may significantly scale down the protective role of obesity in terms of mortality. However, it is still necessary to acknowledge few biases (e.g., reverse causation, attrition bias, selection bias of healthy obese subjects or resilient survivors) that would still apply to obesity even when defined according with body composition. Further research should be prompted in order to promote correct phenotyping of patients in order to capture properly the trajectories of mortality in a number of diseases.
Introduction
The obesity paradox refers to extant evidence showing that obesity in older subjects or in patients with several chronic diseases may be protective and associated with decreased mortality. Gruberg et al. ( 1 ) first observed that overall mortality (1 year follow-up) was significantly higher in patients with coronary artery disease after percutaneous coronary intervention and normal body mass index (BMI) compared to overweight/obese subjects. Since then, a number of studies, encompassed by the umbrella term “reverse epidemiology,” found that obesity, hypercholesterolemia, and hypertension were associated with improved survival among dialysis patients ( 2 ), in chronic heart failure (CHF) ( 3 ), after acute myocardial infarction ( 4 ), in chronic obstructive pulmonary disease ( 5 ), in older nursing home residents ( 6 ), in peripheral arterial disease, in stroke and thromboembolism, in post-operative complications during catheter ablation for atrial fibrillation and after cardiac surgery, in surgical intensive care unit, in patients undergoing non-bariatric surgery, in type 2 diabetes (reducing amputation risk among non-elderly diabetic men), and in critically ill and osteoporosis patients ( 7 ).
The aim of the present narrative review is to summarize evidence underlying the concept of obesity paradox, focusing on limitations and bias related to this phenomenon, with emphasis on the use of BMI.
Biological Hypotheses and Mechanisms Underlying the Obesity Paradox
Different mechanisms have been postulated to support the existence of obesity paradox.
Body structure and body composition: increased body weight may hinder the metabolic consequences of diseases and of treatments by providing adequate muscle and adipose reserves ( 8 ).
Lipid metabolism: high levels of total cholesterol and lipoproteins may improve the endotoxin-scavenging effect, while patients with CHF and low total serum cholesterol level are more prone to endotoxemia and its inflammatory consequences due to bacterial/endotoxin translocation from bowel wall edema ( 3 ).
The release of N-terminal pro-B-type natriuretic peptide (NT-proBNP) by cardiomyocytes, due to increased wall tension, may be considered as a major prognostic factor for mortality in acute coronary disease. NT-proBNP levels are significantly reduced in patients who are overweight or obese compared to subjects with a lower BMI after myocardial infarction ( 4 ).
Prothrombotic factors (e.g., thromboxane B2) are negatively correlated with BMI and leptin since their production is influenced by endothelial function that is paradoxically better in subjects with obesity than in non-obese individuals ( 4 ).
Increase in ghrelin production/sensitivity has been showed to be a compensatory mechanism to hinder the evolution of heart failure, since it may improve cardiac contractility by increasing left ventricular function and exercise capacity, while it reduces muscle wasting in patients with CHF; ghrelin also affects appetite and can be responsible for a parallel rise in food intake and weight gain ( 9 ).
Cytokines production: cardiometabolic risk is associated with augmented production of cytokines (e.g., tumor necrosis factor TNF-α). The production, by subcutaneous adipose tissue, of soluble TNF-α receptors I and II, which is correlated with BMI and percent body fat, in patients with heart failure, is lower in subjects with obesity. These receptors are supposed to bind TNF-α and to counteract its negative effects on the myocardium ( 7 ). Several adipokines (e.g., adiponectin, apelin, omentin, and others) produced by adipose tissue have shown to be cardioprotective and to exert a variety of favorable effects on cardiovascular function ( 10 ).
Endothelial/vascular aspects: increased mobilization of endothelial progenitor cells may protect patients with severe obesity from atherogenesis through promotion of regeneration processes in the damaged myocardium and the development of new blood vessels. This process leads to the reduction of the afterload due to higher flow-mediated dilation and lower intima-media thickness, to the enhancement of myocardial contractile function and metabolic processes in cardiomyocytes, to the reduction of apoptosis and fibrosis of the myocardium ( 11 ).
Cancer biology: obesity seems to be associated with lower stage disease, smaller tumor size, and less aggressive biological subtypes. Moreover, overweight and obesity may positively influence treatment outcome since excess adipose tissue affects pharmacokinetics of cancer treatment regimens, while providing a nutritional supply to deal with surgical and anticancer treatments ( 12 , 13 ).
Limitations to the Studies Assessing the Presence of the Obesity Paradox
Significant heterogeneity was found across studies supporting the presence of the obesity paradox (e.g., study population, degree of control for confounding factors, length of follow-up), and this has cast doubt on the actual existence of this phenomenon ( 14 , 15 ).
A wide range of normal BMIs (18.5–25.0 kg/m 2 ) may include heterogeneous groups, and mortality rates tend to be significantly higher at the lower end of the BMI range ( 15 ). In a systematic review conducted by Flegal et al. ( 16 ) (97 studies, around 3 million individuals, more than 270,000 deaths), the “obesity paradox” was significantly downsized. All-cause mortality was significantly greater in patients with BMI ≥ 35 kg/m 2 compared to normal weight subjects. Class I obesity (BMI 30- 34.9 kg/m 2 ) was not associated with greater all-cause mortality, and overweight was associated with a significantly lower mortality rate. Moreover, none of the different classes of BMI was associated with mortality in subjects aged 65 years and older. Another systematic review concerning obesity in the elderly ( 17 ) confirmed that obesity represents a mortality risk in older adults, but with different BMI thresholds compared to adult population ( 18 ). A U-shaped-curve correlation between BMI and mortality has been shown with an increased risk of death for low (<18.5 kg/m 2 ) as well as very high BMI values (> 35 kg/m 2 ). But the nadir of the curves differs from what is known in younger obese subjects, and we can hypothesize a shift of the nadir toward a higher BMI (between 23.5 and 27.5 kg/m 2 ) in the elderly, which is at least 1–5 points higher than that in young and middle-aged adults ( 17 ).
Also, a selection bias has been accounted for by different authors ( 3 , 4 , 19 , 20 ). Patients with obesity often present with comorbidities, and they undergo medical check-ups more frequently and consequently, all diseases associated with obesity paradox may have been diagnosed at earlier stages ( 12 ). Subjects with obesity, especially those affected by high levels of comorbidity, are more prone to early death and cannot be included in later cohorts. Thus, the obese population represented in these studies is characterized by obese but likely healthier individuals ( 15 ).
The increased survival of patients with high BMI may also be related to the lack of consideration, in the cohorts with lower BMI, though within the normal BMI range, of subjects with extremely low BMI and of causes explaining low BMI: significant unintentional weight loss due to the presence of high levels of comorbidity (e.g., greater predisposition to develop bleeding and anemia; higher prevalence and severity of hypertension and valvular regurgitation, chronic obstructive pulmonary disease, arrhythmias, infectious diseases) and of the “malnutrition-inflammation complex syndrome” (MICS): in coronary heart disease and dialysis patients, both protein-energy malnutrition and inflammation, or the combination of the two, are more frequent compared to the general population, while different aspects of MICS (e.g., low weight-for-height, hypocholesterolemia, hypocreatininemia) may be considered as risk factors of poor outcome in CHF and dialysis patients ( 20 , 21 ). Comparing subjects with overweight or obesity to subjects belonging to this heterogeneous stratum may lead to a misinterpretation of the correlation between BMI and mortality ( 22 ).
In addition, in some studies, the protective effect of obesity was found in subjects who were significantly younger than their normal-weight counterparts (younger subjects usually have less severe coronary heart disease, a preserved cardiac function and thus better survival rates) ( 23 ) or in elders with overweight or obesity who could be considered “resistant” to negative consequences of higher BMI at younger age ( 6 ). On the other hand, subjects who were normal weight at the time of death could represent a high-risk group for mortality because of unintentional weight loss due to hormonal changes, decreased appetite, and/or chronic undetected medical or mental illness ( 22 ). Finally, some normal-weight subjects may have previously been obese but have lost weight due to illness (reverse causality), hence representing a high-risk of mortality with normal or low BMI being the consequence of a significant illness ( 13 ).
Several studies accounting for the presence of an obesity paradox have an important performance bias, since more appropriate medical treatments were administered to patients with a high BMI than in those with a normal BMI ( 4 , 6 , 7 ), together with an attrition bias ( 3 , 6 ). The median follow-up period of these studies (around 2 years) could have been too short to show negative effects of obesity, while undernutrition may have a greater impact on mortality in a reduced period of time (time discrepancy). In a study conducted by Nigam et al. ( 24 ), comparing three different classes of BMI (<25; 25–29.9; ≥ 30 kg/m 2 ), different mortality risks were described for subjects with overweight or obesity in the short term (<6 months) compared to a longer period of observation. In addition, among the three classes of BMI, they observed that incidence of cardiac-related mortality in the long term was higher in subjects with overweight or obesity than that observed in the normal BMI population. After myocardial infarction, the reduced obesity survival paradox was explained by younger age at the time of initial infarction and by a reduced prevalence of non-cardiovascular comorbidities ( 24 ).
Timing of BMI ascertainment may also significantly influence obesity paradox: different studies considered BMI assessed several years before, whereas other studies, which used BMI calculated at diagnosis or several months to 1–2 years after cancer diagnosis, did not find any association or lower mortality with higher BMI.
Similarly, timing of diagnosis of diseases, such as cardiovascular disease, in patients with obesity may occur earlier than in normal-weight subjects because presence of the obesity, and this can be at the origin of a lead time bias ( 25 ).
Other studies did not control for race/ethnicity or sex, while obesity-mortality association seems to be affected by these variables and obesity paradox is more evident in men than in women ( 26 ).
Finally, confounding factors have not been always considered in those studies. In fact, the adjustment for potential confounders may scale down the protective association of obesity with mortality ( 27 – 30 ). In a study conducted by Hakimi et al. ( 31 ) the association of higher BMI with reduced cancer-specific mortality was lost after adjusting for cancer stage and grade. Confounding by smoking is another major threat to BMI-mortality analysis. Indeed, differences in intensity, inhalation, frequency, and duration of smoking habit, and its association with lower body weight, may represent an important limitation to studies concerning obesity paradox ( 13 , 22 ).
The Case of BMI as a Proxy of Obesity
Although observed associations between obesity and mortality do not prove causality, a major cause of the discrepancy across studies may be related to the use of BMI in the definition of obesity that should consider, instead, excess body fat as the main characteristic of this disease and as the unique determinant of its complications ( 32 ). BMI represents the sum of fat-mass index (FMI) and fat-free mass index (FFMI) ( 33 ). The latter accounts for skeletal muscle mass, bone, and organs, while FMI is composed of peripheral and visceral adipose tissues. All these components of BMI have different roles in contributing to health status, and changes in BMI are not related to a proportional and linear modification of body compartments ( 34 ). For these reasons, different authors have pointed out the limitations of BMI in defining nutritional status ( 35 – 37 ): BMI fails to reflect adiposity and body composition (and their distribution), and to detect “normal weight obese” subjects ( 38 ), patients with sarcopenic obesity ( 39 ), and the presence of undernutrition in overweight subjects ( 40 , 41 ); BMI varies depending on sex (men and women do not have the same body composition at similar levels of BMI) and ethnicity (Asians, Chinese, and Aboriginal people have similar metabolic risk factors at significantly lower—~6 kg/m 2 –BMI values compared to Caucasians) ( 42 ); BMI fails to account for fitness related to the proportion of lean mass to adiposity ( 43 ). Fat-free mass (FFM) is strictly correlated to cardiorespiratory fitness and to physical functional abilities ( 43 ). The correlation between BMI and mortality tends to be modified by the cardiorespiratory fitness status, as it happens in chronic-obstructive pulmonary disease (COPD): the risk of death in unfit men is two-fold higher compared to fit men regardless of obesity status ( 44 ). Caan et al. ( 45 ) have shown that body composition may partially explain the U-shaped association between BMI and cancer (e.g., colorectal cancer) survival. The correlation between BMI and fat mass (FM)—especially in subjects with obesity—is not linear, while the relationship between BMI and FFM tends to be linear. Therefore, higher BMI values are frequently associated with higher FFM (and not necessarily to obesity or to an increase of FM) and cancer patients who are overweight or obese have higher levels of lean mass than their normal-weight counterparts. On the contrary, lower BMI (and lower lean mass) is associated with higher risk of recurrence, surgical complications, treatment-related toxicities, and overall and cancer-specific mortality ( 45 – 48 ).
Similar results were found by Lin et al. ( 49 ) in patients with chronic kidney disease. Using BMI cut-points, 27.9% of patients were obese; while agreeing with the definition based on body fat percentage, the prevalence of obesity raised to 48.8% with a marked percentage of patients (29.4%) who had excess body fat with a normal BMI. When adjusting the regression models for either BMI or body fat percentage, obesity defined by BMI was associated with a significantly lower mortality hazard ratio (HR: 0.23; 95% CI: 0.07–0.71; p = 0.011), whereas the result was inverted when obesity was defined by body fat percentage (HR: 2.75; 95% CI: 1.28–5.89; p = 0.009). Subjects with excess fat mass, irrespective of BMI, were characterized by a reduced lean mass (e.g., sarcopenic obesity) and had higher death risk compared with patients with obesity defined by both BMI and body fat (HR: 5.11; 95% CI: 1.43–18.26; p = 0.012) ( 49 ).
Nonetheless, regardless of body composition, conflicting results emerged when using markers of central obesity in place of BMI. In a systematic review by Coutinho et al. ( 50 ), BMI, waist circumference, and waist-to-hip ratio were compared against mortality outcome in coronary artery disease (CAD) patients. Interestingly, central obesity was positively associated with higher mortality in individuals with CAD, whereas BMI was inversely associated with mortality. The effect of central obesity on mortality was observed even in patients with normal BMI ( 50 ).
Use of body composition analysis is indeed an attempt to overcome the misleading properties of BMI: in an elegant study by Gonzalez et al. ( 48 ), obesity paradox was explored in cancer patients using either BMI or body composition obtained by bioimpedance analysis, indicating that obesity paradox emerged when using BMI, but it was not confirmed by analyses based on body composition. Though just a minority of studies investigating the obesity paradox relied on body composition assessment, evidence supports the role of low lean mass as the actual predictor of mortality when used in place of BMI ( 51 ).
The actual paradox seems to be keeping defining obesity using BMI, which is not able to quantify body fat percentage and adiposity distribution, nor the degree of metabolic disturbances that it can underlie. In fact, obesity is characterized by a significant complexity related to alterations of nutritional status (energy and nutrient intake, body composition), to the interaction of psychological and social factors, to functional impairment, to hormonal and metabolic alterations, to the impairment of different organs (e.g., cardiovascular and respiratory systems) and quality of life that cannot be adequately described by BMI.
However, replacing BMI by body composition is not an easy fix for the issue of the obesity paradox: some of the above mentioned biases reported in previous studies would still apply to obesity even when defined by body composition methods, such as reverse causation and selection bias of healthy obese subjects or resilient survivors. In addition, no universal cut-points have been yet defined to classify obesity based on body fat that are accurate by sex, ethnicity, age, or physiological groups (e.g., post-menopausal women).
Body composition phenotypes, taking into account both body fat and lean mass, and metabolic and functional variables, and duration of obesity (as well as of normal weight), can capture properly the trajectories of mortality in a wealth of diseases. Further research should be prompted in order to promote correct phenotyping of patients. The obesity paradox is just a lesson to be learned.
Author Contributions
LD led the study design, was actively involved in the study conception, design, strategic decisions, and drafted the manuscript. AL, AP, AG, and EP contributed to the analysis of the literature, interpreted the findings, and helped in drafting the manuscript. AL and EP participated in the study design and coordination and gave intellectual inputs on the manuscript. All authors have read and approved the manuscript.
Conflict of Interest
The authors declare that the research was conducted in the absence of any commercial or financial relationships that could be construed as a potential conflict of interest.
1. Gruberg L, Weissman NJ, Waksman R, Fuchs S, Deible R, Pinnow EE, et al. The impact of obesity on the short-term and long-term outcomes after percutaneous coronary intervention: the obesity paradox? J Am Coll Cardiol . (2002) 39:578–84. doi: 10.1016/S0735-1097(01)01802-2
PubMed Abstract | CrossRef Full Text | Google Scholar
2. Kalantar-Zadeh K, Block G, Humphreys MH, Kopple JD. Reverse epidemiology of cardiovascular risk factors in maintenance dialysis patients. Kidney Int . (2003) 63:793–808. doi: 10.1046/j.1523-1755.2003.00803.x
3. Kalantar-Zadeh K, Block G, Horwich T, Fonarow GC. Reverse epidemiology of conventional cardiovascular risk factors in patients with chronic heart failure. J Am Coll Cardiol . (2004) 43:1439–44. doi: 10.1016/j.jacc.2003.11.039
4. Wang L, Liu W, He X, Chen Y, Lu J, Liu K, et al. Association of overweight and obesity with patient mortality after acute myocardial infarction: a meta-analysis of prospective studies. Int J Obes . (2016) 40:220–8. doi: 10.1038/ijo.2015.176
5. Cao C, Wang R, Wang J, Bunjhoo H, Xu Y, Xiong W. Body mass index and mortality in chronic obstructive pulmonary disease: a meta-analysis. PLoS ONE . (2012) 7:e43892. doi: 10.1371/journal.pone.0043892
6. Veronese N, Cereda E, Solmi M, Fowler SA, Manzato E, Maggi S, et al. Inverse relationship between body mass index and mortality in older nursing home residents: a meta-analysis of 19,538 elderly subjects. Obes Rev . (2015) 16:1001–15. doi: 10.1111/obr.12309
7. Hainer V, Aldhoon-Hainerová I. Obesity paradox does exist. Diabetes Care . (2013) 36(Suppl. 2):S276–81. doi: 10.2337/dcS13-2023
8. Casas-Vara A, Santolaria F, Fernandez-Bereciartua A, González-Reimers E, García-Ochoa A, Martínez-Riera A, et al. The obesity paradox in elderly patients with heart failure: analysis of nutritional status. Nutrition . (2012) 28:616–22. doi: 10.1016/j.nut.2011.10.006
9. Khatib MN, Simkhada P, Gode D. Cardioprotective effects of ghrelin in heart failure: from gut to heart. Heart Views . (2014) 15:74–76. doi: 10.4103/1995-705X.144792
10. Mattu HS, Randeva HS. Role of adipokines in cardiovascular disease. J Endocrinol. (2013) 216:T17–36. doi: 10.1530/JOE-12-0232
11. Biasucci LM, Graziani F, Rizzello V, Liuzzo G, Guidone C, De Caterina AR, et al. Paradoxical preservation of vascular function in severe obesity. Am J Med . (2010) 123:727–34. doi: 10.1016/j.amjmed.2010.02.016
12. Trestini I, Carbognin L, Bonaiuto C, Tortora G, Bria E. The obesity paradox in cancer: clinical insights and perspectives. Eating Weight Disord . (2018) 23:185–93. doi: 10.1007/s40519-018-0489-y
13. Lennon H, Sperrin M, Badrick E, Renehan AG. The obesity paradox in cancer: a review. Curr Oncol Rep . (2016) 18:56. doi: 10.1007/s11912-016-0539-4
14. Goyal A, Nimmakayala KR, Zonszein J. Is there a paradox in obesity? Cardiol Rev . (2014) 22:163–70. doi: 10.1097/CRD.0000000000000004
15. Antanopoulos AS, Oikonomou EK, Antoniades C, Tousoulis D. From the BMI paradox to the obesity paradox: the obesity–mortality association in coronary heart disease. Obes Rev. (2016) 17:989–1000. doi: 10.1111/obr.12440
CrossRef Full Text | Google Scholar
16. Flegal KM, Ioannidis JPA. The obesity paradox: a misleading term that should be abandoned. Obesity . (2018) 26:629–30. doi: 10.1002/oby.22140
17. Donini LM, Savina C, Gennaro E, De Felice MR, Rosano A, Pandolfo MM, et al. A systematic review of the literature concerning the relationship between obesity and mortality in the elderly. J Nutr Health Aging . (2012) 16:89–98. doi: 10.1007/s12603-011-0073-x
18. Batsis JA, Mackenzie TA, Bartels SJ, Sahakyan KR, Somers VK, Lopez-Jimenez F. Diagnostic accuracy of body mass index to identify obesity in older adults: NHANES 1999-2004. Int J Obes . (2016) 40:761–7. doi: 10.1038/ijo.2015.243
19. Von Haehling S. The metabolic basis for the obesity paradox in heart failure. Heart Metab . (2013) 61:4–7.
Google Scholar
20. Charnigo R, Guglin M. Obesity paradox in heart failure: statistical artifact, or impetus to rethink clinical practice? Heart Fail Rev . (2017) 22:13–23. doi: 10.1007/s10741-016-9577-0
21. Anand N, Chandrasekaran SC, Alam MN. The malnutrition inflammation complex syndrome-the micsing factor in the perio-chronic kidney disease interlink. J Clin Diagn Res . (2013) 7:763–7. doi: 10.7860/JCDR/2013/5329.2907
22. Tobias DK, Hu FB. Does being overweight really reduce mortality? Obesity . (2013) 21:1746–9. doi: 10.1002/oby.20602
23. Lavie CJ, Pandey A, Lau DH, Alpert MA, Sanders P. Obesity and atrial fibrillation prevalence, pathogenesis, and prognosis: effects of weight loss and exercise. J Am Coll Cardiol . (2017) 70:2022–35. doi: 10.1016/j.jacc.2017.09.002
24. Nigam A, Wright RS, Allison TG, Williams BA, Kopecky SL, Reeder GS, et al. Excess weight at time of presentation of myocardial infarction is associated with lower initial mortality risks but higher long-term risks including recurrent re-infarction and cardiac death. Int J Cardiol . (2006) 110:153–9. doi: 10.1016/j.ijcard.2005.06.040
25. De Schutter A, Lavie CJ, Milani RV. The impact of obesity on risk factors and prevalence and prognosis of coronary heart disease-the obesity paradox. Prog Cardiovasc Dis . (2014) 56:401–8. doi: 10.1016/j.pcad.2013.08.003
26. Park Y, Peterson LL, Colditz GA. The plausibility of obesity paradox in cancer. Cancer Res . (2018) 78:1898–903. doi: 10.1158/0008-5472.CAN-17-3043
27. Kistorp C, Faber J, Galatius S, Gustafsson F, Frystyk J, Flyvbjerg A, et al. Plasma adiponectin, body mass index, and mortality in patients with chronic heart failure. Circulation . (2005) 112:1756–62. doi: 10.1161/CIRCULATIONAHA.104.530972
28. Cicoira M, Maggioni AP, Latini R, Barlera S, Carretta E, Janosi A, et al. Body mass index, prognosis and mode of death in chronic heart failure: results from the Valsartan Heart Failure Trial. Eur J Heart Fail . (2007) 9:397–402. doi: 10.1016/j.ejheart.2006.10.016
29. Frankenstein L, Zugck C, Nelles M, Schellberg D, Katus HA, Remppis BA. The obesity paradox in stable chronic heart failure does not persist after matching for indicators of disease severity and confounders. Eur J Heart Fail . (2009) 11:1189–94. doi: 10.1093/eurjhf/hfp150
30. Güder G, Gelbrich G, Edelmann F, Wachter R, Pieske B, Pankuweit S, et al. Competence network heart failure germany. Reverse epidemiology in different stages of heart failure. Int J Cardiol . (2015) 184:216–24. doi: 10.1016/j.ijcard.2015.02.009
31. Hakimi AA, Furberg H, Zabor EC, Jacobsen A, Schultz N, Ciriello G, et al. An epidemiologic and genomic investigation into the obesity paradox in renal cell carcinoma. J Natl Cancer Inst . (2013) 105:1862–70. doi: 10.1093/jnci/djt310
32. Villareal DT, Apovian CM, Kushner RF, Klein S American Society for Nutrition; NAASO The Obesity Society. Obesity in older adults: technical review and position statement of the American Society for Nutrition and NAASO, The Obesity Society. Obes Res . (2005) 13:1849–63. doi: 10.1038/oby.2005.228
33. Dulloo AG, Jacquet J, Solinas G, Montani JP, Schutz Y. Body composition phenotypes in pathways to obesity and the metabolic syndrome. Int J Obes . (2010) 34(Suppl. 2):S4–17. doi: 10.1038/ijo.2010.234
34. Rothman KJ. BMI-related errors in the measurement of obesity. Int J Obes . (2008) 32(Suppl. 3):S56–9. doi: 10.1038/ijo.2008.87
35. Pories WJ, Dohm LG, Mansfield CJ. Beyond the BMI: the search for better guidelines for bariatric surgery. Obesity . (2010) 18:865–71. doi: 10.1038/oby.2010.8
36. Mascie-Taylor CG, Goto R. Human variation and body mass index: a review of the universality of BMI cut-offs, gender and urban-rural differences, and secular changes. J Physiol Anthropol . (2007) 26:109–12. doi: 10.2114/jpa2.26.109
37. Stevens J, Cai J, Pamuk ER, Williamson DF, Thun MJ, Wood JL. The effect of age on the association between body-mass index and mortality. N Engl J Med . (1998) 338:1–7. doi: 10.1056/NEJM199801013380101
38. Di Renzo L, Del Gobbo V, Bigioni M, Premrov MG, Cianci R, De Lorenzo A. Body composition analyses in normal weight obese women. Eur Rev Med Pharmacol Sci . (2006) 10:191–6.
PubMed Abstract | Google Scholar
39. Poggiogalle E, Migliaccio S, Lenzi A, Donini LM. Treatment of body composition changes in obese and overweight older adults: insight into the phenotype of sarcopenic obesity. Endocrine . (2014) 47:699–716. doi: 10.1007/s12020-014-0315-x
40. Kaidar-Person O, Person B, Szomstein S, Rosenthal RJ. Nutritional deficiencies in morbidly obese patients: a new form of malnutrition? Part B: minerals. Obes Surg . (2008) 18:1028–34. doi: 10.1007/s11695-007-9350-5
41. Kaidar-Person O, Person B, Szomstein S, Rosenthal RJ. Nutritional deficiencies in morbidly obese patients: a new form of malnutrition? Part A: vitamins. Obes Surg . (2008) 18:870–6. doi: 10.1007/s11695-007-9349-y
42. Razak F, Anand SS, Shannon H, Vuksan V, Davis B, Jacobs R, et al. Defining obesity cut points in a multiethnic population. Circulation . (2007) 115:2111–8. doi: 10.1161/CIRCULATIONAHA.106.635011
43. Yanek LR, Vaidya D, Kral BG, Dobrosielski DA, Moy TF, Stewart KJ, et al. Lean mass and fat mass as contributors to physical fitness in an overweight and obese African American Population. Ethn Dis . (2015) 25:214–9.
44. Spelta F, Fratta Pasini AM, Cazzoletti L, Ferrari M. Body weight and mortality in COPD: focus on the obesity paradox. Eat Weight Disord. (2018) 23:15–22. doi: 10.1007/s40519-017-0456-z
45. Caan BJ, Meyerhardt JA, Kroenke CM, Alexeeff S, Xiao J, Weltzien E, et al. Explaining the obesity paradox: The association between body composition and colorectal cancer survival (C-SCANS study). Cancer Epidemiol Biomarkers Prev . (2017) 26:1008–15. doi: 10.1158/1055-9965.EPI-17-0200
46. Caan BJ, Feliciano EMC, Kroenker CH, Prado CM. The Importance of body composition in explaining the overweight paradox in cancer. Cancer Res . (2018) 78:1906–12. doi: 10.1158/0008-5472.CAN-17-3287
47. Schachar SS, Williams GR. The obesity paradox in cancer – moving beyond BMI. Cancer Epidemiol Biomarkers Prev . (2017) 26:13–6. doi: 10.1158/1055-9965.EPI-16-0439
48. Gonzalez MC, Pastore CA, Orlandi SP, Heymsfield SB. Obesity paradox in cancer: new insights provided by body composition. Am J Clin Nutr . (2014) 99:999–1005. doi: 10.3945/ajcn.113.071399
49. Lin TY, Lim PS, Hung SC. Impact of misclassification of obesity by body mass index on mortality in patients with CKD. Kidney Int Rep . (2017) 3:447–55. doi: 10.1016/j.ekir.2017.12.009
50. Coutinho T, Goel K, Corrêa de Sá D, Kragelund C, Kanaya AM, Zeller M, et al. Central obesity and survival in subjects with coronary artery disease: a systematic review of the literature and collaborative analysis with individual subject data. J Am Coll Cardiol . (2011) 57:1877–86. doi: 10.1016/j.jacc.2010.11.058
51. Prado CM, Gonzalez MC, Heymsfield SB. Body composition phenotypes and obesity paradox. Curr Opin Clin Nutr Metab Care . (2015) 18:535–51. doi: 10.1097/MCO.0000000000000216
Keywords: obesity, obesity paradox, nutritional status, body composition, body mass index
Citation: Donini LM, Pinto A, Giusti AM, Lenzi A and Poggiogalle E (2020) Obesity or BMI Paradox? Beneath the Tip of the Iceberg. Front. Nutr. 7:53. doi: 10.3389/fnut.2020.00053
Received: 07 January 2020; Accepted: 30 March 2020; Published: 07 May 2020.
Reviewed by:
Copyright © 2020 Donini, Pinto, Giusti, Lenzi and Poggiogalle. This is an open-access article distributed under the terms of the Creative Commons Attribution License (CC BY) . The use, distribution or reproduction in other forums is permitted, provided the original author(s) and the copyright owner(s) are credited and that the original publication in this journal is cited, in accordance with accepted academic practice. No use, distribution or reproduction is permitted which does not comply with these terms.
*Correspondence: Lorenzo Maria Donini, lorenzomaria.donini@uniroma1.it
This article is part of the Research Topic
Analyzing the Relationship Between Dietary Patterns, Health Outcomes and Individual Food Choices
Europe PMC requires Javascript to function effectively.
Either your web browser doesn't support Javascript or it is currently turned off. In the latter case, please turn on Javascript support in your web browser and reload this page.
Search life-sciences literature (44,013,255 articles, preprints and more)
- Free full text
- Citations & impact
- Similar Articles
The Obesity Paradox in Cancer: a Review.
Author information, affiliations.
- Lennon H 1, 2
- Badrick E 1, 2
- Renehan AG 1, 2
- Sperrin M 2
ORCIDs linked to this article
- Sperrin M | 0000-0002-5351-9960
Current Oncology Reports , 01 Sep 2016 , 18(9): 56 https://doi.org/10.1007/s11912-016-0539-4 PMID: 27475805 PMCID: PMC4967417
Abstract
Free full text , the obesity paradox in cancer: a review, hannah lennon.
1 Institute of Cancer Sciences, University of Manchester, Manchester, UK
2 Farr Institute, MRC Health eResearch Centre (HeRC North), University of Manchester, Manchester, UK
Matthew Sperrin
Ellena badrick, andrew g. renehan.
There is a common perception that excess adiposity, commonly approximated by body mass index (BMI), is associated with reduced cancer survival. A number of studies have emerged challenging this by demonstrating that overweight and early obese states are associated with improved survival. This finding is termed the “obesity paradox” and is well recognized in the cardio-metabolic literature but less so in oncology. Here, we summarize the epidemiological findings related to the obesity paradox in cancer. Our review highlights that many observations of the obesity paradox in cancer reflect methodological mechanisms including the crudeness of BMI as an obesity measure, confounding, detection bias, reverse causality, and a specific form of the selection bias, known as collider bias. It is imperative for the oncologist to interpret the observation of the obesity paradox against the above methodological framework and avoid the misinterpretation that being obese might be “good” or “protective” for cancer patients.
- Introduction
Excess body adiposity is a major global public health problem, with 67 % of the US, 63 % of the UK, and 64 % of Australia’s population being classified as overweight or obese, by body mass index (BMI) criteria, in 2014 [ 1 ]. A report from the World Cancer Research Fund (WCRF) [ 2 ], and a systematic review with standardized meta-analysis from one of the present authors [ 3 ], established, approximately a decade ago, that elevated BMI is associated with increased cancer incidence for several common adult cancer types. There are now ten established obesity-related cancers listed by the WCRF, including post-menopausal breast, endometrial, ovarian, advanced prostate, colorectal, renal, pancreatic, liver, and gallbladder cancers and esophageal adenocarcinoma. There is a common perception that, compared with normal-weight patients, elevated BMI is also associated with poorer prognosis after cancer diagnosis. This certainly is observed in systematic reviews of the literature among women with breast cancer [ 4 ] and forms a key rationale for weight management recommendations among cancer survivors, endorsed by clinical guidelines, for example, by the American Society of Clinical Oncology [ 5 ], with similar recommendations from the American Cancer Society [ 6 ] and European Society for Medical Oncology [ 7 ].
However, a number of isolated historic studies [ 8 – 10 ] and an emerging number of recent studies [ 11 – 15 ] have observed that among patients with cancer, elevated BMI is associated with improved survival compared with normal-weight patients. The surprising nature of this finding suggests the existence of an “obesity paradox”. This phenomenon is well described in the cardiovascular and metabolic literature [ 16 – 21 ] but less well appreciated in oncology. The repeated observation of the obesity paradox has spawned research that attempts to explain its occurrence. Posited explanations range from methodological (observed associations that contradict underlying causality due to confounding and bias) to clinical (seeking mechanistic explanations for obesity acting protectively in specific populations). In this review, we first explain what the obesity paradox is; summarize the current epidemiological findings for the association between overweight or obese status at cancer diagnosis and subsequent survival; review clinical and methodological explanations for the obesity paradox; and conclude with clinical implications and recommendations for further research.
- What Is the Obesity Paradox?
A BMI of 22.5 kg/m 2 has been widely accepted as a mid-reference point for normal weight [ 22 ]. The obesity paradox occurs where the risk of outcome, typically mortality, is significantly reduced for BMI values above this referent, where an increased risk is expected. At very high BMI values, risk either returns to unity or is increased as illustrated in Fig. 1 .

An illustration of the obesity paradox. The vertical axis represents hazard ratio of mortality (log scale), compared with the baseline BMI of 22.5 kg/m 2 . The plot represents a population in which the obesity paradox is observed, since the hazard ratio is below 1 in the overweight and obese range. The 95 % confidence intervals are shown with dashed lines
- Epidemiological Evidence
There have been mixed findings in incident cancer populations where there has been exploration for the obesity paradox, with the paradox being observed in some studies [ 8 – 11 , 13 – 15 , 23 ], but not in all [ 24 – 26 ]. Consequently, there have been attempts to unify the conflicting results in the literature with systematic reviews on adiposity and cancer survival [ 27 – 30 ] but with inconsistent summaries.
The obesity paradox has been observed in different cancer settings including, for example, in patients with colorectal cancer undergoing surgery [ 11 ]; patients with renal cancer undergoing surgery [ 10 , 12 ]; patients with colorectal metastases undergoing liver resection [ 13 ]; elderly patients with acute myeloid leukemia [ 14 ]; and patients with lymphoma undergoing autologous hematopoietic cell transplantation [ 9 ]. The obesity paradox is not limited to non-metastatic disease and has been observed in a study of 4010 Taiwanese patients where the most common metastases were the lungs, liver, brain, and bone, requiring radiotherapy [ 15 ], and the hazard ratios decreased across BMI categories (overweight: HR 0.84 and obese: HR 0.67).
- General Points on Interpretation
Given the variations in study findings, there is a need to have an initial framework to interpret whether the obesity paradox is a true or artificial association. There are two broad principles to consider in the study characteristics: (i) when (in relation to cancer diagnosis) BMI was determined and (ii) the age of the participants under study.
When BMI was determined is relevant. The recent WCRF report on the effect of risk factors on survival among women with breast cancer added a very useful classification—namely, determination of BMI either at pre-, peri-, or post-diagnosis (the later typically 12 months after the initial treatment) of cancer [ 31 ]. From these, different patterns of associations emerge. In a meta-analysis of 29 studies evaluating the impact of BMI on survival in patients with colorectal cancer, Wu et al. [ 30 ] observed that increasing pre-diagnosis BMI prognosticated for a poor survival but that post-treatment overweight was associated with improved survival, i.e., the obesity paradox. Table Table1 1 demonstrates that the obesity paradox can be illustrated in all three settings of pre- [ 32 ], peri- [ 15 ], and post-diagnosis [ 11 ] for different cancer types.
Examples of studies demonstrating the obesity paradox in patients with cancer, where BMI was determined either pre-, peri-, or post-diagnosis of cancer
BMI body mass index, EQD equieffective dose (of radiotherapy), CRC colorectal cancer
Age is an additional attribute for consideration. For example, studies involving patients with leukemia are challenging to interpret due to the great age ranges of included individuals. Navarro and colleagues [ 33 ] showed that in over 4000 adults with acute myeloid leukemia under marrow transplantation, the obesity paradox was absent in young patients but present in those over age 60. Similar findings were noted by Brunner and colleagues, in a treatment cohort of adults with AML aged greater than 60 years [ 14 ].
- Explanations for the Obesity Paradox
Determining whether the obesity paradox is a causal phenomenon among patients with cancer is clinically relevant, as it informs weight management strategies among cancer survivors. There are many potential causes of the obesity paradox, and understanding these is central to clinical implications. These are grouped into two broad categories [ 16 ]: the first is methodological and reflects spurious or artificial associations; the second is clinical and potentially reflects true associations and is clinically useful.
Methodological Explanations
Bmi as an inadequate measure of adiposity.
BMI is commonly used as an approximation of general body adiposity in studies that have observed the obesity paradox. BMI is appealing as it is routinely measured in primary care and hospital settings and there are well-defined criteria for normal, overweight, and obese categories. However, BMI is a relatively crude measure of body adiposity and body composition and does not differentiate between lean mass and fat mass. In turn, body composition varies with age, sex, and ethnicity [ 22 ], such that there are currently no specific age-gender-ethnicity indices to define obesity in a standardized manner. Thus, for example, in a cancer population, overweight individuals (defined by BMI) might be younger with high muscle mass (compared with normal weight), explaining their better outcome compared with normal weight.
The paradox might not exist if alternate measures of body composition or adipose tissue were used. Thus, for example, we found no examples of studies in patients with cancer demonstrating the obesity paradox when anthropometric measures other than BMI or body composition indices were used. Alternate indices include measurements such as waist circumference, waist to hip ratio, skinfold, and body composition assessment techniques such as dual-energy X-ray absorptiometry, CT, and MRI, and quantify different body fat components such as subcutaneous adipose tissue (SAT) and visceral adipose tissue (VAT) [ 34 , 35 ]. Gonzalez and colleagues [ 36 ] recently explored this hypothesis and showed that the obesity paradox was present in 175 patients with various cancers (breast, gynecological, head and neck, lung, and gastrointestinal) when BMI was the exposure of interest but disappeared when obesity was defined using fat mass index and fat-free mass index.
Confounding
Confounding occurs when there are variables that are associated with both the outcome (death) and the exposure (obesity) and are not on the causal pathway between them. A common example is smoking, where BMI values are generally lower in current smokers than in never smokers. Other examples include deprivation, socioeconomic status, physical activity, and diet. It is difficult to adjust for all confounding factors, as many are unobserved. Measurement error may lead to incomplete removal of confounding, and to avoid this in the smoking context, some studies exclude smokers from their analysis [ 37 ], but usually, this is at the cost of disregarding a large proportion of the sample. Another approach would be to quantify exposure more accurately, for example, in terms of smoking duration and intensity using pack-years variables or cumulative lifetime exposure.
Selection Bias/Collider Stratification Bias
The obesity paradox might be due to a specific form of selection bias, known as the collider stratification bias, caused during the statistical analysis due to conditioning on a subpopulation selected based on a collider variable. In turn, a collider variable is one with at least two causes common to the risk of the variable and the outcome of interest. For example, cancer incidence is a collider variable because it is “caused” by both obesity and other risk factors (e.g., smoking). There is a well-recognized inverse relationship between BMI and smoking. Thus, cancer patients who are not obese are more likely to have other risk factors, such as smoking, and in the analyzed subpopulation, an inverse association is artificially generated (or strengthened) between obesity and the other risk factors. Additionally, Banack and Kaufman [ 38 ••] demonstrate how confounding due to smoking is increased in the presence of collider stratification bias. In a contrary direction, Sperrin et al. [ 39 ••], using an equation-derived approach within a counterfactual framework, show that the biases attributable to collider stratification are small and cannot explain the large paradoxical relationships seen in epidemiological studies.
Detection Bias
A further dimension is detection bias. This is the co-occurrence of two diagnoses together. Thus, for example, being overweight and obese is associated with the development of diabetes and cardiovascular disease. Where patients present with new diagnoses of these conditions, they undergo several investigations, which in turn detect incidental diseases including silent cancers—a form of “opportunistic surveillance”. This overestimation of the occurrence of cancer diagnosis concurrent with a new diagnosis of diabetes is well recognized [ 40 ]. These silent cancers might have a low-stage disease with generally good prognosis and account for the obesity paradox in overweight and obese patients. One approach to minimize detection bias is to adjust for tumor stage at presentation or alternatively where the date of diagnosis of, say, diabetes is known, use a washout of 2 years to “bypass” the detection bias.
Reverse Causality
Reverse causality refers to the phenomenon that some normal-weight patients may have previously been obese but lost weight due to illness, here cancer. Cancer is known to cause weight loss by loss of appetite or increased metabolic demands. The extent of weight loss correlates with initial BMI and occurs in patients with early-stage as well as late-stage tumors [ 41 ]. The potential causal link of reverse causality with the obesity paradox has been shown in several examples. Thus, Gelber and colleagues [ 42 ] evaluated the relationship between BMI and mortality in 99,253 male physicians in the Physicians’ Health Study and initially showed a U-shaped association with all-cause mortality, which converted to a linear relationship in their optimal model excluding men who died within 2 years of initial assessment. Similarly, Tseng [ 43 ] evaluated a nationally representative cohort of 89,056 Taiwanese patients with type 2 diabetes, matched with the National Death Certificate Database, and on initial analysis found that BMI was inversely associated with mortality from all-cause, cancer, and diabetes complications, but after excluding patients with a follow-up duration less than 2 years, BMI categories were not significantly prognostic for cancer-related mortality, suggesting a bias induced by cancer-induced weight loss.
Reverse causality is better explored and minimized by using longitudinal data to obtain a description of the patient’s usual weight and its trajectory up to cancer diagnosis. Weight histories (repeated weight measurements) give a useful dimension of obesity exposure, but there is a paucity of such data. Research to define obesity-equivalence of pack-years will be informative. A simpler approach when weight histories are not available is to include an individual’s maximum lifetime BMI and is robust to confounding by illness-induced weight loss. Stokes and Preston [ 37 ] have illustrated this approach demonstrating a reduction in biases when including maximum lifetime BMI into their models. This approach is similar to evaluating weight change, for example, the rate of change and size of variability over time [ 28 , 44 ].
Clinical Explanations
Tumor biology is less aggressive.
There are some examples where tumors among obese patients have less aggressive characteristics compared with those among normal-weight patients. In obese women with endometrial cancer, there is a predominance of good prognosis type 1 tumors compared with poor prognosis type 2 endometrial cancer. Tumors are molecularly heterogeneous, and it is speculated that obesity is associated with less aggressive biological subtypes. For renal carcinoma, obesity is associated with more indolent molecular variants (for example, reduced fatty acid synthase, FASN , gene expression) [ 12 ], while in contrast, for ovarian cancer, elevated BMI is associated with good prognosis cancers (low-grade serous and endometrioid), but within this histological type subpopulation, there is a linear positive association between BMI and mortality, which is absent in high-grade serous ovarian cancers [ 45 ].
Tumors Respond Better in Obese Patients
The overweight and obese state might influence treatment outcomes, both in terms of how the tumor (changed for consistency) behaves to treatment and in terms of the differential pharmacokinetics of cancer treatment regimens. For example, high-intra-abdominal fat volume predicts for greater doxorubicin exposure and hematologic toxicities in women with breast cancer compared with body surface area [ 46 ]. Similarly, overweight and obese patients might be differentially allocated to less radical cancer surgery, though Gurunathan and Myles [ 47 ] point out the limitations of BMI as a predictor of peri-operative complication risk and indicate that mildly obese and overweight patients outperform normal-weight patients after many types of surgeries.
A specific mention is worthwhile for the complex inter-relationships between adjuvant chemotherapy (after curative resection) and the overweight/obese state. There is a well-recognized clinical practice among many oncologists to dose cap chemotherapy in obese patients with body surface area (BSA) greater than 2.0, and together with differential allocation and differential adherence to adjuvant chemotherapy, obese patients may simply have poorer outcomes compared with normal-weight patients because they are sub-optimally treated. This is illustrated by Sinicrope and colleagues [ 48 ], who examined the prognostic impact of BMI in 25,291 patients with stage II and III colon carcinoma within the Adjuvant Colon Cancer Endpoints (ACCENT) database, a consortium of randomized trials of 5-fluorouracil-based adjuvant chemotherapy. With disease-free survival (DFS) as a key outcome measure, compared with normal-weight patients, they showed a significant reduction in DFS limited to men with class 2 and 3 obesity (BMI ≥35.0 kg/m 2 ) but an improved survival for overweight and class 1 obese (BMI 25.0 to 34.9 kg/m 2 ) men, i.e., the obesity paradox. There were different dose-capping practices among the trials, and there was lack of data on chemotherapy adherence by BMI status, such that the study was unable to conclude whether or not these confounders contributed to the differential impact of BMI states on survival.
Energy Reserve or Hibernation Hypothesis
A third hypothesis is that excess adipose tissue serves as a nutrient reserve and confers a survival advantage in times of stress, such as anti-cancer treatment. This is akin to the hibernation theories in evolutional biology whereby species store up energy in anticipation of harsh times ahead. On a parallel note, it remains unclear if obesity drives cancer progression, whether it is due to excess adiposity or the energy imbalance [ 49 ].
- Conclusions and Future Directions
This review has highlighted the mixed findings in studies evaluating the obesity paradox in cancer populations. In terms of interpreting these studies, and designing future studies on this topic, there is a need to apply a methodological framework to determine whether the obesity paradox is a true or spurious relationship for a given setting. If a framework is not used, mistaken interpretations can be reached. Thus, in the cardiovascular literature, some commentators have concluded that the obesity paradox is a true causal association arguing that the optimum body weight is above the normal BMI range in individuals with some chronic diseases [ 17 ].
First, where the primary interest is the effect of obesity on survival, it is preferred to incorporate as much information regarding the patients’ weight history, i.e., consider the patient’s BMI trajectory throughout a long period of time or ideally through the whole life-course. Variations on this include modeling BMI at pre-, peri-, and post-diagnosis of cancer. An alternative is to use maximum lifetime weight and weight variability measures.
Second, it is important to work within datasets with richness for potential confounding. The following are potential effect modifiers or confounders of the relationship between BMI and survival but are not always captured: smoking, hormonal replacement therapy, and ethnicity. Cancers diagnosed through screening programs have better prognosis than non-screened cancer, and in turn, obesity tends to be associated with lower uptake rates in cancer screening.
Third, conflicting findings may be partly explained by heterogeneity within cancer types, the timing of when BMI was determined, unmeasured confounders, and statistical biases. To further understand the observed associations, directions for future research include (i) improving the “subtyping” of cancer by better recording of staging, tumor type; (ii) improving data linkage so BMI, adiposity measures, and confounding variables can readily be extracted from records and incorporated; and (iii) further research into the gender-specific links of the effect of obesity and overweight on survival in cancer populations.
It is imperative for the oncologist to interpret the observation of the obesity paradox against the above methodological framework and avoid the misinterpretation that being obese might be “good” or “protective” for cancer patients. ‘First, do no harm’.
- Acknowledgments
This study was partly supported by the University of Manchester’s Health eResearch Centre (HeRC) funded by the Medical Research Council (MRC) Grant MR/K006665/1 and the National Awareness and Early Detection Initiative funding scheme from Cancer Research UK Grant A17962.
- Compliance with Ethical Standards
- Conflict of Interest
Hannah Lennon, Matthew Sperrin, Ellena Badrick, and Andrew G. Renehan declare that they have no conflict of interest.
- Human and Animal Rights and Informed Consent
This article does not contain any studies with human or animal subjects performed by any of the authors.
This article is part of the Topical Collection on Integrative Care
- Papers of particular interest, published recently, have been highlighted as: •• Of major importance
Full text links
Read article at publisher's site: https://doi.org/10.1007/s11912-016-0539-4
Citations & impact
Impact metrics, citations of article over time, alternative metrics.

Smart citations by scite.ai Smart citations by scite.ai include citation statements extracted from the full text of the citing article. The number of the statements may be higher than the number of citations provided by EuropePMC if one paper cites another multiple times or lower if scite has not yet processed some of the citing articles. Explore citation contexts and check if this article has been supported or disputed. https://scite.ai/reports/10.1007/s11912-016-0539-4
Article citations, longitudinal bmi change and outcomes in chronic obstructive pulmonary disease: a nationwide population-based cohort study..
Kim T , Shin SH , Kim H , Im Y , Cho J , Kang D , Park HY
Respir Res , 25(1):150, 30 Mar 2024
Cited by: 0 articles | PMID: 38555459 | PMCID: PMC10981805
Clinical variables associated with immune checkpoint inhibitor outcomes in patients with metastatic urothelial carcinoma: a multicentre retrospective cohort study.
Labidi S , Meti N , Barua R , Li M , Riromar J , Jiang DM , Fallah-Rad N , Sridhar SS , Del Rincon SV , Pezo RC , Ferrario C , Cheng S , Sacher AG , Rose AAN
BMJ Open , 14(3):e081480, 29 Mar 2024
Cited by: 0 articles | PMID: 38553056 | PMCID: PMC10982788
Obesity paradox in uveal melanoma: high body mass index is associated with low metastatic risk.
Sabazade S , Opalko A , Herrspiegel C , Gill VT , Plastino F , André H , Stålhammar G
Br J Ophthalmol , 108(4):578-587, 20 Mar 2024
Cited by: 0 articles | PMID: 37028917 | PMCID: PMC10958277
A lack of association between BMI and chemoimmunotherapy efficacy in advanced non-small cell lung cancer: Secondary analysis of the IMpower150 and IMpower130 clinical trials.
Li LX , Socinski MA , Kichenadasse G , Karapetis CS , Shahnam A , McKinnon RA , Rowland A , Hopkins AM , Sorich MJ
BMC Cancer , 24(1):379, 25 Mar 2024
Cited by: 0 articles | PMID: 38528478 | PMCID: PMC10964615
Body Composition and Clinical Outcomes in Esophageal Cancer Patients Treated with Immune Checkpoint Inhibitors.
Kosumi K , Baba Y , Hara Y , Wang H , Nomoto D , Toihata T , Ohuchi M , Harada K , Eto K , Ogawa K , Ishimoto T , Iwatsuki M , Iwagami S , Miyamoto Y , Yoshida N , Baba H
Ann Surg Oncol , 29 Feb 2024
Cited by: 0 articles | PMID: 38421531
Similar Articles
To arrive at the top five similar articles we use a word-weighted algorithm to compare words from the Title and Abstract of each citation.
The Obesity Paradox in Cancer: Epidemiologic Insights and Perspectives.
Lee DH , Giovannucci EL
Curr Nutr Rep , 8(3):175-181, 01 Sep 2019
Cited by: 58 articles | PMID: 31129887
Adiposity-Mortality Relationships in Type 2 Diabetes, Coronary Heart Disease, and Cancer Subgroups in the UK Biobank, and Their Modification by Smoking.
Jenkins DA , Bowden J , Robinson HA , Sattar N , Loos RJF , Rutter MK , Sperrin M
Diabetes Care , 41(9):1878-1886, 03 Jul 2018
Cited by: 19 articles | PMID: 29970414
Obesity and Cancer Treatment Outcomes: Interpreting the Complex Evidence.
Slawinski CGV , Barriuso J , Guo H , Renehan AG
Clin Oncol (R Coll Radiol) , 32(9):591-608, 25 Jun 2020
Cited by: 22 articles | PMID: 32595101
From the BMI paradox to the obesity paradox: the obesity-mortality association in coronary heart disease.
Antonopoulos AS , Antonopoulos AS , Oikonomou EK , Antoniades C , Tousoulis D
Obes Rev , 17(10):989-1000, 13 Jul 2016
Cited by: 70 articles | PMID: 27405510
Body mass index versus surrogate measures of central adiposity as independent predictors of mortality in type 2 diabetes.
Orsi E , Solini A , Penno G , Bonora E , Fondelli C , Trevisan R , Vedovato M , Cavalot F , Lamacchia O , Haxhi J , Nicolucci A , Pugliese G , Renal Insufficiency And Cardiovascular Events (RIACE) Study Group
Cardiovasc Diabetol , 21(1):266, 02 Dec 2022
Cited by: 4 articles | PMID: 36461034 | PMCID: PMC9716975
Funding
Funders who supported this work.
Cancer Research UK (2)
Development of a risk prediction tool for early cancer detection in patients with type 2 diabetes.
Professor Andrew Renehan, University of Manchester
Grant ID: 17962
7 publication s
Grant ID: A17962
1 publication
Medical Research Council (3)
Grant ID: 1863381
Health e-Research Centre -HeRC
Prof Iain Buchan, The University of Manchester
Grant ID: MC_PC_13042
542 publication s
MICA: Health e-Research Centre
Professor John Ainsworth, The University of Manchester
Grant ID: MR/K006665/1
607 publication s
University of Manchester
Europe PMC is part of the ELIXIR infrastructure
Thank you for visiting nature.com. You are using a browser version with limited support for CSS. To obtain the best experience, we recommend you use a more up to date browser (or turn off compatibility mode in Internet Explorer). In the meantime, to ensure continued support, we are displaying the site without styles and JavaScript.
- View all journals
- My Account Login
- Explore content
- About the journal
- Publish with us
- Sign up for alerts
- Open access
- Published: 25 April 2024
Surgery is associated with better long-term outcomes than pharmacological treatment for obesity: a systematic review and meta-analysis
- Leonardo Zumerkorn Pipek 1 ,
- Walter Augusto Fabio Moraes 2 ,
- Rodrigo Massato Nobetani 2 ,
- Vitor Santos Cortez 2 ,
- Alberto Santos Condi 2 ,
- João Victor Taba 2 ,
- Rafaela Farias Vidigal Nascimento 3 ,
- Milena Oliveira Suzuki 2 ,
- Fernanda Sayuri do Nascimento 2 ,
- Vitoria Carneiro de Mattos 2 ,
- Leandro Ryuchi Iuamoto 4 ,
- Wu Tu Hsing 4 ,
- Luiz Augusto Carneiro-D’Albuquerque 5 ,
- Alberto Meyer 5 &
- Wellington Andraus 5
Scientific Reports volume 14 , Article number: 9521 ( 2024 ) Cite this article
368 Accesses
1 Altmetric
Metrics details
- Endocrine system and metabolic diseases
- Gastrointestinal diseases
Obesity is a highly prevalent disease with numerous complications. Both intensive medical treatment with the use of pharmacological drugs and bariatric surgery are current options. The objective of this meta-analysis was to compare, in the long-term, intensive medical treatment and surgery based on twelve parameters related to weight loss, cardiovascular and endocrine changes. A review of the literature was conducted in accordance with the PRISMA guidelines (PROSPERO: CRD42021265637). The literature screening was done from inception to October 2023 through PubMed, EMBASE and Web of Science databases. We included randomized clinical trials that had separate groups for medical treatment and bariatric surgery as an intervention for obesity. The risk of bias was assessed through RoB2. A meta-analysis was performed with measures of heterogeneity and publication bias. Subgroup analysis for each surgery type was performed. Data is presented as forest-plots. Reviewers independently identified 6719 articles and 6 papers with a total 427 patients were included. All studies were randomized controlled trials, three had a follow up of 5 years and two had a follow up of 10 years. Both groups demonstrated statistical significance for most parameters studied. Surgery was superior for weight loss (− 22.05 kg [− 28.86; − 15.23), total cholesterol (− 0.88 [− 1.59; − 0.17]), triglycerides (− 0.70 [− 0.82; − 0.59]), HDL (0.12 [0.02; 0.23]), systolic pressure (− 4.49 [− 7.65; − 1.33]), diastolic pressure (− 2.28 [− 4.25; − 0.31]), Hb glycated (− 0.97 [− 1.31; − 0.62]), HOMA IR (− 2.94; [− 3.52; − 2.35]) and cardiovascular risk (− 0.08; [− 0.10; − 0.05]). Patient in the surgical treatment group had better long term outcomes when compared to the non-surgical group for most clinical parameters.
Similar content being viewed by others
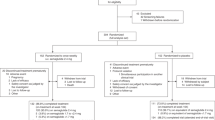
Two-year effects of semaglutide in adults with overweight or obesity: the STEP 5 trial
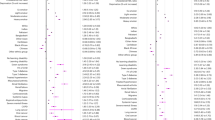
Development and validation of a new algorithm for improved cardiovascular risk prediction
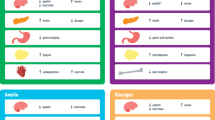
What is the pipeline for future medications for obesity?
Introduction.
Obesity has been a known condition for over 2000 years 1 but that has become much more prevalent in recent decades. Despite great efforts to prevent this disease, the prevalence in adults in the United States has increased in recent decades and reached 42.4% in 2018. The GBD Obesity Study 2 Collaborators 2015 showed that this increasing trend occurred in more than 70 countries and is highly expressive in adolescents.
The classification of obesity is defined by a body mass index (BMI) greater than 30 kg/m 2 . The psychological damage that many of these patients suffer in a society governed by aesthetic standards is just one of the most visible and immediate consequences of obesity. Mortality from cardiovascular causes and its relationship with BMI has already been widely studied 3 , showing that the risk increases progressively with the increase of the index. Similarly, obesity was associated with a higher incidence of cancer 4 , respiratory 5 and metabolic 6 diseases.
In this context, the importance of effective treatment of this condition is clear, reducing mortality and improving the quality of life of these patients. While some benefits are evident with a loss of just 5% 6 of their weight, many patients require a more expressive loss to reduce the risks associated with obesity.
There are several treatments available for weight loss. Lifestyle changes, low calorie diet and increasing physical activity are the mainstay treatment for all patients 7 , 8 . Specific weight loss diets and exercise programs have also been developed for this purpose, yielding varying results. Finally, pharmacological, and surgical treatment has gained more attention in recent years for selected patients in whom other measures were insufficient.
Several studies have demonstrated the effectiveness of bariatric surgery in the short and medium term for the treatment of obesity. More recent studies have also shown that new drugs developed for weight loss may be a viable option for the treatment of this disease 8 , 9 . Comparison of these new drugs with surgical treatment is scarce in the literature and aimed only at evaluating changes related to weight loss in a short period of time.
This systematic review evaluated the hypothesis whether surgical treatment is superior than non-surgical treatment for patients with obesity. We evaluated the long-term effect of these treatments on anthropometric measures (weight, waist circumference, BMI) and on obesity related pathologies (triglycerides, LDL, HDL, total cholesterol, cardiovascular risk, systolic and diastolic blood pressure, HOMA and glycated hemoglobin).
Materials and methods
This systematic review was carried out in accordance with the items of Preferred Reports for Systematic Reviews and Protocol Meta-Analysis (PRISMA-P) 10 and assessing the methodological quality of systematic reviews (AMSTAR-2) guidelines 11 . This study was registered by the Prospective Register of Systematic Reviews (PROSPERO, 258667) before the research was carried out.
Drafting of the research question was based on the PICO strategy 12 , considering: P (Patients with obesity with indication for bariatric surgery based on BMI); I (Bariatric Surgery); C (Pharmacological treatment); O (Long term morbidity/mortality—at least 5 years of follow up).
Eligibility criteria
Inclusion criteria.
Types of studies: Randomized clinical trials.
Types of participants: Patients eligible for bariatric surgery, according to the American Society for Metabolic and Bariatric Surgery (ASMBS).
Types of intervention: Bariatric surgery or medical treatment.
Exclusion criteria
Studies were excluded if they: (1) did not have one group for each type of intervention (surgery or pharmacologic treatment); (2) had a heterogeneous population; (3) did not use a standard assessment method for the entire duration of the study, or did not have pre-assessment; (4) were not related to the question in the review; (5) were in a language other than English, Portuguese or Spanish; (6) were incomplete, unpublished or inaccessible to the authors.
Types of variables/parameters analyzed
Data was collected and arranged in tables, including the authors name, date and country of publication, number of participants included in the final analysis, sex, age, and body mass index.
Literature revision
The survey was from inception to October 10, 2023, without language restrictions, in the Medline database (via PubMed), EMBASE and Web of Science.
Using the search tool, we selected MeSH terms from the most relevant publications to conduct a new search to obtain articles that could be included in this systematic review. In addition, a manual search of theses, meetings, references, study records and contact with experts in the field was carried out.
Search strategy
The same keywords were used in all databases, according to each database input format.
The search strategy was:
(Bariatric Surgery) AND ((nonsurgical) OR (Orlistat) OR (phentermine) OR (topiramate) OR (lorcaserin) OR (naltrexone) OR (bupropion) OR (liraglutide) OR (conservative) OR (conventional) OR (Anti-Obesity Agents) OR (Intensive medical)) AND (obesity) → 3024.
(Bariatric Surgery) AND ((nonsurgical) OR (conservative) OR (Anti-Obesity Agents) OR (Intensive medical)) AND (obesity) → 4732.
Web of Science:
(Bariatric Surgery) AND ((nonsurgical) OR (conservative) OR (Anti-Obesity Agents) OR (Intensive medical)) AND (obesity) → 1772.
Data extraction
The data for each study was extracted independently by two authors. Disagreements were resolved by consensus. If no consensus was reached, a third author was consulted. Data extraction was carried out using the Rayyan tool— https://rayyan.qcri.org/ 13 .
All studies were analyzed by their titles and abstracts, according to inclusion and exclusion criteria. If the eligibility criteria was met, the full text would be extracted. All studies eligible for qualitative analysis are described in the “Results” section.
Missing data was clarified by contacting the authors directly.
Data validation
The risk of bias for intervention-type studies was analyzed using the guidelines of the Cochrane Back Review Group (CBRG) 14 .
Statistical analysis
As several studies of sufficient quality were available, a meta-analysis was carried out with measures of heterogeneity and publication bias. The data was presented through forest-plots, according to their statistical relevance.
Characteristics of study participants are presented as means, minimum and maximum values for quantitative variables, and as frequencies and percentages for qualitative variables. The prevalence values and 95% confidence intervals was calculated using the Wilson method To assess the global heterogeneity between the studies, Cochran's Q test was calculated, as well as the I2 (percentage of variation). The results of the studies' association measures and their respective 95% confidence intervals are presented in forest-plots.
Statistical analysis were performed using the Stata/MP 14.0 software for Windows.
Study selection
The electronic search found 9528 results for the keywords used. After removing 2809 duplicates and screening through abstract, we considered 55 potentially eligible studies for full-text analysis. Of these, 49 did not respect the exclusion criteria. Only 6 studies were considered eligible for qualitative analysis and 6 articles were eligible for meta-analysis [Fig. 1 ].
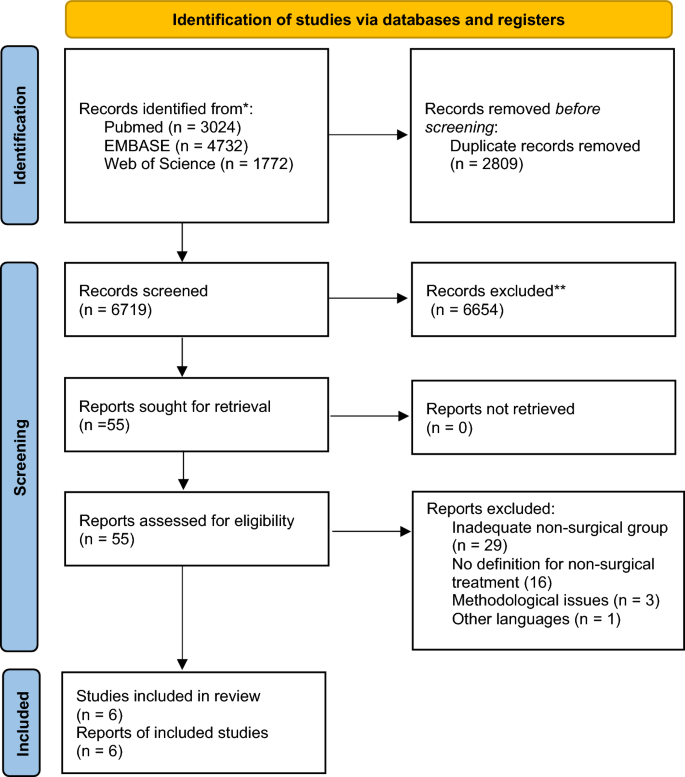
PRISMA 2020 flow diagram for new systematic reviews.
Many studies were excluded due to lack of description for the intervention in the non-surgical group.
Study characteristics
The following articles were included in the systematic review and meta-analysis 15 , 16 , 17 , 18 , 19 , 20 . In total, there were 427 participants. All studies were RCT. Four had a follow up of five years 15 , 16 , 19 and two had a follow up of 10 years 17 , 18 . Of the six eligible studies, two were undertaken in the United States of America 15 , 16 , two in Italy 17 , 19 , one in Australia 18 , and one in Singapore 20 . Study characteristics and detailed demographics can be found in Tables 1 and 2 . All studies included a group treated exclusively with intensive medical treatment (IMT). The definition of IMT differed between them but were considered if the patients had frequent follow up visits and were instructed on health habits including exercise and diet, with or without the use of pharmacological treatment.
There were four modalities of surgery used for weight loss: Roux-en-Y Gastric Bypass (RYGB) 15 , 17 , 18 , 19 , 20 ; Biliopancreatic diversion (BPD) 17 , 19 ; Laparoscopic Sleeve Gastrectomy (LSG) 15 , 16 ; Laparoscopic Adjustable Gastric Band (LAGB) 18 . The subgroup analysis for outcomes separated studies in RYGB, LSG and other types of surgery. The non-surgical treatment for obesity included one or the combination of the following medications: Orlistat, Phentermine, Naltrexone, Bupropion, Liraglutide, Lorcaserin, Sibutramine.
Risk of bias
After reading the articles included in the systematic review, the following elements were analyzed to determine the level of evidence: study design and selection, detection, loss, reporting and information bias. The summary of the risk of bias analysis for each of the included articles is presented in Fig. 2
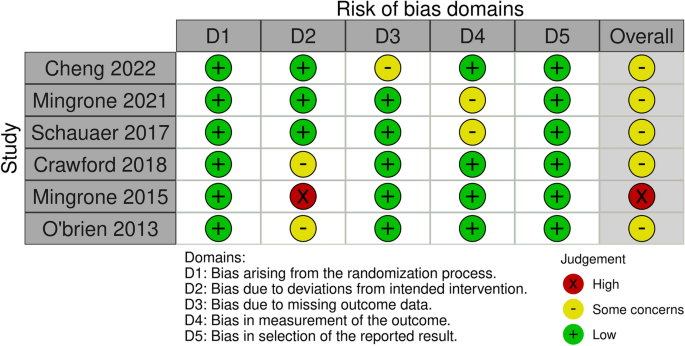
Risk of bias analysis.
All studies had a low risk of bias for most criteria. In three of the studies, assessors were aware of the intervention received by study participants or the information was not available 16 , 17 , 20 . Three other studies 15 , 18 , 19 had bias regarding deviations from intended interventions due to the fact that an appropriate analysis to estimate the effects of assignment to intervention was not performed 15 ; patients assigned to the control group crossed over to the intervention group, and no measures were reportedly taken to balance that deviation 19 ; there was a significant loss of follow-up for all groups 20 .
All six studies had data on weight loss after treatment. Mean difference values and their respective 95% confidence intervals (95% CI) were calculated. In Fig. 3 A, the forest plot is shown. All publications found that surgical procedures were more efficient for long term weight loss. The global MD value was − 22.1 kg (95% CI [− 28.9; − 15.2). The measure of heterogeneity I2 (Higgins heterogeneity measure) was 77.8%, a value considered as high heterogeneity. According to Cochran’s Q heterogeneity test, the sample evidence did allow us to reject the null hypothesis of non-heterogeneity ( p = 0.01).The subgroup analysis showed that there was not a significant difference between the types of surgery ( p = 0.30).
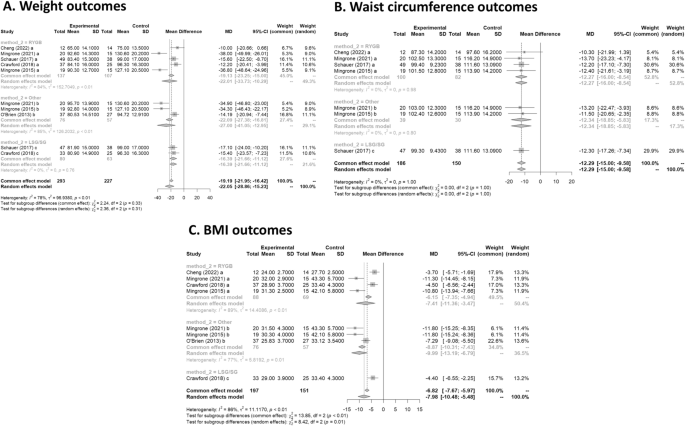
(A ) Weight outcomes; ( B ) Waist circumference outcomes; ( C ) BMI outcomes.
Waist circumference
Four studies had data on waist circumference 16 , 17 , 19 , 20 . In Fig. 3 B, the forest plot is shown. Patients treated with surgery had a mean difference of − 12.3 (95% CI [− 15.0; − 9.6]) compared to IMT. The measure of heterogeneity I2 (Higgins heterogeneity measure) was 0%, a value considered as low heterogeneity. According to Cochran’s Q heterogeneity test, the sample evidence did not allow us to reject the null hypothesis of non-heterogeneity ( p = 0.99).
The subgroup analysis showed that there was not a significant difference between the types of surgery ( p = 0.99).
Five studies had data on BMI 16 , 17 , 18 , 19 , 20 . In Fig. 3 C, the forest plot is shown. Patients treated with surgery had a mean difference of − 8.0 (95% CI [− 10.5; − 5.5]) compared to IMT. The measure of heterogeneity I2 (Higgins’s heterogeneity measure) was 84%, a value considered high heterogeneity. According to Cochran’s Q heterogeneity test, the sample evidence did allow us to reject the null hypothesis of non-heterogeneity ( p = 0.01).
The subgroup analysis showed that there was a significant difference between the types of surgery ( p = 0.01). The group with LAGB and BPD surgery had the highest decrease in BMI, with a mean of − 10.0.
Triglycerides
Three studies had data on tryglycerides 17 , 19 , 20 . In Fig. 4 A, the forest plot is shown. Patients treated with surgery had a mean difference of − 0.7 (95% CI [− 0.8; − 0.6]) compared to IMT. The measure of heterogeneity I2 (Higgins’s heterogeneity measure) was 50.4%, a value considered high heterogeneity. According to Cochran’s Q heterogeneity test, the sample evidence did not allow us to reject the null hypothesis of non-heterogeneity ( p = 0.08).
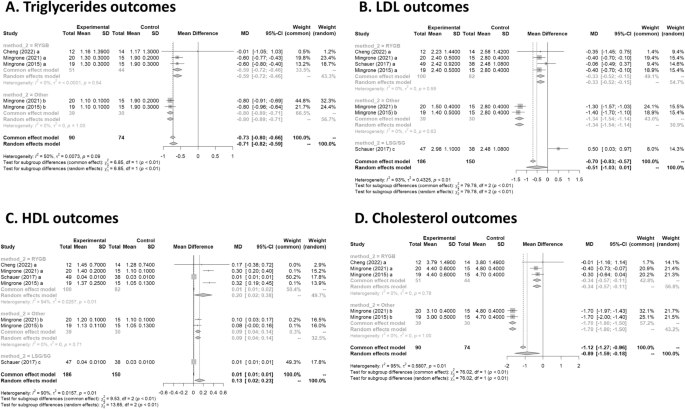
(A ) Triglycerides outcomes; ( B ) LDL outcomes; ( C ) HDL outcome; ( D ) Cholesterol outcomes.
The subgroup analysis showed that there was a significant difference between the types of surgery ( p = 0.01), with a worse outcome for RYGB.
Four studies had data on LDL 16 , 17 , 19 , 20 . In Fig. 4 B, the forest plot is shown. Patients treated with surgery had a mean difference of − 0.5 (95% CI [− 1.0; 0.0]) compared to IMT. The measure of heterogeneity I2 (Higgins’s heterogeneity measure) was 92.7%, a value considered high heterogeneity. According to Cochran’s Q heterogeneity test, the sample evidence did allow us to reject the null hypothesis of non-heterogeneity ( p = 0.01).
The subgroup analysis showed that there was a significant difference between the types of surgery ( p = 0.01). There was an increase of 0.5 in LDL for the LSG group. The group with LAGB and BPD surgery had the highest decrease in LDL, with a mean of − 1.3.
Four studies had data on HDL 16 , 17 , 19 , 20 . In Fig. 4 C, the forest plot is shown. Patients treated with surgery had a mean difference of 0.1 (95% CI [0.0; 0.2]) compared to IMT. The measure of heterogeneity I2 (Higgins’s heterogeneity measure) was 90.5%, a value considered high heterogeneity. According to Cochran’s Q heterogeneity test, the sample evidence did allow us to reject the null hypothesis of non-heterogeneity ( p = 0.01).
The subgroup analysis showed that there was a significant difference between the types of surgery ( p = 0.01). The group with RYGB surgery had the highest significant increase in HDL, with a mean of 0.2.
Cholesterol
Three studies had data on cholesterol 17 , 19 , 20 . In Fig. 4 D, the forest plot is shown. Patients treated with surgery had a mean difference of − 0.9 (95% CI [− 1.6; − 0.2]) compared to IMT. The measure of heterogeneity I2 (Higgins’s heterogeneity measure) was 94.8%, a value considered as high heterogeneity. According to Cochran’s Q heterogeneity test, the sample evidence did allow us to reject the null hypothesis of non-heterogeneity ( p = 0.01).
The subgroup analysis showed that there was a significant difference between the types of surgery ( p = 0.01). The group with LAGB and BPD surgery had the highest decrease in cholesterol, with a mean of − 1.7.
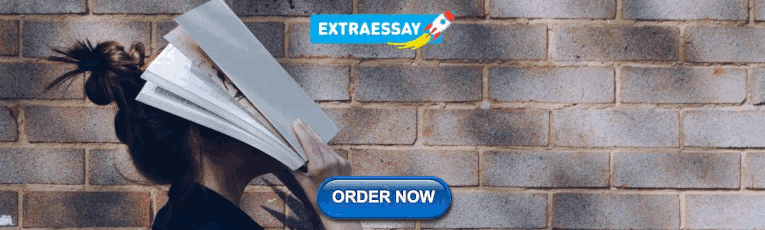
Cardiovascular risk
Two studies had data on cardiovascular risk 17 , 19 . In Fig. 5 A, the forest plot is shown. Patients treated with surgery had a mean difference of − 0.08 (95% CI [− 0.10; − 0.05]) compared to IMT. The measure of heterogeneity I2 (Higgins’s heterogeneity measure) was 0%, a value considered as low heterogeneity. According to Cochran’s Q heterogeneity test, the sample evidence did not allow us to reject the null hypothesis of non-heterogeneity ( p = 0.44).
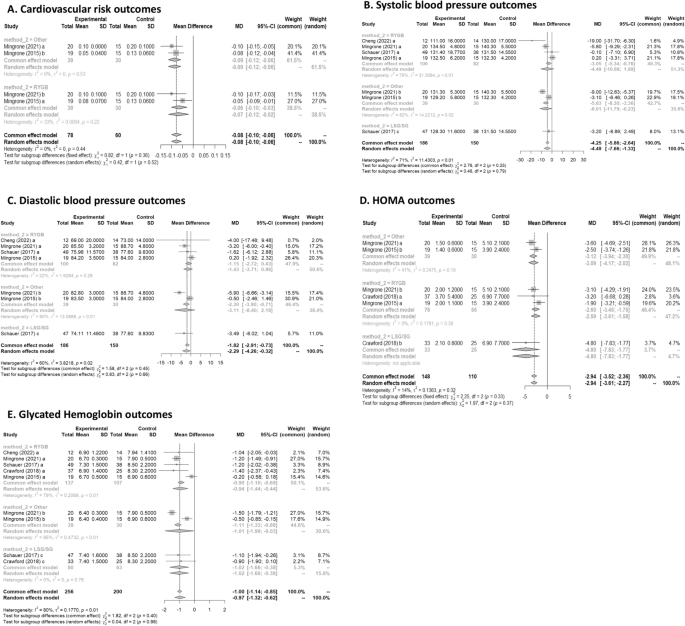
(A ) Cardiovascular risk outcomes; ( B ) Systolic blood pressure outcomes; ( C ) Diastolic blood pressure outcomes; ( D ) HOMA outcomes; ( E ) Glycated Hemoglobin outcomes.
The subgroup analysis showed that there was no significant difference between the types of surgery ( p = 0.36).
Systolic blood pressure
Four studies had data on systolic blood pressure 16 , 17 , 19 , 20 . In Fig. 5 B, the forest plot is shown. Patients treated with surgery had a mean difference of − 4.49 (95% CI [− 7.65; − 1.33]) compared to IMT. The measure of heterogeneity I2 (Higgins’s heterogeneity measure) was 71%, a value considered as high heterogeneity. According to Cochran’s Q heterogeneity test, the sample evidence did allow us to reject the null hypothesis of non-heterogeneity ( p = 0.01).
The subgroup analysis showed that there was not a significant difference between the types of surgery ( p = 0.79).
Diastolic blood pressure
Four studies had data on diastolic blood pressure 16 , 17 , 19 , 20 . In Fig. 5 C, the forest plot is shown. Patients treated with surgery had a mean difference of − 2.28 (95% CI [− 4.25; − 0.31]) compared to IMT. The measure of heterogeneity I2 (Higgins’s heterogeneity measure) was 60.5%, a value considered as high heterogeneity. According to Cochran’s Q heterogeneity test, the sample evidence did allow us to reject the null hypothesis of non-heterogeneity ( p = 0.01).
The subgroup analysis showed that there was not a significant difference between the types of surgery ( p = 0.66).
Three studies had data on HOMA 15 , 17 , 19 . In Fig. 5 D, the forest plot is shown. Patients treated with surgery had a mean difference of − 2.94 (95% CI [− 3.52; − 2.35]) compared to IMT. The measure of heterogeneity I2 (Higgins’s heterogeneity measure) was 14%, a value considered as low heterogeneity. According to Cochran’s Q heterogeneity test, the sample evidence did not allow us to reject the null hypothesis of non-heterogeneity ( p = 0.32).
The subgroup analysis showed that there was no significant difference between the types of surgery ( p = 0.33).
Glycated Hemoglobin
Five studies had data on glycated haemoglobin 15 , 16 , 17 , 19 , 20 . In Fig. 5 E, the forest plot is shown. Patients treated with surgery had a mean difference of − 1.0(95% CI [− 1.3; − 0.6]) compared to IMT. The measure of heterogeneity I2 (Higgins’s heterogeneity measure) was 79.8%, a value considered as high heterogeneity. According to Cochran’s Q heterogeneity test, the sample evidence did allow us to reject the null hypothesis of non-heterogeneity ( p = 0.01).
The subgroup analysis showed that there was no significant difference between the types of surgery ( p = 0.98).
Obesity is defined as a BMI greater than or equal to 30 by the CDC and is currently among the most prevalent diseases in the world, in addition to being an important risk factor for many other diseases. It has high rates of morbidity and mortality 21 , 22 and, in this context, weight loss can bring countless positive impacts to the individual. Currently, there are several treatments for obesity, and we can divide them into non-surgical or surgical.
Non-surgical treatments include non-drug and drug treatments. Among the non-medicated, we can highlight the change in eating habits, regular physical exercise, and cognitive behavioral therapy 8 . Ideally, these measures should be implemented for all patients living with obesity, even for those who will undergo drug or surgical treatment. Recently, in addition to lifestyle change, neuromodulation with deep transcranial stimulation has also been studied and has shown effectiveness in weight loss reduction 23 .
A systematic review carried out in 2021, which analyzed 64 articles concluded that among the most effective non-surgical interventions are low-carbohydrate or low-fat diets and combined therapies. This study also showed that non-drug interventions, such as physical exercise, when used alone, are not very effective in reducing the weight of these patients Therefore, a combination of two or more therapies should be chosen 24 .
Pharmacological treatment must be chosen together with the patient. One or more drugs can be used, the main ones used being: Liraglutide, Semaglutide, Tirzepatide, Orlistat, Phentermine and Sibutramine 25 .
Liraglutide was recently approved for the treatment of obesity and is now one of the most widely used drugs. It acts as a GLP-1 receptor agonist 26 , 27 , 28 , enhancing its effects. This group of drugs is already known in the treatment of Type 2 Diabetes Mellitus, a condition that can often be associated with obesity 29 , 30 , since its pathophysiology involves increased insulin resistance. The main actions of this drug are: increased satiety due to a reduction in the speed of gastric emptying, increased insulin release and decreased glucagon release. Semaglutide is a drug with a similar mechanism of action who demonstrated not only a substantial weight loss 31 , but was also associated with a lower 10-year T2D risk in people with overweight or obesity after 2 years of follow up 32 . More recently, a new drug that combines GLP-1 and GIP receptor agonist, Tirzepatide, has shown even better results in the short term 33 .
Orlistat, in turn, reversibly inhibits the lipase enzyme 34 , which has the function of breaking down fat from food for its absorption, as well as inhibiting the absorption of ingested triglycerides. Thus, there is elimination of fat in the feces 35 . The main adverse effects are gastrointestinal symptoms, however this can be beneficial as it leads to a change in behavior, for example causing a lower consumption of foods rich in fat 36 .
Phentermine, an amphetamine analogue, can be used in conjunction with topiramate for the treatment of obesity. The mechanism of action of the drugs is not yet known, however, significant weight loss has already been observed, in addition to a reduction in the consumption of hypercaloric foods and a decrease in the speed of gastric emptying with the use of this combination of drugs 37 , 38 .
Sibutramine, widely used in the 1990s, acts to inhibit the reuptake of serotonin, norepinephrine, and dopamine 34 . Serotonin, in turn, activates POMC system neurons and inhibits NPY neurons, thereby promoting reduced appetite and increased satiety. Despite generating weight reduction 39 , some data show increased cardiovascular risk 40 , and therefore, it is no longer used as a first-line drug.
Among the possible surgeries, the most performed today are: Roux-en-Y Gastric Bypass (RYGB), Biliopancreatic diversion (BPD), Laparoscopic Sleeve Gastrectomy (LSG) and Laparoscopic Adjustable Gastric Band (LAGB). According to the NIH and the American Bariatric Society 41 , 42 , some indications for performing bariatric surgery are adults with BMI greater than or equal to 40 and adults with BMI greater than 35 accompanied by some comorbidity such as type 2 diabetes mellitus, obstructive sleep apnea or hypertension.
RYGB is one of the best-known procedures and its complications vary according to the surgical technique used. Some complications include gastric distention, ulcers, cholelithiasis, hernias, dumping syndrome, and hyperammonaemia encephalopathy.
BPD presents long-term nutritional complications, such as anemia, bone diseases and fat-soluble vitamin deficiency. This technique has high mortality rates, mainly due to the complexity of the technique.
Among the procedures described, LSG is the one with the fewest complications, being described in the literature bleeding or stenosis of the stoma. An alternative technique using endoscopy for sleeve gastroplasty has shown to be safe and efficient for weight loss after 104 weeks, with important improvements in metabolic comorbidities 43 .
The procedure with the lowest mortality rate is the LAGB 44 . Despite this, it can present complications such as obstruction, band erosion, band slippage and gastric prolapse, esophagitis, hernia, in addition to having a high rate of reoperation, reaching 50% of patients who underwent this surgery 45 .
In this article, we compare data on weight loss through intensive drug treatment, which includes changes in eating habits, physical exercise, and medications, and through surgical treatment. Both treatments showed that weight loss caused an improvement in the lipid panel, with a reduction in total cholesterol, triglycerides and LDL, an increase in HDL, improvement in systolic and diastolic blood pressure, decrease in glycated hemoglobin and insulin resistance (accessed through HOMA), in addition to reducing the risk for cardiovascular diseases.
Our systematic review confirmed the findings of individual studies that bariatric surgery has a greater potential for weight reduction, BMI and waist circumference, as already described in individual articles and widely in the literature. It should be noted that even in the long term, this difference remained. Similarly, a 2014 Cochrane systematic review 46 comparing RCT with more than 1 year of follow-up showed that all 7 articles included demonstrated an advantage of the surgical group. An article 47 on the use of pharmacological treatment for obesity showed that even recent drugs approved, including GLP 1 agonists, are not able to reduce weight to levels similar to those of bariatric surgery to date, despite the emergence of new drugs still in initial phase 48 . It is worth mentioning that in these studies the comparison time is relatively short (12 months) and that we do not have data on the long-term impact. Thus, in relation to long term weight loss, bariatric surgery is still the best option.
Most articles were not able to individually demonstrate that surgical treatment is superior to non-surgical in terms of pressure reduction. However, the result of the meta-analysis showed a superiority of the surgical group in relation to both systolic and diastolic pressure, more pronounced in the BPD group. Wang 49 performed a systematic review focused on the impact on pressure and demonstrated that there was a reduction in systolic and diastolic values, but the subgroup analysis showed that this occurs only in the RYGB groups for systolic pressure. Similarly, Schiavon also demonstrated a significant reduction in the need of blood pressure medication after 3 years in the RYGB group when compared intensive medical treatment for obesity 50 . This difference found in only one subtype of surgery seems to be just a reflection of the sample size, which can be interpreted that surgical treatment in general tends to reduce pressure to a greater extent than non-surgical treatment. The fact that different types of surgery are significant may reflect the studies selected in our meta-analysis, which have longer follow-ups.
In relation to both HOMA-IR and glycated Hb, there was a more significant improvement in the group that underwent surgery. The way in which the data on diabetes remission was reported in the articles did not allow a meta-analysis to be carried out with these data and, therefore, it was not included. However, individual data from the Mingrone 2015, Mingrone 2021 and Schauer articles showed that the surgery group had better results. A network meta-analysis from 2021 51 comparing the different types of metabolic surgery for the treatment of obesity and diabetes showed that RYGB was 20% more likely to result in remission of type 2 diabetes compared to SG. There was no significant difference between the other groups. Moreover, the effects of bariatric surgery on diabetes is not exclusive for patients with obesity, as shown by a study with patients with a BMI of 27–32 kg/m 2 that had a better glycemic control when treated with RYGB 20 . Regarding the lipid profile, Schauer's study was not able to demonstrate superiority in relation to LDL and HDL parameters. However, by combining the data from Mingrone's articles, it is possible to demonstrate that surgical treatment is superior. Regarding cholesterol reduction, Mingrone's studies showed that although RYGB and BDP were better in relation to non-surgical treatment, the BDP technique had a statistically greater reduction in relation to RYGB. This can be explained by the greater intestinal exclusion in BDP and, therefore, having a greater impact on lipid absorption. Despite Sayeed's study 52 et al. was not included in this meta-analysis due to the inadequate way of separating the groups for analysis, the results regarding the lipid profile showed that the group that received both interventions was superior to the exclusive non-surgical treatment. It is important to point out that despite a statistically significant difference between the groups, the effect size of this difference is probably not clinically significant.
The choice of treatment for obesity can also have an impact on several other patient comorbidities. Hossain et al. 53 performed a systematic review with 26 studies that showed that bariatric surgery appears to be more effective in the treatment of asthma. Similarly, a study by Crawford et al. 15 showed that there is a greater increase in bone turnover in groups undergoing bariatric surgery in relation to pharmacological treatment. Other than that, bariatric surgery is also demonstrated to be superior in the treatment of other obesity related pathologies, such as Non-Alcoholic Steatohepatitis (NASH), and in the treatment of obesity in adolescents 54 , 55 .
The effect of major cardiovascular adverse events (MACE) and mortality 56 have also been promising for bariatric surgery. A recent cohort comparing bariatric surgery in patients with obesity and use of GLP1-agonists inpatients with diabetes showed a lower risk of MACE in the surgical group 57 . The surgical treatment has also shown superiority when compared to medical treatment regarding the prevention of diabetic kidney disease in 5 years for patients with diabetes and obesity 58 . Boyers et al. evaluated the cost-effectiveness of surgical and pharmacological treatment in the treatment of obesity and found that RYGB should be the treatment of choice only if the optimization of health system costs is considered 59 .
Another important consideration is the fact that pharmacological and surgical treatment for obesity are not mutually exclusive. Most clinicians choose to combine both treatment modalities in practice to improve results. Weight gain after bariatric surgery is a known possibility, and for those patients, two-thirds of the weight regain can be safely lost with GLP1 agonist, providing clinicians with a therapeutic option for this clinical challenge.
Methodologies and limitations of the studies
Despite the large number of articles in the literature on the treatment of obesity, there are few RCTs comparing non-surgical and surgical treatment, and most of them only follow up in the short term. In addition, many articles do not adequately describe the strategy used in non-surgical treatment. This lack of data and standardization in this type of treatment can lead to bias and possibly the formation of extremely heterogeneous groups for analysis.
Most of the studies included in our systematic review have diabetes as an inclusion criteria. In this circumstance, our findings may not be generalized to patients with obesity without diabetes.
Another important limitation of our systematic review refers to pharmacological treatment in the non-surgical group. The use of GLP 1 agonists has great potential in the treatment of obesity, but they have only started to be used recently. As the purpose of our article is to assess the long-term impact, there are still few articles available that used this drug. The use of the most recent medications, such as Tirzepatide, could not be evaluated in our study, once there are no RCTs in the literature presenting its long-term effects. Those drugs proved to be very efficient and might have similar effect in the long term. Future systematic reviews may reveal a different results when including the new generation of weight loss medication.
Finally, choosing the most appropriate treatment often involves individual characteristics of each patient, and the impact on quality of life can be extremely subjective and difficult to assess.
Obesity is a disease that increases the morbidity and mortality of patients, contributing to several secondary diseases. This systematic review evaluated the impact on the main variables related to obesity in the long term. The findings indicated that both treatment modalities are efficacious in managing obesity; however, the surgical group demonstrated superior outcomes in comparison to the non-surgical group across most variables. Nonetheless, the advent of novel pharmacological treatments has shown promising potential. Further studies focusing on the long-term impacts of these new drug treatments should be undertaken to allow for a comprehensive comparison with non-surgical treatment methods.
Data availability
Data is provided within the manuscript or supplementary information files.
Bray, G. The Battle of the Bulge: A History of Obesity Research (Dorrance Pub., 2007).
Collaborators GBD 2015 O, Afshin, A., Forouzanfar, M. H., Reitsma, M. B., Sur, P., Estep, K. et al. Health effects of overweight and obesity in 195 countries over 25 years. N. Engl. J. Med. 377 , 13–27 (2017).
Whitlock, G. et al. Body-mass index and cause-specific mortality in 900 000 adults: Collaborative analyses of 57 prospective studies. Lancet 373 , 1083–1096 (2009).
Article PubMed Google Scholar
Steele, C. B. et al. Vital Signs: Trends in incidence of cancers associated with overweight and obesity—United States, 2005–2014. MMWR Morb. Mortal. Wkly. Rep. 66 , 1052–1058 (2017).
Article PubMed PubMed Central Google Scholar
Goldhaber, S. Z. et al. Risk factors for pulmonary embolism. The Framingham Study. Am. J. Med. 74 , 1023–1028 (1983).
Article CAS PubMed Google Scholar
Knowler, W. C. et al. Reduction in the incidence of type 2 diabetes with lifestyle intervention or metformin. N. Engl. J. Med. 346 , 393–403 (2002).
Bray, G. A., Frühbeck, G., Ryan, D. H. & Wilding, J. P. H. Management of obesity. The Lancet 387 , 1947–1956 (2016).
Article Google Scholar
Perdomo, C. M., Cohen, R. V., Sumithran, P., Clément, K. & Frühbeck, G. Contemporary medical, device, and surgical therapies for obesity in adults. The Lancet 401 , 1116–1130 (2023).
Updike, W. H. et al. Is it time to expand glucagon-like peptide-1 receptor agonist use for weight loss in patients without diabetes?. Drugs 81 , 881–893 (2021).
Moher, D. et al. Preferred reporting items for systematic review and meta-analysis protocols (PRISMA-P) 2015 statement. Syst. Rev. 4 , 1 (2015).
Shea, B. J. et al. AMSTAR 2: A critical appraisal tool for systematic reviews that include randomised or non-randomised studies of healthcare interventions, or both. BMJ 358 , j4008 (2017).
Brown, D. A review of the PubMed PICO Tool: using evidence-based practice in health education. Health Promot. Pract. 21 , 496–498 (2020).
Ouzzani, M., Hammady, H., Fedorowicz, Z. & Elmagarmid, A. Rayyan—A web and mobile app for systematic reviews. Syst. Rev. 5 , 210 (2016).
Leeflang, M. M. G., Deeks, J. J., Takwoingi, Y. & Macaskill, P. Cochrane diagnostic test accuracy reviews. Syst. Rev. 2 , 82 (2013).
Crawford, M. R. et al. Increased bone turnover in type 2 diabetes patients randomized to bariatric surgery versus medical therapy at 5 years. Endocr. Pract. 24 , 256–264 (2018).
Schauer, P. R. et al. Bariatric surgery versus intensive medical therapy for diabetes—5-Year outcomes. N. Engl. J. Med. 376 , 641–651 (2017).
Mingrone, G. et al. Metabolic surgery versus conventional medical therapy in patients with type 2 diabetes: 10-Year follow-up of an open-label, single-centre, randomised controlled trial. The Lancet 397 , 293–304 (2021).
O’Brien, P. E., Brennan, L., Laurie, C. & Brown, W. Intensive medical weight loss or laparoscopic adjustable gastric banding in the treatment of mild to moderate obesity: Long-term follow-up of a prospective randomised trial. Obes. Surg. 23 , 1345–1353 (2013).
Mingrone, G. et al. Bariatric-metabolic surgery versus conventional medical treatment in obese patients with type 2 diabetes: 5 Year follow-up of an open-label, single-centre, randomised controlled trial. The Lancet 386 , 964–973 (2015).
Cheng, A. et al. Roux-en-Y gastric bypass versus best medical treatment for type 2 diabetes mellitus in adults with body mass index between 27 and 32 kg/m 2 : A 5-year randomized controlled trial. Diabetes Res. Clin. Pract. 188 , 109900 (2022).
Chooi, Y. C., Ding, C. & Magkos, F. The epidemiology of obesity. Metabolism 92 , 6–10 (2019).
Christensen, S. Recognizing obesity as a disease. J. Am. Assoc. Nurse Pract. 32 , 497–503 (2020).
Ferrulli, A. et al. Weight loss induced by deep transcranial magnetic stimulation in obesity: A randomized, double-blind, sham-controlled study. Diabetes Obes. Metab. 21 , 1849–1860 (2019).
Twells, L. K. et al. Nonsurgical weight loss interventions: A systematic review of systematic reviews and meta-analyses. Obes. Rev. 22 , e13320 (2021).
Rosa-Gonçalves, P. & Majerowicz, D. Pharmacotherapy of obesity: Limits and perspectives. Am. J. Cardiovasc. Drugs 19 , 349–364 (2019).
Pi-Sunyer, X. et al. A randomized, controlled trial of 3.0 mg of liraglutide in weight management. N. Engl. J. Med. 373 , 11–22 (2015).
Knudsen, L. B. & Lau, J. The discovery and development of liraglutide and semaglutide. Front. Endocrinol. (Lausanne) 10 , 155 (2019).
de Oca alejandra PZMTS, PelliTero S, PUig-DoMingo M. obesity and glP-1. Minerva Endocrinology 46 , 168–176 (2021).
Kahn, S. E., Hull, R. L. & Utzschneider, K. M. Mechanisms linking obesity to insulin resistance and type 2 diabetes. Nature 444 , 840–846 (2006).
Article ADS CAS PubMed Google Scholar
Rubio-Almanza, M., Cámara-Gómez, R. & Merino-Torres, J. F. Obesity and type 2 diabetes: Also linked in therapeutic options. Endocrinol. Diabetes Nutr. 66 , 140–149 (2019).
Wharton, S. et al. Two-year effect of semaglutide 2.4 mg on control of eating in adults with overweight/obesity: STEP 5. Obesity 31 , 703–715 (2023).
Wilkinson, L. et al. Effect of semaglutide 2.4 mg once weekly on 10-year type 2 diabetes risk in adults with overweight or obesity. Obesity 31 , 2249–2259 (2023).
Frías, J. P. et al. Tirzepatide versus semaglutide once weekly in patients with type 2 diabetes. N. Engl. J. Med. 385 , 503–515 (2021).
Son, J. W. & Kim, S. Comprehensive review of current and upcoming anti-obesity drugs. Diabetes Metab. J. 44 , 802–818 (2020).
Ballinger, A. & Peikin, S. R. Orlistat: Its current status as an anti-obesity drug. Eur. J. Pharmacol. 440 , 109–117 (2002).
Zhou, Y. H. et al. Effect of anti-obesity drug on cardiovascular risk factors: A systematic review and meta-analysis of randomized controlled trials. PLoS One 7 , e39062 (2012).
Article ADS CAS PubMed PubMed Central Google Scholar
Cosentino, G., Conrad, A. O. & Uwaifo, G. I. Phentermine and topiramate for the management of obesity: A review. Drug Des. Dev. Ther. 7 , 267–278 (2013).
CAS Google Scholar
Smith, S. M., Meyer, M. & Trinkley, K. E. Fentermina/topiramato (qsymia) para el tratamiento de obesidad. Ann. Pharmacother. 47 , 340–349 (2013).
Sharma, B. & Henderson, D. C. Sibutramine: Current status as an anti-obesity drug and its future perspectives. Expert Opin. Pharmacother. 9 , 2161–2173 (2008).
Tziomalos, K., Krassas, G. E. & Tzotzas, T. The use of sibutramine in the management of obesity and related disorders: An update. Vasc. Health Risk Manag. 5 , 441–452 (2009).
CAS PubMed PubMed Central Google Scholar
Burguera, B. et al. Critical assessment of the current guidelines for the management and treatment of morbidly obese patients. J. Endocrinol. Invest. 30 , 844–852 (2007).
Grundy, S. M. et al. Gastrointestinal surgery for severe obesity. Ann. Intern. Med. 115 , 956–961 (1991).
Abu Dayyeh, B. K. et al. Endoscopic sleeve gastroplasty for treatment of class 1 and 2 obesity (MERIT): A prospective, multicentre, randomised trial. The Lancet 400 , 441–451 (2022).
Chapman, A. E. et al. Laparoscopic adjustable gastric banding in the treatment of obesity: A systematic literature review. Surgery 135 , 326–351 (2004).
Himpens, J. et al. Long-term outcomes of laparoscopic adjustable gastric banding. Arch. Surg. 146 , 802–807 (2011).
Colquitt, J. L., Pickett, K., Loveman, E. & Frampton, G. K. Surgery for weight loss in adults. Cochrane Database Syst. Rev. 2014 , CD003641 (2014).
Cotugno, M. et al. Clinical efficacy of bariatric surgery versus liraglutide in patients with type 2 diabetes and severe obesity: A 12-month retrospective evaluation. Acta Diabetol. 52 , 331–336 (2014).
Tan, Q. et al. Recent advances in incretin-based pharmacotherapies for the treatment of obesity and diabetes. Front. Endocrinol. (Lausanne) https://doi.org/10.3389/fendo.2022.838410 (2022).
Wang, L. et al. The impact of bariatric surgery versus non-surgical treatment on blood pressure: Systematic review and meta-analysis. Obes. Surg. 31 , 4970–4984 (2021).
Schiavon, C. A. et al. Three-year outcomes of bariatric surgery in patients with obesity and hypertension. Ann. Intern. Med. 173 , 685–693 (2020).
Currie, A. C., Askari, A., Fangueiro, A. & Mahawar, K. Network meta-analysis of metabolic surgery procedures for the treatment of obesity and diabetes. Obes. Surg. 31 , 4528–4541 (2021).
Ikramuddin, S. et al. Lifestyle intervention and medical management with vs without roux-en-y gastric bypass and control of hemoglobin a1c, ldl cholesterol, and systolic blood pressure at 5 years in the diabetes surgery study. JAMA J. Am. Med. Assoc. 319 , 266–278 (2018).
Hossain, N., Arhi, C. & Borg, C. M. Is bariatric surgery better than nonsurgical weight loss for improving asthma control? A systematic review. Obes. Surg. 31 , 1810–1832 (2021).
Järvholm, K. et al. Metabolic and bariatric surgery versus intensive non-surgical treatment for adolescents with severe obesity (AMOS2): A multicentre, randomised, controlled trial in Sweden. Lancet Child Adolesc. Health 7 , 249–260 (2023).
Verrastro, O. et al. Bariatric–metabolic surgery versus lifestyle intervention plus best medical care in non-alcoholic steatohepatitis (BRAVES): A multicentre, open-label, randomised trial. The Lancet 401 , 1786–1797 (2023).
Courcoulas, A. P. et al. Reduction in long-term mortality after sleeve gastrectomy and gastric bypass compared to nonsurgical patients with severe obesity. Ann. Surg. 277 , 442–448 (2023).
Stenberg, E. & Näslund, E. Major adverse cardiovascular events among patients with type-2 diabetes, a nationwide cohort study comparing primary metabolic and bariatric surgery to GLP-1 receptor agonist treatment. Int. J. Obes. 47 , 251–256 (2023).
Article CAS Google Scholar
Bjornstad, P. et al. Effect of surgical versus medical therapy on diabetic kidney disease over 5 years in severely obese adolescents with type 2 diabetes. Diabetes Care 43 , 187–195 (2020).
Boyers, D. et al. Cost-effectiveness of bariatric surgery and non-surgical weight management programmes for adults with severe obesity: A decision analysis model. Int. J. Obes. 45 , 2179–2190 (2021).
Download references
Acknowledgements
The authors are thankful to Justin Axel-Berg for the English corrections and Rossana V. Mendoza López for the statistical analysis.
Author information
Authors and affiliations.
Department of Neurology, Hospital das Clínicas HCFMUSP, Faculdade de Medicina, Universidade de Sao Paulo, São Paulo, SP, Brazil
Leonardo Zumerkorn Pipek
Faculty of Medicine FMUSP, University of São Paulo, São Paulo, Brazil
Walter Augusto Fabio Moraes, Rodrigo Massato Nobetani, Vitor Santos Cortez, Alberto Santos Condi, João Victor Taba, Milena Oliveira Suzuki, Fernanda Sayuri do Nascimento & Vitoria Carneiro de Mattos
Centro Universitário FMABC, Santo André, São Paulo, Brazil
Rafaela Farias Vidigal Nascimento
Center of Acupuncture, Department of Orthopaedics and Traumatology, University of São Paulo, São Paulo, Brazil
Leandro Ryuchi Iuamoto & Wu Tu Hsing
Department of Gastroenterology, Hospital das Clínicas, HCFMUSP, Avenida Doutor Arnaldo, 455, São Paulo, Brazil
Luiz Augusto Carneiro-D’Albuquerque, Alberto Meyer & Wellington Andraus
You can also search for this author in PubMed Google Scholar
Contributions
Conceptualization: L.Z.P., A.M. Methodology: L.Z.P., L.R.I., A.M. Formal analysis: L.Z.P., R.F.V.N., A.M. Data Curation: L.Z.P., W.A.F.B., R.M.N., V.S.C., A.S.C., J.V.T., R.F.V.N., M.O.S., F.S.N., V.C.M. Writing—Original Draft: L.Z.P., W.A.F.B., R.M.N., V.S.C., A.S.C., J.V.T., R.F.V.N., M.O.S., F.S.N., V.C.M. Writing—Review and Editing: L.Z.P., R.F.V.N., L.R.I., W.T.H., L.A.C., A.M., W.A. Visualization: L.Z.P., R.F.V.N., A.M. Supervision: L.R.I., W.T.H., L.A.C., A.M., W.A. Project administration: L.R.I., W.T.H., L.A.C., A.M., W.A.
Corresponding author
Correspondence to Alberto Meyer .
Ethics declarations
Competing interests.
The authors declare no competing interests.
Additional information
Publisher's note.
Springer Nature remains neutral with regard to jurisdictional claims in published maps and institutional affiliations.
Rights and permissions
Open Access This article is licensed under a Creative Commons Attribution 4.0 International License, which permits use, sharing, adaptation, distribution and reproduction in any medium or format, as long as you give appropriate credit to the original author(s) and the source, provide a link to the Creative Commons licence, and indicate if changes were made. The images or other third party material in this article are included in the article's Creative Commons licence, unless indicated otherwise in a credit line to the material. If material is not included in the article's Creative Commons licence and your intended use is not permitted by statutory regulation or exceeds the permitted use, you will need to obtain permission directly from the copyright holder. To view a copy of this licence, visit http://creativecommons.org/licenses/by/4.0/ .
Reprints and permissions
About this article
Cite this article.
Pipek, L.Z., Moraes, W.A.F., Nobetani, R.M. et al. Surgery is associated with better long-term outcomes than pharmacological treatment for obesity: a systematic review and meta-analysis. Sci Rep 14 , 9521 (2024). https://doi.org/10.1038/s41598-024-57724-5
Download citation
Received : 19 November 2023
Accepted : 21 March 2024
Published : 25 April 2024
DOI : https://doi.org/10.1038/s41598-024-57724-5
Share this article
Anyone you share the following link with will be able to read this content:
Sorry, a shareable link is not currently available for this article.
Provided by the Springer Nature SharedIt content-sharing initiative
- Long term outcome
- Pharmacological treatment
By submitting a comment you agree to abide by our Terms and Community Guidelines . If you find something abusive or that does not comply with our terms or guidelines please flag it as inappropriate.
Quick links
- Explore articles by subject
- Guide to authors
- Editorial policies
Sign up for the Nature Briefing newsletter — what matters in science, free to your inbox daily.

- Open access
- Published: 27 April 2024
Obesity-induced blood-brain barrier dysfunction: phenotypes and mechanisms
- Ziying Feng 1 , 2 ,
- Cheng Fang 1 ,
- Yinzhong Ma 1 , 3 &
- Junlei Chang 1 , 2 , 3
Journal of Neuroinflammation volume 21 , Article number: 110 ( 2024 ) Cite this article
529 Accesses
19 Altmetric
Metrics details
Obesity, a burgeoning global health issue, is increasingly recognized for its detrimental effects on the central nervous system, particularly concerning the integrity of the blood-brain barrier (BBB). This manuscript delves into the intricate relationship between obesity and BBB dysfunction, elucidating the underlying phenotypes and molecular mechanisms. We commence with an overview of the BBB’s critical role in maintaining cerebral homeostasis and the pathological alterations induced by obesity. By employing a comprehensive literature review, we examine the structural and functional modifications of the BBB in the context of obesity, including increased permeability, altered transport mechanisms, and inflammatory responses. The manuscript highlights how obesity-induced systemic inflammation and metabolic dysregulation contribute to BBB disruption, thereby predisposing individuals to various neurological disorders. We further explore the potential pathways, such as oxidative stress and endothelial cell dysfunction, that mediate these changes. Our discussion culminates in the summary of current findings and the identification of knowledge gaps, paving the way for future research directions. This review underscores the significance of understanding BBB dysfunction in obesity, not only for its implications in neurodegenerative diseases but also for developing targeted therapeutic strategies to mitigate these effects.
Introduction
Obesity, characterized by excessive fat accumulation, represents a burgeoning health issue of global populations. The World Health Organization reports an alarming rise in obesity rates worldwide, with an estimated 2.5 billion adults being overweight (body mass index, BMI > 25), of which approximately 890 million are obese (BMI > 30) as of 2022 [ 1 ]. This pervasive phenomenon profoundly impacts human health, inciting a multitude of disorders including cardiovascular disease, stroke, diabetes, and certain types of cancer.
Of particular interest, however, is the intricate relationship between obesity and neurological disorders, a link that has increasingly captivated scientific attention. Alzheimer’s disease [ 2 , 3 , 4 ] and stroke [ 5 , 6 , 7 ], for instance, are two representative neurological disorders that have been recurrently associated with obesity. Recent evidence points to obesity as a critical risk factor for mitochondrial dysfunction, as astrocytic fatty acid oxidation (FAO) and oxidative phosphorylation (OxPhos) are involved in these lipid-involving neurodegenerative disorders, implicating obesity’s role in influencing the disease onset and progression [ 8 , 9 ]. This escalating prevalence of obesity and its potential implications for neurological disorders presents a grave concern for global health. It necessitates a comprehensive understanding of obesity’s detrimental consequences towards the central nervous system (CNS), specifically its effects on the cerebral vascular system.
A critical component of the cerebrovascular system is the blood-brain barrier (BBB), a critical function of brain blood microvessels that maintains CNS homeostasis. The BBB strictly regulates the exchange of substances between the blood and the brain. This dynamic barrier ensures the stability of brain microenvironment, protects against neurotoxins, and facilitates proper neuronal function [ 10 , 11 ]. However, in pathological states such as obesity, this pivotal barrier can be compromised, leading to various neurological disorders. Understanding the intricate relationship between obesity and BBB dysfunction therefore becomes a matter of paramount importance.
We aim to delve into the complexities of this relationship, exploring the phenotypes and underlying mechanisms of BBB dysfunction in obesity. Through a comprehensive examination of the current literature, we provide an in-depth analysis of the potential changes in BBB structure and function induced by obesity. Additionally, we elucidate the mechanistic pathways that may be implicated in BBB dysfunction in obesity. This review ultimately seeks to underscore the significance of understanding BBB dysfunction in obesity and its implications for neurological health, with the goal of guiding future research and clinical practice.
Blood-brain barrier: cellular and molecular components
The blood-brain barrier (BBB) is a sophisticated and highly specialized biological construct, known for its selective permeability and protective role within the CNS. It owes its unique functionality to its intricately assembled cellular and molecular components, which work together to maintain CNS homeostasis. The structural and functional foundation of the BBB is the neurovascular unit (NVU) [ 12 ], a complex ensemble encompassing brain endothelial cells (BECs), pericytes, astrocytes, extracellular matrix (ECM), microglia, and neurons (Fig. 1 ).
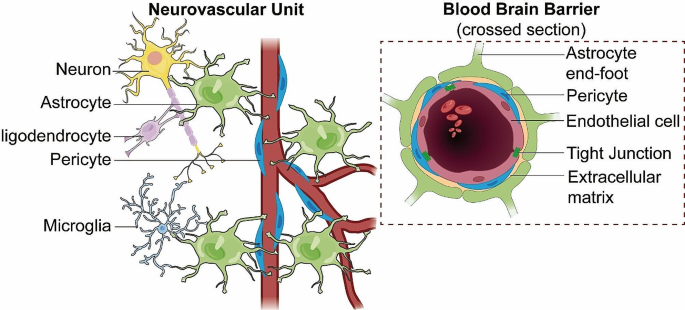
BBB integrated within the NVU . This diagram depicts the structural components of the blood-brain barrier (BBB) within the neurovascular unit (NVU), illustrating the tight junctions of endothelial cells, the supportive pericytes, and the encircling astrocytic end-feet. It highlights the BBB’s integral structural role in the NVU, which is essential for maintaining cerebral integrity and function
Endothelial cells
As the primary component of the BBB, brain endothelial cells (BECs), line the interior surfaces of the brain’s capillaries. These cells are distinctive in their unique morphology and possess attributes that separate them from peripheral ECs [ 13 , 14 , 15 ]. BECs are closely interconnected through tight junctions (TJs), which restrict the paracellular pathway and control the ionic balance across the barrier. This feature is instrumental in preventing the free passage of substances from the blood into the brain. BECs also exhibit limited pinocytic activity and lack fenestrations, thereby further restricting transcellular transport. In order to provide brain with abundant nutrients and a healthy environment, specific transporters which allows for the selective passage of molecules are expressed in BECs. Through analyzing single-cell RNA-sequencing data of mouse ECs from different organs provided by the Tabula Muris consortium, Paik et al. found that BECs possess not only its own unique transcriptomic identities, but also the most specialized differentially expressed genes (DEGs) profiles that expressed primarily solute carrier transporters [ 16 ]. For instance, glucose transporter protein-1(Glut-1) is responsible for transporting glucose, which being a crucial energy fuel, from blood to the brain [ 17 ]. On the other hand, P-glycoprotein (P-gp) acts as an efflux pump that removes harmful substances out of the brain to keep a safe environment [ 18 ].
Worth noticed, BECs can also transport molecules through low rates of transcytosis, a process that plays a vital role in endothelial transport [ 19 , 20 ]. Transcytosis allows for selective or non-selective passage of molecules across the endothelial cells. In BECs, transcytosis can be divided into receptor-mediated (selective) transcytosis, which includes the transport of transferrin and insulin, and bulk-phase or fluid-phase (non-selective) transcytosis, typically exemplified by the transport of albumin [ 21 ]. Notably, while native albumin crosses the BBB via non-selective bulk-phase transcytosis, cationic albumin is transported more efficiently as it engages in adsorptive transcytosis, highlighting the distinct pathways for different forms of albumin. A key regulator in this process is Major Facilitator Superfamily Domain Containing 2 A (Mfsd2a), which specifically inhibits bulk-phase transcytosis in BECs [ 22 ]. Mfsd2a modifies the lipid composition of the cell membrane, thereby limiting the formation of caveolae vesicles and reducing caveolae-mediated transcytosis. This action helps maintain the selective permeability and overall integrity of the BBB. Contrary to inhibiting all forms of transcytosis, Mfsd2a’s role is particularly pivotal in restraining non-selective transcytosis or bulk-phase transcytosis. Research indicates that overexpression of Mfsd2a in CNS endothelial cells leads to a decreased number of transcytotic vesicles, which in turn reduces hematoma levels and alleviates BBB injury in mouse models of intracerebral hemorrhage (ICH) [ 23 ]. This highlights the potential therapeutic value of Mfsd2a in mitigating BBB disruption by specifically targeting non-selective transcytosis mechanisms post-injury.
In comparison to endothelial cells found elsewhere in the body, brain endothelial cells (BECs) demonstrate a unique profile of leukocyte adhesion molecule (LAM) expression. This profile contributes to the CNS’s immune privilege by limiting the extravasation of leukocytes under normal conditions. While LAMs are integral to leukocyte adhesion and migration — key steps in the immune response — their regulated expression in BECs ensures a controlled interaction with leukocytes, thereby maintaining CNS homeostasis [ 24 ]. Contrary to the constant expression in peripheral tissues, the presence and activity of LAMs in the BBB vary contextually. Notably, BECs typically lack selectins in their Weibel-Palade bodies, which reduces their ability for rapid leukocyte recruitment compared to peripheral endothelial cells. This does not equate to a complete suppression of immune response but represents a finely tuned regulation ensuring selective leukocyte passage without compromising BBB integrity [ 25 ]. During certain pathological conditions, leukocytes such as neutrophils and T cells may interact with the BBB in ways that can affect its permeability, through the release of cytokines, reactive oxygen species, and other mediators. However, these interactions do not universally result in BBB disruption and can occur in the absence of significant leakage, depending on the specific disease context and the nature of leukocyte engagement. In the context of neuroinflammatory diseases, such as multiple sclerosis (MS), alterations in the expression and functionality of LAMs have been observed. These changes can facilitate increased adhesion of leukocytes to BECs, potentially leading to BBB disruption under these pathological states [ 26 ]. However, this process reflects a departure from the normative regulatory mechanisms in place under healthy conditions and underscores the complex role of LAMs in both maintaining CNS immunity and contributing to neuroinflammation when dysregulated.
Pericytes is a kind of multi-functional cells embedded within the walls of capillaries throughout the body, including the brain, and play a crucial role in maintaining the integrity of the BBB. They were first identified in the 1870s, and more recently, numerous vascular functions of pericytes have been identified. These include regulation of cerebral blood flow, maintenance of the BBB integrity, and control of vascular development and angiogenesis [ 27 , 28 , 29 ]. In addition to these roles, pericytes have been found to play an active role during neuroinflammation in the adult brain [ 28 , 30 , 31 ]. They can respond differentially, depending on the degree of inflammation, by secreting a set of neurotrophic factors to promote cell survival and regeneration, or by potentiating inflammation through the release of inflammatory mediators (e.g., cytokines and chemokines), and the overexpression of pattern recognition receptors [ 32 ]. In neuroinflammatory conditions like multiple sclerosis, pericytes undergo morphological changes, elongating their processes within inflamed perivascular cuffs [ 33 ]. Exposure to cytokines and extracellular matrix proteins like chondroitin sulfate proteoglycans enhances pericyte secretion of chemokines and promotes macrophage migration [ 33 ]. This implicates pericytes in propagating neuroinflammation through immune cell recruitment. However, pericytes also have neuroprotective capacities dependent on the degree of inflammation [ 34 ]. Through release of neurotrophic factors like BDNF, pericytes can promote neuronal survival and regeneration [ 35 ]. Their expression of cell adhesion molecules likewise facilitates interactions with endothelial cells and astrocytes to maintain cerebrovascular stability [ 36 ]. The dual functionality of pericytes is highlighted by their differential secretome profiles in response to IL-1β [ 37 ]. While pericytes secrete certain pro-inflammatory genes like CCL2 [ 38 ], they also show expression of vascular-stabilized mediators like TIMP3 [ 34 , 39 ]. This nuanced, context-dependent pericyte reactivity fine-tunes neuroinflammatory responses.
Pericyte dysfunction is increasingly recognized as a contributor to the progression of vascular diseases such as stroke and neurodegenerative diseases [ 40 , 41 ]. The therapeutic potential of pericytes to repair cerebral blood vessels and promote angiogenesis due to their ability to possess stem cell-like properties has recently been brought to light. In the context of cerebral blood vessels repair, pericytes can migrate to the site of injury and differentiate into cells that are needed for repair [ 42 , 43 ]. As for promoting angiogenesis, pericytes play a vital role in the formation of new blood vessels from pre-existing ones, a process known as angiogenesis [ 44 ]. They stabilize the newly formed endothelial tubes, modulate blood flow and vascular permeability, and regulate endothelial proliferation, differentiation, migration and survival. However, research has shown that pericytes and endothelial cells have overlapping but distinct secretome profiles in response to IL-1β [ 45 ]. This indicates that these two cell types may respond differently to inflammatory stimuli, which could have implications for understanding how inflammation affects the cerebrovasculature.
In conclusion, pericytes play a critical role in maintaining BBB integrity by controlling various processes. Understanding these mechanisms could provide valuable insights into BBB function and CNS immunity. Future research directions could include exploring the role of other proteins involved in these processes, further investigating the function of pericytes in disease states, and studying how changes in pericyte function could impact BBB integrity and CNS health.
Astrocytes, another critical component of the NVU, also play a crucial role in BBB maintenance. Their end-foot processes enwrap the brain capillaries and provide physical support to the BBB. Through their extensive contact with endothelial cells, astrocytes are vital in the formation and maintenance of the BBB. More specifically, astrocytes can secrete Sonic hedgehog (Shh), thereby stimulating the expression of TJs proteins and junctional adhesion molecule-A (JAM-A) while promoting immune quiescence of BBB by decreasing the expression of chemokines and LAMs [ 46 ]. The Hedgehog (Hh) pathway is involved in embryonic morphogenesis, neuronal guidance, and angiogenesis [ 47 ]. In adult tissues, it participates in vascular proliferation, differentiation, and tissue repair. The Hh pathway provides a barrier-promoting effect and an endogenous anti-inflammatory balance to CNS-directed immune attacks [ 48 ]. In terms of cerebrovascular accidents, astrocytes have been found to play a crucial role in maintaining blood-brain barrier function [ 49 ]. They support neurons and other glia, and react to changes in both the local and external environment. Beyond these homeostatic functions, astrocytes can respond to several stimuli and subsequently display profound genetic, morphological, and functional changes in a process termed reactive astrogliosis.
In addition to their role in maintaining BBB integrity, astrocytes also play a crucial role in controlling water homeostasis in the brain. Aquaporins (AQPs) are transmembrane proteins responsible for fast water movement across cell membranes, including those of astrocytes [ 50 ]. Various subtypes of AQPs (AQP1, AQP3, AQP4, AQP5, AQP8 and AQP9) have been reported to be expressed in astrocytes. The expressions and subcellular localizations of AQPs in astrocytes are highly correlated with both their physiological and pathophysiological functions [ 51 , 52 ].
Basement membrane
The basement membrane (BM) was secreted and maintained by BECs, pericytes and astrocytes. The BM is composed of various proteins, including Laminins, Collagen-IV, Perlecan, and Fibronectin [ 53 , 54 ]. These components interact with each other and with other cells at the BBB. For example, Laminins provide structural support to the BBB and are involved in processes such as cell adhesion, differentiation, migration, and apoptosis, while Collagen-IV provides mechanical strength to the BBB and contributes to its stability [ 53 , 54 ]. Any alterations in these components can lead to BBB dysfunction, which is associated with various neurological disorders. For instance, in conditions such as stroke, the BBB undergoes significant changes, including alterations in the expression of integrins and degradation of surrounding ECM, which indirectly affect the vascular barrier function [ 55 ]. In an animal model of autoimmunity, considerable information has been revealed about the function of different BMs in maintaining BBB integrity and causing neuroinflammation [ 56 , 57 , 58 ].
ECM receptors, such as integrins and dystroglycan, are also expressed at the brain microvasculature and mediate the connections between cellular and matrix components in physiology and disease [ 59 , 60 , 61 ]. These proteins and receptors elicit diverse molecular signals that allow cell adaptation to environmental changes and regulate growth and cell motility [ 62 ].
Microglia and neurons
Finally, microglia and neurons, while not directly forming the BBB, significantly contribute to its function and maintenance. Microglia, the resident immune cells of the brain, play a crucial role in maintaining the integrity of the BBB. Following ischemic stroke, microglia interact with endothelial cells in a paracrine manner to promote angiogenesis and barrier repair [ 63 ]. However, this reparative crosstalk is impeded in aging, implicating declined microglial function in age-related BBB disruption [ 64 ]. Accordingly, experimental depletion of microglia exacerbates injury and edema in aged mice subjected to ischemic stroke, further confirming microglia’s protective influence [ 65 ]. Beyond strokes, microglia also maintain BBB stability in neuroinflammatory conditions by modulating astrocyte reactivity and phagocytosing synapses [ 66 ]. In a model of multiple sclerosis, microglia limited astrocyte activation and protected against BBB leakage, stressing their homeostatic role [ 67 ]. During reactive gliosis induced by stroke, microglia and macrophages preferentially eliminated excitatory synapses while astrocytes cleared inhibitory synapses, preventing excessive imbalance of excitatory/inhibitory tone [ 67 ].These findings underscore diverse mechanisms whereby microglia maintain cerebrovascular equilibrium, ranging from paracrine support of angiogenesis to controlled synapse engulfment. Their protective influence likely declines with aging, permitting BBB hyperpermeability. Boosting microglial function represents a promising strategy to strengthen BBB integrity in neurological disorders.
Neurons also play a significant role in BBB’s function and maintenance. Neurons communicate with other components of the neurovascular unit (NVU), such as endothelial cells, pericytes, and astrocytes, to regulate BBB properties. This communication is also critical for maintaining the CNS homeostasis and for responding to changes in neural activity. In the early postnatal mouse barrel cortex, it was demonstrated that manipulations of sensory inputs resulted in vascular structural changes [ 68 ]. Specifically, this study showed that local sensory-related neural activity promoted the formation of cerebrovascular networks.
Neurovascular coupling refers to the relationship between local neural activity and subsequent changes in cerebral blood flow (CBF) [ 69 ]. The magnitude and spatial location of blood flow changes are tightly linked to changes in neural activity through a complex sequence of coordinated events involving neurons, glia, and vascular cells. This mechanism is crucial as it matches the high energy demand of the brain with a supply of energy substrates from the blood. Evoked the neural activity by high-intensity visual stimulation could drive macroscopic cerebrospinal fluid (CSF) flow in the human brain [ 70 ]. The timing and amplitude of CSF flow were matched to the visually evoked hemodynamic responses, suggesting neural activity can modulate CSF flow via neurovascular coupling.
In addition to their role in neurovascular coupling, neurons also contribute to BBB function through some transporters. It was found that neuronal activity regulates BBB efflux transporter expression and function [ 71 ], which is critical for excluding many small lipophilic molecules from the brain parenchyma. These findings suggest that sensory-related neural activity can influence both vascular structure and BBB function, which could have significant implications for understanding neuro-vascular interaction. An understanding of these intricate structures and their functions is pivotal in comprehending how pathological states, such as obesity, could affect the BBB and ultimately lead to neurological disorders.
Obesity-induced BBB leakage: phenotypes and mechanisms
The impact of obesity on BBB integrity has been an area of increasing scientific scrutiny, given the relationship between obesity and various neurological disorders. The disruption of BBB integrity or non-specific leakage of the BBB, as it is commonly referred to, is a recurrent phenotype observed in obesity, especially in the context of high-fat diet (HFD) intake with a wide duration from 8 to 36 w [ 72 , 73 , 74 , 75 ]. This section seeks to elucidate the molecular mechanisms and phenotypic changes underlying obesity-induced BBB leakage and associated BBB markers of this pathological state.
Changes in tight junctions
A key factor implicated in obesity-induced BBB leakage is the dysregulation of tight junction proteins (TJs). TJs, as earlier stated, are critical in maintaining the restrictive properties of the BBB. However, obesity, particularly under conditions of HFD, can alter the expression and function of these proteins. For instance, Ouyang et al. observed alterations in the expression levels of ZO-1 in microvessels from obese mice modeling by 8 weeks (w) of HFD [ 76 ]. In line with this observation, HFD significantly decreased the protein levels of ZO-1, Claudin-5 and Occludin along with the leakage of brain microvessles after 8 weeks (w) of HFD [ 77 ]. In adult rats, 90 days of high-energy diet (high in saturated fat and glucose) consumption decreased mRNA expression of TJs, particularly Claudin-5 and − 12, in the choroid plexus and the BBB. Consequently, an increased blood-to-brain permeability of sodium fluorescein was observed in the hippocampus [ 78 ]. This underscores the potential for obesity-induced modifications to BBB structure and function.
The adenosine receptor 2a (Adora2a) is increasingly recognized for its significant role in the modulation of neurovascular and neuroinflammatory responses. Activation of Adora2a receptors has been linked to heightened inflammatory responses, contributing to the disruption of the blood-brain barrier (BBB) and subsequent neuronal damage, conditions often exacerbated by obesity and metabolic syndrome. In a rodent model of diet-induced insulin resistance by 16 w of HFD, it was found that chronic activation of Adora2a eroded TJs between BECs, as evidenced by diminished Occludin and Claudin-5 in hippocampal lysates. Considering the detrimental effects associated with Adora2a activation on BBB integrity, antagonism of this receptor presents a promising therapeutic strategy. By inhibiting Adora2a, it is possible to mitigate the receptor-mediated exacerbation of inflammatory processes within the CNS, thereby preserving BBB function and reducing neuroinflammatory sequelae. This premise is supported by several studies demonstrating that Adora2a antagonists can effectively reduce BBB permeability and alleviate inflammatory damage in various neuroinflammatory and neurovascular disorders [ 79 , 80 , 81 ].
In addition, it is noteworthy that obesity not only affects one’s own BBB function, but also has an impact on its offspring. A recent study showed that maternal obesity during pregnancy could impaired BBB formation of the fetal, leading to changes in TJ components of the arcuate nucleus region in offspring’s brain, thereby significantly increasing BBB permeability [ 82 ]. This dysregulation of TJs compromises the integrity of the BBB, increasing its permeability and enabling the passage of potentially harmful substances from the blood into the CNS. Interestingly, recent studies have also linked prolonged HFD intake for 32 w to anxiety-like and depression-like behaviors in mice [ 83 ]. It was found that 24 weeks of HFD consumption induced neurobehavioral deterioration, including increased anxiety-like and depression-like behavior. These behavioral changes were associated with impaired gut microbiota homeostasis and inflammation. Long term HFD may induce certain behavioral phenotypes related to neurological disorders through the gut-brain axis [ 83 ]. In line with this, treatment with the anti-inflammatory molecule palmitoylethanolamide was found to reduce anxiety-like behavior in obese mice modeling by 19 w of HFD, along with dampening systemic and central inflammation [ 84 ]. Taken together, these studies suggest prolonged HFD may not only directly disrupt TJs and BBB integrity through inflammatory and other mechanisms, but also trigger neurobehavioral changes that could secondarily impact BBB function.
Changes in fenestration
As mentioned above, BECs are characterized by a lack of fenestrations, a characteristic that contributes to the high selectivity and restrictive nature of the BBB. Stan et al. identified PV-1 (also known as PLVAP, plasmalemma vesicle-associated protein; or MEGA-32 antigen) as a component of fenestral diaphragms in endothelial cells [ 85 ]. As PV-1 comprises these structures, its regulation could influence fenestration numbers. While PLVAP expressed on fenestrated endothelia and associated with the formation of diaphragms in vesicular structures, it does not serve as a marker for fenestrations within the BBB context. Instead, increases in PLVAP expression may reflect alterations in the molecular composition associated with transcellular pathways rather than the formation of true fenestrae. In a single-cell profiling study, analysis of BECs revealed that among eight major clusters, fenestrated BECs in areas such as the choroid plexus showed the most unique obesity-induced DEGs, where fenestrated endothelia are typical, rather than suggesting the emergence of fenestrations within the BBB due to obesity modeling by 12 w of HFD [ 86 ]. In line with this, Previous study have demonstrated that HFD intake can lead to an increase in endothelial fenestration in the BBB, such as the observed changes in the offspring of gestational obesity in mice [ 82 ]. Worth noticed, these findings, particularly those related to increased permeability in regions like the arcuate nucleus, may reflect localized alterations in BBB properties rather than systemic induction of fenestrations akin to those in inherently fenestrated structures like the choroid plexus.
Therefore, while the effects of HFD on endothelial cell biology are undeniable, the notion that these effects lead to the formation of fenestrations within the typical BBB structure remains debatable. This perspective allows for the possibility that PLVAP-regulated alterations may occur regionally within specific areas of the brain under certain conditions, rather than implying a global change across the entire barrier. The DEGs identified in obesity models, particularly those affecting endothelial cells of the BBB, should be interpreted with an understanding that obesity-induced stress may lead to changes in molecular signaling and barrier properties without necessitating the creation of actual fenestral openings.
Changes in matrix metalloproteinases
Matrix metalloproteinases (MMPs) are a family of endopeptidases that function to degrade and remodel the extracellular matrix (ECM). MMPs are secreted by various cell types including epithelial cells, fibroblasts, and inflammatory cells, playing important roles in physiological processes as well as disease states characterized by tissue damage and inflammation. However, excessive MMPs can lead to pathological ECM degradation and impairment of tissue structure and function, as in the case of blood-brain barrier (BBB) disruption by MMP-2 and MMP-9 [ 87 , 88 , 89 ]. Obesity has been linked to increased expression and activity of certain MMPs, which can impair BBB integrity. For example, plasma MMP-9 levels were found to be elevated in obese subjects and decreased with anti-diabetic treatment [ 90 , 91 ]. Cotemporally, lipocalin-2 was also increased in adipose tissue of obese individuals and correlated with MMP-2 and MMP-9 activity [ 92 ]. MMP-8 levels were similarly increased in obesity and associated with insulin resistance [ 93 ]. Additional studies have assessed levels of MMPs like MMP-2 and MMP-9 along with their inhibitors TIMPs in obese children [ 94 ]. Together, these findings suggest obesity creates a pro-inflammatory state characterized by upregulation of MMPs like MMP-8 and MMP-9, potentially driven by increases in mediators like lipocalin-2. By degrading ECM proteins, these MMPs can impair BBB structural integrity. A general description of obesity-induced BBB leakage was shown in Fig. 2 .
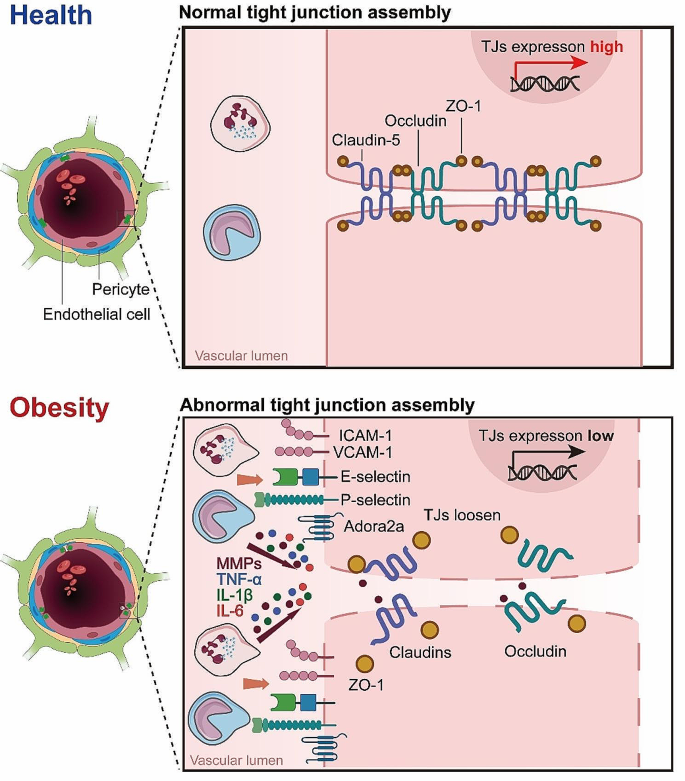
Mechanisms of Obesity-Induced BBB Leakage. Illustration of the complex alterations in the BBB induced by obesity, highlighting the loosening of endothelial cell junctions, increased permeability, and the unusual occurrence of fenestrations. These structural changes are further exacerbated by the activity of MMPs, which degrade extracellular matrix components, facilitating the infiltration of peripheral immune cells, such as neutrophils. This cascade of events underscores the multifaceted and interconnected impact of obesity on BBB integrity and the resulting immune response within the cerebral vasculature
Obesity-induced BBB transport dysfunction: phenotypes and mechanisms
In addition to disrupting the BBB integrity, obesity is associated with alterations in the BBB’s transport functions. These changes not only affect the nutrient supply to the brain but can also influence the entry and clearance of toxins, signaling molecules, and therapeutics. The transport across the BBB is highly regulated, with specific receptors and transporters expressed in BECs mediating the process. However, obesity can induce significant changes in these receptors and transporters, leading to BBB transport dysfunction.
Glucose transport-1 (Glut-1)
One of the key alterations in BBB transport function under obesity conditions is the dysregulation of glucose transport. The primary transporter responsible for glucose transport across the BBB is the glucose transporter 1 (Glut-1), which ensures the supply of glucose from the blood to the brain. However, in the state of obesity, particularly in HFD-induced obesity, the expression and function of Glut-1 have been found to be altered. Specifically, studies in mouse models have demonstrated that acute high-fat feeding for 2–3 days suppressed Glut-1 expression and glucose uptake in the brain [ 95 , 96 ]. Similarly, the mouse insulin resistance model showed that when 2 weeks of HFD significantly downregulated the expression of Glut-1 in the brain, 10 weeks of HFD normalized the expression of Glut-1, suggesting the duration of insulin resistance may influenced the regulation of Glut-1 [ 97 ]. In humans, genetic factors were found to impact Glut-1 levels, which in turn modulates cognitive effects of high-fat intake [ 98 ].
Interestingly, upon more prolonged high-fat feeding for 10 weeks, Glut-1 expression was restored, which was related to the initiation of compensatory mechanisms of vascular endothelial growth factor (VEGF), a key regulator of angiogenesis and vascular function [ 95 ]. Recent studies have illuminated the role of VEGF in respond to metabolic stresses and hypoxic conditions often prevalent in obesity. This response includes the potential to upregulate Glut-1 expression, ostensibly to compensate for altered metabolic demands and ensure sufficient glucose transport across the BBB. However, the interplay between VEGF and Glut-1 in the setting of obesity is multifaceted. While moderate increases in VEGF can be beneficial, aiding in the restoration of Glut-1 levels and maintaining cerebral glucose metabolism, chronic elevations—common in prolonged obesity—may lead to adverse effects [ 95 , 96 ]. Excessive VEGF can contribute to vascular abnormalities and exacerbate BBB disruption, complicating the metabolic landscape of the CNS. Therefore, understanding the dynamic between VEGF-induced Glut-1 modulation and obesity provides insight into the broader implications of metabolic syndrome on BBB health and brain metabolism. This section explores how the nuanced changes in VEGF and Glut-1 expression influenced by obesity underscore the complexity of maintaining BBB integrity and highlight the need for targeted therapeutic strategies to address these metabolic challenges.
Taken together, while short-term high-fat intake appears to impair Glut-1 function and glucose transport at the BBB, compensation may occur with prolonged obesity to normalize Glut-1 expression. However, the brain is the most energy-consuming organ, requiring constant high energy to maintain its function, thereby the temporary disruption in glucose delivery to the brain may be sufficient to impact neuronal health and cognition. Further research into the kinetics of Glut-1 regulation in response to high-fat feeding could delineate the timeframe of BBB transport deficits. This may provide insights into critical windows where impaired brain glucose uptake contributes to neurological disorders associated with obesity.
Alterations in insulin transport have also been observed in obesity, which can profoundly impact neuronal function. Insulin enters the brain via saturation transport and binds to insulin receptors (IR) on BECs, triggering receptor autophosphorylation and downstream signaling cascades involved in glucose uptake, metabolism, neuronal plasticity and survival [ 99 ]. However, studies in obese animal models and human subjects indicate that obesity and HFD feeding can impair insulin transport across the BBB. In preclinical studies using mouse and canine models, HFD feeding for 7 w reduced transport of intravenously injected insulin from the circulation into the brain parenchyma [ 100 , 101 ]. This reduction is associated with central insulin resistance, as evidenced by impaired insulin receptor signaling cascades in the brain from mice subjected to 4 w of HFD [ 102 ]. In human clinical studies, obese subjects were found to have lower cerebrospinal fluid (CSF) insulin levels and attenuated CSF insulin increases after systemic insulin infusion compared to healthy controls [ 103 ]. Together, these animal and human studies demonstrate that obesity disrupts brain endothelial insulin transport, reducing insulin delivery to the CNS. This transport dysfunction contributes to central insulin resistance, a phenomenon often linked with cognitive dysfunction and neurological disorders [ 104 ]. Consistence with this, brain insulin resistance has been recognized as an early characteristic of Alzheimer’s disease [ 105 ]. Elucidating the mechanisms by which high-fat feeding alters insulin receptor expression, trafficking and downstream signaling at the BBB will be critical for developing therapeutic strategies to overcome CNS insulin resistance.
In addition to insulin, the transport of the hormone leptin across the BBB is also altered in obesity. Leptin is also transported into the brain via a saturable transport system and binds to leptin receptors on neurons involved in regulating food intake and energy expenditure. However, multiple studies indicate HFD-induced obesity impairs leptin transport across the BBB. For instance, in obese individuals, the transport level of leptin to the brain is downregulated, as evidenced by a significantly lower ratio of the leptin cerebrospinal fluid (CSF)/serum compared to the healthy lean individuals [ 106 , 107 ]. In obese animal models including rodents subjected to 10 w of HFD and sheep subjected to 40 w of HFD, there is a significant decrease in the rate of leptin transport from blood to brain compared to lean controls [ 108 , 109 , 110 ]. Obesity can inhibit the transport of leptin across the BBB, making it impossible for the brain to receive the “satiety signal” emitted by leptin, leading to overeating and worsening of obesity, which may lead to a series of metabolic diseases. Of notice, with the development of obesity, obese mice modeling by 56 days of HFD respond to leptin for central administration (intracerebroventricularly) rather than peripheral administration (intraperitoneally or subcutaneously) [ 111 , 112 ]. This suggests that. the impairment in leptin transport does not appear to be due to altered leptin receptor expression at the BBB. Rather, transport may be inhibited due to saturation of the carrier system and interactions with other circulating factors, such as the high-level triglycerides [ 113 , 114 , 115 ]. Overall, these findings indicate obese states inhibit leptin’s ability to enter the CNS and bind neuronal targets, despite normal BBB leptin receptor levels. Overcoming the transport block could potentiate leptin’s effects on appetite and weight regulation.
P-glycoprotein (P-gp)
Obesity also affects the function of efflux transporters at the BBB, such as the P-glycoprotein (P-gp). P-gp is an ATP-dependent transporter that functions to pump foreign substances and metabolites out of the brain back into the bloodstream. This helps protect the brain from accumulation of potentially toxic compounds. P-gp is encoded by the ABCB1 gene. A human study found a negative correlation between BMI values and the expression levels of ABCB1 in the brain, suggesting P-gp levels are reduced in obesity [ 116 ]. While the mechanisms linking obesity to P-gp regulation require further elucidation, systemic inflammation appears to play a role. In obese pregnant mice, placental P-gp expression was decreased in tandem with increases in inflammatory cytokines like tumor necrosis factor-alpha (TNF-α), interleukin-1 beta (IL-1β), and interleukin-6 (IL-6) [ 117 ]. Changes in P-gp likely impair efflux of substrates from the brain back into circulation. Overall, although there is limited research on obesity and P-gp, current evidence indicates obesity can suppress P-gp expression and function at the BBB, at least in the obese human. This impairment in a key transporter for xenobiotic clearance may enable accumulation of toxins and drugs in the brain.
L-type amino acid transporter-1 (LAT1)
Amino acid transporters are also affected by obesity. The system L amino acid transporter 1 (LAT1) expressed at the BBB is responsible for transport of large neutral amino acids like leucine into the brain. LAT1 plays a key role in regulating mTORC1 signaling, which controls processes like protein synthesis and autophagy [ 118 ]. Recent studies have found that LAT1 function is altered in obesity models. Mice lacking neuronal LAT1 develop obesity phenotypes including increased adiposity [ 119 ]. LAT1 expression and amino acid uptake are reduced in the hypothalamus of obese, diabetic mice [ 120 ]. In humans, lower expression of the related transporter SLC7A10/ASC-1 in adipose tissue is associated with increased visceral fat, insulin resistance, and adipocyte hypertrophy [ 121 ]. Together, these findings indicate obesity impairs the function of multiple amino acid transporters at the BBB and periphery. This likely dysregulates mTORC1 signaling and other nutrient-sensing pathways, contributing to metabolic dysfunction. The alterations of transporters expressed in endothelial cells was summarized in Fig. 3 .
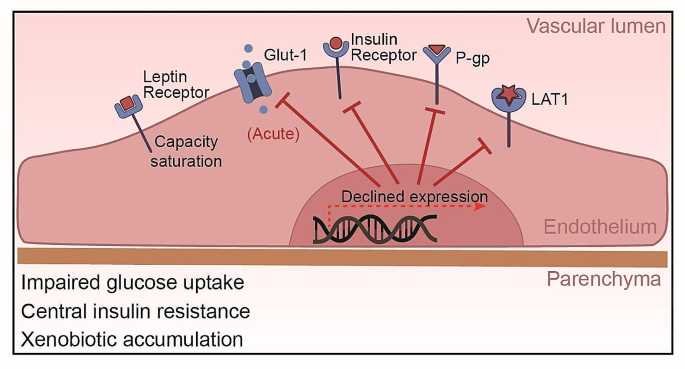
Obesity-Induced Alterations in BBB Transporters. This figure demonstrates the effects of obesity on BBB transporters: Glut-1 function is initially impaired by high-fat intake but may be normalized with prolonged obesity, despite potential impacts on neuronal health and cognition. Brain endothelial insulin transport is disrupted, contributing to central insulin resistance, and often associated with cognitive dysfunction and neurological disorders. Leptin transport impairment is linked to carrier system saturation, independent of leptin receptor expression changes. Obesity suppresses P-glycoprotein expression and function, potentially increasing brain accumulation of toxins and drugs. LAT1 expression and amino acid uptake are reduced in the hypothalamus of obese, diabetic mice, reflecting impaired amino acid transporter function at the BBB.
Evidence of BBB disruption in obesity and type 2 diabetes
Recent evidence has increasingly indicated that metabolic disorders such as obesity and Type 2 Diabetes (T2D) are closely linked to the disruption of the BBB. This section aims to elucidate the relationship between these conditions and BBB integrity, underpinned by recent scientific findings.
BBB Permeability in Type 2 Diabetes: Among the most compelling evidence in this domain is the study conducted by Starr and colleagues [ 122 ], which has demonstrated a significant increase in BBB permeability to gadolinium in patients with well-controlled T2D. This landmark study utilized advanced magnetic resonance imaging techniques to compare BBB integrity between diabetic patients and healthy individuals. The results indicated a pronounced increase in BBB permeability, notably in the basal ganglia region, suggesting a direct impact of T2D on neurovascular integrity. These findings are critical as they highlight the potential for well-controlled T2D to contribute to BBB dysfunction independently of other comorbidities often associated with metabolic syndrome.
The link between obesity and BBB disruption has been further substantiated by studies investigating the levels of specific BBB markers in obese individuals. A longitudinal perspective explores how factors related to adiposity in mid-life can have long-lasting effects on BBB integrity. The study highlights the enduring impact of obesity on neurovascular health, suggesting that the consequences of increased body weight extend far beyond the immediate metabolic disturbances commonly associated with obesity [ 123 ]. Another study focused on Adipsin, a complement system component known to be influenced by adiposity levels, and studied within the cerebrospinal fluid, providing insights into the biochemical pathways through which obesity might mediate changes in BBB integrity. This research suggests a direct link between metabolic health markers and the biochemical status of the BBB, illustrating the intricate connection between systemic metabolic health and neurovascular function [ 124 ]. These studies have shown elevated levels of certain markers, indicating compromised BBB integrity in the context of excessive body weight and associated metabolic derangements. The disruption is believed to be multifactorial, involving mechanisms such as increased systemic inflammation, altered lipid metabolism, and hypertension, all of which are prevalent in obesity and can adversely affect the endothelial cells constituting the BBB.
The mechanistic pathways through which obesity and T2D exacerbate BBB disruption are complex and multifaceted. Inflammatory cytokines, often elevated in obesity and T2D, are known to compromise BBB integrity by altering tight junction protein expression and endothelial cell function. Additionally, the hyperglycemic environment in T2D can induce oxidative stress and microvascular complications, further impairing BBB function. These alterations not only have direct implications for neurovascular health but also predispose individuals to a range of neurological disorders. Takechi et al. [ 125 ] shows that BBB dysfunction precedes cognitive decline and neurodegeneration in a diabetic insulin-resistant mouse modeled by 24 w of high fat and fructose fed, which may imply a causal link. Although this study is more centered around diabetes, the intersection with obesity (through insulin resistance) makes it pertinent. The above findings emphasizing the need for comprehensive management of these metabolic conditions to maintain BBB integrity and overall brain health.
In summary, the accumulating evidence underscores a significant association between obesity, T2D, and BBB disruption. This relationship highlights the importance of managing these metabolic disorders not only for cardiovascular health but also for maintaining the integrity of the neurovascular unit. As research in this field continues to evolve, understanding the specific pathways and impacts will be crucial for developing targeted interventions aimed at preserving BBB integrity in the face of growing obesity and T2D prevalence.
Obesity-induced neuroinflammation: phenotypes and mechanisms
Neuroinflammation, characterized by the activation of resident brain cells (microglia and astrocytes) and infiltration of peripheral immune cells, has been widely recognized as a critical pathological feature of obesity. The increased BBB permeability induced by obesity, as discussed earlier, not only allows harmful substances to penetrate into the CNS but also paves the way for peripheral immune cells to infiltrate the brain. Once in the CNS, these immune cells can instigate an inflammatory response, contributing significantly to obesity-induced neuroinflammation.
Microglia, the primary immune cells of the CNS, show enhanced activation in obesity. Once activated, microglia release a plethora of pro-inflammatory factors, including cytokines like TNF-α, IL-1β, and IL-6, thereby promoting a pro-inflammatory environment within the CNS [ 84 , 126 , 127 , 128 ]. In the research employed bone marrow chimerism mouse subjected to 15 or 30 w of HFD, it was also found that the aggregated inflammatory monocytes/macrophages located in the parenchyma and expressed the microglial marker Iba1 [ 129 ]. Notably, while microglia often exhibit a pro-inflammatory phenotype in the early stages of HFD-induced obesity, their activation state appears to change over time with prolonged exposure. As the study by Baufeld et al. [ 130 ] demonstrated, the initial microglial reaction in the hypothalamus of mice with 3 days of HFD was not accompanied by sustained increased pro-inflammatory cytokines with prolonged 20 w of HFD consumption. Rather, anti-inflammatory genes were upregulated while microglial sensing genes were downregulated [ 130 ]. This indicates that microglia may shift to a more anti-inflammatory or homeostatic phenotype after longer-term HFD consumption.
The regional heterogeneity of microglial responses is another important consideration. Microglia in the hypothalamic arcuate nucleus, for instance, displayed a markedly reaction to 8 weeks of HFD in a region-specific manner [ 130 ]. This underscores how microglia in different brain regions may uniquely adapted to their specific microenvironments and react differently to the metabolic challenges imposed by obesity. Furthermore, the plasticity and ability of microglia to respond to additional stimuli was preserved even after prolonged HFD feeding. When stimulated with LPS ex vivo after 8 weeks of HFD, hypothalamic microglia upregulated inflammatory genes comparable to microglia from control diet mice [ 130 ]. This indicates the microglia retain responsiveness despite adapting to the HFD conditions. Mechanically, Kim et al. [ 131 ] has been instrumental in highlighting the dynamic increase in uncoupling protein 2 ( Ucp2 ) mRNA expression in the hypothalamic microglia of mice following an 8 w-HFD regimen. This increase influences mitochondrial modifications that activate microglia, further contributing to hypothalamic inflammation and the overall susceptibility to obesity. In summary, emerging evidence indicates microglial phenotypes and functions are altered in a temporal and spatial manner by obesity and HFD consumption. While often displaying pro-inflammatory features acutely, microglia may adapt with anti-inflammatory or homeostatic responses over time. Their heterogeneous phenotypes across brain regions and retained ability to respond to stimuli highlight the complexity of microglial reactions in obesity.
Astrocytes, another critical cell type in the CNS, undergo reactive astrogliosis in obesity, characterized by changes in their morphology, proliferation, and function. Similar to microglia, activated astrocytes can also secrete pro-inflammatory cytokines, further exacerbating the neuroinflammatory response. Astrocytes in 16 w-HFD consumption displayed reactive astrogliosis, characterized by altered morphology and upregulation of intermediate filaments like glial fibrillary acidic protein (GFAP) [ 132 ]. This phenotypic shift was observable early during HFD feeding, even preceding substantial weight gain. Lin et al. [ 133 ] elucidates the upregulation of disease-associated astrocyte (DAA) and microglia markers in response to an 12 w-HFD that are similar to the pathogenesis of Alzheimer’s disease. providing a direct link between dietary habits, neuroinflammation, and neurodegeneration.
The functional profile of astrocytes was also altered by obesity-induced astrogliosis. Activated astrocytes upregulate expression of pro-inflammatory cytokines, including IL-6, IL-1β, and TNF-α in mice following an 11 w-HFD regimen [ 134 ]. This creates a self-perpetuating cycle, as increased cytokine levels can further stimulate astrogliosis. Additionally, aberrant release of gliotransmitters like glutamate from reactive astrocytes can also occur, potentially impacting neuronal excitability after a 16 w of HFD [ 126 ].
Multiple signaling pathways have been implicated in driving the astrogliotic transformation of astrocytes in obesity. As Thaler et al. demonstrated, astrocyte-specific inhibition of IKKβ/NF-κB signaling mitigated weight gain, glucose intolerance, and hypothalamic inflammation induced by 11 w of HFD consumption [ 134 ]. This suggests the IKKβ/NF-κB pathway is critical for obesity-related astrogliosis and its metabolic consequences. Calcineurin signaling has also been linked to astrocyte reactivity in response to HFD for 16 w [ 132 ]. Calcineurin inhibition attenuated gliosis in the arcuate nucleus, ventromedial hypothalamus, and dorsomedial hypothalamus of HFD mice. This implicates calcineurin/NFAT as another important mediator of astrocyte activation. In summary, the morphological and functional changes accompanying astrogliosis position astrocytes as key propagators of neuroinflammatory responses in obesity models induced by high-fat feeding. Delineating the intracellular signaling pathways driving these astrocyte alterations, such as IKKβ/NF-κB and calcineurin/NFAT, will contribute to uncover therapeutic targets for mitigating obesity-associated hypothalamic inflammation.
Monocytes/macrophages
Peripheral immune cells, particularly monocytes, have been reported to infiltrate the CNS in obesity. These monocytes can differentiate into macrophages, producing a variety of pro-inflammatory cytokines that exacerbate neuroinflammation. For instance, a bone marrow chimerism mouse model demonstrated that 15 or 30 w of HFD-induced obesity led to a 30% increase of immune cells in the CNS compared to controls [ 129 ]. Most of these cells exhibited a microglia/macrophage phenotype, being CD45 + CD11b + . The ratio of CD11b + CD45 hi to CD11b + CD45 lo cells was elevated, indicating an inflammatory state. In addition to the infiltration, HFD also promotes the differentiation of monocytes into macrophages. It was demonstrated that 14 w of HFD consumption induced the Ly6c high monocytes to differentiate into macrophages in the brain [ 135 ]. Another study showed that prolonged HFD consumption for 4 and 20 w led to expansion of the monocyte-derived macrophage pool in the hypothalamic arcuate nucleus, attributed to enhanced macrophage proliferation [ 136 ]. In mouse models of leptin receptor deficiency, breakdown of the BBB was found to enable macrophage infiltration into the brain of db/db mice [ 137 ]. Leptin resistance, glucose intolerance, and elevated cytokines like IL-1β and TNF-α accompanied the accumulation of macrophages. Notably, this study also suggested that IL-1β potentially play an important role in trafficking of peripheral monocytes into the brain. Overall, these studies indicate HFD-induced obesity facilitates the recruitment and proliferation of macrophages in the brain, propagating inflammation.
While the involvement of monocytes and macrophages in mediating neuroinflammatory responses under obese conditions is evident, it is imperative to scrutinize the methodologies employed in these studies for potential confounding factors. Specifically, investigations utilizing GFP bone marrow chimeras, such as those by Buckman et al. [ 129 ] and Baufeld et al. [ 130 ] mention above, provide critical insights into the trafficking of these immune cells in the context of obesity. However, the integral role of radiation treatment in these experimental designs warrants a careful evaluation of its impact on the observed outcomes. Radiation used to establish bone marrow chimeras, as a preparatory step for tracking immune cell migration, is known to independently alter BBB permeability and trigger pro-inflammatory responses, as detailed by Kierdorf et al. [ 138 ] This raises important considerations for interpreting studies on obesity-associated neuroinflammation: the increased permeability of the BBB and the augmented infiltration of monocytes/macrophages observed could be confounded by the radiation treatment itself, rather than being solely attributable to the effects of obesity.
Acknowledging these potential confounding effects is crucial for a comprehensive understanding of the dynamics between obesity and neurovascular integrity. Future research aimed at elucidating the specific roles of monocytes and macrophages in obesity-induced BBB disruption and brain inflammation should consider employing alternative methodologies that circumvent the need for radiation-induced bone marrow ablation. This approach will ensure a clearer delineation of the direct consequences of obesity on neuroimmune interactions and BBB integrity, free from the complicating effects of experimental interventions.
By carefully considering these methodological nuances, we can enhance our understanding of the complex interplay between obesity, BBB integrity, and the role of monocytes/macrophages in neuroinflammation, thereby paving the way for more targeted and effective therapeutic strategies.
Pro-inflammatory factors
Obesity-induced neuroinflammation is also marked by elevated expression levels of pro-inflammatory factors. In addition to TNF-α, IL-1β, and IL-6, other pro-inflammatory molecules such as inducible nitric oxide synthase (iNOS) and cyclooxygenase-2 (COX-2) show increased expression in obesity. These molecules, produced by both infiltrating peripheral immune cells and activated resident brain cells, not only propagate inflammation but can also contribute to BBB disruption and neuronal damage. In human frontal cortex, it was reported that increased BMI has been found to cause iNOS-mediated inflammatory activity [ 139 ]. A recent finding demonstrated that iNOS promotes hypothalamic insulin resistance in obese rats. Aberrant nitrosative stress such as S-nitrosatiion (also referred to as S-nitrosylation) is closely associate with various neurological disorders such as AD and Parkinson’s Disease (PD) [ 140 , 141 ]. Inhibition of central iNOS ameliorated not only glucose metabolism, but also macrophage activation induced inflammation in hypothalamus of HFD-induced obesity mice [ 136 ]. In addition, 18 or 20 w of HFD consumption also can significantly enhance the expression of COX-2 in the hippocampus of the mice [ 142 , 143 ]. An increased activity of COX-2-PEG2 signaling pathway has been considered to play a key role in impairing hippocampal neuronal function and cognition [ 144 , 145 ].
Additionally, the aberrant activation of the inflammasome complexes, especially NOD-like receptor thermal protein domain associated protein 3 (NLRP3), plays a vital role in obesity-induced neuroinflammatory [ 146 ]. Within the CNS, microglia accumulate lipid droplets and activate NLRP3 inflammasomes under hyperglycemic conditions due to impaired lipophagy [ 147 ]. The microglial specific inflammatory amplifier TREM1 (triggering receptor expressed on myeloid cells), resulting in the buildup of microglial TREM1, was found to aggravates the HG-induced lipophagy damage and subsequently promoted HG-induced neuroinflammatory cascades via NLRP3 (NLR family pyrin domain containing 3) inflammasome. Pharmacological blockade of TREM1 with LP17 in db/db mice and HFD/STZ mice inhibited accumulation of lipid droplets and TREM1, reduced hippocampal neuronal inflammatory damage, and consequently improved cognitive functions [ 147 ].
Together, these diverse peripheral and central inflammatory pathways contribute to BBB disruption, neurotoxicity, and cognitive deficits associated with obesity. Targeting shared processes like nitrosative stress, which interacts with iNOS signaling, may simultaneously mitigate obesity-related neuroinflammation. The upregulation of pivotal inflammatory enzymes like iNOS and COX-2 highlights the multi-faceted nature of obesity-induced inflammation and the need for strategies that address key underlying pathways.
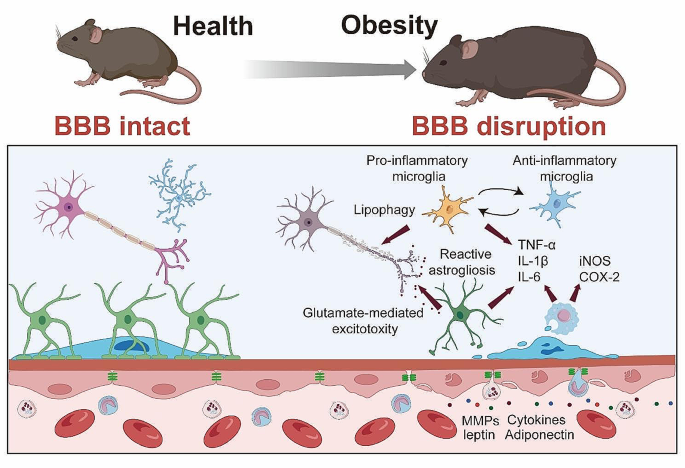
Mechanisms of Obesity-Induced Neuroinflammation . Enhanced microglial activation in obesity leads to increased release of pro-inflammatory cytokines (TNF-α, IL-1β, IL-6), contributing to a pro-inflammatory CNS environment. Concurrently, obesity triggers reactive astrogliosis in astrocytes characterized by morphological and functional changes, and secretion of pro-inflammatory cytokines. The infiltration of peripheral monocytes into the CNS, and differentiation into macrophages further exacerbates neuroinflammation through additional cytokine production. Elevated expression of pro-inflammatory factors such as iNOS and COX-2 in obesity are also depicted, highlighting their potential role in neuroinflammation, BBB disruption, and neuronal damage
Dietary influences on BBB integrity and obesity outcomes
The intricate relationship between obesity and inflammation underscores the multifaceted nature of BBB dysfunction. While the systemic inflammation associated with obesity undoubtedly impacts BBB integrity, it is imperative to address the direct and indirect effects of dietary patterns on neurovascular health. The link between diet, obesity, and BBB integrity provides a unique lens through which to understand the broader implications of nutritional habits on cerebral health and disease susceptibility. Freeman et al. [ 148 ] highlights the adverse effects of a 6 month consumption of high-fat/high-cholesterol (HFHC) diet on BBB function, demonstrating a reduction in BBB integrity markers and an increase in inflammatory responses within the hippocampus in middle-aged rats. This suggests that HFHC diets, beyond contributing to obesity, may directly compromise the BBB, thereby facilitating a neuroinflammatory state that could underpin cognitive decline. Similarly, Davidson et al. [ 149 ] reported on the effects of high-energy diets on hippocampal-dependent cognitive functions and BBB integrity. Their findings emphasized that diets high in saturated fats and sugars, hallmarks of the “Western” diet, not only contribute to obesity but also impair types of learning and memory dependent on the hippocampus. The unique aspect of this research is the differentiation between diet-induced obese and diet-resistant rats, highlighting how diet affects cognitive function and BBB integrity differently based on individual susceptibility to obesity. This is particularly concerning as it implies that dietary components can directly influence cognitive functions by altering hippocampal integrity and possibly disrupting the BBB. The dietary components and direct influences on BBB was detailed in Table 1 , which underscores the potential for dietary modifications as preventive or mitigative strategies against obesity-induced neurovascular alterations.
Emerging therapeutic strategies in tackling obesity-induced BBB dysfunction
In addressing the complexities of obesity-induced metabolic syndrome and its impact on the central nervous system, a spectrum of experimental therapies is currently under investigation, each targeting distinct pathological mechanisms. One promising approach involves the decrease of BBB permeability. Agents such as Palmitoylethanolamide, Topiramate, and Nicotine have demonstrated potential in modulating BBB dynamics, suggesting a pivotal role in mitigating obesity-related neurological sequelae in mice subjected to 10–36 w of HFD or 10 w of high saturated fatty acids diet consumption [ 72 , 73 , 84 ]. Among these, Palmitoylethanolamide has shown promise, not only in attenuating anxiety-like behavior but also in modulating neurotransmitter levels such as dopamine turnover and γ-aminobutyric acid (GABA) levels in the amygdala from mice subjected to 19 w of HFD consumption [ 84 ]. Additionally, it has been found to reduce systemic inflammation markers like TNF-α and IL-1β, attenuate hypothalamic injury, and decrease neuroinflammation and BBB permeability in the hippocampus. However, while its effects on BBB permeability are notable, further investigation is necessary to fully understand its long-term efficacy and safety profile, particularly in relation to chronic administration and potential systemic effects.
Topiramate, another therapeutic agent, has been demonstrated its capability to decrease BBB permeability in mice subjected to 13 and 36 w of HFD [ 72 ]. It achieves this through increasing the expression of tight junction proteins like ZO-1 and Claudin-12, which are crucial in maintaining BBB integrity. Topiramate also exhibits properties that inhibit oxidative stress, a common pathological feature in obesity-related neurological disorders. In this regard, agents like Dapsone and Resveratrol have garnered attention for their potential to protect tight junctions and reduce BBB disruption in mice following 8 w of HFD [ 74 , 77 ]. Dapsone, for instance, has been found to decrease brain microvascular leakage, which is often exacerbated in obesity. It does so by inhibiting the oxidation of low-density lipoproteins (LDL), a process that is detrimental to the BBB [ 77 ]. Additionally, Dapsone has shown efficacy in protecting tight junction proteins such as ZO-1, Claudin-5, and Occludin, further enhancing its role in preserving BBB integrity in mice following 8 w of HFD. Similarly, Resveratrol, a naturally occurring polyphenolic compound, has been demonstrated its capacity to fortify BBB tight junctions. Its neuroprotective properties extend beyond just maintaining the BBB; Resveratrol also exhibits antioxidative and anti-inflammatory effects, which are beneficial in addressing the multifactorial aspects of obesity-induced neural damage in mice subjected 8 w of HFD [ 74 ]. However, while these agents show promise, their clinical application faces challenges. The variability in individual responses and potential side effects, such as hypersensitivity reactions with Dapsone, require careful consideration. Future research must focus on optimizing these therapies, possibly through targeted delivery systems or combination therapies, to enhance their protective effects on the BBB while minimizing adverse reactions. Despite these benefits, the challenge with Topiramate lies in its potential side effects, such as cognitive disturbances and weight loss, which might limit its use in certain patient populations. Future research should focus on optimizing its dosage and delivery mechanisms to maximize its therapeutic benefits while minimizing adverse effects. Besides the aforementioned strategies, other pharmacological effects pivotal in managing obesity-induced complications include the modulation of oxidative stress and cellular death, the regulation of metabolic pathways, and the enhancement of neural regeneration, each contributing uniquely towards mitigating the multifaceted challenges posed by obesity.
In conclusion, the emerging therapeutic strategies discussed in this section underscore the complexity and multidimensionality of tackling obesity-induced metabolic syndrome and its neurological implications. From enhancing BBB integrity to addressing oxidative stress, metabolic dysregulation, and promoting neuroregeneration, each approach offers a unique angle in combating the extensive impact of obesity [ 150 , 151 ]. This multifaceted approach not only broadens our understanding but also paves the way for innovative and comprehensive treatments in the ongoing battle against obesity-related neurological disorders. The major experimental therapies are summarized in Table 2 , which presents a curated list of compounds demonstrated to exert protective effects against the adverse consequences of obesity on the brain, particularly focusing on maintaining or restoring BBB integrity. Each compound included has been selected based on empirical evidence from studies highlighting its efficacy in counteracting obesity-induced neurovascular dysfunction.
Conclusion and future directions
The intricate association between obesity and the BBB has been the primary focus of this review. We have delved into the fundamental understanding of BBB dysfunction in the context of obesity, detailing the altered BBB permeability, transport dysfunction, and neuroinflammation, each contributing to a multifaceted pathophysiological landscape that paves the way for obesity-related neurological disorders. Despite the progress made, several gaps persist in our understanding of obesity-induced BBB dysfunction. For instance, more in-depth studies on the temporal relationship between obesity and BBB dysfunction could provide insight into the initial triggers of these changes. Furthermore, it remains unclear whether BBB dysfunction is a universal feature of obesity or if it varies with factors such as the degree and duration of obesity, age, sex, and genetic predisposition. Moreover, the potential reversibility of obesity-induced BBB changes and the optimal strategies for achieving such reversibility warrant exploration.
Recognizing BBB dysfunction’s role in obesity-related neurological diseases holds significant promise for future therapeutic advancements. By better understanding the interactions between BBB dysfunction and neurodegeneration, we may discover new strategies to mitigate or even prevent the deleterious effects of obesity on the CNS. There lies immense potential in targeting the BBB for therapeutic intervention, with the promise of not only alleviating obesity-induced BBB dysfunction but also mitigating its downstream effects, including neuroinflammation and neurodegeneration.
In conclusion, obesity-induced BBB dysfunction represents an area of research with implications extending beyond the realm of obesity to a broad spectrum of neurological disorders. A more comprehensive understanding of obesity’s influence on the CNS would ultimately benefit those affected by obesity and its neurological consequences.
Data availability
No datasets were generated or analysed during the current study.
WHO. Obesity and overweight. 2022.
Pedditzi E, Peters R, Beckett N. The risk of overweight/obesity in mid-life and late life for the development of dementia: a systematic review and meta-analysis of longitudinal studies. Age Ageing. 2016;45:14–21.
Article PubMed Google Scholar
Flores-Cordero JA, Perez-Perez A, Jimenez-Cortegana C, Alba G, Flores-Barragan A, Sanchez-Margalet V. Obesity as a Risk Factor for Dementia and Alzheimer’s Disease: The Role of Leptin. Int J Mol Sci 2022, 23.
Wieckowska-Gacek A, Mietelska-Porowska A, Wydrych M, Wojda U. Western diet as a trigger of Alzheimer’s disease: from metabolic syndrome and systemic inflammation to neuroinflammation and neurodegeneration. Ageing Res Rev. 2021;70:101397.
Article CAS PubMed Google Scholar
Haley MJ, Lawrence CB. Obesity and stroke: can we translate from rodents to patients? J Cereb Blood Flow Metab. 2016;36:2007–21.
Article CAS PubMed PubMed Central Google Scholar
Lee SH, Jung JM, Park MH. Obesity paradox and stroke outcomes according to stroke subtype: a propensity score-matched analysis. Int J Obes (Lond). 2023;47:669–76.
Akyea RK, Doehner W, Iyen B, Weng SF, Qureshi N, Ntaios G. Obesity and long-term outcomes after incident stroke: a prospective population-based cohort study. J Cachexia Sarcopenia Muscle. 2021;12:2111–21.
Article PubMed PubMed Central Google Scholar
Mi Y, Qi G, Vitali F, Shang Y, Raikes AC, Wang T, Jin Y, Brinton RD, Gu H, Yin F. Loss of fatty acid degradation by astrocytic mitochondria triggers neuroinflammation and neurodegeneration. Nat Metab. 2023;5:445–65.
Morant-Ferrando B, Jimenez-Blasco D, Alonso-Batan P, Agulla J, Lapresa R, Garcia-Rodriguez D, Yunta-Sanchez S, Lopez-Fabuel I, Fernandez E, Carmeliet P, et al. Fatty acid oxidation organizes mitochondrial supercomplexes to sustain astrocytic ROS and cognition. Nat Metab. 2023;5:1290–302.
Profaci CP, Munji RN, Pulido RS, Daneman R. The blood-brain barrier in health and disease: important unanswered questions. J Exp Med 2020, 217.
Knox EG, Aburto MR, Clarke G, Cryan JF, O’Driscoll CM. The blood-brain barrier in aging and neurodegeneration. Mol Psychiatry. 2022;27:2659–73.
Schaeffer S, Iadecola C. Revisiting the neurovascular unit. Nat Neurosci. 2021;24:1198–209.
Sweeney MD, Zhao Z, Montagne A, Nelson AR, Zlokovic BV. Blood-brain barrier: from physiology to Disease and back. Physiol Rev. 2019;99:21–78.
Langen UH, Ayloo S, Gu C. Development and Cell Biology of the blood-brain barrier. Annu Rev Cell Dev Biol. 2019;35:591–613.
Huang X, Hussain B, Chang J. Peripheral inflammation and blood-brain barrier disruption: effects and mechanisms. CNS Neurosci Ther. 2021;27:36–47.
Paik DT, Tian L, Williams IM, Rhee S, Zhang H, Liu C, Mishra R, Wu SM, Red-Horse K, Wu JC. Single-cell RNA sequencing unveils Unique Transcriptomic signatures of Organ-Specific endothelial cells. Circulation. 2020;142:1848–62.
Nguyen YTK, Ha HTT, Nguyen TH, Nguyen LN. The role of SLC transporters for brain health and disease. Cell Mol Life Sci. 2021;79:20.
Chai AB, Callaghan R, Gelissen IC. Regulation of P-Glycoprotein in the brain. Int J Mol Sci 2022, 23.
Cui Y, Wang Y, Song X, Ning H, Zhang Y, Teng Y, Wang J, Yang X. Brain endothelial PTEN/AKT/NEDD4-2/MFSD2A axis regulates blood-brain barrier permeability. Cell Rep. 2021;36:109327.
Andreone BJ, Chow BW, Tata A, Lacoste B, Ben-Zvi A, Bullock K, Deik AA, Ginty DD, Clish CB, Gu CH. Blood-brain barrier permeability is regulated by lipid transport-dependent suppression of Caveolae-Mediated Transcytosis. Neuron. 2017;94:581–.
Ayloo S, Gu C. Transcytosis at the blood-brain barrier. Curr Opin Neurobiol. 2019;57:32–8.
Ben-Zvi A, Lacoste B, Kur E, Andreone BJ, Mayshar Y, Yan H, Gu C. Mfsd2a is critical for the formation and function of the blood-brain barrier. Nature. 2014;509:507–11.
Yang YR, Xiong XY, Liu J, Wu LR, Zhong Q, Zhou K, Meng ZY, Liu L, Wang FX, Gong QW et al. Mfsd2a (Major Facilitator Superfamily Domain Containing 2a) attenuates Intracerebral Hemorrhage-Induced blood-brain barrier disruption by inhibiting vesicular transcytosis. J Am Heart Assoc 2017, 6.
Chapman PT, Haskard DO. Leukocyte adhesion molecules. Br Med Bull. 1995;51:296–311.
Rossler K, Neuchrist C, Kitz K, Scheiner O, Kraft D, Lassmann H. Expression of leucocyte adhesion molecules at the human blood-brain barrier (BBB). J Neurosci Res. 1992;31:365–74.
Allavena R, Noy S, Andrews M, Pullen N. CNS elevation of vascular and not mucosal addressin cell adhesion molecules in patients with multiple sclerosis. Am J Pathol. 2010;176:556–62.
Armulik A, Genove G, Mae M, Nisancioglu MH, Wallgard E, Niaudet C, He L, Norlin J, Lindblom P, Strittmatter K, et al. Pericytes regulate the blood-brain barrier. Nature. 2010;468:557–61.
Longden TA, Zhao G, Hariharan A, Lederer WJ. Pericytes and the Control of Blood Flow in Brain and Heart. Annu Rev Physiol. 2023;85:137–64.
Hall CN, Reynell C, Gesslein B, Hamilton NB, Mishra A, Sutherland BA, O’Farrell FM, Buchan AM, Lauritzen M, Attwell D. Capillary pericytes regulate cerebral blood flow in health and disease. Nature. 2014;508:55–60.
Bell RD, Winkler EA, Sagare AP, Singh I, LaRue B, Deane R, Zlokovic BV. Pericytes control key neurovascular functions and neuronal phenotype in the adult brain and during brain aging. Neuron. 2010;68:409–27.
Sweeney MD, Ayyadurai S, Zlokovic BV. Pericytes of the neurovascular unit: key functions and signaling pathways. Nat Neurosci. 2016;19:771–83.
Nyul-Toth A, Kozma M, Nagyoszi P, Nagy K, Fazakas C, Hasko J, Molnar K, Farkas AE, Vegh AG, Varo G, et al. Expression of pattern recognition receptors and activation of the non-canonical inflammasome pathway in brain pericytes. Brain Behav Immun. 2017;64:220–31.
Kaushik DK, Bhattacharya A, Lozinski BM, Wee Yong V. Pericytes as mediators of infiltration of macrophages in multiple sclerosis. J Neuroinflammation. 2021;18:301.
Medina-Flores F, Hurtado-Alvarado G, Deli MA, Gomez-Gonzalez B. The active role of Pericytes during Neuroinflammation in the adult brain. Cell Mol Neurobiol. 2023;43:525–41.
Arimura K, Ago T, Kamouchi M, Nakamura K, Ishitsuka K, Kuroda J, Sugimori H, Ooboshi H, Sasaki T, Kitazono T. PDGF receptor beta signaling in pericytes following ischemic brain injury. Curr Neurovasc Res. 2012;9:1–9.
Shimizu F, Sano Y, Saito K, Abe MA, Maeda T, Haruki H, Kanda T. Pericyte-derived glial cell line-derived neurotrophic factor increase the expression of claudin-5 in the blood-brain barrier and the blood-nerve barrier. Neurochem Res. 2012;37:401–9.
Dave JM, Mirabella T, Weatherbee SD, Greif DM. Pericyte ALK5/TIMP3 Axis contributes to endothelial morphogenesis in the developing brain. Dev Cell. 2018;44:665–e678666.
Duan L, Zhang XD, Miao WY, Sun YJ, Xiong G, Wu Q, Li G, Yang P, Yu H, Li H, et al. PDGFRbeta cells rapidly relay Inflammatory Signal from the Circulatory System to neurons via chemokine CCL2. Neuron. 2018;100:183–e200188.
Schrimpf C, Koppen T, Duffield JS, Boer U, David S, Ziegler W, Haverich A, Teebken OE, Wilhelmi M. TIMP3 is regulated by Pericytes upon Shear stress detection leading to a modified endothelial cell response. Eur J Vasc Endovasc Surg. 2017;54:524–33.
Cai W, Liu H, Zhao J, Chen LY, Chen J, Lu Z, Hu X. Pericytes in Brain Injury and Repair after ischemic stroke. Transl Stroke Res. 2017;8:107–21.
Lendahl U, Nilsson P, Betsholtz C. Emerging links between cerebrovascular and neurodegenerative diseases-a special role for pericytes. EMBO Rep. 2019;20:e48070.
Sakuma R, Kawahara M, Nakano-Doi A, Takahashi A, Tanaka Y, Narita A, Kuwahara-Otani S, Hayakawa T, Yagi H, Matsuyama T, Nakagomi T. Brain pericytes serve as microglia-generating multipotent vascular stem cells following ischemic stroke. J Neuroinflammation. 2016;13:57.
Nakata M, Nakagomi T, Maeda M, Nakano-Doi A, Momota Y, Matsuyama T. Induction of Perivascular neural stem cells and possible contribution to Neurogenesis following transient brain Ischemia/Reperfusion Injury. Transl Stroke Res. 2017;8:131–43.
Caporarello N, D’Angeli F, Cambria MT, Candido S, Giallongo C, Salmeri M, Lombardo C, Longo A, Giurdanella G, Anfuso CD, Lupo G. Pericytes in Microvessels: from mural function to brain and retina regeneration. Int J Mol Sci 2019, 20.
Smyth LCD, Rustenhoven J, Park TI, Schweder P, Jansson D, Heppner PA, O’Carroll SJ, Mee EW, Faull RLM, Curtis M, Dragunow M. Unique and shared inflammatory profiles of human brain endothelia and pericytes. J Neuroinflammation. 2018;15:138.
Alvarez JI, Dodelet-Devillers A, Kebir H, Ifergan I, Fabre PJ, Terouz S, Sabbagh M, Wosik K, Bourbonniere L, Bernard M, et al. The hedgehog pathway promotes blood-brain barrier integrity and CNS immune quiescence. Science. 2011;334:1727–31.
Hill SA, Fu M, Garcia ADR. Sonic hedgehog signaling in astrocytes. Cell Mol Life Sci. 2021;78:1393–403.
Xie Y, Kuan AT, Wang W, Herbert ZT, Mosto O, Olukoya O, Adam M, Vu S, Kim M, Tran D, et al. Astrocyte-neuron crosstalk through hedgehog signaling mediates cortical synapse development. Cell Rep. 2022;38:110416.
Song S, Huang H, Guan X, Fiesler V, Bhuiyan MIH, Liu R, Jalali S, Hasan MN, Tai AK, Chattopadhyay A, et al. Activation of endothelial Wnt/beta-catenin signaling by protective astrocytes repairs BBB damage in ischemic stroke. Prog Neurobiol. 2021;199:101963.
Zhou Z, Zhan J, Cai Q, Xu F, Chai R, Lam K, Luan Z, Zhou G, Tsang S, Kipp M et al. The water transport system in astrocytes-aquaporins. Cells 2022, 11.
Verkman AS, Smith AJ, Phuan PW, Tradtrantip L, Anderson MO. The aquaporin-4 water channel as a potential drug target in neurological disorders. Expert Opin Ther Targets. 2017;21:1161–70.
Chai RC, Jiang JH, Wong AY, Jiang F, Gao K, Vatcher G. Hoi Yu AC: AQP5 is differentially regulated in astrocytes during metabolic and traumatic injuries. Glia. 2013;61:1748–65.
Xu L, Nirwane A, Yao Y. Basement membrane and blood-brain barrier. Stroke Vasc Neurol. 2019;4:78–82.
Baeten KM, Akassoglou K. Extracellular matrix and matrix receptors in blood-brain barrier formation and stroke. Dev Neurobiol. 2011;71:1018–39.
Nirwane A, Yao Y. Laminins and their receptors in the CNS. Biol Rev Camb Philos Soc. 2019;94:283–306.
Wu C, Ivars F, Anderson P, Hallmann R, Vestweber D, Nilsson P, Robenek H, Tryggvason K, Song J, Korpos E, et al. Endothelial basement membrane laminin alpha5 selectively inhibits T lymphocyte extravasation into the brain. Nat Med. 2009;15:519–27.
Zhang X, Wang Y, Song J, Gerwien H, Chuquisana O, Chashchina A, Denz C, Sorokin L. The endothelial basement membrane acts as a checkpoint for entry of pathogenic T cells into the brain. J Exp Med 2020, 217.
Sixt M, Engelhardt B, Pausch F, Hallmann R, Wendler O, Sorokin LM. Endothelial cell laminin isoforms, laminins 8 and 10, play decisive roles in T cell recruitment across the blood-brain barrier in experimental autoimmune encephalomyelitis. J Cell Biol. 2001;153:933–46.
Wu X, Reddy DS. Integrins as receptor targets for neurological disorders. Pharmacol Ther. 2012;134:68–81.
Lilja J, Ivaska J. Integrin activity in neuronal connectivity. J Cell Sci 2018, 131.
Park YK, Goda Y. Integrins in synapse regulation. Nat Rev Neurosci. 2016;17:745–56.
Krishnaswamy VR, Benbenishty A, Blinder P, Sagi I. Demystifying the extracellular matrix and its proteolytic remodeling in the brain: structural and functional insights. Cell Mol Life Sci. 2019;76:3229–48.
Jin C, Shi Y, Shi L, Leak RK, Zhang W, Chen K, Ye Q, Hassan S, Lyu J, Hu X, et al. Leveraging single-cell RNA sequencing to unravel the impact of aging on stroke recovery mechanisms in mice. Proc Natl Acad Sci U S A. 2023;120:e2300012120.
Li X, Li Y, Jin Y, Zhang Y, Wu J, Xu Z, Huang Y, Cai L, Gao S, Liu T, et al. Transcriptional and epigenetic decoding of the microglial aging process. Nat Aging. 2023;3:1288–311.
Marino Lee S, Hudobenko J, McCullough LD, Chauhan A. Microglia depletion increase brain injury after acute ischemic stroke in aged mice. Exp Neurol. 2021;336:113530.
Liddelow SA, Guttenplan KA, Clarke LE, Bennett FC, Bohlen CJ, Schirmer L, Bennett ML, Munch AE, Chung WS, Peterson TC, et al. Neurotoxic reactive astrocytes are induced by activated microglia. Nature. 2017;541:481–7.
Shi X, Luo L, Wang J, Shen H, Li Y, Mamtilahun M, Liu C, Shi R, Lee JH, Tian H, et al. Stroke subtype-dependent synapse elimination by reactive gliosis in mice. Nat Commun. 2021;12:6943.
Gour A, Boergens KM, Heike N, Hua Y, Laserstein P, Song K, Helmstaedter M. Postnatal connectomic development of inhibition in mouse barrel cortex. Science 2021, 371.
Kaplan L, Chow BW, Gu C. Neuronal regulation of the blood-brain barrier and neurovascular coupling. Nat Rev Neurosci. 2020;21:416–32.
Williams SD, Setzer B, Fultz NE, Valdiviezo Z, Tacugue N, Diamandis Z, Lewis LD. Correction: neural activity induced by sensory stimulation can drive large-scale cerebrospinal fluid flow during wakefulness in humans. PLoS Biol. 2023;21:e3002123.
Pulido RS, Munji RN, Chan TC, Quirk CR, Weiner GA, Weger BD, Rossi MJ, Elmsaouri S, Malfavon M, Deng A, et al. Neuronal activity regulates blood-brain barrier Efflux Transport through endothelial circadian genes. Neuron. 2020;108:937–e952937.
Salameh TS, Mortell WG, Logsdon AF, Butterfield DA, Banks WA. Disruption of the hippocampal and hypothalamic blood-brain barrier in a diet-induced obese model of type II diabetes: prevention and treatment by the mitochondrial carbonic anhydrase inhibitor, topiramate. Fluids Barriers CNS. 2019;16:1.
Elahy M, Lam V, Pallebage-Gamarallage MM, Giles C, Mamo JC, Takechi R. Nicotine attenuates disruption of blood-brain Barrier Induced by Saturated-Fat Feeding in Wild-Type mice. Nicotine Tob Res. 2015;17:1436–41.
Chang HC, Tai YT, Cherng YG, Lin JW, Liu SH, Chen TL, Chen RM. Resveratrol attenuates high-fat diet-induced disruption of the blood-brain barrier and protects brain neurons from apoptotic insults. J Agric Food Chem. 2014;62:3466–75.
Takechi R, Pallebage-Gamarallage MM, Lam V, Giles C, Mamo JC. Nutraceutical agents with anti-inflammatory properties prevent dietary saturated-fat induced disturbances in blood-brain barrier function in wild-type mice. J Neuroinflammation. 2013;10:73.
Ouyang S, Hsuchou H, Kastin AJ, Wang Y, Yu C, Pan W. Diet-induced obesity suppresses expression of many proteins at the blood-brain barrier. J Cereb Blood Flow Metab. 2014;34:43–51.
Zhan R, Zhao M, Zhou T, Chen Y, Yu W, Zhao L, Zhang T, Wang H, Yang H, Jin Y, et al. Dapsone protects brain microvascular integrity from high-fat diet induced LDL oxidation. Cell Death Dis. 2018;9:683.
Kanoski SE, Zhang Y, Zheng W, Davidson TL. The effects of a high-energy diet on hippocampal function and blood-brain barrier integrity in the rat. J Alzheimers Dis. 2010;21:207–19.
Yamamoto M, Guo DH, Hernandez CM, Stranahan AM. Endothelial Adora2a activation promotes blood-brain barrier breakdown and cognitive impairment in mice with Diet-Induced insulin resistance. J Neurosci. 2019;39:4179–92.
Zhang Y, Shen L, Xie J, Li L, Xi W, Li B, Bai Y, Yao H, Zhang S, Han B. Pushen capsule treatment promotes functional recovery after ischemic stroke. Phytomedicine. 2023;111:154664.
Zhou Y, Zeng X, Li G, Yang Q, Xu J, Zhang M, Mao X, Cao Y, Wang L, Xu Y, et al. Inactivation of endothelial adenosine A(2A) receptors protects mice from cerebral ischaemia-induced brain injury. Br J Pharmacol. 2019;176:2250–63.
Kim DW, Glendining KA, Grattan DR, Jasoni CL. Maternal obesity in the mouse compromises the blood-brain barrier in the Arcuate Nucleus of offspring. Endocrinology. 2016;157:2229–42.
Wu H, Zhang W, Huang M, Lin X, Chiou J. Prolonged high-Fat Diet Consumption throughout Adulthood in mice Induced Neurobehavioral Deterioration via Gut-Brain Axis. Nutrients 2023, 15.
Lama A, Pirozzi C, Severi I, Morgese MG, Senzacqua M, Annunziata C, Comella F, Del Piano F, Schiavone S, Petrosino S, et al. Palmitoylethanolamide dampens neuroinflammation and anxiety-like behavior in obese mice. Brain Behav Immun. 2022;102:110–23.
Stan RV, Kubitza M, Palade GE. PV-1 is a component of the fenestral and stomatal diaphragms in fenestrated endothelia. Proc Natl Acad Sci U S A. 1999;96:13203–7.
Bondareva O, Rodriguez-Aguilera JR, Oliveira F, Liao L, Rose A, Gupta A, Singh K, Geier F, Schuster J, Boeckel JN, et al. Single-cell profiling of vascular endothelial cells reveals progressive organ-specific vulnerabilities during obesity. Nat Metab. 2022;4:1591–610.
Barr TL, Latour LL, Lee KY, Schaewe TJ, Luby M, Chang GS, El-Zammar Z, Alam S, Hallenbeck JM, Kidwell CS, Warach S. Blood-brain barrier disruption in humans is independently associated with increased matrix metalloproteinase-9. Stroke. 2010;41:e123–128.
Palus M, Zampachova E, Elsterova J, Ruzek D. Serum matrix metalloproteinase-9 and tissue inhibitor of metalloproteinase-1 levels in patients with tick-borne encephalitis. J Infect. 2014;68:165–9.
Dal-Pizzol F, Rojas HA, dos Santos EM, Vuolo F, Constantino L, Feier G, Pasquali M, Comim CM, Petronilho F, Gelain DP, et al. Matrix metalloproteinase-2 and metalloproteinase-9 activities are associated with blood-brain barrier dysfunction in an animal model of severe sepsis. Mol Neurobiol. 2013;48:62–70.
Dandona P, Ghanim H, Monte SV, Caruana JA, Green K, Abuaysheh S, Lohano T, Schentag J, Dhindsa S, Chaudhuri A. Increase in the mediators of asthma in obesity and obesity with type 2 diabetes: reduction with weight loss. Obes (Silver Spring). 2014;22:356–62.
Article CAS Google Scholar
Unal R, Yao-Borengasser A, Varma V, Rasouli N, Labbate C, Kern PA, Ranganathan G. Matrix metalloproteinase-9 is increased in obese subjects and decreases in response to pioglitazone. J Clin Endocrinol Metab. 2010;95:2993–3001.
Catalan V, Gomez-Ambrosi J, Rodriguez A, Ramirez B, Silva C, Rotellar F, Gil MJ, Cienfuegos JA, Salvador J, Fruhbeck G. Increased adipose tissue expression of lipocalin-2 in obesity is related to inflammation and matrix metalloproteinase-2 and metalloproteinase-9 activities in humans. J Mol Med (Berl). 2009;87:803–13.
Lauhio A, Farkkila E, Pietilainen KH, Astrom P, Winkelmann A, Tervahartiala T, Pirila E, Rissanen A, Kaprio J, Sorsa TA, Salo T. Association of MMP-8 with obesity, smoking and insulin resistance. Eur J Clin Invest. 2016;46:757–65.
Belo VA, Souza-Costa DC, Lana CM, Caputo FL, Marcaccini AM, Gerlach RF, Bastos MG, Tanus-Santos JE. Assessment of matrix metalloproteinase (MMP)-2, MMP-8, MMP-9, and their inhibitors, the tissue inhibitors of metalloproteinase (TIMP)-1 and TIMP-2 in obese children and adolescents. Clin Biochem. 2009;42:984–90.
Jais A, Solas M, Backes H, Chaurasia B, Kleinridders A, Theurich S, Mauer J, Steculorum SM, Hampel B, Goldau J, et al. Myeloid-cell-derived VEGF maintains brain glucose uptake and limits cognitive impairment in obesity. Cell. 2016;165:882–95.
Haley MJ, Krishnan S, Burrows D, de Hoog L, Thakrar J, Schiessl I, Allan SM, Lawrence CB. Acute high-fat feeding leads to disruptions in glucose homeostasis and worsens stroke outcome. J Cereb Blood Flow Metab. 2019;39:1026–37.
Ogata S, Ito S, Masuda T, Ohtsuki S. Changes of blood-brain barrier and brain parenchymal protein expression levels of mice under different insulin-resistance conditions Induced by High-Fat Diet. Pharm Res. 2019;36:141.
Schuler R, Seebeck N, Osterhoff MA, Witte V, Floel A, Busjahn A, Jais A, Bruning JC, Frahnow T, Kabisch S, et al. VEGF and GLUT1 are highly heritable, inversely correlated and affected by dietary fat intake: consequences for cognitive function in humans. Mol Metab. 2018;11:129–36.
Banks WA, Owen JB, Erickson MA. Insulin in the brain: there and back again. Pharmacol Ther. 2012;136:82–93.
Kaiyala KJ, Prigeon RL, Kahn SE, Woods SC, Schwartz MW. Obesity induced by a high-fat diet is associated with reduced brain insulin transport in dogs. Diabetes. 2000;49:1525–33.
Urayama A, Banks WA. Starvation and triglycerides reverse the obesity-induced impairment of insulin transport at the blood-brain barrier. Endocrinology. 2008;149:3592–7.
Gray SM, Aylor KW, Barrett EJ. Unravelling the regulation of insulin transport across the brain endothelial cell. Diabetologia. 2017;60:1512–21.
Kern W, Benedict C, Schultes B, Plohr F, Moser A, Born J, Fehm HL, Hallschmid M. Low cerebrospinal fluid insulin levels in obese humans. Diabetologia. 2006;49:2790–2.
Kothari V, Luo Y, Tornabene T, O’Neill AM, Greene MW, Geetha T, Babu JR. High fat diet induces brain insulin resistance and cognitive impairment in mice. Biochim Biophys Acta Mol Basis Dis. 2017;1863:499–508.
Talbot K, Wang HY, Kazi H, Han LY, Bakshi KP, Stucky A, Fuino RL, Kawaguchi KR, Samoyedny AJ, Wilson RS, et al. Demonstrated brain insulin resistance in Alzheimer’s disease patients is associated with IGF-1 resistance, IRS-1 dysregulation, and cognitive decline. J Clin Invest. 2012;122:1316–38.
Caro JF, Kolaczynski JW, Nyce MR, Ohannesian JP, Opentanova I, Goldman WH, Lynn RB, Zhang PL, Sinha MK, Considine RV. Decreased cerebrospinal-fluid/serum leptin ratio in obesity: a possible mechanism for leptin resistance. Lancet. 1996;348:159–61.
Schwartz MW, Peskind E, Raskind M, Boyko EJ, Porte D Jr. Cerebrospinal fluid leptin levels: relationship to plasma levels and to adiposity in humans. Nat Med. 1996;2:589–93.
Burguera B, Couce ME, Curran GL, Jensen MD, Lloyd RV, Cleary MP, Poduslo JF. Obesity is associated with a decreased leptin transport across the blood-brain barrier in rats. Diabetes. 2000;49:1219–23.
Adam CL, Findlay PA. Decreased blood-brain leptin transfer in an ovine model of obesity and weight loss: resolving the cause of leptin resistance. Int J Obes (Lond). 2010;34:980–8.
Banks WA, Farrell CL. Impaired transport of leptin across the blood-brain barrier in obesity is acquired and reversible. Am J Physiol Endocrinol Metab. 2003;285:E10–15.
Van Heek M, Compton DS, France CF, Tedesco RP, Fawzi AB, Graziano MP, Sybertz EJ, Strader CD, Davis HR Jr. Diet-induced obese mice develop peripheral, but not central, resistance to leptin. J Clin Invest. 1997;99:385–90.
Halaas JL, Boozer C, Blair-West J, Fidahusein N, Denton DA, Friedman JM. Physiological response to long-term peripheral and central leptin infusion in lean and obese mice. Proc Natl Acad Sci U S A. 1997;94:8878–83.
Banks WA, Niehoff ML, Martin D, Farrell CL. Leptin transport across the blood-brain barrier of the Koletsky rat is not mediated by a product of the leptin receptor gene. Brain Res. 2002;950:130–6.
Banks WA, Coon AB, Robinson SM, Moinuddin A, Shultz JM, Nakaoke R, Morley JE. Triglycerides induce leptin resistance at the blood-brain barrier. Diabetes. 2004;53:1253–60.
Banks WA, Farr SA, Salameh TS, Niehoff ML, Rhea EM, Morley JE, Hanson AJ, Hansen KM, Craft S. Triglycerides cross the blood-brain barrier and induce central leptin and insulin receptor resistance. Int J Obes (Lond). 2018;42:391–7.
Vendelbo J, Olesen RH, Lauridsen JK, Rungby J, Kleinman JE, Hyde TM, Larsen A. Increasing BMI is associated with reduced expression of P-glycoprotein (ABCB1 gene) in the human brain with a stronger association in African americans than caucasians. Pharmacogenomics J. 2018;18:121–6.
Wang C, Li H, Luo C, Li Y, Zhang Y, Yun D, Mu D, Zhou K, Hua Y. The effect of maternal obesity on the expression and functionality of placental P-glycoprotein: implications in the individualized transplacental digoxin treatment for fetal heart failure. Placenta. 2015;36:1138–47.
Cormerais Y, Vucetic M, Parks SK, Pouyssegur J. Amino acid transporters are a vital focal point in the control of mTORC1 Signaling and Cancer. Int J Mol Sci 2020, 22.
Park G, Fukasawa K, Horie T, Masuo Y, Inaba Y, Tatsuno T, Yamada T, Tokumura K, Iwahashi S, Iezaki T et al. l-Type amino acid transporter 1 in hypothalamic neurons in mice maintains energy and bone homeostasis. JCI Insight 2023, 8.
Jersin RA, Jonassen LR, Dankel SN. The neutral amino acid transporter SLC7A10 in adipose tissue, obesity and insulin resistance. Front Cell Dev Biol. 2022;10:974338.
Jersin RA, Tallapragada DSP, Madsen A, Skartveit L, Fjaere E, McCann A, Lawrence-Archer L, Willems A, Bjune JI, Bjune MS, et al. Role of the neutral amino acid transporter SLC7A10 in adipocyte lipid storage, obesity, and insulin resistance. Diabetes. 2021;70:680–95.
Starr JM, Wardlaw J, Ferguson K, MacLullich A, Deary IJ, Marshall I. Increased blood-brain barrier permeability in type II diabetes demonstrated by gadolinium magnetic resonance imaging. J Neurol Neurosurg Psychiatry. 2003;74:70–6.
Gustafson DR, Karlsson C, Skoog I, Rosengren L, Lissner L, Blennow K. Mid-life adiposity factors relate to blood-brain barrier integrity in late life. J Intern Med. 2007;262:643–50.
Schmid A, Hochberg A, Berghoff M, Schlegel J, Karrasch T, Kaps M, Schaffler A. Quantification and regulation of adipsin in human cerebrospinal fluid (CSF). Clin Endocrinol (Oxf). 2016;84:194–202.
Takechi R, Lam V, Brook E, Giles C, Fimognari N, Mooranian A, Al-Salami H, Coulson SH, Nesbit M, Mamo JCL. Blood-brain barrier dysfunction precedes cognitive decline and Neurodegeneration in Diabetic insulin resistant mouse model: an implication for Causal Link. Front Aging Neurosci. 2017;9:399.
Nerurkar PV, Johns LM, Buesa LM, Kipyakwai G, Volper E, Sato R, Shah P, Feher D, Williams PG, Nerurkar VR. Momordica charantia (bitter melon) attenuates high-fat diet-associated oxidative stress and neuroinflammation. J Neuroinflammation. 2011;8:64.
Yi CX, Tschop MH, Woods SC, Hofmann SM. High-fat-diet exposure induces IgG accumulation in hypothalamic microglia. Dis Model Mech. 2012;5:686–90.
CAS PubMed PubMed Central Google Scholar
Sundaram K, Mu J, Kumar A, Behera J, Lei C, Sriwastva MK, Xu F, Dryden GW, Zhang L, Chen S, et al. Garlic exosome-like nanoparticles reverse high-fat diet induced obesity via the gut/brain axis. Theranostics. 2022;12:1220–46.
Buckman LB, Hasty AH, Flaherty DK, Buckman CT, Thompson MM, Matlock BK, Weller K, Ellacott KL. Obesity induced by a high-fat diet is associated with increased immune cell entry into the central nervous system. Brain Behav Immun. 2014;35:33–42.
Baufeld C, Osterloh A, Prokop S, Miller KR, Heppner FL. High-fat diet-induced brain region-specific phenotypic spectrum of CNS resident microglia. Acta Neuropathol. 2016;132:361–75.
Kim JD, Yoon NA, Jin S, Diano S. Microglial UCP2 mediates inflammation and obesity Induced by High-Fat Feeding. Cell Metab. 2019;30:952–e962955.
Pfuhlmann K, Schriever SC, Legutko B, Baumann P, Harrison L, Kabra DG, Baumgart EV, Tschop MH, Garcia-Caceres C, Pfluger PT. Calcineurin a beta deficiency ameliorates HFD-induced hypothalamic astrocytosis in mice. J Neuroinflammation. 2018;15:35.
Lin L, Basu R, Chatterjee D, Templin AT, Flak JN, Johnson TS. Disease-associated astrocytes and microglia markers are upregulated in mice fed high fat diet. Sci Rep. 2023;13:12919.
Douglass JD, Dorfman MD, Fasnacht R, Shaffer LD, Thaler JP. Astrocyte IKKbeta/NF-kappaB signaling is required for diet-induced obesity and hypothalamic inflammation. Mol Metab. 2017;6:366–73.
Lee AG, Kang S, Im S, Pak YK. Cinnamic acid attenuates peripheral and hypothalamic inflammation in High-Fat Diet-Induced obese mice. Pharmaceutics 2022, 14.
Lee CH, Kim HJ, Lee YS, Kang GM, Lim HS, Lee SH, Song DK, Kwon O, Hwang I, Son M, et al. Hypothalamic macrophage inducible nitric oxide synthase mediates obesity-Associated Hypothalamic inflammation. Cell Rep. 2018;25:934–e946935.
Stranahan AM, Hao S, Dey A, Yu X, Baban B. Blood-brain barrier breakdown promotes macrophage infiltration and cognitive impairment in leptin receptor-deficient mice. J Cereb Blood Flow Metab. 2016;36:2108–21.
Kierdorf K, Katzmarski N, Haas CA, Prinz M. Bone marrow cell recruitment to the brain in the absence of irradiation or parabiosis bias. PLoS ONE. 2013;8:e58544.
Lauridsen JK, Olesen RH, Vendelbo J, Hyde TM, Kleinman JE, Bibby BM, Brock B, Rungby J, Larsen A. High BMI levels associate with reduced mRNA expression of IL10 and increased mRNA expression of iNOS (NOS2) in human frontal cortex. Transl Psychiatry. 2017;7:e1044.
Nakamura T, Tu S, Akhtar MW, Sunico CR, Okamoto S, Lipton SA. Aberrant protein s-nitrosylation in neurodegenerative diseases. Neuron. 2013;78:596–614.
Nakamura T, Lipton SA. Protein S-Nitrosylation as a therapeutic target for neurodegenerative diseases. Trends Pharmacol Sci. 2016;37:73–84.
de Paiva IHR, da Silva RS, Mendonca IP, Duarte-Silva E, Botelho de Souza JR, Peixoto CA. Fructooligosaccharide (FOS) and Galactooligosaccharide (GOS) improve Neuroinflammation and Cognition by Up-regulating IRS/PI3K/AKT signaling pathway in Diet-induced obese mice. J Neuroimmune Pharmacol. 2023;18:427–47.
Terzo S, Calvi P, Nuzzo D, Picone P, Allegra M, Mule F, Amato A. Long-Term Ingestion of Sicilian Black Bee Chestnut Honey and/or D-Limonene counteracts brain damage Induced by High Fat-Diet in obese mice. Int J Mol Sci 2023, 24.
Zhu X, Yao Y, Yang J, Zhengxie J, Li X, Hu S, Zhang A, Dong J, Zhang C, Gan G. COX-2-PGE(2) signaling pathway contributes to hippocampal neuronal injury and cognitive impairment in PTZ-kindled epilepsy mice. Int Immunopharmacol. 2020;87:106801.
Sil S, Ghosh T. Role of cox-2 mediated neuroinflammation on the neurodegeneration and cognitive impairments in colchicine induced rat model of Alzheimer’s Disease. J Neuroimmunol. 2016;291:115–24.
Litwiniuk A, Bik W, Kalisz M, Baranowska-Bik A. Inflammasome NLRP3 Potentially Links Obesity-Associated Low-Grade Systemic Inflammation and Insulin Resistance with Alzheimer’s Disease. Int J Mol Sci 2021, 22.
Li Q, Zhao Y, Guo H, Li Q, Yan C, Li Y, He S, Wang N, Wang Q. Impaired lipophagy induced-microglial lipid droplets accumulation contributes to the buildup of TREM1 in diabetes-associated cognitive impairment. Autophagy. 2023;19:2639–56.
Freeman LR, Granholm AC. Vascular changes in rat hippocampus following a high saturated fat and cholesterol diet. J Cereb Blood Flow Metab. 2012;32:643–53.
Davidson TL, Monnot A, Neal AU, Martin AA, Horton JJ, Zheng W. The effects of a high-energy diet on hippocampal-dependent discrimination performance and blood-brain barrier integrity differ for diet-induced obese and diet-resistant rats. Physiol Behav. 2012;107:26–33.
Wang Q, Yuan J, Yu Z, Lin L, Jiang Y, Cao Z, Zhuang P, Whalen MJ, Song B, Wang XJ, et al. FGF21 attenuates high-Fat Diet-Induced Cognitive Impairment via Metabolic Regulation and anti-inflammation of obese mice. Mol Neurobiol. 2018;55:4702–17.
Li S, Liang T, Zhang Y, Huang K, Yang S, Lv H, Chen Y, Zhang C, Guan X. Vitexin alleviates high-fat diet induced brain oxidative stress and inflammation via anti-oxidant, anti-inflammatory and gut microbiota modulating properties. Free Radic Biol Med. 2021;171:332–44.
Download references
This work was supported by the National Natural Science Foundation of China (32170985, 82273923), National Key Research and Development Program of China (2023YFE0202200 and 2021YFA0910000), Guangdong Province Basic and Applied Basic Research Grant (2021B1515120089), Shenzhen Science and Technology Program (JCYJ20210324115800003, ZDSYS20190902093409851 JCYJ20220531100203008), International collaboration project of Chinese Academy of Sciences (172644KYSB20200045), CAS-Croucher Funding Scheme for Joint Laboratories, and Guangdong Innovation Platform of Translational Research for Cerebrovascular Diseases.
Author information
Authors and affiliations.
Key Laboratory of Biomedical Imaging Science, Shenzhen Institute of Advanced Technology, System of Chinese Academy of Sciences, Chinese Academy of Sciences, Shenzhen, Guangdong, China
Ziying Feng, Cheng Fang, Yinzhong Ma & Junlei Chang
University of Chinese Academy of Sciences, Beijing, China
Ziying Feng & Junlei Chang
Institute of Biomedicine and Biotechnology, Shenzhen Institute of Advanced Technology, Chinese Academy of Sciences, Xueyuan Ave 1068, Nanshan, Shenzhen, 518055, Guangdong, China
Yinzhong Ma & Junlei Chang
You can also search for this author in PubMed Google Scholar
Contributions
Ziying Feng and Yinzhong Ma wrote the main manuscript text and created the table and figures. Cheng Fang participated in the literature review and data summary. Junlei Chang was the guarantor and revised the manuscript. All authors reviewed the final manuscript.
Corresponding authors
Correspondence to Yinzhong Ma or Junlei Chang .
Ethics declarations
Ethics approval and consent to participate.
Not applicable. This manuscript is a review article and does not involve any new studies of human or animal subjects performed by any of the authors.
Competing interests
The authors declare no competing interests.
Additional information
Publisher’s note.
Springer Nature remains neutral with regard to jurisdictional claims in published maps and institutional affiliations.
Rights and permissions
Open Access This article is licensed under a Creative Commons Attribution 4.0 International License, which permits use, sharing, adaptation, distribution and reproduction in any medium or format, as long as you give appropriate credit to the original author(s) and the source, provide a link to the Creative Commons licence, and indicate if changes were made. The images or other third party material in this article are included in the article’s Creative Commons licence, unless indicated otherwise in a credit line to the material. If material is not included in the article’s Creative Commons licence and your intended use is not permitted by statutory regulation or exceeds the permitted use, you will need to obtain permission directly from the copyright holder. To view a copy of this licence, visit http://creativecommons.org/licenses/by/4.0/ . The Creative Commons Public Domain Dedication waiver ( http://creativecommons.org/publicdomain/zero/1.0/ ) applies to the data made available in this article, unless otherwise stated in a credit line to the data.
Reprints and permissions
About this article
Cite this article.
Feng, Z., Fang, C., Ma, Y. et al. Obesity-induced blood-brain barrier dysfunction: phenotypes and mechanisms. J Neuroinflammation 21 , 110 (2024). https://doi.org/10.1186/s12974-024-03104-9
Download citation
Received : 31 January 2024
Accepted : 17 April 2024
Published : 27 April 2024
DOI : https://doi.org/10.1186/s12974-024-03104-9
Share this article
Anyone you share the following link with will be able to read this content:
Sorry, a shareable link is not currently available for this article.
Provided by the Springer Nature SharedIt content-sharing initiative
- Blood-brain barrier
- Neuroinflammation
- Vascular permeability
- Neurological disorders
Journal of Neuroinflammation
ISSN: 1742-2094
- Submission enquiries: [email protected]
Sleeve Migration Following Sleeve Gastrectomy: A Systematic Review of Current Literature
- Published: 04 May 2024
Cite this article
- Mohammad Aloulou ORCID: orcid.org/0000-0003-1825-1900 1 , 2 ,
- Alessandro Martinino 3 ,
- Tala Jouma Alhejazi 4 ,
- Sjaak Pouwels 5 ,
- Ahmed Ahmed 6 ,
- James Byrne 7 &
- Chetan Parmar 8 , 9 , 10
Sleeve gastrectomy (SG) is the most frequently performed bariatric procedure worldwide. The incidence and consequences of sleeve migration (SM) are not clearly understood. There is no clear consensus on appropriate measures to reduce the risk of SM. This study systematically reviewed the literature and identified 405 cases of SM from 21 studies. Age ranged from 18 to 68 years. Thirty-two percent and 11% of patients were females and males respectively, while sex was not reported in 57%. Time to diagnosis ranged from 1 day to 5 years postoperatively. A total of 9.6% and 58.8% of patients had or had no previous hiatal hernia respectively. SM incidence, risk factors, proposed mechanisms, clinical presentation, diagnosis, management, and potential preventive strategies are described in this review.
Graphical Abstract
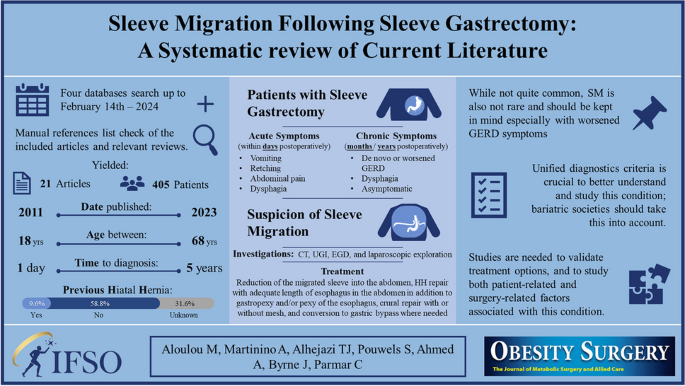
This is a preview of subscription content, log in via an institution to check access.
Access this article
Price includes VAT (Russian Federation)
Instant access to the full article PDF.
Rent this article via DeepDyve
Institutional subscriptions
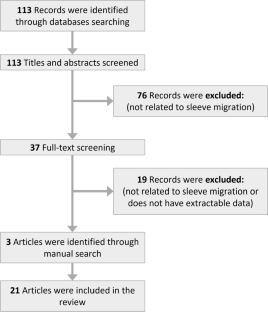
IFSO. 8TH global registry report. 2023. https://www.ifso.com/pdf/8th-ifso-registry-report-2023.pdf .
Baumann T, Grueneberger J, Pache G, Kuesters S, Marjanovic G, Kulemann B, et al. Three-dimensional stomach analysis with computed tomography after laparoscopic sleeve gastrectomy: sleeve dilation and thoracic migration. Surg Endosc. 2011;25(7):2323–9.
Article PubMed Google Scholar
Karila-Cohen P, Pelletier AL, Saker L, Laouénan C, Bachelet D, Khalil A, et al. Staple line intrathoracic migration after sleeve gastrectomy: correlation between symptoms, CT three-dimensional stomach analysis, and 24-h pH monitoring. Obes Surg. 2022;32(7):1–9.
Sabry K, Elmaleh HM, El-Swaify ST, Refaat MA, Atiya MAN, Alazab E, et al. Surgical management algorithm for intrathoracic sleeve migration: a retrospective series and literature review. J Laparoendosc Adv Surg Tech. 2022;32(10):1078–91.
Article Google Scholar
Saber AA, Shoar S, Khoursheed M. Intra-thoracic sleeve migration (ITSM): an underreported phenomenon after laparoscopic sleeve gastrectomy. Obes Surg. 2017;27(8):1917–23.
Oshiro T, Wakamatsu K, Nabekura T, Moriyama Y, Kitahara N. The potential of a polyglycolic acid sheet for preventing intrathoracic sleeve migration. Minim Invasive Ther Allied Technol. 2023;32(4):199–206.
Fouad MMB, Ibraheim SMN, Ibraheim P, Maurice KK, Saber AT. Assessment of the role of omentopexy in laparoscopic sleeve gastrectomy: a tertiary center experience. J Laparoendosc Adv Surg Tech. 2022;32(9):962–8.
Castagneto-Gissey L, Genco A, Del Corpo G, Badiali D, Pronio AM, Casella G. Sleeve gastrectomy and gastroesophageal reflux: a comprehensive endoscopic and pH-manometric prospective study. Surg Obes Relat Dis. 2020;16(11):1629–37.
Arnoldner MA, Felsenreich DM, Langer FB, Weber M, Mang T, Kulinna-Cosentini C, et al. Pouch volume and pouch migration after Roux-en-Y gastric bypass: a comparison of gastroscopy and 3 D-CT volumetry: is there a “migration crisis”? Surg Obes Relat Dis. 2020;16(12):1902–8.
Meknat A, Fernandez-Ranvier G, Hariri K, Guevara DE, Beitner M, Herron DM. Intrathoracic sleeve migrations after sleeve gastrectomy: a compilation of case reports. J Laparoendosc Adv Surg Tech. 2020;30(9):1013–7.
Amor Ben I, Debs T, Kassir R, Anty R, Amor Ben V, Gugenheim J. De novo hiatal hernia of the gastric tube after sleeve gastrectomy. Int J Surg Case Rep. 2015;15:78–80.
Bhandarkar S, Kalikar V, Nasta A, Goel R, Patankar R. Post-laparoscopic sleeve gastrectomy, intrathoracic sleeve migration and its management: a case series and review of literature. J Minim Access Surg. 2023;19(4):544–7.
Article PubMed PubMed Central Google Scholar
Boru CE, Termine P, Antypas P, Iossa A, Ciccioriccio CM, de Angelis F, et al. Concomitant hiatal hernia repair during bariatric surgery: does the reinforcement make the difference? Minerva Chir. 2021;76(1):33–42.
Google Scholar
Choi SJ, Kim SM. Intrathoracic migration of gastric sleeve affects weight loss as well as GERD–an analysis of remnant gastric morphology for 100 patients at one year after laparoscopic sleeve gastrectomy. Obes Surg. 2021;31(7):2878–86.
Dalkılıç MS, Erdem H, Gençtürk M, Yılmaz M, Sisik A. Acute intrathoracic migration with incarceration of laparoscopic sleeve gastrectomy due to incomplete hiatal hernia repair. Cureus. 2022;14(11):11–4.
Genco A, Soricelli E, Casella G, Maselli R, Castagneto-Gissey L, Di Lorenzo N, et al. Gastroesophageal reflux disease and Barrett’s esophagus after laparoscopic sleeve gastrectomy: a possible, underestimated long-term complication. Surg Obes Relat Dis. 2017;13(4):568–74.
Hon HH, Birriel TJ, El CM. Gastric sleeve migration conversion to a Roux-en-Y gastric bypass in combination with wedge fundectomy and paraesophageal hernia repair. Surg Obes Relat Dis. 2018;14(3):426–7.
Mizrahi I, Tabak A, Grinbaum R, Beglaibter N, Eid A, Simanovsky N, et al. The utility of routine postoperative upper gastrointestinal swallow studies following laparoscopic sleeve gastrectomy. Obes Surg. 2014;24(9):1415–9.
Pavelko Y, Bustos R, Gruessner S, Hassan C. Acute hiatal hernia with incarcerated proximal half of recent sleeve gastrectomy: super rare complication. Obes Surg. 2021;31(1):469–71.
Sebastiani S, Nagliati C, Barreca M. Gastric herniation following laparoscopic sleeve gastrectomy: a rare acute complication. Austin J Surg. 2014;1(9):1044.
Termine P, Boru CE, Iossa A, Ciccioriccio MC, Campanelli M, Bianciardi E, et al. Transhiatal migration after laparoscopic sleeve gastrectomy: myth or reality? A multicenter, retrospective study on the incidence and clinical impact. Obes Surg. 2021;31(8):3419–26.
Al-Sanea O, Al-Garzaie A, Dernaika M, Haddad J. Rare complication post sleeve gastrectomy: acute irreducible paraesophageal hernia. Int J Surg Case Rep. 2015;8:88–91.
Article PubMed Central Google Scholar
Alwatari Y, Roriz-Silva R, Bolckmans R, Campos GM. Intrathoracic sleeve gastrectomy migration with gastric volvulus treated with laparoscopic repair and conversion to gastric bypass. J Surg Case Reports. 2020;2020(8):1–4.
Parmar CD, Khalil H, Lakdawala M, Bhan C, Sufi P. Gastro-colic fistula after sleeve gastrectomy leak: our experience with this rare complication. Obes Surg. 2019;29(11):3771–2.
Stier C, Parmar C, Koschker AC, Bokhari M, Stier R, Chiappetta S. Computed tomography-3D-volumetry: a valuable adjunctive diagnostic tool after bariatric surgery. Mini-invasive Surg. 2020;4–18. https://doi.org/10.20517/2574-1225.2019.75 .
Parmar CD, Gan J, Stier C, Dong Z, Chiappetta S, El-Kadre L, et al. One Anastomosis/Mini Gastric Bypass (OAGB-MGB) as revisional bariatric surgery after failed primary adjustable gastric band (LAGB) and sleeve gastrectomy (SG): a systematic review of 1075 patients. Int J Surg. 2020;81(July):32–8.
Rheinwalt KP, Schipper S, Plamper A, Alizai PH, Trebicka J, Brol MJ, et al. Roux-en-Y versus one anastomosis gastric bypass as redo-operations following sleeve gastrectomy: a retrospective study. World J Surg. 2022;46(4):855–64.
Nakanishi H, Mosleh KA, Al-Kordi M, Farsi S, Chaudhry S, Marrero K, et al. One anastomosis gastric bypass as revisional surgery following sleeve gastrectomy: a systematic review and meta-analysis. Obes Surg. 2024;34(2):429–41.
Biter LU, ‘tHart JW, Noordman BJ, Smulders JF, Nienhuijs S, Dunkelgrün M, et al. Long-term effect of sleeve gastrectomy vs Roux-en-Y gastric bypass in people living with severe obesity: a phase III multicentre randomised controlled trial (SleeveBypass). Lancet Reg Heal Eur. 2024;38:100836.
Elmaleh HM, Elnabeel Mortada A, Khaled RA. Evaluation of anterior phrenoesophageal ligament preservation during hiatus hernia repair in laparoscopic sleeve gastrectomy as an anti-reflux measure. J Laparoendosc Adv Surg Tech. 2021;31(5):507–14.
Stier C, Chen W, Chong TH, Yang J, Parmar C, Wang C. Step-by-step instructions for the innovative three-port-technique of laparoscopic sleeve gastrectomy: how I do it? 2021;1–13. https://doi.org/10.21203/rs.3.rs-146710/v1 .
Skalli EM, Parmar CD. Necrosis of the Nissen-sleeve gastrectomy (N-SG) wrap. Obes Surg. 2020;30(10):4174–5.
Kermansaravi M, Parmar C, Chiappetta S, Shikora S, Aminian A, Abbas SI, et al. Best practice approach for redo-surgeries after sleeve gastrectomy, an expert’s modified Delphi consensus. Surg Endosc. 2023;37(3):1617–28.
Download references
Author information
Authors and affiliations.
Department of Surgery, Faculty of Medicine, University of Aleppo, Aleppo, Syria
Mohammad Aloulou
Nabd Alhayat Medical Center, Aleppo, Syria
Department of Surgery, Duke University, Durham, NC, USA
Alessandro Martinino
Faculty of Medicine, University of Aleppo, Aleppo, Syria
Tala Jouma Alhejazi
Departments of Surgery, Marien Hospital Herne, University Hospital of the Ruhr University Bochum, Herne, Germany
Sjaak Pouwels
Department of Surgery, Imperial College London, London, UK
Ahmed Ahmed
Department of Surgery, University Hospital Southampton, Southampton, UK
James Byrne
Department of Surgery, Whittington Hospital, London, UK
Chetan Parmar
Apollo Hospitals Education and Research Foundation, Apollo Hospitals, Chennai, India
University College London, London, UK
You can also search for this author in PubMed Google Scholar
Corresponding author
Correspondence to Mohammad Aloulou .
Ethics declarations
Competing interests.
The authors declare no competing interests.
Consent to Participate
Informed consent does not apply.
Additional information
Publisher's note.
Springer Nature remains neutral with regard to jurisdictional claims in published maps and institutional affiliations.
• SM should be a DDx after SG, especially in those with worsened GERD symptoms.
• Long-term follow-up after MBS is crucial to deliver the best possible patient care.
• Further studies are needed to validate the current treatment options for SM.
• Bariatric societies should work on a unified diagnostics criteria for SM.
Rights and permissions
Springer Nature or its licensor (e.g. a society or other partner) holds exclusive rights to this article under a publishing agreement with the author(s) or other rightsholder(s); author self-archiving of the accepted manuscript version of this article is solely governed by the terms of such publishing agreement and applicable law.
Reprints and permissions
About this article
Aloulou, M., Martinino, A., Alhejazi, T.J. et al. Sleeve Migration Following Sleeve Gastrectomy: A Systematic Review of Current Literature. OBES SURG (2024). https://doi.org/10.1007/s11695-024-07259-5
Download citation
Received : 04 March 2024
Revised : 18 April 2024
Accepted : 24 April 2024
Published : 04 May 2024
DOI : https://doi.org/10.1007/s11695-024-07259-5
Share this article
Anyone you share the following link with will be able to read this content:
Sorry, a shareable link is not currently available for this article.
Provided by the Springer Nature SharedIt content-sharing initiative
- Sleeve migration
- Sleeve gastrectomy
- Bariatric surgery
- Systematic review
- Find a journal
- Publish with us
- Track your research
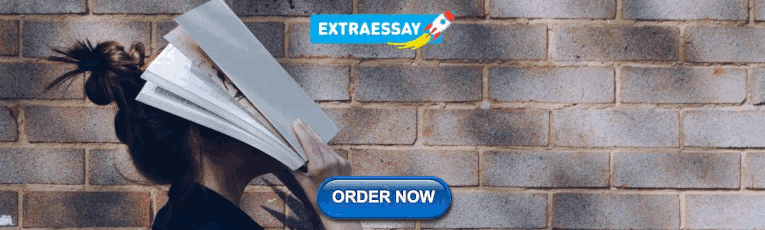
IMAGES
VIDEO
COMMENTS
This systematic review was performed to summarize the publications related to the obesity paradox in older adults, to gain an in-depth understanding of this phenomenon. PubMed©, Embase©, and Scopus© were used to perform literature search for all publications up to 20 March 2022.
There is a growing body of literature on potential methodological explanations for the obesity paradox, such as misclassification bias caused by using BMI as a measure of obesity, 1, 2 reverse ...
"Obesity paradox" describes the counterintuitive finding that aged overweight and obese people with a particular disease may have better outcomes than their normal weight or underweight counterparts. This systematic review was performed to summarize the publications related to the obesity paradox in older adults, to gain an in-depth understanding of this phenomenon. PubMed©, Embase©, and ...
The obesity paradox refers to extant evidence showing that obesity in older subjects or in patients with several chronic diseases may be protective and associated with decreased mortality. A number of mechanisms have been postulated to support the existence of obesity paradox; however, marked heterogeneity was found across studies and this has cast doubt on the actual presence of this phenomenon.
'Obesity Paradox' published in 'Encyclopedia of Gerontology and Population Aging' ... There is a large body of literature demonstrating the obesity paradox across of plethora of health conditions including heart disease, diabetes, kidney disease, cancer, and chronic obstructive pulmonary disease, with evidence suggesting it is a more common ...
Aging and obesity are two global concerns in public health. Sarcopenic obesity (SO), defined as the combination of age-related sarcopenia and obesity, has become a pressing issue. This systematic review and meta-analysis summarize the current clinical evidence relevant to SO.
The obesity paradox has been described in a plethora of chronic diseases. That said, it is important to note that despite the literature pointing towards an obesity paradox, there is very strong evidence supporting obesity's role in the pathogenesis of multiple diseases including CVD and is associated with reduced overall survival. The ...
More than 400 records were examined, with 150 being included in the present review. Literature provides evidence for an obesity paradox in a broad range of HF patients, including acute and chronic ...
This systematic review was performed to summarize the publications related to the obesity paradox in older adults, to gain an in-depth understanding of this phenomenon. PubMed©, Embase©, and Scopus© were used to perform literature search for all publications up to 20 March 2022. Studies were included if they reported data from older adults ...
literature suggests that the survival benefit associ-ated with higher body weight, the obesity para-dox,growsstrongerwithadvancingage,suchthat those aged 65 years with lower BMIs tend to have a greater number of comorbidities (Wang and Ren 2018). The Obesity Paradox in Clinical Populations The obesity paradox has been documented in a
Abstract. In contrast to the convincing evidence that obesity (measured by body mass index, BMI) increases the risk of many different types of cancer, there is an ambiguity in the role of obesity in survival among cancer patients. Some studies suggested that higher BMI decreased mortality risk in cancer patients, a phenomenon called the obesity paradox. The spurious positive association ...
Obesity is one of the most significant public health crises in the United States today. During the past 30 years, the prevalence of adult obesity (body mass index [BMI; calculated as kg/m 2] ≥30) has doubled from 15.0% to 32.2% (1, 2).Trends in children have shown an even more dramatic increase during this time: the overweight prevalence (BMI ≥95th percentile for age) has nearly tripled in ...
This review has highlighted the mixed findings in studies evaluating the obesity paradox in cancer populations. In terms of interpreting these studies, and designing future studies on this topic, there is a need to apply a methodological framework to determine whether the obesity paradox is a true or spurious relationship for a given setting.
There is a common perception that excess adiposity, commonly approximated by body mass index (BMI), is associated with reduced cancer survival. A number of studies have emerged challenging this by demonstrating that overweight and early obese states are associated with improved survival. This finding is termed the "obesity paradox" and is well recognized in the cardio-metabolic literature ...
Food insecurity is paradoxically associated with obesity in the United States. Current hypotheses to explain this phenomenon are descriptive regarding the low food security population's dietary and physical activity habits, but are not mechanistic. Herein it is proposed that a resource scarcity hypothesis may explain this paradox, such that fattening is a physiologically regulated response to ...
Obesity is a highly prevalent disease with numerous complications. Both intensive medical treatment with the use of pharmacological drugs and bariatric surgery are current options. The objective ...
By employing a comprehensive literature review, we examine the structural and functional modifications of the BBB in the context of obesity, including increased permeability, altered transport mechanisms, and inflammatory responses. ... Obesity paradox and stroke outcomes according to stroke subtype: a propensity score-matched analysis. Int J ...
During the past decade, rates of food insecurity and obesity have risen, and an association has been made between these two seemingly paradoxical states. Although this relationship has not been repeatedly seen in men, research suggests a correlation in women. Studies have not been able to consistently show a relationship in children, because findings differ based on age, race/ethnicity ...
Sleeve gastrectomy (SG) is the most frequently performed bariatric procedure worldwide. The incidence and consequences of sleeve migration (SM) are not clearly understood. There is no clear consensus on appropriate measures to reduce the risk of SM. This study systematically reviewed the literature and identified 405 cases of SM from 21 studies. Age ranged from 18 to 68 years. Thirty-two ...